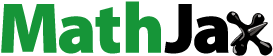
ABSTRACT
In a variety of highly enriched uranium cores of Kyoto University Critical Assembly, many different subcriticality measurements have been strenuously performed. However, any influence of neutron source inherent in the highly enriched uranium fuels on these measurements has hardly been studied. This is because the influence has been expected to be negligible in the fuels. In this study, we revaluated the influence on pulsed neutron, accelerator-beam trip and rod drop measurements to reveal an unexpected impact of the weak inherent source. Especially, the inherent source was injurious to most of the beam trip and the rod drop measurements based on the integral count method. The least-squares inverse kinetics analysis also had a significant influence on the inherent source. In the area ratio analysis for a pulsed neutron measurement, a considerable number of neutrons from the inherent source was mixed into delayed-neutron area. When the influence was considered in these data analyses, the subcritical reactivity of the above measurements was in good agreement with that calculated by the continuous-energy Monte Carlo code MVP.
1. Introduction
In a variety of highly enriched uranium cores of Kyoto University Critical Assembly (KUCA), these 10 years, many different subcriticality measurements have been strenuously performed. For pulsed neutron experiments using a 14 MeV pulsed neutron generator [Citation1] and a pulsed spallation neutron source [Citation2] with a high-energy proton accelerator [Citation3,Citation4], the extrapolated area ratio method [Citation5] and the Simmons & King method [Citation6] have been employed to determine the subcriticality of the various KUCA cores [Citation7–Citation14]. For accelerator-beam trip experiments based on the same measurement principle as source jerk experiment, the integral count method (technique) [Citation15] and the least-squares inverse kinetics method (LSIKM) [Citation16–Citation18] have been applied to obtain the subcriticality [Citation10,Citation19]. In reactor noise analyses for the pulsed 14 MeV and the pulsed spallation sources, the Feynman-α method [Citation20] on time domain and the power spectral technique [Citation21] on frequency domain have been used to obtain the prompt-neutron decay constant α and to determine the subcriticality from the decay constant [Citation10,Citation22–Citation26]. The rod drop experiment has been most frequently carried out to measure a reactivity worth of each control rod and to estimate a reference subcritical reactivity provided by the insertion of several rods from the total sum of the respective reactivity worth of the inserted rods, where the integral count method has been invariably employed in KUCA [Citation7,Citation27,Citation28].
Quite recently, Sakon et al. reported a very interesting observation in a University Training Reactor (UTR) [Citation29], where a neutron counter registered a considerable count rate originated from a neutron source inherent in highly enriched uranium fuels, even at the shutdown state. According to their report, a fuel plate of the UTR was made of an enriched uranium and aluminum alloy plate with a cladding, and the inherent neutron source of the fuel plate was generated from a significant number of (α, n) reactions between α particles emitted from radioactive decay of 235U and aluminum nuclides of base fuel material, but from only a few spontaneous fissions of 238U. The highly enriched fuel plate of KUCA is made of a similar uranium and aluminum alloy to that of the UTR. Therefore, all the highly enriched uranium cores must have a considerable inherent neutron source, and we are very worried about whether the considerable inherent source was injurious to the many previous subcriticality measurements or not. In all the highly enriched and most of low-enriched uranium cores, however, any influence of the inherent source on reactor-physics measurements has been never considered. This is because the influence has been blindly expected to be negligible in the zero-power uranium cores. The revaluation of the influence is an urgent task to improve the data quality of KUCA.
The main objectives of this paper are to confirm that neutron counters placed in a highly enriched uranium core of KUCA register a considerable count rate originated from the inherent source and to evaluate the influence of the source on pulsed neutron, accelerator-beam trip, and rod drop measurements. The further objectives are to consider the influence in the data analyses for these experiments and consequently to determine the subcriticality in a higher quality. In this paper, the reactor configuration and the experimental setups are presented in Section 2, the discussion of the experimental results in Section 3, and the conclusion of this study in Section 4.
2. Reactor configuration and experimental setups
2.1. Description of KUCA facility
shows a side view of a fuel assembly referred to as 1/8″P60EU-EU. Fuel and moderator plates of the assembly were set in a 1.5-mm-thick aluminum sheath, and the cross section of these plates within the assembly was the square of 2″ (50.8 mm). The fuel assembly consisted of 60 unit cells. The unit cell was composed of two (1/16″ thick) uranium plates with Al cladding and one (1/8″ thick) polyethylene plate. Each of the fuel assemblies had 120 sheets of the uranium plates. The active height of the core, namely 60 unit cells, was about 38 cm. Adjacent to both axial sides of the active region of each fuel assembly, about 56 cm upper and 55 cm lower polyethylene reflectors were attached, respectively. The core configuration employed in this study is shown in . The 25 fuel assemblies were loaded on locations (I-M,13–17) of the grid matrix shown in this figure, to constitute a reactor core. The core was surrounded by many polyethylene-reflector assemblies.
KUCA has six control rods, where three are assigned as safety rods for reactor scram and are referred to as S4 on location (L,12), S5 on (I,18) and S6 on (O,18), respectively. Others are rods for adjustment of reactivity and are referred to as C1 on (L18), C2 on (O,12) and C3 on (I,12), respectively. The solid-moderated core of KUCA has an emergency shutdown system to prevent any reactivity accidents. Several fuel and reflector assemblies assigned as the central fuel loading are downward withdrawn to shut down urgently the reactor operation. To the central fuel loading withdrawn by the emergency system, we assigned three fuel assemblies on locations (I,14–16) and six polyethylene-reflector ones on locations (G-H,14–16) of the grid matrix. The subcritical reactivity for the present experiment was adjusted by changing the axial position of the central fuel loading, as well as the positions of safety and control rods. These subcritical and critical patterns employed in the present experiments are shown in , where these respective reactivities calculated by the continuous-energy Monte Carlo code MVP (version 2.0) [Citation30] with the nuclear data library JENDL-4.0 [Citation31] are also included. For each of these patterns, 20,000 history × 10,000 batch were taken for the eigenvalue calculation. Validation of the MVP and the MCNP4C [Citation32] codes with the JENDL-3.2 [Citation33] and JENDL-3.3 [Citation34] libraries were demonstrated using benchmarks for fast and thermal subcritical cores [Citation35]. It is expected that the latest versions of these Monte Carlo codes with the latest nuclear library will be validated in a further ADS benchmark.
Table 1. Experimental patterns of control rods and central fuel loading
2.2. Nuclear instrumentation system and experimental conditions
A pulsed neutron generator was combined with the core, where pulsed 14 MeV neutrons were injected into the subcritical system through the polyethylene reflector. The generator consisted of a duoplasmatron-type ion source, a Cockcroft-Walton-type accelerator for deuteron beam and a tritium target of gas-in-metal type [Citation1]. The pulsed neutrons were generated through the D-T reaction between pulsed deuteron beam and tritium in the target metal. The target was placed on location (W,14–15) outside the polyethylene reflector, as shown in . The pulse width and the repetition period (repetition frequency) of the pulsed beam could be remotely controlled by using an arc-pulser installed at the control room of KUCA. As the repetition frequencies for the accelerator-beam trip and the pulsed neutron measurement, respectively, 500 Hz and 20 Hz were employed.
In KUCA, three fission counters (2″ dia.) were employed as reactor startup channel and three uncompensated ionization chambers (UIC1~ UIC3) were assigned as linear power, logarithmic power and safety power channel, respectively. These fission counters were also used for the present rod drop experiment, and the counters placed on locations (H-I,23–24), (H-I,6–7), and (A-B,11–12) are referred to as FC1, FC2, and FC3, respectively, as shown in . In addition to the above nuclear instrumentation for reactor operation, two BF3 proportional counters (1″ dia.) were employed as experimental channels. These BF3 counters on locations (J,11) and (J,19) are referred to as B1 and B2, respectively. The present nuclear instrumentation system consisted of conventional detector bias-supply, pre-amplifier, spectroscopy amplifier and discriminator modules. Finally, signal pulses from two BF3 and three fission counters were fed to a multi-channel scaler (MCS) to record a neutron decay behavior. In the pulsed neutron experiment, the MCS was repeatedly initiated by a trigger from the accelerator and neutron count decay data were successively accumulated for around 10 min. In the accelerator-beam trip and the rod drop experiments, time-sequence count data were acquired over 2 and 5 min before and after a beam trip and a rod drop operation, respectively.
3. Results and discussion
3.1. Inherent source strength and count rate originated from inherent source
Shiozawa et al. [Citation36] evaluated an inherent source strength of the highly enriched uranium fuel plate of KUCA. Twenty-five fuel assemblies of the present core have 120 × 25 sheets of the fuel plate, but the core of subcritical pattern D and E without the central fuel loading has 22 assemblies including 120 × 22 plate sheets. shows the inherent source strength of each fuel plate (quoted from the above reference), two whole cores with or without the central fuel loading. The contribution of (α, n) reaction to the inherent source strength is dominant in highly enriched uranium and aluminum alloy fuels of KUCA, as well as the UTR [Citation29].
Table 2. Inherent neutron source strength [neutrons/s]
The count rate of each neutron counter was measured in respective subcritical patterns without any external sources. shows a dependence of the count rate originated from the inherent source on the subcriticality calculated by the MVP code. Until the present day, the count rate originated from the source inherent in highly enriched uranium fuels has been expected to be negligible. However, the BF3 counters, which were placed close to the core region and sensitive to thermal neutron, had a higher count rate than expected. We were convinced that the considerable neutron counts originated from the inherent source should be considered in all subcriticality measurements of KUCA. However, we expected that a few count rates of fission counters, which were located far from the core, would have no need to be considered. But the present study indicates later that this expectation is wrong.
3.2. Influence on area ratio method for pulsed neutron experiment
shows neutron count decay behavior measured by counter B1, where the subcritical pattern is D. The conventional area ratio method [Citation37] determines the subcriticality from the ratio of an area to which prompt neutrons contribute to another area to which delayed neutrons contribute. When a transient count rate of prompt neutrons after pulse generation and another constant count rate of delayed neutrons are represented by Np(t) and Nd, respectively, the formula can be described as:
where Tp is repetition period of pulse generation and β is effective delayed-neutron fraction. The method is advantageous for no need of neutron generation time but suffers from a higher harmonics effect [Citation38,Citation39] observed immediately after pulse generation as shown in . To avoid a considerable error resulting from the above effect, the extrapolated area ratio method [Citation5] was proposed, and the formula could be described as:
where Tw is a time required to make the harmonics die out and α is the prompt-neutron decay constant. In the present study, the die-out time and the decay constant could be determined by applying the mask technique [Citation8,Citation10,Citation40]. The time and the decay constant are also shown in .
Here, we get down to the main issue of this study. includes a contribution of the inherent source to the accumulated neutron counts. The contribution was evaluated using the measured count rate originated from the inherent source. When the above formulae (1) and (2) were applied to the previous data analyses, a considerable number of neutrons from the inherent source was mixed into delayed-neutron area. The contribution of the inherent source never results from delayed neutrons generated by the pulsed neutron source and thus should be removed from the delayed-neutron area. The subcriticality obtained by the various area ratio methods for subcritical pattern D is shown in , where ‘Neglected’ and ‘Considered’ indicate whether the inherent-source contribution is mixed into the delayed-neutron area or is removed from the area. The inherent source has a considerable impact on the subcriticality analysis. Only the extrapolated area ratio method considering the inherent source has a consistent result with that calculation by the MVP code. In , the subcriticality obtained by the extrapolated area ratio method for each subcritical pattern is compared with the calculated result, whose statistical uncertainties are too small to be drawn as horizontal error bars in this figure as can be seen from . The conventional extrapolated area ratio method, where no influence of the inherent source is considered, has a considerable underestimation of the subcriticality for all patterns. However, the present analysis considering the inherent source has a consistent subcriticality with the calculation.
Table 3. Subcriticality obtained by various area ratio methods for a pulsed neutron experiment (subcritical pattern-D)
The delayed neutron data of the present reactor system are shown in . These data and the prompt-neutron generation time Λ of 2.8036 × 10−5 s were generated using the SRAC2006 code system [Citation41], where a three-dimensional, 107-energy-group diffusion calculation was done with the nuclear data library JENDL-4.0 [Citation31].
Table 4. Delayed neutron data of the present reactor system
3.3. Influence on data analyses for accelerator-beam trip experiment
shows neutron count decay behavior measured by counter B1 after a beam trip operation, where the beam is turned off at zero time, and the subcritical pattern is D. The time-sequence N(t) data are indicated as neutron counts accumulated during every 0.2 s. After the beam trip operation, the count data promptly drop and then decrease with the passage of time. However, the count rate never tends to zero and a residual background count is maintained. The background count is expected to be originated from the inherent source, and the inherent count is also included in this figure. The considerable background count should be taken into account in data analysis.
As data analysis method for the present beam trip experiment, both the integral count method [Citation15,Citation16] and the least-squares inverse kinetics method (LSIKM) [Citation16–Citation18] were employed. The detailed description of the previous application of these methods to beam trip experiment was given by Taninaka et al. [Citation19] and Sakon et al. [Citation10]. First, we discuss an influence of the inherent source on the integral count method.
3.3.1. Fatal influence on integral count method
The integral count method has been frequently employed to determine subcritical reactivity and rod reactivity worth from source jerk and rod drop experiment, respectively. When a neutron source is rapidly taken out of a subcritical reactor core or a control rod is suddenly dropped into a critical core at t = 0, the reactivity of the core can be expressed as
The above familiar formula was applied to the present beam trip experiment. Prior to a beam trip operation, the average count rate represented by N(0) was measured using a conventional counting scaler. Then, the integral count after the operation, which appeared in the denominator of the above equation, was also measured by the scaler. The denominator indicates that the counting time should be infinite; however, practically, the counting is terminated at several hundred seconds after the beam trip operation. Considering the non-negligible background count shown in , in the present experiment, this termination at a definite time T is expected to give a significant influence on the reactivity obtained. The reactivity must monotonously decrease and tend to zero with an increase in the termination time T. We also apply the following formula improved by Taninaka et al. [Citation16] to remove the background count.
where Nin represents a predetermined average count-rate originated from the inherent source.
shows a dependence of the subcriticality on the termination time T of integral for a beam-trip experiment at subcritical pattern D, where ‘Inherent Source Considered’ or ‘Neglected’ indicate whether Nin of EquationEquation (4)(4)
(4) is considered or neglected, respectively. Although a counting scaler had usually been used for the integral count method mentioned above, the time-sequence count data recorded by the MCS were employed for the method to observe the termination-time dependence. Neglecting the background count originated from the inherent source, as shown in this figure, the subcriticality monotonously decreased with an extension in the termination time and thus was estimated fatally smaller than the value 9.331 ± 0.015 calculated by the MVP code. Considering the background, however, the derived subcriticality had no decreasing trend in a range of the termination time beyond 100 s. All the subcriticalities within the range agreed mutually within statistical uncertainty ±1σ and became close to the calculated value. The application of EquationEquation (4)
(4)
(4) is successful in considering the inherent source.
3.3.2. Significant influence on least-squares inverse kinetics method
The least-squares inverse kinetics method (LSIKM) can determine a reactivity ρ and an effective source strength S of a subcritical system from the least-squares fit of the following equation:
where Q(t) is a time-dependent quantity on delayed-neutron emission rate and can be obtained by solving a discretized differential equation on the precursors [Citation16–Citation19]. When the LSIKM is applied to accelerator-beam trip and rod drop experiments, and when the subcritical system has no external source after these operations, the source strength is set to zero and the above equation is reduced to
EquationEquation (5)(5)
(5) was applied to the beam restart experiment [Citation19]; however, EquationEquation (6)
(6)
(6) was applied to all the previous beam trip and rod drop experiments in KUCA. Considering the non-negligible background count originated from the inherent source, we expected that EquationEquation (5)
(5)
(5) should be applied to the present experiment.
In , the time-sequence Q(t) and N(t) data are plotted on the X-Y coordinate, where the fitted broken and solid lines indicate whether respective lines are obtained by fitting EquationEquation (6)(6)
(6) or EquationEquation (5)
(5)
(5) . In the LSIKM, the reactivity of a subcritical system is determined from the gradient of these fitted lines. As shown in this figure, the gradients of these broken and solid lines are very different from each other and hence the derived reactivities are also expected to be very different. A chi-square value (6551) of the fitting of EquationEquation (5)
(5)
(5) is less than half of another chi-square value (14372) of the fitting of EquationEquation (6)
(6)
(6) and thus the former fitting is expected to be more successful than the latter.
The subcriticality obtained by the LSIKM for subcritical pattern D is shown in , where ‘Neglected’ and ‘Considered’ indicate whether EquationEquation (6)(6)
(6) or (Equation5
(5)
(5) ) is fitted, respectively. This table also includes the subcriticality calculated by the MVP. The application of EquationEquation (6)
(6)
(6) estimated the subcriticality significantly smaller than the calculated value. When EquationEquation (5)
(5)
(5) was applied to the least-squares fitting, the subcriticality of counter B1 was just a little larger than the calculated value, but that of counter B2 and the average of the two counters were consistent with the calculated value within the range of statistical uncertainty. In , the subcriticality obtained by the LSIKM for each pattern are compared with the calculated result. The application of EquationEquation (6)
(6)
(6) , where the influence of the inherent source is neglected, has a significant underestimation of the subcriticality for two patterns D and E. However, the fitting of EquationEquation (5)
(5)
(5) considering the inherent source leads to a consistent subcriticality with the calculation.
Table 5. Subcriticality obtained by LSIKM for a beam trip experiment (subcritical pattern-D)
3.4. Influence on data analyses for rod drop experiment
shows neutron count decay behavior measured by counter FC3 after a rod drop operation, where all safety and control rods were simultaneously dropped at zero time and the reactor system shifted from the critical state to the subcritical state of pattern C. After the rod drop, the neutron count promptly drops and then decreases slowly with the passage of time. However, the count of the fission counter never tends to zero, and a very few background counts are maintained. The background count is expected to be originated from the inherent source similar to the beam trip experiment but the background count of fission counters is much less than that of BF3 counters, as shown in . also includes the count level originated from the inherent source. The concern of us is not a difference in experimental method between rod drop and beam trip experiment, but another difference in background count rate between fission counter and BF3 counter. We try to consider how much the few background influences the data analysis.
3.4.1. Considerable influence on integral count method
First, we discuss an influence of the inherent source on the integral count method. shows a dependence of the subcriticality on termination time T of integral for the above all-rods drop experiment, where ‘Inherent Source Considered’ or ‘Neglected’ indicate whether Nin of EquationEquation (4)(4)
(4) is considered or not, respectively. Neglecting the very few background count of the fission counter, contrary to our expectations, the subcriticality monotonously decreased with an extension in the termination time of integral and thus was estimated considerably smaller than the calculated value 3.185 ± 0.015. Taking into account the background count originated from the inherent source, however, the derived subcriticality had no decreasing trend in a range of the termination time beyond 150 s. All the subcriticalities within the range agreed mutually within statistical uncertainty ±1σ and were consistent with the calculated value. Therefore, the application of the conventional formula to the present rod drop experiment fails, but that of EquationEquation (4)
(4)
(4) is so successful.
3.4.2. Negligible influence on least-squares inverse kinetics method
Next, we discuss an influence of the inherent source to the LSIKM for the present rod drop experiment. In , the time-sequence Q(t) and N(t) data are plotted on the X-Y coordinate, where the fitted broken and solid lines indicate whether respective lines are obtained by fitting EquationEquation (6)(6)
(6) or EquationEquation (5)
(5)
(5) . As shown in this figure, these lines almost overlap with each other and hence the reactivities derived from these LSIKM analyses have no significant difference between each other.
In addition to the above rod drop experiment (all safety and control rods dropped), another rod drop experiment was carried out, where three control rods were simultaneously dropped and consequently the reactor system shifted from the critical state to the subcritical state of pattern B. The subcritical reactivities obtained by the LSIKM for the two rod drop experiments are summarized in , where ‘Neglected’ and ‘Considered’ indicate whether EquationEquation (6)(6)
(6) or (Equation5
(5)
(5) ) is fitted, respectively. In contrast to the integral count method, the LSIKM has scarcely the influence of a few background counts of all fission counters. Instead of the integral count method, the LSIKM should be employed to avoid the influence of the few background count.
Table 6. Subcriticality obtained by LSIKM for a rod drop experiment
In a rod drop experiment, generally, a neutron counter, which looks at a dropped rod and a core in the same direction, has a direct influence of a spatial harmonics excited by the rod drop and overestimates the subcriticality [Citation28,Citation42]. In the all-rods drop experiment, as shown in , the fission counter FC1 looks at the dropped rod S5 and the core, and the FC2 looks at the dropped C3 and the core in the same direction. Consequently, as shown in , these FC1 and FC2 overestimate the subcriticality compared with the FC3, whose reactivity agrees very well with a value calculated by the MVP code. In the three-rods drop experiment, only the FC2 looks at the dropped C3 and the core in the same direction and consequently overestimates the subcriticality. The subcriticalities of the FC1 and FC3 agree very well with that done by the MVP.
4. Concluding remarks
In a highly enriched uranium core of the KUCA, we measured neutron counts originated from a neutron source inherent in the fuels. BF3 counters placed closely to the core had a higher count rate than expected. However, fission counters located far from the core registered a much less count rate than the above BF3 counters. We evaluated the influence of the inherent source on pulsed neutron, accelerator-beam trip, and rod drop measurements to reveal an unexpected impact of the inherent source. Especially, the inherent source was very injurious to most of the subcriticality measurements based on the integral count method. The least-squares inverse kinetics analysis also had a significant influence on the inherent source. In the area ratio analysis for a pulsed neutron measurement, a considerable number of neutrons from the inherent source was mixed into delayed-neutron area. When the influence was considered in these data analyses, the subcriticality derived from the above measurements was in good agreement with that calculated by the MVP code. This agreement demonstrates the validity of the MVP code with JENDL-4.0. In further subcriticality benchmarks, the MVP code with JENDL-4.0 and the prospective revised nuclear library should be validated.
We are convinced that some influence of the inherent neutron source on most of the subcriticality measurements should be considered even in highly enriched uranium core. In low-enriched uranium cores having the total uranium amount much more than that of highly enriched uranium cores, the influence is expected to be more important. It goes without mentioning that the influence is much more important in any mixed oxide and highly burnup cores including a very large amount of various inherent sources derived from plutonium and minor actinides. In a variety of low-enriched uranium cores of KUCA, this year, many different static and kinetic experiments have just started. We hope that some information and insight of this study will be effectively used in these experiments.
Acknowledgments
The present work was performed as a joint research program of the KUCA at the Institute for Integrated Radiation and Nuclear Science, Kyoto University.
Disclosure statement
No potential conflict of interest was reported by the authors.
References
- Ichihara C, Nakamura H, Kobayashi K, et al. [Characteristics of KUCA pulsed neutron generator]. Kumatori (Japan): Kyoto University Research Reactor Institute; 1983. ( (KURRI-TR-240) [in Japanese]).
- Pyeon CH, Misawa T, Lim JY, et al. First injection of spallation neutrons generated by high-energy protons into the Kyoto University Critical Assembly. J Nucl Sci Technol. 2009;46:1091–1093.
- Yonemura Y, Takagi A, Yoshii M, et al. Development of RF acceleration system for 150 MeV FFAG accelerator. Nucl Instrum Methds Phys Res A. 2007;576:294–300.
- Mori Y. Development of FFAG accelerators and their applications for intense secondary particle production. Nucl Instrum Methds Phys Res A. 2006;562:591–595.
- Gozani T. A modified procedure for the evaluation of pulsed source experiments in subcritical reactors. Nukleonik. 1962;4:348–349.
- Simmons BE, King JS. A pulsed neutron technique for reactivity determination. Nucl Sci Eng. 1958;3:595–608.
- Pyeon CH, Hervault M, Misawa T, et al. Static and Kinetic experiments on accelerator-driven system with 14 MeV neutrons in the Kyoto University Critical Assembly. J Nucl Sci Technol. 2008;45:1171–1182.
- Taninaka H, Hashimoto K, Pyeon CH, et al. Determination of lambda-mode eigenvalue separation of a thermal accelerator-driven system from pulsed neutron experiment. J Nucl Sci Technol. 2010;47:376–383.
- Shahbunder H, Pyeon CH, Misawa T, et al. Subcritical multiplication factor and source efficiency in accelerator-driven system. Ann Nucl Energy. 2010;37:1214–1222.
- Sakon A, Hashimoto K, Maarof MA, et al. Measurement of large negative reactivity of an accelerator-driven system in the Kyoto University Critical Assembly. J Nucl Sci Technol. 2014;51:116–126.
- Pyeon CH, Yagi T, Sukawa K, et al. Mockup experiments on the thorium-loaded accelerator-driven system at the Kyoto University Critical Assembly. Nucl Sci Eng. 2014;177:156–168.
- Pyeon CH, Nakano H, Yamanaka M, et al. Neutron characteristics of solid targets in accelerator-driven system with 100-MeV protons at Kyoto University Critical Assembly. Nucl Technol. 2015;192:181–190.
- Yamanaka M, Pyeon CH, Misawa T. Monte Carlo approach of effective delayed neutron fraction by k-ratio method with external neutron source. Nucl Sci Eng. 2016;184:551–560.
- Iwamoto H, Nishihara K, Yagi T, et al. On-line subcriticality measurement using a pulsed spallation neutron source. J Nucl Sci Technol. 2017;54:432–443.
- Hogan WS. Negative-reactivity measurements. Nucl Sci Eng. 1960;8:518–522.
- Taninaka H, Hashimoto K, Ohsawa T. An extended rod drop method applicable to subcritical reactor system driven by neutron source. J Nucl Sci Technol. 2010;47:351–356.
- Hoogenboom JE. van der Sluijs AR. Neutron source strength determination for on-line reactivity measurements. Ann Nucl Energy. 1988;15:553–559.
- Tamura S. Signal fluctuation and neutron source in inverse kinetics method for reactivity measurement in the sub-critical domain. J Nucl Sci Technol. 2003;40:153–157.
- Taninaka H, Hashimoto K, Pyeon CH, et al. Determination of subcritical reactivity of a thermal accelerator-driven system from beam trip and restart experiment. J Nucl Sci Technol. 2011;48:873–879.
- Feynman RP, De Hoffmann F, Serber R. Dispersion of the neutron emission in U-235 fission. J Nucl Energy. 1956;3:64–69.
- Nomura T. Reactivity measurements by reactor noise analysis using two-detector correlation method. J Nucl Sci Technol. 1966;3:14–19.
- Taninaka H, Miyoshi A, Hashimoto K, et al. Feynman-α analysis for a thermal subcritical reactor system driven by an unstable 14 MeV neutron source. J Nucl Sci Technol. 2011;48:1272–1280.
- Yamanaka M, Pyeon CH, Yagi T, et al. Accuracy of reactor physics parameters in thorium-loaded accelerator-driven system experiments at Kyoto University Critical Assembly. Nucl Sci Eng. 2016;183:96–106.
- Kong C, Choe J, Yum S, et al. Application of advanced Rossi-alpha technique to reactivity measurements at Kyoto University Critical Assembly. Ann Nucl Energy. 2018;118:92–98.
- Sakon A, Hashimoto K, Sugiyama W, et al. Power spectral analysis for a thermal subcritical reactor system driven by a pulsed 14 MeV neutron source. J Nucl Sci Technol. 2013;50:481–492.
- Sakon A, Hashimoto K, Sugiyama W, et al. Determination of prompt-neutron decay constant from phase shift between beam current and neutron detection signals for an accelerator-driven system in the Kyoto University Critical Assembly. J Nucl Sci Technol. 2015;52:204–213.
- Pyeon CH, Hirano Y, Misawa T, et al. Preliminary experiments on accelerator-driven subcritical reactor with pulsed neutron generator in Kyoto University Critical Assembly. J Nucl Sci Technol. 2007;44:1368–1378.
- Misawa T, Unesaki H, Pyeon CH. Nuclear reactor physics experiment. Kyoto (Japan): Kyoto University Press; 2010.
- Sakon A, Nakajima K, Hohara S, et al. Experimental study of neutron counting in a zero-power reactor driven by a neutron source inherent in highly-enriched uranium fuels. J Nucl Sci Technol.
- Nagaya Y, Okumura K, Mori T, et al. MVP/GMVP II: general purpose Monte Carlo codes for neutron and photon transport calculations based on continuous energy and multigroup methods. Tokai-mura (Japan): Japan Atomic Energy Research Institute; 2005. ( (JAERI 1348)).
- Shibata K, Iwamoto O, Nakagawa T, et al. JENDL-4.0: A new library for nuclear science and engineering. J Nucl Sci Technol. 2011;48:1–30.
- Briesmeister JF. MCNP – A general monte carlo N-Particle transport code version 4C. Los Alamos (United States): Los Alamos National Laboratory; 2000.((LA-13709-M)).
- Nakagawa T, Shibata K, Chiba S, et al. Japanese evaluated nuclear data library version 3 revision-2: JENDL-3.2. J Nucl Sci Technol. 1995;32:1259–1271.
- Shibata K, Kawano T, Nakagawa T, et al. Japanese evaluated nuclear data library version 3 revision-3: JENDL-3.3. J Nucl Sci Technol. 2002;39:1125–1136.
- Research committee on reactor physics. Activity report of working party on reactor physics of subcritical system. Tokai-mura (Japan): Japan Atomic Energy Research Institute; 2004. (JAERI-Review 2004-006) [in Japanese].
- Shiozawa T, Endo T, Yamamoto A, et al. Investigation on subcriticality measurement using inherent neutron source in nuclear fuel. In: Suyama K, Sugawara T, Tada K, et al., editors. Proceedings of the international conference on physics of reactors (PHYSOR 2014) September 28-October 3, 2014, Kyoto, Japan. Tokai-mura (JAPAN): Japan Atomic Energy Agency; 2015. (JAEA-Conf-2014-003 and 2014-003-appendix). doi: https://doi.org/10.11484/jaea-conf-2014-003.
- Sjostrand NG. Measurements on a subcritical reactor using a pulsed neutron source. Arkiv Fysik. 1956;11:233–246.
- Furuhashi A. Characteristic spectra in neutron thermalization. J At Energy Soc Jpn. 1962;4:677–684.
- Takahashi H. Space and time dependent eigenvalue problem in neutron thermalization. Italy: European Atomic Energy Community - EURATOM; 1962. ( (EUR-22.e)).
- Tonoike K, Miyoshi Y, Kikuchi T, et al. Kinetic parameter βeff/ℓ measurement on low enriched uranyl nitrate solution with single unit cores (600φ,280T,800φ) of STACY. J Nucl Sci Technol. 2002; 39 : 1227–1236.
- Okumura K, Kugo T, Kaneko K, et al. SRAC2006: A comprehensive neutronics calculation code system. Tokai-mura (Japan): Japan Atomic Energy Agency; 2007. ( (JAEA-Data/Code 2007-004)).
- Akino F, Yasuda H, Kaneko Y. Determination of large negative reactivity by integral version of various experimental methods. J Nucl Sci Technol. 1980;17:593–615.