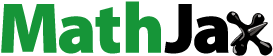
ABSTRACT
High-level liquid waste (HLLW) contains more than 30 kinds of nitrates which consist of fission products. A series of such phenomena as evaporation, melting and thermal decomposition of HLLW takes place within the ‘cold cap’, which is floating on top of the molten glass pool. An understanding of the varied thermal decomposition behavior of various nitrates constituting HLLW is necessary to elucidate a series of phenomena occurring within the cold cap. In this study, gadolinium nitrate, which is one of main compositions of HLLW, was chosen as an example of the nitrate salt and its thermal decomposition was investigated using non-isothermal thermogravitmetry-differential thermal analysis-mass spectrometry and X-ray powder diffraction. Ozawa method was applied for gaining kinetic parameters for the consecutive reaction of gadolinium nitrate. Reaction rate equation of the decomposition under non-isothermal condition was described.
1. Introduction
Thermal decomposition of metal nitrate has been the subject of many researches [Citation1–Citation3]. High-level Liquid Waste (HLLW) in nuclear fuel reprocessing contains more than 30 kinds of metal nitrate and the nitrate salts are thermally decomposed in glass melter. The decomposition reactions mainly occur in ‘cold cap’, formed on top of the layer in which both of HLLW and glass beas keep molten at 1200°C in the melter. Cold cap covering molten glass plays an important role as ‘cap’ that has big effect on heat transfer in the melter [Citation4]. Consequently, modeling of thermal decomposition reaction of nitrate salts in HLLW and those kinetic parameters are necessary for controlling cold cap formation and vitrification processes [Citation4,Citation5].
In previous study [Citation6], reaction rates of the thermal decomposition reaction of 15 kinds of nitrates, which are main constituents of simulated HLLW, were investigated using thermogravimetrical instrument over a range of room temperature to 1000°C. The overlapping of reaction rates for the nitrates was depicted according to composition ratio (wt%) of each nitrate in simulated HLLW. It was found that the thermal decomposition of simulated HLLW was able to predict by overlapping the reaction rates and reaction temperatures for the individual nitrates.
In this work, gadolinium nitrate, one of the main components in HLLW, was chosen as an example of the nitrate salt and its thermal decomposition rate was investigated using non-isothermal thermogravitmetry-differential thermal analysis-mass spectrometry (TG-DTA-MS) and X-ray powder diffraction (XRD). Several methods for describing thermal decomposition reaction kinetics of solid compounds, including apparent activation energy, frequency factor and mechanism function have been proposed such as Ozawa method [Citation7] and Kissinger–Akahira–Sunose (KAS) method [Citation8], etc. Ozawa method was applied for gaining kinetic parameters and mechanism function of the reaction rate equation. Reaction rate equation of the decomposition reaction was described as single equation by optimization of the parameters which depends on value of conversion ratio.
2. Experimental procedure
2.1. Materials and measurement
Gadolinium nitrate, Gd(NO3)3·6H2O (≥99.5%, Wako pure chemical), was used without further purification. Thermal decomposition reaction rate and gas products of gadolinium nitrate were investigated using TG (TGA-50, Shimadzu) and TG-DTA-MS(ThermoMass Photo, rigaku). Experiments for kinetic analysis were conducted under the heating rate of 1, 2, 5, 10°C min−1 in a temperature range of room temperature to 1000°C in N2 atmosphere at a rate of 75 cm3 min−1 by using TG. Differential thermal analysis and mass spectrometry of gas products were conducted under the heating rate of 10°C min−1 in a temperature range of room temperature to 1000°C in He atmosphere by using TG-MS (ThermoMass Photo, rigaku). Gadolinium nitrate and its thermal transformation products were identified using X-ray diffraction (D8 DISCOVER μHR, Bruker AXS), using Cu-Kα radiation.
2.2. Determination of the most probable mechanism function
The fundamental equation of chemical reaction rate can be described as a function of conversion α = (mini-mt)/(mini-mfin), where mini, mfin and mt are the weight at initial, final and a given time of sample mass, respectively:
where A (frequency factor [sec−1]), Ea (the apparent activation energy [kJ/mol]) are the Arrhenius parameters. R is the gas constant (8.314 J mol−1 K−1). f(α) is reaction mechanism function. For thermal decomposition reaction in constant temperature rising rate, [°C min−1], following reaction rate equation can be applied:
In above EquationEquation (4)(4)
(4) , log
is plotted against logβ at constant T and a linear regression of least square method were conducted (EquationEquation (4
(4)
(4) )). The slope of the straight line was closest to –1.000 and the linear correlation r2 should be nearly 1.000 when the most appropriate mechanism function was chosen.
2.3. Calculation and optimization of apparent activation energy and pre-exponential factor by iso-conversional procedure
In kinetic study, Ozawa equation was applied to calculate the values of the apparent activation energy, Ea, for the each decomposition reaction step of Gd(NO3)3 as follows:
Data from TG curves in the temperature range of reaction were used to determine at different temperature rising rates (
=1, 2, 5, 10°C min−1). Apparent activation energy,
, which depend on decomposition at different values of
, can be estimated from slope of the plot of
against 1/T (EquationEquation (5
(5)
(5) )). Frequency factor, A, was calculated from the intercepts of the lines using
and most probable mechanism function, g(α). Optimal A and Ea, which is independent on values of
, in each reaction step were obtained by least square method using EquationEquation (1)
(1)
(1) to fit experimental data.
3. Results and discussion
3.1. Solid and gas products during the thermal decomposition of gadolinium nitrate
Thermogravitmetry and differential thermal analysis curve of the thermal decomposition of gadolinium nitrate at a heating rate of 10°C min−1 in He atmosphere are illustrated in and mass spectrometry of released gas during the decomposition reaction is illustrated in . Three decomposition reactions were identified after dehydration [Citation9] in DTG and gas generation was detected in each temperature region. Nitrogen oxide (NO, NO2 or N2O), nitrogen and Oxygen were generated in first and second step of the reactions. In third step, nitrogen and oxygen were mainly observed. From the above results, it can be elucidated that the thermal decomposition takes place through following scheme:
Figure 1. TG curve and reaction rate of the thermal decomposition of Gd(NO3)3•6H2O at heating rate β of 10°C/min.
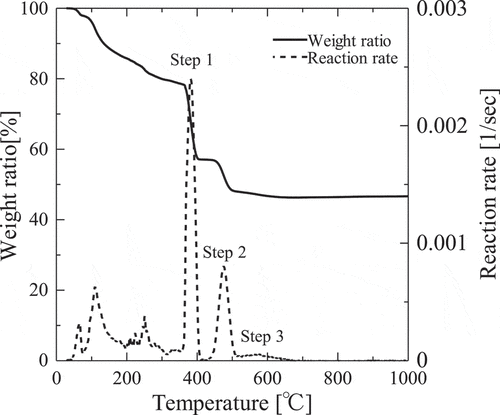
Figure 2. Mass spectrometry of the thermal decomposition of Gd(NO3)3•6H2O at heating rate β of 10°C/min.
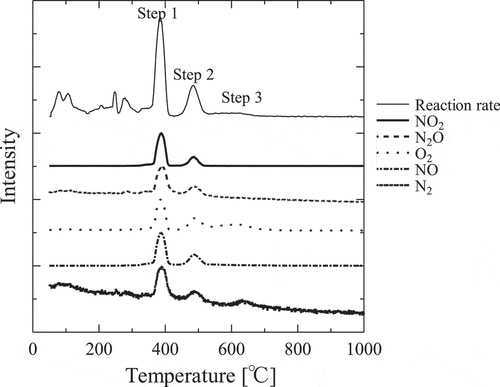
The XRD patterns of denitrate products (at 420, 700°C) are illustrated in . Denitration XRD patterns of dehydrated gadolinium suggested gadolinium oxide nitrate (GdO(NO3)) at 420°C and gadolinium oxide (Gd2O3) at 700°C. The morphology of gadolinium compound denitrated at 520°C couldn’t be identified, however, 9%-weight-loss in the temperature range of 400–500°C and Melnikov’s report [Citation9] support that gadolinium oxide nitrate was decomposed to the gadolinium compound which seems to be Gd4O5(NO3)2 in the temperature around 500°C, and it decomposes to gadolinium oxide in the temperature range around 600°C.
3.2. Kinetic parameter for the reaction of gadolinium nitrate
shows the TG curves in the temperature ranges of decomposition reaction of Gd(NO3)3, with four heating rates of 2, 5, 10, 20°C min−1 in N2 atmosphere. Temperature regions of three decomposition reaction steps of Gd(NO3)3, Gd(NO3)3 → GdO(NO3), GdO(NO3) (1st step) → Gd4O5(NO3)2 (2nd step) and Gd4O5(NO3)2 → Gd2O3(3rd step), in each heating rates are illustrated in . Data of α and T collected from the TG curves in each temperature ranges of decomposition reaction at various heating rates are listed in , and they were used to determine the kinetics parameters of three decomposition reaction steps, except dehydration, in all calculation procedures. According to EquationEquation (4)(4)
(4) combined with 13 conversion functions (), slope and linear correlation coefficient, r2, can be determined from plot of logg(α), which calculated different α values at the same temperature, against logβ. lists the results for all of the 13 types of mechanism functions. Function R2, A2 and A1 are the best suited for reaction step 1, 2 and 3.
Table 1. Temperature region of three decomposition reaction steps of gadolinium nitrate
Table 2. The α vs T data of thermal decomposition of gadolinium nitrate at different heating rates
Table 3. List of mechanism function
Table 4. The most probable mechanism function, slope and correlation coefficient of the reaction of gadolinium nitrate
Figure 4. TG curves of the thermal decomposition of Gd(NO3)3•6H2O at heating rate β of 2, 5, 10, 20°C/min.
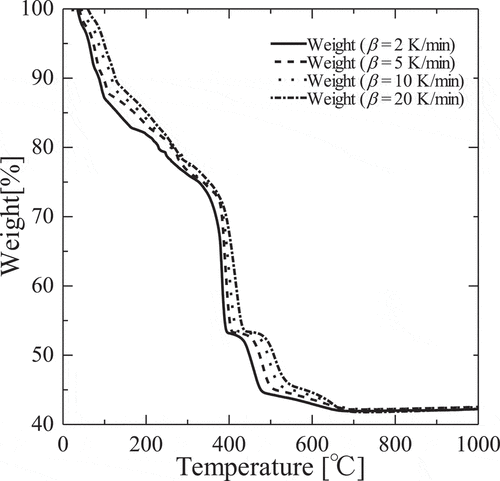
shows the plots of logβ versus 1/T (EquationEquation (5(5)
(5) )) for first step of decomposition process of gadolinium nitrate. The apparent activation energies, Ea, and frequency factor, A, which were calculated from the slope and intercepts of the lines using most probable conversion function, g(α), are tabulated in . The values of Ea and A depend on conversion ratio, α because of complexity of heterogeneous reaction. Optimized parameters are listed in and optimized results and experimental ones for thermal decomposition of gadolinium nitrate to gadolinium oxide are showed in . Calculated results are in fair agreement with the experimental ones. It can be seen that this method of optimization of reaction equation is available for complex reaction systems like heterogeneous thermal decomposition.
Table 5. The most probable mechanism function and kinetic parameters of thermal decomposition reaction of gadolinium nitrate
4. Conclusion
(1) Thermal decomposition reaction scheme of gadolinium nitrate to gadolinium oxide was confirmed as follows.
Gadolinium compound (which seems to be Gd4O5(NO3)2) Gd2O3
(2) Kinetic parameters and most probable conversion function of the reaction were calculated using thermogravimetric data and analytical approach (Ozawa method).
(3) Reaction rate equation of the each reaction was described as single equation successfully by optimization of frequency factor, A, and apparent activation energy, Ea, which depends on value of conversion ratio, α. Optimized equations are as follows.
Acknowledgements
This work is a part of the research supported by Japan Nuclear Fuel Limited with Grant-in-Aid by the Ministry of Economy, Trade and Industry. XRD measurement was provided by Center for Advanced Materials Analysis in Tokyo Institute of Technology.
Disclosure statement
No potential conflict of interest was reported by the authors.
References
- Bond BD, Jacobs MWP. The thermal decomposition of sodium nitrate. J Chem Soc. 1966;(A):1265–1268.
- Jankovic B, Mentus S, Jelic D. A kinetic study of non-isothermal decomposition process of anhydrous nickel nitrate under air atmosphere. Physica B. 2009;404:2263–2269.
- Melnikov P, Nascimento AV, Zanoni CZL. Thermal decomposition of gallium nitrate hydrate and modeling of thermolysis products. J Therm Anal Calorim. 2012;107:1117–1121.
- Pokorny R, Hrma P. Mathematical modeling of cold cap. J Nucl Mater. 2012;429:245–256.
- Schill P, Chmelar J. Use of computer flow dynamics in glass technology. J Non Cryst Solids. 2004;345&346:771–776.
- Kawai K, Fukuda T, Nakano Y, et al. Thermal decomposition analysis of simulated high-level liquid waste in cold-cap. EPJ Nuclear Sci Technol. 2016;44(2):1–7.
- Ozawa T, New A. Method of analyzing thermogravimetric data. Bull Chem Soc Jpn. 1965;38(11):1881–1886.
- Liqing L, Donghua C. Application of iso-temperature method of multiple rate to kinetic analysis. J Therm Anal Calorim. 2004;78:283–293.
- Melnikov P, Nascimento V, Consolo AZ. Computerized modeling of intermediate compounds formed during thermal decomposition of gadolinium nitrate hydrate. Russian J Phys Chem A. 2012;86(11):1659–1663.