ABSTRACT
In order to evaluate the accuracies of the kinetics parameters predicted with the continuous-energy Monte Carlo calculation code, MVP3, core analysis was performed for light-water moderated UO2 and mixed oxide (MOX) fuel cores for which the ratios of effective delayed-neutron fraction (βeff) to prompt-neutron life time (ℓ) – represented hereafter by βeff/ℓ – and βeff values were measured. The results obtained with the JENDL-4.0-based neutron library showed that (the calculation values (C)/the measurement values (E)−1) ranged from −1.0% to 4.0% for the βeff/ℓ values with an average of 1.0% and a standard deviation of 1.1% for the 17 cores (the UO2, and UO2-MOX mixed cores) tested in the Tank-Type Critical Assembly (TCA). With respect to the βeff of one UO2 core in TCA, C/E − 1 was 1.2%. For the βeff values of the UO2 and MOX cores tested in the EOLE critical facility, C/E − 1 was −1.5% for the former and −4.6% for the latter. The calculated βeff value of the MOX core using the JEFF-3.2-based neutron library was larger by 4% than that calculated using the JENDL-4.0-based neutron library and showed better agreement to the measurement.
1. Introduction
Effective delayed-neutron fractions (βeff) and prompt-neutron life times (ℓ) are basic kinetic parameters in controlling the nuclear behavior of reactor cores when they depart from the static state by insertion of reactivity. Accordingly, these kinetic parameters are involved in the safety analysis of nuclear power plants. While they can be directly measured, they are usually predicted with nuclear analysis codes. Therefore, it is important to evaluate the accuracies of the predicted results.
While deterministic analysis codes have traditionally been used for predicting kinetic parameters, stochastic analysis methods have also been developed since they are expected to provide more accurate results. Nagaya et al. [Citation1,Citation2] developed the eigenvalue method using the differential-operator sampling technique associated with the Monte Carlo perturbation techniques, and installed it in the continuous-energy Monte Carlo calculation code, MVP3 [Citation3]. Using the methods coupled with the neutron library based on JENDL-4.0 [Citation4], Nagaya [Citation2] validated both the methods and the library by analyzing the measured kinetic parameters for the experimental cores with various neutron-energy spectra. While these experimental cores widely covered bare spheres, spherical cores with a fertile reflector, heterogeneous fast cores, light-water moderated solution cores and light-water moderated lattice cores, the number of the light-water moderated lattice cores were limited to two cores.
The aim of this study is to evaluate the accuracy of kinetic parameters calculated with MVP3 by analyzing the measured kinetic parameters for light-water moderated lattice cores. The measured parameters included βeff/ℓ [Citation5] and βeff [Citation6,Citation7], which were obtained for the critical cores tested in the Tank-Type Critical Assembly (TCA) of the Japan Atomic Energy Agency and the EOLE critical facility (EOLE) of the French Atomic and Alternative Energies Commission. As a neutron library of MVP3, the library based on JENDL-4.0 [Citation4] was mainly used; the other libraries based on JENDL-3.3 [Citation8], ENDF/B-VII.1 [Citation9] and JEFF-3.2 [Citation10] were also examined.
This study adopted the calculation methods which are recommended by Nagaya et al. [Citation1,Citation2] to provide most precise results of the kinetic parameters for comparison with the measured results. In addition to these results, the calculation results with some other methods [Citation3] were also examined to discuss the accuracy of the methods installed in MVP3. Furthermore, the calculated effective neutron multiplication factors of the cores were also summarized in order to provide the comprehensive information relating to the reactivity calculations of MVP3.
Section 2 highlights the characteristics of the experimental cores which the kinetic parameters were measured for, Section 3 explains the analysis models and calculation conditions of MVP3, Section 4 presents the analysis results and discussions, and Section 5 mentions the conclusions. Appendix 1 is also provided to present the analysis results with the other methods in MVP3 for the kinetic parameters, and Appendix 2 shows the analysis results of the effective neutron multiplication factors.
2. Major characteristics of experimental cores for measuring kinetic parameters
summarizes the major characteristics of the experimental cores in TCA and EOLE for the measurements of the kinetic parameters. In , ‘Core ID’ represents the identifications of the cores in this study.
2.1. TCA cores
Tsuruta and Kitamoto [Citation5] performed the measurements of the βeff/ℓ values for the 17 light-water moderated cores in TCA with the pulsed-neutron technique. The cores were composed of UO2 fuel rods with enrichments of 2.6 wt% and 2.59 wt%, and MOX fuel rods with Pu contents of 3.4 wt% and 4.9 wt%. The major specifications of the fuel rods are listed in . It is evident that the isotopic compositions of 239Pu of the MOX fuel rods are roughly 90 wt%; moreover, the density of the pellets for the 3.4 MOX fuel rods is lower than those of the other pellets, since green pellets without sintering were used for the 3.4 MOX fuel rods.
Table 2. Major specifications of the UO2 and MOX fuel rods loaded in the TCA cores [Citation5].
The core configurations of TCA1 through TCA7 are the one-region cores of the UO2 fuel rods. Those of TCA8 through TCA17 are the two-region cores composed of a central core region of either the UO2 or MOX fuel rods and a driver region of the UO2 fuel rods. The one-region cores and the central and driver regions of the two-region cores were loaded with fuel rods in a homogeneous square lattice. The moderation (M/F) ratio of the fuel cells, which is defined as a moderator-to-fuel-pellet volume ratio, ranged from 1.50 to 2.96, which is consistent with typical M/F ratios of the fuel cells and fuel assemblies at the cold and hot operating conditions of commercial light-water reactor cores.
The series of the cores, TCA1, TCA2 and TCA7, were used to investigate the influence of the M/F ratios of the UO2 cores, and that fromTCA2 through TCA5 to study the influence of the boron concentrations in the moderator. The cores from TCA8 through TCA11 were the references for the cores from TCA12 through TCA15, which had the central MOX core regions (7 × 7 lattice) with the M/F ratios ranging from 1.66 to 1.96. The driver regions of the cores from TCA8 through TCA15 were composed of the 2.60 UO2 fuel rods with a M/F ratio of 1.82. TCA 16 and TCA 17 were used to examine the influence of the extended central MOX core regions (10 × 10 lattice) composed of the 4.9 MOX fuel rods. The core radial configurations of TCA1 through TCA17 [Citation5] are schematically shown in Figure S1 in the online supplemental material accompanying this manuscript.
The relative uncertainties (1σ) in the measured results ranged from 0.19% to 2.62% depending on the cores. They were obtained as the errors in the least-square fitting of the measured prompt neutron decay constants for the subcritical cores in order to determine that corresponding to the critical core [Citation5]. The measured results were compared with the calculated results, and the results of the comparison are shown in the later section.
The measured result of βeff for one UO2 core tested in TCA was originally obtained by Haga and Kobayashi [Citation11] using the substitution technique; it was later reevaluated by Nakajima [Citation6]. The major characteristics of the core is shown as TCA18 in . The specifications of TCA18 are similar to those of TCA2; however, the numbers of fuel rods, the critical water heights and the radial core shapes are slightly different. The core radial configuration of TCA18 is shown in Figure S2 in the online supplemental material accompanying this manuscript. The measured βeff is 7.71 × 10−3, and the relative uncertainty (1σ) is 2.2% [Citation6].
2.2 EOLE cores
The measured results of βeff for one UO2 core (MISTRAL1) and one MOX core (MISTRAL2) tested in EOLE were analyzed in this study. The measurements were performed with the noise-analysis technique [Citation7] as a part of the MISTRAL experimental program [Citation12]. MISTRAL1 was composed of 3.7 wt% UO2 fuel rods, and MISTRAL2 was composed of MOX fuel rods with Pu contents of 7.0 wt% and 8.7 wt%. The fuel rods were PWR-type test fuel rods with an effective fuel length of 80 cm. They were covered by over-claddings of aluminum alloy (AG3), which were used to adjust the M/F ratio of the cores to about 1.8. The enrichment of the UO2 fuel rods and the Pu contents of the MOX fuel rods are relatively higher than those of the TCA cores; moreover, they are closer to those used in current commercial light-water reactors. The core radial configurations of MISTRAL1 and MISTRAL2 are shown in Figure S2 in the online supplemental material accompanying this manuscript. The relative uncertainties (1σ) in the measured results were 1.5% for MISTRAL1 and 1.6% for MISTRAL2. They were obtained considering the uncertainties in the Diven factor, the fission integral of the core, the spectral density of interaction power, and the average voltages of fission chamber amplifiers [Citation7]. The measured results were compared with the calculated results and the comparison results are shown in the later section.
3. Analysis models and calculation conditions of MVP3
Based on the specifications of the fuel rods and the structure materials of TCA [Citation5,Citation13–Citation15], the three-dimensional geometrical models of the TCA cores were composed of the fuel rods, water, core grids, structure materials and a core tank (an outer diameter of 184 cm and a height of 208 cm). The boundaries of the outside of the core tank were assumed to be in a vacuum condition. The modes of the vertical cross sections of the TCA cores are schematically shown in Figure S3 in the online supplemental material accompanying this manuscript.
The three-dimensional geometrical models of MISTRAL1 and MISTRAL2 were composed according to the procedure in Reference [Citation16], where the core radial geometrical model included the core and water reflector inside the core tank (an inner diameter of 94 cm). The core axial geometrical model consists of six regions. For the fuel rods, in addition to the effective fuel part, the model included a fuel spacer and a spring in the top part of the fuel rod, as well as a fuel spacer in the bottom part of the fuel rod. Water reflectors (a length of 5 cm) were placed at the outsides of the fuel rods. The two intermediate grids, which were located outside of the effective fuel section in the axial direction, and the top and end grids were not included in the model. The modes of the MISTRAL cores [Citation16] are schematically shown in Figure S4 in the online supplemental material accompanying this manuscript. Some studies [Citation17,Citation18] demonstrated that the differences in neutron multiplication factors (keff) between the models with and without the grids were comparable to the statistical uncertainty in the calculations, 0.02%Δkeff, and negligibly small.
The calculations with MVP3 for the TCA and MISTRAL cores were performed using the neutron library based on JENDL-4.0 [Citation4] for 100 inactive and 5,000 active batches with 40,000 histories per batch. Accordingly, the calculations provided the results of βeff, ℓ and βeff /ℓ calculated with the eigenvalue method using the differential-operator-sampling technique associated with the Monte Carlo perturbation technique [Citation1,Citation2]. The adopted values in this study were those obtained by taking into account the indirect-perturbed-source effect [Citation1]. Under the neutron history conditions, the statistical uncertainties (1σ) of βeff /ℓ and βeff ranged from 0.3% to 0.4%, which are comparable to the measurement uncertainties or lower than them, while those of ℓ ranged from 0.03% to 0.07%, which were considerably smaller than those of βeff/ℓ and βeff . The statistical uncertainties of keff ranged from 0.005%Δkeff to 0.006%Δkeff. The neutron libraries based on JENDL-3.3 [Citation8], ENDF/B-VII.1 [Citation9], and JEFF-3.2 [Citation10] were also partly used for examining the sensitivity of βeff /ℓ and βeff to nuclear-data libraries.
Since the MVP3 calculations provide the results of kinetic parameters with some other methods [Citation3] in addition to the above-mentioned methods recommended by Nagaya [Citation1,Citation2] for the most accurate calculation, the calculation results with the other methods were also examined and the results are presented in Appendix 1.
4. Analysis results and discussions
4.1. Calculated results of βeff and ℓ
The results of βeff and ℓ in the MVP3 calculations coupled with the JENDL-4.0-based neutron library are shown in for all the cores.
The following paragraphs involve a brief discussion on the trends in the kinetic parameters relating to the core characteristics.
Since the delayed neutron fractions in the total numbers of fission neutrons (β) for major nuclides contributing to fissions in nuclear fuel in light-water reactor cores are considerably different. The β values are 6.507 × 10−3 (at 0.0253 eV) for the thermal fission of 235U, 2.161 × 10−3 (at 0.0253 eV) for the thermal fission of 239Pu and 1.762 × 10−2 (at 2.0 MeV) for the fast fission of 238U, according to JENDL-4.0 [Citation4]. Therefore, the values of βeff mainly depend on the fuel compositions within the cores. While the fractional contributions of 238U to fissions are roughly 5% for the TCA cores, and while their differences between the cores are relatively small, the fractional contributions of 239Pu vary according to the fractions of the MOX fuel rods in the cores. Moreover, because the β value of 239Pu is smaller than that of 235U, the βeff values of the cores from TCA12 through to TCA17 are smaller than those of the other UO2 cores. Since MISTRAL2 was fully loaded with MOX fuel rods, the βeff value is the smallest among all the cores.
The ratio of the leakage of prompt neutrons from the cores to that of delayed neutrons also has effect on βeff, and therefore, the core volumes are also major factors to affect the βeff values. The systematic trend of slightly decreasing βeff values from TCA2 through to TCA6 is the result of the core volume increasing in order to compensate for the negative reactivity caused by the boron-concentration increase in the moderator [Citation5].
The ℓ values depend on macroscopic absorption cross sections of the cores which are sensitive to fuel and moderator conditions Comparison of the calculated ℓ values of TCA1, TCA2 and TCA7 indicates that the values increase with the M/F ratios. The calculated ℓ values of the cores from TCA2 through TCA6 decrease with the content of boron in the moderator. The differences in the core configurations of TCA8 through TCA11 are related to the M/F ratios in the central core regions. In addition, there are the effect of the water-gap widths between the central core regions and the driver regions as indicated by Tsuruta and Kitamoto [Citation5]. The gaps varied: 0 cm for TCA8, 1.787 cm for TCA9, 1.454 cm for TCA10 and 0 cm for TCA11. Since the central core regions consisted of the small 7 × 7 lattice, the effect of changing the water-gap width was relatively large with respect to the ℓ values. The ℓ values of TCA12 through TCA15, which were partially loaded with MOX fuel rods, are smaller than those of the cores from TCA8 through TCA11. This is because the MOX fuel rods have relatively large thermal-neutron-absorption cross sections. The effect of the changes in the water-gap widths was similar to that of TCA8 through TCA11. For TCA16 and TCA17, the central core regions of the MOX fuel rods (the 10 × 10 lattice) are larger than those of TCA12 through TCA15. Moreover, the water-gap width of TCA17 is larger than that of TCA16; accordingly, the ℓ values for those cores are smaller than those of the cores TCA12 through TCA15; moreover, the ℓ value of TCA17 is slightly larger than that of TCA16. The ℓ value of MISTRAL1 is smaller than that of TCA18 since the enrichment (3.7 wt%) of the UO2 fuel for MISTRAL1 is larger than that of TCA18 (2.6 wt%). Since the absorption cross sections of the MOX fuel are larger than those of the UO2 fuel, the ℓ value of MISTRAL2 is smaller than that of MISTRAL1.
4.1.1. Comparison of calculated and measured βef /ℓ values
The calculated results of βeff/ℓ with respect to the TCA cores were compared with the measured results. The comparison results are shown in and , represented by (the calculation values (C)/the measurement values (E)−1)%. Accordingly, the uncertainties (1σ) in C/E-1 are composed of the associated uncertainties in the calculated and measured results. The error bars in with the MVP3 calculation results show the range of uncertainties in C/E-1. While the uncertainties in the calculations range from 0.30% to 0.36% and their deviations are relatively small, those in the measured results ranged from 0.19% to 2.62% and their deviations are relatively large. The calculated results of this study generally agreed to the measurements even though the characteristics of the cores vary in terms of M/F ratios, boron concentrations, and fractions of MOX fuel rods in the cores. They might have slightly overestimated the measurements. After all, the average value of C/E-1 was 1.0% with a standard deviation of 1.1%. The largest overestimation was seen for TCA6 which is the core with the largest boron content (554 ppm) in the moderator and the largest underestimation was seen for TCA7 which is the UO2 core with the largest M/F ratio. In the scope of this study, correlations of C/E-1 with the boron concentrations and the M/F ratios were not confirmed.
Table 3. Comparison of the calculated and measured βeff/ℓ values in C/E-1% for the TCA cores.
Figure 2. Comparison of the calculated and measured βeff/ℓ values in C/E-1% for the TCA cores. This figure shows the results of this study (MVP3 + JENDL-4.0) and those of Tsuruta and Kitamoto [Citation5]. The error bars for this study show the range of the uncertainties (1σ) in C/E-1 composed of those in the calculated and measured results.
![Figure 2. Comparison of the calculated and measured βeff/ℓ values in C/E-1% for the TCA cores. This figure shows the results of this study (MVP3 + JENDL-4.0) and those of Tsuruta and Kitamoto [Citation5]. The error bars for this study show the range of the uncertainties (1σ) in C/E-1 composed of those in the calculated and measured results.](/cms/asset/76752108-2b71-4ae0-95b7-de0d260774a5/tnst_a_1727375_f0002_oc.jpg)
The calculated results of Tsuruta and Kitamoto [Citation5], which were reported for some of the cores, are also shown in and . Their calculation results were obtained using deterministic calculations in two-dimensional geometry and four neutron-energy groups. The used codes were UGMG42 for the fast-energy group constants, THERMOS for the thermal-energy group constants, KAK for the diffusion calculations and 2DPERT for the βeff and ℓ calculations. The neutron leakage in the axial direction was taken into account by using the axial buckling. Their calculation results were slightly lower than those obtained in this study; however, the general trend of C/E-1 is similar to this study. Their largest discrepancies were also observed for TCA6 and TCA7.
4.1.2. Comparison of calculated and measured βeff values
The comparison of the calculated results obtained with the MVP3 calculations in this study and the measured results is shown in . As before, the uncertainties (1σ) in C/E-1 are composed of the uncertainties from the calculated and measured results. The calculation of βeff for TCA18 has previously been performed by Nakajima [Citation6] with the deterministic code TWODANT in the 107 neutron-energy groups in combination with the neutron library based on JENDL-3.2 [Citation19], as well as Nagaya [Citation2] with MVP-2 [Citation20] modified to include the eigenvalue method using the differential-operator-sampling technique. The calculated results of Nakajima [Citation6] overestimated the measurement by 2.9%, thereby exceeding the measurement uncertainty of 2.2%. Nagaya [Citation2] reported 7.80 × 10−3 for βeff, which agreed to 7.803 × 10−3 of this study as expected. The deviations of the results from the measurement were within the uncertainty in C/E-1.
Table 4. Comparison of the calculated and measured βeff values in C/E-1%.
The calculated result of MISTRAL1 in this study showed a slight underestimation; however, the deviation from the measurement was within the uncertainty in C/E-1. The value of C/E-1 for MISTRAL2 was −4.61%; the deviation of the calculated result exceeded the uncertainty in C/E-1. also shows the C/E-1 values for the MISTRAL cores in the references [Citation7,Citation12,Citation17]. Litaize et al. [Citation7] analyzed MISTRAL1 and MISTRAL2 with the deterministic code, APOLLO2.5, in combination with the CEA93.V6 library based on the nuclear-data library, JEF2.2. Hibi et al. [Citation12] analyzed the same core with the deterministic codes, SRAC-CITATION [Citation21], in combination with the neutron library based on JENDL-3.2 [Citation19] using a three-dimensional geometrical model and the 21 neutron-energy groups. The results of the Nuclear Power Engineering Corporation (NUPEC) [Citation17] were obtained with the same methods as Hibi et al. [Citation12], with JENDL-3.2 replaced by JENDL-3.3 [Citation8]. While the results of Hibi et al. [Citation12] and NUPEC [Citation17] are similar to those of this study with respect to MISTRAL1 and MISTRAL2, those of Litaize et al. [Citation7] are slightly larger for MISTRAL1; however, the results of Litaize et al. shows better agreement with the measurement of MISTRAL2 than the any other analysis.
4.1.3 Sensitivity of kinetics parameters to nuclear-data libraries
The differences in C/E-1 for the measured kinetic parameters are the result of the uncertainties in the analysis codes and the nuclear-data libraries on which the neutron libraries of the codes are based. Since the calculations of the kinetic parameters with MVP3 adopt the detailed-physics models in order to exclude uncertainties in the physics models of the deterministic calculation codes, they are expected to provide information on the uncertainties in the nuclear-data libraries. Therefore, the results of C/E − 1 of this study can be attributed to biases in the nuclear data, such as the β values and microscopic-absorption cross sections in JENDL-4.0 for major contributing nuclides. For the future updating of the nuclear data in JENDL-4.0, it would be useful to provide the analysis results of βeff and ℓ for the experimental cores obtained with the neutron libraries based on JENDL-3.3 [Citation8], ENDF/B-VII.1 [Citation9], and JEFF-3.2 [Citation10]. The calculations were performed for TCA1, TCA6 and TCA17 for which the measured βeff/ℓ values were available, and for TCA18, MISTRAL1 and MISTRAL2 for which the measured βeff values were available. The calculation models and conditions were the same as those mentioned in Section 3.
shows the calculated results of βeff, ℓ and βeff/ℓ of the JENDL-4.0-, JENDL-3.3-, ENDF/B-VII.1- and JEFF-3.2-based neutron libraries and the comparison results to the values of the JENDL-4.0-based neutron library, which are shown as (βeff /JENDL-4.0 − 1), (ℓ/JENDL-4.0 − 1) and (βeff/ℓ/JENDL-4.0 − 1): (the calculated result with the other neutron library/that with the JENDL-4.0-based neutron library−1)%.
Table 5. Calculated results of βeff, ℓ and βeff /ℓ with the JENDL-4.0-, JENDL-3.3-, ENDF/B-VII.1- and JEFF-3.2-based neutron libraries and the ratios to those obtained with the JENDL-4.0-based neutron library.
The differences in the calculated results of βeff between the JENDL-3.3-based neutron library and the JENDL-4.0-based neutron library are less than 0.6%, and, for the ENDF/B-VII.1-based neutron library, the differences are less than 1.1%, while the calculated results of the JEFF-3.2-based neutron library are larger than those of JENDL-4.0, and the differences range from 2.1 to 3.6%. The differences in the calculated results of ℓ between the JENDL-3.3-based neutron library and the JENDL-4.0-based neutron library are less than 0.3%; for the ENDF/B-VII.1-based neutron library, the differences are less than 0.7%; finally, for the JEFF-3.2-based neutron library, the differences are less than 0.4%. The differences in the calculated results of βeff/ℓ between the JENDL-3.3-based neutron library and the JENDL-4.0-based neutron library are less than 0.8%, and, for the ENDF/B-VII.1-based neutron library, the differences are less than 1.2%, while the calculated results of the JEFF-3.2-based neutron library are larger than those of JENDL-4.0, and the differences range from 2.5 to 3.9%. The differences in the calculated results of βeff/ℓ between the nuclear-data libraries are mainly caused by the differences in the βeff values.
For further study, the β values of 235U, 239Pu and 238U for the thermal fission or the fast fission in ENDF/B-VII.1 [Citation9] and JEFF-3.2 [Citation10] were examined since the βeff values obtained with the neutron libraries based on them showed noticeable differences compared with those of JENDL-4.0 [Citation4]. They are shown in together with the ratios to those of JENDL-4.0 [Citation4]. As seen in , the β value of 235U in ENDF/B-VII.1 is close to that of JENDL-4.0, and that of 239Pu is 4% larger than that of JENDL-4.0, while that of 238U in ENDF/B-VII.1 are 5% smaller than that of JENDL-4.0. The smaller β value of 238U of ENDF/B-VII.1 explains that the βeff and βeff/ℓ values of the UO2 cores (TCA1, TCA6, TCA18 and MISTRAL1) for the ENDF/B-VII.1-based library are slightly smaller than those for the JENDL-4.0-based library. The trend that while the βeff and βeff/ℓ values of the MOX cores (TCA17 and MISTRAL2) for the ENDF/B-VII.1-based library are slightly larger than those for the JENDL-4.0 is caused by the combination of the larger β value of 239Pu and the smaller β value of 238U.
Table 6. βvalues of 235U, 239Pu, and 238U for JENDL-4.0 [Citation4], ENDF/B-VII.1 [Citation9], JEFF-3.2 [Citation10], and JEFF-3.3 [Citation22].
shows that the β values in JEFF-3.2 are larger than those of JENDL-4.0 by 2% for 235U, 5% for 239Pu, and 6% for 238U. This explains the differences in the βeff and βeff/ℓ values between the calculations with the JEFF-3.2- and JENDL-4.0-based neutron libraries for the TCA and MISTRAL cores. In , the β values in JEFF-3.3 [Citation22] are also shown for comparison with those of JEFF-3.2. The β value of 235U for JEFF-3.3 are 1% larger that of JEFF-3.2, and that of 239Pu is close to that for JEFF-3.2 while that of 238U is 2% smaller than that for JEFF-3.2. Accordingly, the JEFF-3.3-based neutron library is expected to provide similar calculation results of the βeff values to those of JEFF-3.2.
shows the values of (C/E − 1) when the JENDL-3.3-, ENDF/B-VII.1- and JEFF-3.2-based neutron libraries are adopted for comparison with the measured values. As for TCA1, the (C/E − 1) value of the ENDF/B-VII.1-based library is smaller than those of the JENDL-4.0- and JENDL-3.3-based libraries. This is attributed to the smaller β value of 238U for ENDF/B-VII.1. The (C/E − 1) value for the JEFF-3.2-based library is larger than those for the other neutron libraries. This is caused by the larger β values of 235U, and 238U for JEFF-3.2. As for the relatively larger (C/E − 1) values of TCA6, further sturdy including on the measured value is necessary. Concerning TCA17 (a partially-MOX-loaded core), the (C/E − 1) value of the ENDF/B-VII.1-based library is slightly larger than those of the JENDL-4.0- and JENDL-3.3-based libraries. This is caused by the larger β value of 239Pu in ENDF/B-VII.1.
Table 7. Comparison of the calculated and measured βeff/ℓ and βeff values in C/E − 1% for the MVP3 calculations with the JENDL-4.0-, JENDL-3.3-, ENDF/B-VII.1- and JEFF-3.2-based neutron libraries.
With respect to TCA18, the trend in the (C/E − 1) values are similar to those of TCA1. The (C/E − 1) values for ENDF/B-VII.1, JENDL-4.0, and JEFF-3.1.1 [Citation23] have reported for TCA18 by van der Marck [Citation24], who analyzed the core with the continuous-energy Monte Carlo calculation code, MCNP6 [Citation25]. The (C/E − 1) values for JENDL-4.0, and JEFF-3.2 in this study accord with those for JENDL-4.0, and JEFF-3.1.1 by van der Marck [Citation23], respectively. The (C/E − 1) value for ENDF/B-VII.1 in this study agrees to that by van der Marck [Citation23] considering the statistical uncertainties of the calculations. For the measured βeff values of MISTRAL1, the calculation result of the JEFF-3.2-based neutron library is close to the measurement while those of the JENDL-4.0- and JENDL-3.3-based neutron libraries show underestimation of 1%, and that of the ENDF/B-VII.1-based neutron library also shows underestimation of 3%. This trend is explained by the differences in the β values of 235U and 238U for the libraries as mentioned before. For the measurement of MISTRAL2, the calculation result of JEFF-3.2-based neutron library shows better agreement to the measurement than those of the other neutron libraries. This trend is explained by the larger β values of 235U, 239Pu and 238U for JEFF-3.2 compared with the other libraries.
5. Conclusions
The analysis of the measured βeff/ℓ values for the 17 TCA experimental cores indicated that the calculated results of MVP3 coupled with the JENDL-4.0-based neutron library are, generally speaking, in agreement with the associated measurements, and the average value of (C/E-1) are 1.0% with a the standard deviation of 1.1%. According to the sensitivity study with respect to the other nuclear-data libraries, the analysis results coupled with the JENDL-3.3 and ENDF/B-VII.1-based neutron libraries demonstrated similar accuracies to those of JENDL-4.0. Moreover, the analysis results coupled with the JEFF-3.2-based neutron library overestimated the measurements by more than 4%.
The calculated results of MVP3 coupled with the JENDL-4.0-based neutron library agreed to the measured βeff values of TCA18 (UO2 core) and MISTRAL1 (UO2 core), considering the uncertainties composed of the measurement and calculation uncertainties, while they underestimated by 5% the measured one of the MISTRAL2 (MOX core). The analysis results coupled with the JENDL-3.3- and ENDF/B-VII.1-based neutron libraries showed comparable trend as those with JENDL-4.0 for TCA18 and MISTRAL1. The analysis results with JEFF-3.2 overestimated the measurement of TCA18, while they agreed to the measurements of MISTRAL1 UO2 and MISTRAL2. The sensitivity study on the nuclear-data libraries indicated that these differences in the (C/E − 1) values between the neutron libraries were attributed to the differences in the β values of 235U, 239Pu, and 238U of the nuclear-data libraries on which the neutron libraries of MVP3 based.
The calculations of the kinetic parameters with MVP3 were performed with the methods recommended by Nagaya et al. for the most accurate calculation: the differential-operator-sampling technique taking into account the indirect-perturbed-source effect. In addition, the comparison of the calculated results with the other methods available with MVP3 to those with the recommended methods were carried out, and the differences in the kinetic parameters between the other methods and the recommended method were summarized.
Calculation results of the continuous-energy Monte Carlo calculation codes have been used as reference analysis results to verify physics models and neutron libraries adopted by deterministic analysis codes, which have been applied to the nuclear design and the safety analysis of commercial light-water reactors. This study provides information on uncertainties in the kinetic parameters calculated with MVP3. These uncertainties will be taken into account when MVP3 will be used in the verification of the deterministic analysis codes.
Supplemental Material
Download PDF (694.6 KB)Disclosure statement
No potential conflict of interest was reported by the authors.
Supplementary Material:
Supplemental data for this article can be accessed here.
References
- Nagaya Y, Mori T. Calculation of effective delayed neutron fraction with Monte Carlo perturbation techniques. Ann Nucl Eng. 2011;38:254–260.
- Nagaya Y. Calculation of reactor kinetics parameters with Monte Carlo differential operator sampling. Ann Nucl Eng. 2015;82:226–229.
- Nagaya Y, Okumura K, Sakurai T, et al. MVP/GMVP version 3: general purpose Monte Carlo codes for neutron and photon transport calculations based on continuous energy and multigroup methods. Tokai-mura (Japan): Japan Atomic Energy Agency; 2017. (JAEA-Data/Code 2016-018).
- Shibata K, Iwamoto O, Nakagawa T, et al. JENDL-4.0: a new library for nuclear science and engineering. J Nucl Sci Technol. 2011;48:1–30.
- Tsuruta H, Kitamoto K. Experiments and calculation of βeff/ℓ for the light-water moderated two-region core of UO2 and PuO2-UO2 lattices. Tokai-mura (Japan): Japan Atomic Energy Research Institute; 1972. (JAERI-M 4696). [in Japanese].
- Nakajima K. Re-evaluation of the effective delayed neutron fraction measured by the substitution technique for a light water moderated low-enriched uranium core. J Nucl Sci Technol. 2001 Dec;38:1120–1125.
- Litaize O, Santamarina A Experimental validation of effective delayed neutron fraction in LWR-MOX core. Proc. PHYSOR 2002; 2002 Oct 7–10; Seoul (Korea). [CD-ROM].
- Shibata K, Kawano T, Nakagawa T, et al. Japanese evaluated nuclear data library version 3 revision-3: JENDL- 3.3. J Nucl Sci Technol. 2002;39:1125–1136.
- Chadwick MB, Herman M, Oblŏzinský P, et al. ENDF/B-VII.1 nuclear data for science and technology: cross sections, covariances, fission product yields and decay data. Nucl Data Sheets. 2011;112:2887–2996.
- 2019. https://www.oecd-nea.org/dbforms/data/eva/evatapes/jeff_32/ .
- Haga T, Kobayashi K. Space dependent reactivity effect of fission and absorption. J Nucl Sci Technol. 1964 Oct;1:246–254.
- Hibi K, Tatsumi M, Umano T, et al. Analysis of MISTRAL and EPICURE experiments with SRAC and MVP code systems. Physor; 2000 May 7–11; Pittsburgh (PA). [CD-ROM].
- Tsuruta H, Matsuura S, Kobayashi I, et al. Critical experiments and analysis on 7 × 7 PuO2-UO2 lattices in light water moderated UO2 core. Tokai-mura (Japan): Japan Atomic Energy Research Institute; 1974. (JAERI-1234).
- Tsuruta H, Kobayashi I, Suzaki T, et al. Critical sizes of light water moderated UO2 and PuO2-UO2 lattice. Tokai-mura (Japan): Japan Atomic Energy Research Institute; 1978. (JAERI-1254).
- Rahman M, Suzaki T, Mori T. Analytical study of two-region TCA critical experiments with PWR MOX fuel by using Monte Carlo code MVP. Tokai-mura (Japan): Japan Atomic Energy Research Institute; 2003. (JAERI-Research 2003-007).
- Kanda K, Yamamoto T, Matsuura F, et al. MOX fuel core physics experiments and analysis -aiming for plutonium effective use. J At Energy Soc. 1998 Nov;40:834–854. Japan. [in Japanese].
- Study of LWR core concepts on effective utilization of plutonium. Tokyo (Japan): Nuclear Power Engineering Corporation; 2003. [in Japanese].
- Yamamoto T, Sakai T, Ando Y, et al. Neutronics analysis of full MOX BWR core simulation experiments FUBILA. J Nucl Sci Technol. 2011;48:398–420.
- Nakagawa T, Shibata K, Chiba S, et al. Japanese evaluated nuclear data library version 3 revision-2: JENDL-3.2. J Nucl Sci Technol. 1995;32:1259–1271.
- Nagaya Y, Okumura K, Mori T, et al. MVP/GMVP II: general purpose Monte Carlo codes for neutron and photon transport calculations based on continuous energy and multigroup methods. Tokai-mura (Japan): Japan Atomic Energy Research Institute; 2005. (JAERI-1348).
- Okumura K, Kaneko K, Tsuchihash K, et al. SRAC95; general purpose neutronics code system. Tokai-mura (Japan): Japan Atomic Energy Research Institute; 1996. (JAERI-Data/Code 96-015).
- https://www.oecd-nea.org/dbdata/jeff/jeff33/; 2020.
- https://www.oecd-nea.org/dbforms/data/eva/evatapes/jeff_31/index-JEFF3.1.1.html; 2020.
- van der Marck SC. Benchmarking ENDF/B-VII.1, JENDL-4.0 and JEFF-3.1.1 with MCNP6. Nucl Data Sheets. 2012;113:2935–3005.
- Gooley T, James M, Booth T, et al. Initial MCNP6 release overview. Nucl Technol. 2012;180:298–315.
- Chiba G, Okumura K, Sugino K, et al. JENDL-4.0 benchmarking for fission reactor applications. J Nucl Sci Technol. 2011;48:172–187.
- Sakai T, Yamamoto T. Neutron cross section sensitivity analysis of UO2 and MOX core physics experiments on light water reactors. J Nucl Sci Technol. 2014 Feb;51:240–250.
- Yamamoto T, Sakai T. Feedback on neutron capture cross sections of 238Pu and 241Am from analyses of measured isotopic compositions of irradiated LWR fuels and MOX core physics experiments. J Nucl Sci Technol. 2016 Aug;53:1235–1242.
- Terada K, Kimura A, Nakao T, et al. Measurements of neutron total and capture cross sections of 241Am with ANNRI at J-PARCJ. Nucl Sci Technol. 2018;55:1198–1211.
Appendix 1
Calculation Results with the Other Methods Available with MVP3
The MVP3 calculations provided the results of the kinetic parameters, βeff and ℓ, with the methods [Citation3] other than the differential-operator-sampling technique with the indirect-perturbed-source effect [Citation1,Citation2], which were adopted to obtain the calculation results for the comparison with the measured results. The other methods for βeff and ℓ are 1) the differential-operator-sampling technique without the indirect-perturbed-source effect, 2) the correlated-sampling technique with the indirect-perturbed-source effect, 3) the correlated-sampling technique without the indirect-perturbed-source effect [Citation3]. The other methods only for βeff are 4) the Meulekamp’s method, 5) the importance function weight method (the Nauchi’s method), and 6) the constant weight methods (the non-adjoint weighted method) as the next fission probability methods [Citation3]. lists some of numerical results of the other methods compared with those of the differential-operator-sampling technique with the indirect-perturbed-source effect (hereafter, they are referred to as ‘the references.’). and illustrate some of comparison results for the calculated kinetic parameters obtained with the other methods as the ratios to the references for all the cores.
Figure A1. Ratios of the calculated βeff obtained with the other methods in MVP3 [Citation3] to the reference (the results with the differential-operator-sampling technique with the indirect-perturbed-source effect) for all the cores.
![Figure A1. Ratios of the calculated βeff obtained with the other methods in MVP3 [Citation3] to the reference (the results with the differential-operator-sampling technique with the indirect-perturbed-source effect) for all the cores.](/cms/asset/486aa283-f30a-4b31-ba59-73b257ee9fc5/tnst_a_1727375_f0003_oc.jpg)
Figure A2. Ratios of the calculated ℓ obtained without the indirect-perturbed-source effect for the differential-operator-sampling technique to the reference (with the indirect-perturbed-source effect for the differential-operator-sampling technique) for all the cores.
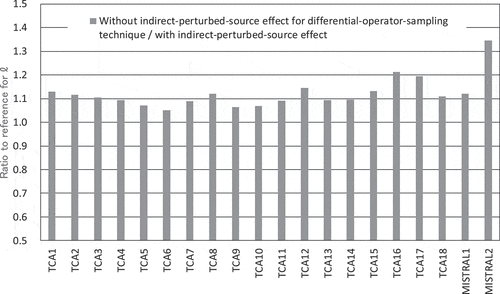
Table A2. Calculated results of βeff with the Meulekamp’s method, the importance function method, and the constant weight method in the next fission probability methods for MISTRAL1.
Concerning βeff calculated with the perturbation method, the results of the differential-operator-sampling technique and the correlated-sampling technique are almost identical as previously indicated by Nagaya et al. [Citation1]. As for the indirect-perturbed-source effect for the perturbation methods, the effects on the calculated βeff are less than 2% for the most cores, and the trends of the effects are different between the cores of from TCA12 through TCA17, which are the two-region cores composed of the central core region of the MOX fuel rods and the driver region of the UO2 fuel rods, and the other cores, as shown in . As for the indirect-perturbed-source effect on the calculated ℓ, the effects are larger than 10% for the most cores, and they are larger for the MOX cores, TCA16, TCA17 and MISTRAL2, which were loaded with the high Pu content MOX fuel rods. It is noted that the number of propagation batches of 10 for the calculation of the indirect-perturbed-source effect were quite enough for all the cores, which accords with the results previously reported by Nagaya et al. [Citation1].
The results of βeff calculated with Meulekamp’s method are compared with the references, and the comparison results are shown in Table A1 and Figure A1. The differences are less than 1% for the most cores. The exceptions are for those of from TCA12 through TCA17, which are the two-region cores composed of the central core region of the MOX fuel and the driver region of the UO2 fuel rods. shows the comparison results of the importance function method, and the constant weight method in the next fission probability methods, which were obtained from a preliminary calculation for MISTRAL1 with smaller neutron histories. The results with the importance function method are close to the reference and that with Meulekamp’s method. The result with the constant weight method are 10% lower than the reference.
Appendix 2
Calculated Effective Neutron Multiplication Factors
The calculated results of keff for the TCA and EOLE cores obtained with the JENDL-4.0-based neutron library are shown in and . The keff values with the JENDL-3.3-, ENDF/B-VII.1- and JEFF-3.2-based libraries are also shown for a part of the cores. The keff values with the JENDL-4.0-, ENDF/B-VII.1- and JEFF-3.2-based libraries were close to 1.0 for the majority of cores. For the UO2 cores of TCA without boron in the moderator, the average value obtained with the JENDL-4.0-based neutron library was 1.00015 with a standard deviation of 0.00038 Δkeff. This suggests that these libraries have a good performance for light-water reactor cores loaded with low-enriched UO2 fuel, which is in-line with the results of Chiba et al. [Citation26].
Figure A3. Keff values for the critical cores obtained with the JENDL-4.0-, JENDL-3.3-, ENDF/B-VII.1-, and JEFF-3.2-based neutron libraries.
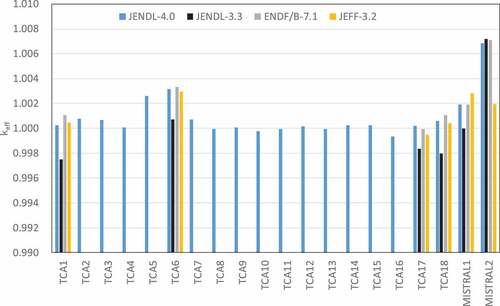
Table A3. Effective neutron multiplication factors for the critical cores with respect to the JENDL-4.0-, JENDL-3.3-, ENDF/B-VII.1- and JEFF-3.2-based neutron libraries. The statistical uncertainties were less than 0.005 to 0.006%Δkeff.
The cores showing keff values of 1.002 to 1.003 were TCA5, TCA6 and MISTRAL1; they were the UO2 cores with boron concentrations of 300 ppm to 500 ppm. Even in the results with the JENDL-3.3-based library for these cores, these results are higher than those of the other UO2 cores. Tsuruta and Kitamoto [Citation5] reported the core characteristics of a derivative core of TCA6 which had the same number of UO2 fuel rods as TCA6. The fuel pitch of the cores was 2.15 cm (M/F ratio: 2.48), the boron concentration was 554 ppm, the critical water height was 86.89 cm, and the water temperature was 25.9 °C. The kinetic parameters were not measured for it. The additional MVP3 calculations were performed for the core in order to add the information pertaining to the correlation between boron concentrations and the keff values. The keff of the derivative core was 1.00243 ± 0.00005(1σ), which is larger than that of the TCA UO2 cores without boron in the moderator. Including the additional result, this study confirmed a correlation between boron concentrations and keff for the light-water moderated UO2 cores. It may indicate potential biases in the thermal-neutron-absorption cross sections of 10B with respect to the nuclear-data libraries.
As shown in Table A and Figure A, the results for JENDL-3.3 were systematically lower than those for JENDL-4.0, ENDF/B-VII and JEFF-3.2 (except for MISTRAL2). The nuclear-data libraries for JENDL-4.0, ENDF/B-VII and JEFF-3.2 had a lower thermal-neutron-capture cross section 1.3% lower than that of JENDL-3.3 with respect to 238U. This results in the keff values for light-water reactor cores to be 0.2 to 0.3% Δkeff lower than those of JENDL-3.3 [Citation27,Citation28].
While the keff value for MISTRAL2 with respect to JEFF-3.2 were 1.00194, keff was approximately 1.007 for JENDL-4.0, JENDL-3.3, and ENDF/B-VII. Relatively large discrepancies in the keff values for MISTRAL2 were correlated to the uncertainties in the cross sections in the nuclear-data libraries [Citation23]. The MOX fuel rods loaded in MISTRAL2 and the other MOX experimental cores of the MISTRAL program had high contents of 241Am. This was because roughly ten years had elapsed from the fuel fabrication when the fuel rods were used in the MISTRAL experiments performed from 1995 to 2000, resulting in the 241Pu isotopes decaying to 241Am isotopes. The reactivity calculations of the MOX cores of the MISTRAL program were influenced by the capture cross sections of 241Am in the thermal- and epithermal- energy regions in addition to the relevant cross sections of the other major uranium and plutonium isotopes. The capture cross sections of 241Am still have large uncertainties. According to Yamamoto et al. [Citation28], the thermal-neutron-capture cross section of 241Am for JENDL-4.0 was 7% larger than that of JENDL-3.3 and 9% lower than that of JEFF-3.2. That of ENDF/B-VII.1 [Citation9] adopted the same value as that of JENDL-4.0. Terada et al. [Citation29] recently reported a new measurement result for the thermal-neutron-capture cross section of 241Am which is 3% larger than that of JENDL-4.0 and 6% lower than that of JEFF-3.2.
The MOX fuel loaded into the TCA cores had high 239Pu contents and low contents of 240Pu and 241Pu; the contents of 241Am were not reported [Citation5]. The uncertainties in the neutron-capture cross sections of 241Am did not affect the keff values for the cores, TCA12 through TCA 17, all of which were partially loaded with MOX fuel rods.