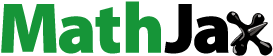
ABSTRACT
The fifth version of Japanese Evaluated Nuclear Data Library, JENDL-5, was developed. JENDL-5 aimed to meet a variety of needs not only from nuclear reactors but also from other applications such as accelerators. Most of the JENDL special purpose files published so far were integrated into JENDL-5 with revisions. JENDL-5 consists of 11 sublibraries: (1) Neutron, (2) Thermal scattering law, (3) Fission product yield, (4) Decay data, (5) Proton, (6) Deuteron, (7) Alpha-particle, (8) Photonuclear, (9) Photo-atomic, (10) Electro-atomic, and (11) Atomic relaxation. The neutron reaction data for a large number of nuclei in JENDL-4.0 were updated ranging from light to heavy ones, including major and minor actinides which affect nuclear reactor calculations. In addition, the number of nuclei of neutron reaction data stored in JENDL-5 was largely increased; the neutron data covered not only all of naturally existing nuclei but also their neighbor ones with half-lives longer than 1 day. JENDL-5 included the originally evaluated data of thermal scattering law and fission product yield for the first time. Light charged-particle and photon-induced reaction data were also included for the first time as the JENDL general purpose file.
GRAPHICAL ABSTRACT
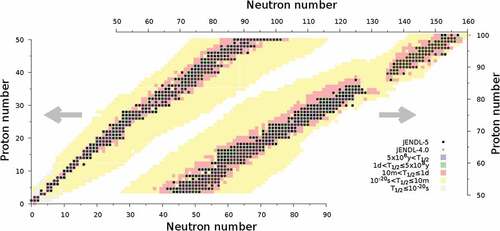
1. Introduction
The first version of Japanese Evaluated Nuclear Data Library JENDL-1 was released in 1977; it included neutron-induced reaction cross sections on 72 nuclides for development of fast reactors [Citation1]. Since then, many updates were made to meet the needs not only for the fast reactors but also for thermal and fusion reactors as well as for neutron shielding [Citation2]. The previous version JENDL-4.0 was released in 2010, in which the minor actinides and fission products were intensively revised; a large amount of covariance data for actinides were sufficiently given for neutronics calculations of fission reactors [Citation3].
To meet other needs, special-purpose files that included various kinds of data were developed and released since 1991. The libraries for the neutron transport such as JENDL-1 and JENDL-4.0 were referred as general purpose files [Citation2]. Before 2000, the special-purpose files focused on neutron reaction-related data such as dosimetry and activation that were not included in the general-purpose files. After that, the special purpose files were extended to charged-particles and photon-induced reactions. The neutron reaction data were also extended in the energy region up to 200 MeV or 3 GeV for high energy accelerators. With the spread of application areas with various types of radiations, the 21 special purpose files were released in total, including their updates, so far [Citation4].
After the release of JENDL-4.0, guidelines for the JENDL development were discussed in the Advisory Subcommittee on Development of JENDL under JENDL Committee, and its final report was published in 2015 [Citation5]. The report suggested to develop JENDL-5 so as to integrate the special purpose files such as activation cross section, high energy, dosimetry, fission product yield and photo nuclear data, as well as to increase the upper limit of energy, kinds of reactions, target nuclides and covariance, in addition to improve the thermal scattering law data. JENDL-5 was also targeting updates of the data with reflecting accumulated experimental and theoretical knowledge as well as the results of the international collaborations on nuclear data evaluations. Besides the JENDL-5 development, for the maintenance of JENDL-4.0, the 38 update files of JENDL-4.0 were released mainly for corrections of the errors that existed in JENDL-4.0 and addition of covariance data for Cr and Pb isotopes [Citation6]; these data were reflected to JENDL-5. Many test files of JENDL-5 named JENDL-5 in the early stage or JENDL-5
close in final stage of the development were created and benchmark tests mainly for reactors and neutron shielding were performed.
JENDL-5 was released in December 2021; it consists of 11 sublibraries of (1) Neutron, (2) Thermal scattering law, (3) Fission product yield, (4) Decay data, (5) Proton, (6) Deuteron, (7) Alpha-particle, (8) Photonuclear, (9) Photo-atomic, (10) Electro-atomic, and (11) Atomic relaxation. The neutron reaction data were largely revised with increasing the number of nuclides. For the first time, JENDL-5 adopted originally evaluated data for fission yields and thermal scattering laws [Citation7–9]. The charged particle reaction data released as the special purpose files were integrated with improvement; for the alpha-particle reaction data, JENDL/AN-2005 [Citation10] was complemented by adding the data needed for radiation transport calculations, since it included only the neutron emission data. For the neutron activation, new evaluations were also performed in addition to adoption of JENDL/AD-2017 [Citation11]. The evaluated data were all stored in the ENDF-6 format [Citation12] except for covariance of fission product yields for which the format was not defined.
Details for each sublibrary of JENDL-5 are described in the following sections.
2. Neutron sublibrary
The neutron sublibrary provides the neutron reaction data on the nuclides from H(Z = 1) to Fm(Z = 100). It consists of reaction cross sections and secondary particle emission data for radiation transport calculations as well as the data of residual nucleus production for neutron activation evaluation. Because JENDL-5 aims to meet the needs of various applications, the number of the nuclides of the neutron reaction data has increased to 795 that is close to double of 406 of JENDL-4.0. shows the nuclides in the neutron sublibrary on a chart of nuclides with half-lives. As shown in the figure, the neutron sublibrary includes the data for all of stable and unstable isotopes with the half-lives longer than 1 day for Z≤100 except 257Es.
Figure 1. Nuclides in the neutron sublibrary. Half life data of each nuclide is given by the decay data sublibrary.
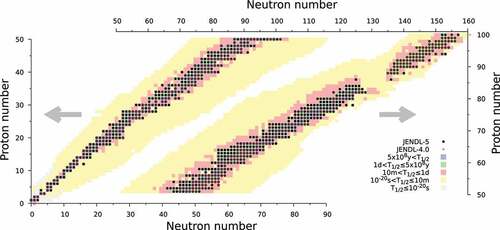
JENDL-5 integrated the neutron reaction data of traditional neutron transport data below 20 MeV, the activation cross section, and high energy reaction data above 20 MeV in a single evaluated file in the ENDF-6 format for each nuclide. In addition, several derived files were prepared for the convenience of JENDL-5 users; they were the four kinds of files, which consist of the pointwise data at 0 K and 300 K, the data up to 20 MeV, and the activation cross sections.
2.1. Actinides
2.1.1. Overview
The revisions of JENDL-4.0 were made mainly for the data that affected reactor benchmark tests. Several main points on the revisions are as follows: (1) the fission cross sections of 233,235,238U and 239,240,241Pu for fast neutrons were fully updated by the simultaneous evaluation extending the energy upper limit to 200 MeV, (2) the resonance parameters of ENDF/B-VIII.0 [Citation13] of 235U, 238U and 239Pu that were evaluated in the CIELO project [Citation14] were adopted, (3) the fission neutron spectra below 5 MeV of 235U were revised by fitting to the available experimental data, (4) the fission neutron multiplicities were revised by taking into accounts both differential experimental data and integral benchmark tests, (5) the minor adjustments of the fission and capture cross sections were made with the results of integral benchmark tests of fast reactors, (6) the resonance parameters of minor actinides were updated with the experimental data measured with ANNRI at J-PARC [Citation15].
shows ratios of thermal cross sections and resonance integrals of JENDL-5 to JENDL-4.0 for the fission and capture reactions for nuclides whose resonance parameters are revised. For the most of those nuclei, the changes from the values of JENDL-4.0 are within 5%. The thermal cross sections of 238Pu() and 240Pu
increase by a relatively large amount; they are based on new measurements as described in the following sections.
2.1.2. Simultaneous evaluation of fission cross section
Fission cross sections are often measured as the ratios to those of 235U and 239Pu. The same as JENDL-4.0, JENDL-5 adopted a method that use the ratio data with the absolute ones simultaneously instead of utilizing the standard cross sections. The fission cross sections of 233,235,238U and 239,240,241Pu above their performed by least-squares fitting of Schmittroth’s roof function [Citation16] to resolved resonance regions were evaluated simultaneously. The evaluation was the logarithms of the experimental cross sections and cross section ratios up to 200 MeV by using the SOK code developed for JENDL-3.3 evaluation [Citation17]. Experimental data published in or after 1970 (1980 for 235U) extracted from the EXFOR library [Citation18] were reviewed and those published with detailed uncertainty description were analysed. They included the experimental works published after release of JENDL-4.0 such as the data measured at n_TOF and LANSCE. Special attention was paid to avoid double counting of the same experimental work compiled in EXFOR several times. The EXFOR entries were revised with the source articles if necessary, and the input file for fitting was generated from the EXFOR source files automatically. The evaluated cross sections were validated against 252Cf spontaneous fission neutron spectrum averaged cross sections, (coupled thermal/fast uranium and boron carbide spherical assembly) spectrum averaged cross sections, and small-sized LANL fast system criticalities. For 235,238U and 239Pu, the output of the SOK code was adjusted for adoption by JENDL-5. Further details of the simultaneous evaluation are published elsewhere [Citation19].
2.1.3. 233U
The resonance analysis was performed with AMUR [Citation20,Citation21] up to 30 eV, based on the measured data of Harvey et al. [Citation22] and Guber et al. [Citation23] for total cross section and transmission, those of Berthoumieux et al. [Citation24] and Calviani et al. [Citation25] for fission cross section, and those of Weston et al. [Citation26] and Berthoumieux et al. [Citation24] for capture cross section. The present results of fission cross section together with JENDL-4.0 and measured data are shown in the top panel of , in which the energy range is 0.01 to 30 eV. The residuals between the fitted data by AMUR and the data measured by Calviani et al. [Citation25] and Berthoumieux et al. [Citation24] are derived. The residuals divided by the measured uncertainties are depicted in the bottom panel of . It is found that they are almost within . However, there are large differences which come from the difficulty of reproducing the peak shape of the resonances. The big resonances around 2 eV are compared with JENDL-4.0 and the measured data [Citation24,Citation25] in . The present evaluation reproduces the data of Calviani et al. [Citation25] well. It is found that JENDL-4.0 is slightly shifted to lower energy at the 1.8-eV resonance. The present thermal constants for fission and capture reactions are 538(2) and 43.5(10) b at 300 K, which are 1.3% larger and 4% smaller than JENDL-4.0, respectively.
Figure 3. Comparison of fission cross section of 233U in JENDL-5 (solid line) with JENDL-4.0 (dashed line) and measured data (top), and the residuals between JENDL-5 and the data of Calviani et al. [Citation25]. or Berthoumieux et al. [Citation24]. relative to their uncertainties (bottom) in the range of 0.01 to 30 eV. The symbol after the authors means the data at the thermal energy.
![Figure 3. Comparison of fission cross section of 233U in JENDL-5 (solid line) with JENDL-4.0 (dashed line) and measured data (top), and the residuals between JENDL-5 and the data of Calviani et al. [Citation25]. or Berthoumieux et al. [Citation24]. relative to their uncertainties (bottom) in the range of 0.01 to 30 eV. The symbol th after the authors means the data at the thermal energy.](/cms/asset/b7d871f8-ffbe-473a-a038-0a2d5a81ec30/tnst_a_2141903_f0003_oc.jpg)
The result of the simultaneous evaluation was adopted for the fission cross section above 10 keV in JENDL-5. However, this revision of fission cross section tended to underestimate criticalities of fast reactors with 233U cores of Jezebel-233 and Flattop-233 [Citation19]. In addition, the prompt fission neutron multiplicity
of JENDL-4.0 was evaluated using 2 linear lines connected at 1.5 MeV and the value at 1.5 MeV located near the lower limit of the experimental data (). The
was updated to have larger values with experimental data by connecting linearly between 1 MeV and 3 MeV of JENDL-4.0 as shown by the red line in the figure, resulting in agreements with the criticalities of Jezebel-233 and Flattop-233.
2.1.4. 235U
The resonance parameters of ENDF-B/VIII.0 (CIELO-1) [Citation13] were adopted. A minor adjustment based on fast reactor benchmark tests was made on the cross sections above 100 eV; the details of the adjustment are described in Section 2.1.18. show the group average cross sections of JENDL-5 for fission and capture reactions, respectively. The ratios to JENDL-4.0 are shown with the other evaluated data of ENDF/B-VIII.0 [Citation13], JEFF-3.3 [Citation27] and the IAEA neutron data standards 2017 [Citation28] for comparison. The fission and capture cross sections were changed from JENDL-4.0 by several percent at most and by more than 10%, respectively, while the changes of the thermal values were small. The deviation observed around 1 keV from ENDF/B-VIII.0 for fission cross section is due to the adjustment mentioned above; the capture cross section is almost identical to that of ENDF/B-VIII.0. The large difference with the IAEA standards for fission cross section is mainly due to the no resonance structure in the standards evaluation.
Figure 6. Group averaged cross section of 235U() (upper panel) and its ratio to JENDL-4.0 (lower panel) in the resolved resonance region, comparing with ENDF/B-VIII.0, JEFF-3.3 and the IAEA neutron data standards 2017.
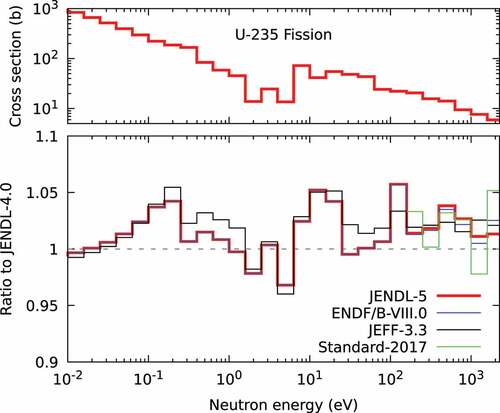
Figure 7. Group averaged cross section of 235U() (upper panel) and its ratio to JENDL-4.0 (lower panel) in the resolved resonance region, comparing with ENDF/B-VIII.0 and JEFF-3.3.
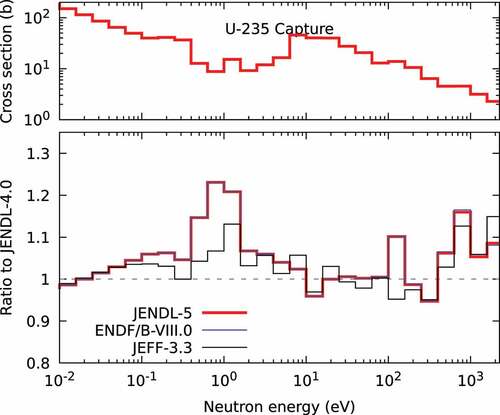
The fission cross section above 10 keV was based on the simultaneous evaluation. Because it was limited above 10 keV, the fission cross section of IAEA neutron standards [Citation28] was adopted in the energy range between 2.25 keV (the upper limit of the resonance region) and 10 keV (the lower limit of simultaneous evaluation) with modification as described in Section 2.1.18.
The prompt fission neutron spectra were evaluated by model-based fitting to the available experimental data [Citation29–34]. The modified Los Alamos model [Citation35], which was adopted above 5.5 MeV in the JENDL-4.0 evaluation, was used with the least-square method including the normalization factors to each experimental data set. The parameters of the nuclear temperature , scission neutron faction
, and s-wave neutron strength function
were searched; the other parameters were fixed to ones of the systematics [Citation35]. The calculated results with these parameters were adopted below 5 MeV. shows the obtained spectrum for 235U
at the thermal neutron energy, comparing with the experimental data and the other evaluated nuclear data. While JENDL-4.0 tends to underestimate below 1 MeV from the experimental data and to overestimate between 2 and 4 MeV, JENDL-5 shows better agreements. JENDL-5 is close to ENDF/B-VIII.0 and JEFF-3.3 except the data of ENDF/B-VIII.0 above 10 MeV. The average energies of the prompt fission neutron spectra are shown in . The present result is approximately 1% lower than JENDL-4.0 and is similar to ENDF/B-VIII.0 and JEFF-3.3 at the thermal neutron energy. The energy dependence is close to ENDF/B-VIII.0, while some deviation is seen for JEFF-3.3 as the incident energy increases.
Figure 8. Fission neutron spectrum of 235U for thermal neutrons. The values of evaluated libraries are shown by lines. The symbols indicate experimental data [Citation29–34] used for fitting.
![Figure 8. Fission neutron spectrum of 235U for thermal neutrons. The values of evaluated libraries are shown by lines. The symbols indicate experimental data [Citation29–34] used for fitting.](/cms/asset/11c143d0-b28e-499d-92c7-54e7839fcf14/tnst_a_2141903_f0008_oc.jpg)
The revision of the resonance parameters and fission neutron spectrum of 235U affected the criticalities of the thermal reactors. The revision of the thermal scattering law for the light water also made significant impact to the benchmark results. The prompt neutron multiplicity of
U was also one of the main contributors to the criticalities. The
was evaluated by taking into account the experimental data and the integral benchmark results of high-enriched uranium cores of HCI(003), HMF(015, 016, 065), HMI(001, 006), HMT(001, 003, 006, 008–010, 012–014, 016, 018, 031, 033), and HST(002, 010, 013, 032, 042, 050), and low-enriched ones of LCT(001–003, 005–011, 017–019, 022, 024–027, 030, 033, 035, 048, 064, 079, 080) LMT(001, 006, 007), and LST(020, 021) in ICSBEP. compares the evaluated values and the experimental data in the thermal neutron energy region. JENDL-4.0(=JENDL-3.3) was evaluated based on the Gwin et al. [Citation36] In addition, JENDL-4.0 took the rather complicated energy dependence in keV energy region below 1 MeV as shown in . However, it was not so evident from viewpoint of the various experimental data. In the JENDL-5 evaluation, the energy dependence of
was assumed to have smoother energy dependence. In the evaluation, the benchmark results of criticalities for the fast reactors in were also taken into account, in addition to the experimental data of
. For the energy region between around 0.5 to 50 keV, only the experimental data of Gwin et al. [Citation36] exist. The JENDL-5 evaluation follows rather upper part of those data. Because in the other energy region, the experimental data spread over by taking wider values, additional measurements are expected to clarify their accuracy.
Figure 11. Prompt neutron multiplicity of 235U. the experimental data after 1970 are shown by various symbols.
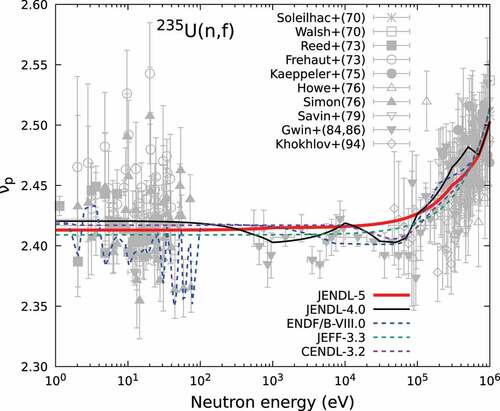
Table 1. Benchmark tests used for the minor adjustment.
2.1.5. 238U
For a test library JENDL-5α1, an evaluated file of 238U was created with adopting the resonance parameters of ENDF-B/VIII.0 (CIELO-1). However, it indicated the significant decrease of criticality comparing to JENDL-4.0 with respect to depletion that was similarly observed for ENDF/B-VIII.0 with respect to ENDF/B-VII.1 [Citation70]. The sensitivity analysis suggested that, in addition to the first resonance of 239Pu, some contributions existed by 238U capture cross section above 10 eV; in this energy region, the average cross section of ENDF-B/VIII.0 was decreased from JENDL-4.0. Since new experimental data of Wright et al. [Citation71] indicated the larger cross sections at the keV neutron energy region and fluctuation of the radiative resonance kernel above 100 eV depending on analysis with the different measurements in in their reference [Citation71], respectively, the resonance parameters were modified so as to increase the average capture cross section by 2% above 100 eV with the similar method of the adjustment in Section 2.1.18. In addition, the minor adjustment in Section 2.1.18 was made to them.
Figure 12. Group averaged fission cross sections in the resolved resonance region of 238U; the same as but for 238U.
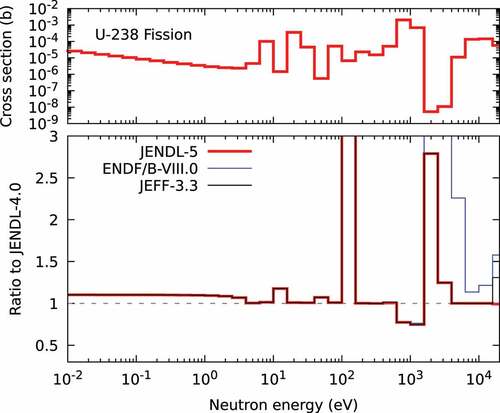
show the group average cross sections of JENDL-5 for fission and capture reactions, respectively. The ratios to JENDL-4.0 are shown with those of the other evaluated libraries. The larger capture cross section above 100 eV than ENDF/B-VIII.0 and JEFF-3.3 is due to the above modification; note that ENDF/B-VIII.0 and JEFF-3.3 give the identical cross sections in the resolved resonance region. Regarding fission, the significant difference from ENDF/B-VIII.0 above 1 keV is due to the background cross section introduced in ENDF/B-VIII.0 so as to reproduce the low-resolution cross section measured by Slovacek et al. [Citation72], which is not taken in JENDL-5 because of the ambiguity in energy dependence and the possible low impact to nuclear reactor applications.
Figure 13. Group averaged capture cross sections in the resolved resonance region of 238U; the same as but for 238U.
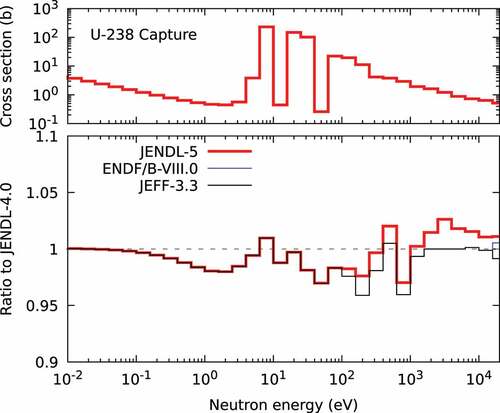
For the fission cross section above 70 keV, the simultaneous evaluation was adopted with modifications. Because the result of the simultaneous evaluation underestimated the fission spectrum average cross section (SACS) of 252Cf spontaneous fission by Mannhart [Citation73] and the integral tests for fast reactors shown in favored larger cross section, the cross sections between 1.5 and 3.5 MeV were increased by 1–2% for JENDL-5β1 as shown in ; they were rather close to JENDL-4.0 below 3 MeV. Using JENDL-5β1, the minor adjustment with the fast reactor integral tests was performed; it resulted in the additional increase of the cross section around 2 MeV (). Regarding the SACSs, the ratios to the Mannhart evaluation (325.7 b 1.64%) were 0.971, 0.979 and 0.984 for the original simultaneous evaluation, JENDL-5β1 and JENDL-5, respectively; the final JENDL-5 estimated value was the closest to Mannhart’s evaluated value.
Figure 14. Fission cross section of 238U for fast neutrons. The experimental data after 2000 are shown by various symbols.
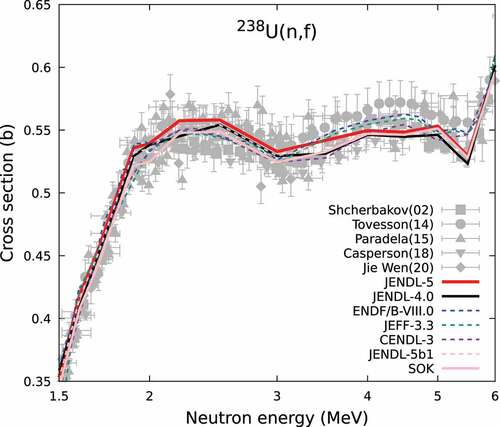
The inelastic scattering cross section of JENDL-4.0 was calculated as the sum of the direct reaction with the coupled channel optical model and the compound reaction with the Hauser-Feshbach statistical model using CCONE [Citation74]. In the CIELO project, the inelastic scattering cross section was evaluated by taking into account the effects of the direct reaction to the compound reaction by using Engelbrecht-Weidennmüller transformation; that caused the increase of the inelastic scattering [Citation75]. Since the CIELO cross section seemed to agree better with the experimental data, the cross section to the first excited level was adopted.
For JENDL-4.0, the was evaluated with a rather simple function whose energy dependence was given by a single straight line below 14 MeV. This assumption was not evident in view of the experimental data and the recent evaluated libraries gave different energy dependencies from each other. Since in the energy region below 5.5 MeV experimental data were abundant, we evaluated the
with the Gaussian process regression that did not need to assume the energy dependence [Citation76]. shows the fitting result comparing with the experimental data and evaluated libraries. While JENDL-4.0 seems slight overestimation around 3.5 MeV, the resulted curve locates in the middle of the experimental data, which is close to JEFF-3.3.
2.1.6. 237Np
Neptunium-237 is one of the important minor actinides for the design of the nuclear fuel cycle. The improvement of the accuracy of capture cross section, which is larger than the fission cross section below 400 keV, has been requested for reactor design of an accelerator-driven system [Citation77]. The resonance parameters up to 109.1 eV were taken from the dataFootnote1 of Rovira et al. [Citation78], in which the capture measurements were made by the NaI detector of ANNRI and the parameters were extracted by REFIT [Citation79,Citation80]. The parameters above 109.1 eV were the same as those of JENDL-4.0. The thermal capture cross section obtained by Rovira et al. was 177.6 (38) b, which is consistent with that of JENDL-4.0 (178.1 b). The recent activation measurements favor a smaller cross section by 2%, which was taken into account for JENDL-5 (173.9(92) b) by the parameter modifications of negative resonances. The capture cross sections between 500 eV and 230 keV were revised, based on the data measured at ANNRI and integral benchmark tests.
2.1.7. 238Pu
The resonance parameters of JENDL-4.0 were evaluated so as to agree with the capture cross section measured by Butler et al. [Citation81] However, the recent measurement by Chyzh et al. [Citation82] was not consistent with the results of Butler et al. Because the resonance parameters of JENDL-3.3 gave better agreement, we took these and revised the parameters of the negative resonance so as to get better agreement with the data of Chyzh et al. as shown in . The obtained parameters give the thermal cross sections that are consistent with the recommendation of Mughabghab [Citation83]. However, for the fission cross section, the energy dependence of the experimental data was not well reproduced by both JENDL-5 and JENDL-4.0 as shown in . Further study might be needed.
Figure 16. Cross sections of total (top), capture (middle) and fission (bottom) reactions for neutron-induced reaction on 238Pu at thermal energy region.
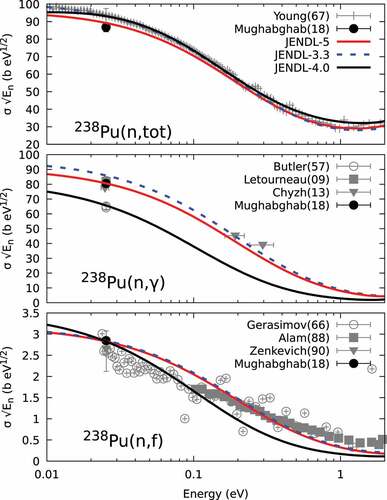
Underestimation of fission rate ratios of 238Pu to 239Pu with JENDL-4.0 was observed in measurements with fast neutron reactor systems of the FCA-IX cores [Citation84]. According to the sensitivity of the experiment, the fission cross section between 1 keV and 5 MeV increased by 2% at most. As shown in , JENDL-5 is rather consistent with the measurement of Budtz-Jørgensen et al. [Citation85] while it is larger than that of Fursov et al. [Citation86]. Recently the cross sections were obtained by surrogate method by Ressler et al. [Citation87] and Hughes et al. [Citation88], but their uncertainties were not small enough to figure out the validity of the JENDL-5 evaluation.
2.1.8. 239Pu
The resonance parameters of ENDF-B/VIII.0 (CIELO-1) [Citation13] were adopted with the minor adjustment in Section 2.1.18. show the group average cross sections of 239Pu fission and capture reactions in the resonance region. The ratios to JENDL-4.0 are shown with the other evaluated libraries of ENDF/B-VIII.0 and JEFF-3.3 for comparison. The cross sections of JENDL-5 and ENDF/B-VIII.0 are almost identical, while the fission cross section of JEFF-3.3 shows deviation from JENDL-5 at the higher energy side of the first resonance.
Figure 18. Group averaged fission cross sections in the resolved resonance region of 239Pu; the same as but for 239Pu.
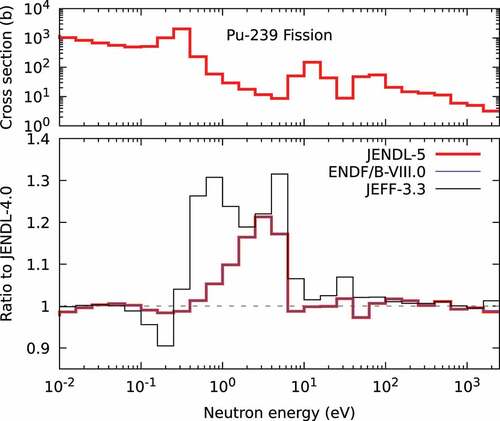
Figure 19. Group averaged capture cross sections in the resolved resonance region of 239Pu; the same as but for 239Pu.
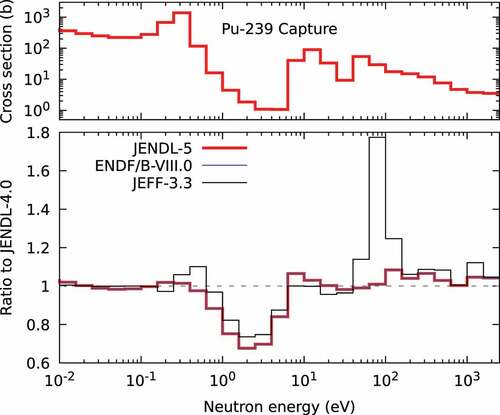
The capture cross section of JENDL-4.0 was evaluated by the theoretical model calculation with CCONE [Citation74]. However, the cross section between 10 keV and 100 keV seemed to have a possibility to be overestimated comparing with the experimental data as shown in . For JENDL-5, the cross section was decreased around 10% between 10 and 300 keV.
Figure 20. 239Pu() cross section in fast neutron energy region. Experimental data are shown by various symbols; darker ones correspond to newer ones in certain periods.
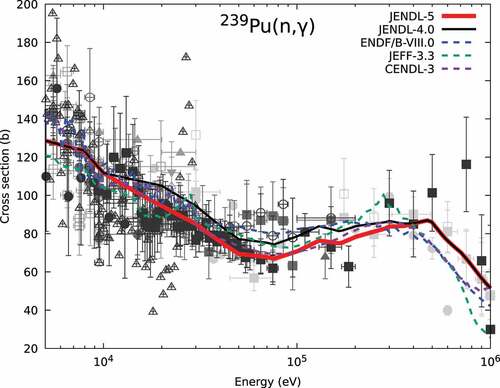
The of 239Pu was studied under WPEC SG-34 [Citation89] to solve the discrepancy between criticalities calculated with the recent evaluated nuclear data libraries and integral experimental data for the plutonium systems. In SG-34,
was evaluated by taking into account the two step (
) process which depends on the spin of the resonance. Below 0.7 eV where the first resonance dominated, JENDL-5 adopted the results of SG-34, but above it the values of SG-34 were not taken because the narrow resonance structure was not consistent with JENDL-5. For fast neutron energy between 1 keV and 100 keV, the JENDL-4.0 evaluation was mainly based on the experimental data of Gwin et al. [Citation90,Citation91] with some energy dependence. However, experimental data were scattered and it seemed to be difficult to deduce the reliable energy dependence. Therefore, a rather smooth energy dependence was assumed as shown in ; the evaluated value was determined with taking into account the experimental data and the benchmark tests.
2.1.9. 240Pu
JENDL-4.0 adopted the resonance parameters obtained by Bouland et al. [Citation92] with modification on the negative resonance so as to reproduce the measured fission cross section by Eastwood et al. [Citation93] at the thermal neutron energy. The recent measurement by Stamatopoulos et al. [Citation94] showed the possibility of the significant underestimation of fission cross section of JENDL-4.0 and the original resonance parameter set by Bouland et al. indicated better consistency with those of Stamatopoulos et al. Therefore, the resonance parameters of JENDL-5 were taken back to the original values of Bouland et al.
For fast neutrons, the simultaneous evaluation of the fission cross section was adopted above 10 keV up to 200 MeV. The energy region was extended up to 200 MeV by merging the cross sections and particle emission spectra of JENDL-4.0/HE except fission cross section. The was evaluated by the systematics described in Section 2.1.19.
2.1.10. 241Pu
The fission cross section for fast neutrons above 10 keV up to 200 MeV was revised by the simultaneous evaluation. The evaluated data of JENDL-4.0/HE were adopted above 20 MeV except the fission cross section. In addition, the was revised by the systematics (Section 2.1.19).
2.1.11. 242Pu
Lerendegui-Marco et al. reported the resonance parameters based on the measured data at n_TOF-EAR1 facility [Citation95]. JENDL-5 adopted their parameters below 1 keV. The new resonance parameters resulted in the decrease of the thermal cross section by 2% and the increase of the resonance integral by 4% from JENDL-4.0 as shown in .
It was reported that the calculated values of the fission reaction rate ratio of Pu to 239Pu with JENDL-4.0 were overestimated in comparison with the experimental data measured at BFS-67 and FCA-IX cores [Citation84,Citation96]. Several measurements of 242Pu fission cross section were reported after the release of JENDL-4.0. These recent experimental data show a trend having smaller values comparing with the previous ones. For JENDL-5, the cross section was evaluated by the least square method with SOK [Citation17] with the experimental data measured after 2000. shows the results with all experimental data and ones limiting after 2000, where it is clearly seen that the result after 2000 indicates smaller values.
2.1.12. 241Am
An improvement of capture cross section for 241Am has been considered to be requisite for design of nuclear transmutation system such as an accelerator-driven system (ADS) [Citation97]. The capture and transmission measurements were made by the Ge and Li-glass detectors, respectively, at ANNRI [Citation98]. The resonance analyses were performed with both capture and transmission data by REFIT [Citation79,Citation80]. The resonance parameters were derived up to 19 eV, above which the data of Mendoza et al. [Citation99] were adopted up to 700 eV. It is confirmed that the capture cross section is almost consistent with those of Mendoza et al. in the region between 1 and 19 eV. The thermal capture cross section at 300 K is 709(47) b, which is 3.7% larger than that of JENDL-4.0 (684 b). shows that the comparison of the obtained capture cross section with that of JENDL-4.0. The 5.8-eV resonance was removed since it was found to be attributed to the impurity 237Np, which is the -decay product of 241Am. The modification of fission width was made to preserve the area of fission cross sections. The fission widths in the energy region higher than 160 eV were assumed to be 0.28 meV.
Figure 23. Comparison of capture cross section of 241Am in JENDL-5 (solid line) with that in JENDL-4.0 (dashed line) below 20 eV.
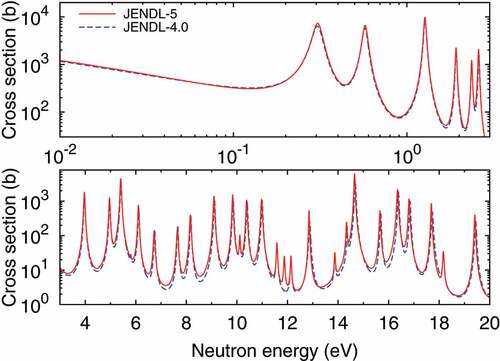
In the fast energy region the capture cross section between 10 and 70 keV was adjusted, based on the data of Fe-filtered experiments [Citation100]. This leads to the capture cross section by maximum of 4% larger than that of JENDL-4.0.
The integral benchmark test of FCA fission reaction ratio of 241Am to 239Pu showed 3–5% underestimation of JENDL-4.0 to the experimental data [Citation84]. For the JENDL-5 evaluation, the fission cross section around between 1 and 3 MeV of JENDL-4.0 was increased by 3%. In , the evaluated fission cross section of JENDL-5 is compared with the recent evaluated libraries and the experimental data after 1970. From a viewpoint of the experimental values of fission cross section, JENDL-4.0 may be favorable. However, the revision of the fission cross section was made because the integral data of the fission reaction ratio had rather pure sensitivity to the fission cross section in addition to the existence of experimental data that were larger than JENDL-4.0.
2.1.13. 243Am
Capture cross sections of Am isotopes are also important for burnup calculation of light water reactors especially with MOX fuel [Citation101]. The resonance parameters of 243Am below 19 eV were obtained by the resonance analysis with the transmission and capture yield data measured by Kimura et al. with ANNRI in MLF at J-PARC [Citation102]. The transmission data with a 240 MBq sample and capture yield data with 60, 120 and 240 MBq samples were used as the inputs of the REFIT code [Citation79,Citation80]. The impurities of 239,240Pu, 241,242mAm and 244Cm were taken into account in the analysis. The fitted examples were shown in . Kimura et al. reported the thermal value 87.7±5.4 b for the capture cross section. However, it was found that they did not include the effect of 242mAm() in the analysis. We obtained the result of 79.6 b for JENDL-5 that was rather close to 79.2 b of JENDL-4.0.
Above 19 eV, the resonance parameters obtained by Mendoza et al. [Citation103] with the capture yields measured at n_TOF were adopted because the energy resolution of the n_TOF data was better than that of ANNRI. The upper limit of the resolved resonance energy region was extended from 250 eV of JENDL-4.0 to 400 eV.
Figure 25. Fitted results below 20 eV with transmission and capture yield data of Kimura et al. for the 243Am 240 MBq sample.
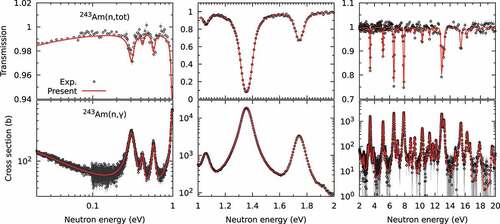
In the fast energy region the capture cross section between 10 and 84 keV was adjusted, based on the data of Fe-filtered experiments [Citation104]. This leads to the capture cross section by max 7.8% larger than that of JENDL-4.0.
Similarly to the case of 241Am, the calculated results of JENDL-4.0 for the FCA fission reaction ratio of 243Am to 239Pu showed 5% underestimation with respect to the experimental data [Citation84]. For the JENDL-5 evaluation, the fission cross section around 2 MeV of JENDL-4.0 was increased by 3%. shows a comparison of JENDL-5 with the recent evaluated libraries and the experimental data after 1970. The JENDL-5 evaluation results in closer values to Knitter et al. [Citation105] and the recent measurements of Belloni et al. [Citation106] and Kessedjian et al. [Citation107].
2.1.14. 243Cm
The thermal cross section for 243Cm() measured by Popscu et al. [Citation108] after the release of JENDL-4.0 had a significantly larger value than JENDL-4.0. The weighted average of the available experimental data was obtained to be 623±16 b. The fission and neutron widths of the negative resonance were adjusted so as to reproduce the above value.
2.1.15. 244Cm
Kawase et al. obtained the resonance parameters with the experimental data of 244Cm() measured with ANNRI at J-PARC [Citation109]. JENDL-5 adopted the resonance parameters below 420 eV with modifying the fission widths so as to reproduce the fission cross sections of JENDL-4.0. Since Kawase et al. did not obtain cross section below 1 eV, the widths of the negative resonance at −6.65 eV were modified so as to reproduce the reference values of thermal cross sections used in the JENDL-4.0 evaluation. The decrease of JENDL-5 by around 8% () for the thermal capture cross section was due to the uncertainty of the reference cross section. The fission widths of the first and second resonances were adjusted with referring the resonance integral 6.14±0.31 b measured by Alekseev et al for the energy range of 0.5 eV to 20 keV [Citation110].
Since the calculated results of the fission reaction rate ratios of 244Cm to 239Pu with JENDL-4.0 indicated significant overestimation in comparison with the measured data at the BFS-67, BFS-69 and FCA-IX cores [Citation84,Citation96], the fission cross section of 244Cm was revised taking into account the possible overestimation in a few MeV energy region. The JENDL-4.0 evaluation was made by the least square fitting with the experimental data with GMA [Citation111,Citation112]. The experimental data of Fursov et al. [Citation86] seemed to be large comparing with the other experimental data. For JENDL-5, the data of Fursov et al. were renormalized with multiplying the factor of 0.85 so as to agree with the other experimental data at the plateau of the first chance fission. In addition, the energies of the data measured Fomushkin et al. [Citation113] were corrected by modifying the zero time of time-of-flight (TOF) so as to be consistent with the other experimental data. By using these and other experimental data, the fission cross section was evaluated with SOK [Citation17]. shows the result with the experimental data used in the fitting. JENDL-5 indicates a smaller trend below 5 MeV than JENDL-4.0.
Figure 27. Fission cross section of 244Cm. Experimental data are shown by various symbols. The averaged data of Moore are also shown for comparison.
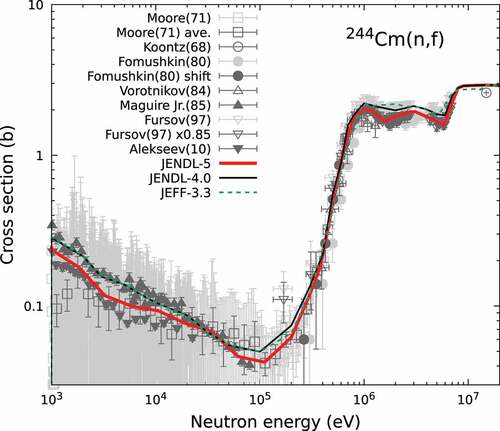
The of 244Cm(
) at thermal energy for JENDL-4.0 was evaluated to be 3.0, based on the experimental data of 2.75±0.08 measured by Zhuravlev et al. [Citation114]. However, their experimental data were for the spontaneous fission of 244Cm. Hirose et al. deduced a preliminary result of
for 244Cm(
) using a surrogate method at JAEA Tandem Accelerator [Citation115] with indicating the larger value. In addition, with a linear energy dependence for
, the benchmark test for the replacement measurements with 244Cm using Jezebel [Citation41] suggested the underestimation of the
of JENDL-4.0. Based on these evidences, the
of JENDL-5 for thermal neutron was determined to be 3.4 which was increased by 13% from JENDL-4.0. The linear energy dependence of JENDL-4.0 was taken over with keeping the value at 20 MeV the same.
2.1.16. 245Cm
Since no experimental data of the capture cross section were reported except for thermal neutrons, the JENDL-4.0 evaluation was purely based on the nuclear model calculation. The post irradiation examination (PIE) data obtained at the Prototype Fast Reactor (PFR) at Dounreay with MA samples indicated overestimation of the ratio of 246Cm to 245Cm which had a large sensitivity to the 245Cm() cross section from 100 eV to 2 MeV [Citation96]. Therefore, the capture cross section of 245Cm from 100 eV to 2 MeV was increased by 10% to improve the PIE calculation.
2.1.17. 246Cm
Kawase et al. [Citation109] obtained the resonance parameters for 246Cm with the capture yield data measured with ANNRI at J-PARC up to 1 keV. Since it seemed that they missed resonances above 400 eV, their parameters were adopted only below 400 eV. The fission widths were modified so as to reproduce the fission cross section of JENDL-4.0. Since the thermal cross section and the resonance integral were almost the same values as JENDL-4.0 and no new experimental data were reported after the release of JENDL-4.0, adjustment of the resonance parameters for the thermal cross section was not performed.
The JENDL-4.0 capture cross section above the resonance region was based on the nuclear model calculation. It was reported that the PIE data obtained at PFR showed the underestimation of the ratio of 247Cm to 246Cm [Citation96]. Since the PIE data had the large sensitivity to the 246Cm() cross section from 100 eV to 2 MeV, the capture cross section was increased by 10% to 20% from 400 eV to 2 MeV.
The of 246Cm was evaluated by the same way as 244Cm with the experimental data for spontaneous fission by Zhuravlev et al. [Citation114]. The data was revised based on the measured data with the surrogate method by Hirose et al. [Citation115].
2.1.18. Minor adjustment of cross section
Nuclear characteristics such as criticalities in integral benchmark tests of nuclear reactors often have high sensitivities to the cross sections with various energy dependencies. To obtain the nuclear data with high prediction capabilities for reactor calculations, detailed feedbacks from the benchmark tests to the nuclear data evaluations are unavoidable because uncertainties of the integral benchmark tests have much smaller uncertainties than those of differential data. However, modification of the cross sections with respect to the benchmark tests is not unique because sensitivities of benchmarks are spread over various cross sections in general. One possible reasonable approach is to determine the cross section by referring the sensitivities of the benchmarks. The adjustment with the reactor benchmark tests would not always infer the true values of the nuclear data, but it would suggest the practical values which agree the measured data for both integral and differential experiments.
In this work, the fission, capture and elastic scattering cross sections above 100 eV for 235U, 238U and 239Pu, which have large sensitivities, were adjusted using the integral benchmark tests of the fast reactors. This adjustment was intended to get the suggestions for the improvements of the integral benchmark tests with the aid of sensitivities; here we did not take the rigorous way for the adjustment that would need the carefully evaluated covariances for both of cross sections and benchmark tests.
The adjustment was performed using the generalized least square method with the group cross sections with a constant lethargy width in the energy range from 1 meV to 20 MeV with the number of 100. The changing amounts of the cross sections depended on the prior covariances and the sensitivities. To avoid the large change of the cross sections and complicated procedure, the prior uncertainties of the cross sections were set to energy independent constant values of 1.0%, 1.5% and 2.0% for fission, capture and elastic-scattering cross sections, respectively. The correlations were not introduced for simplicity. The 36 criticalities of small, middle and large size fast reactors were used for the adjustment targets. The 4 data sets of Na void and 2 sets of control-rod worth were also included. The used benchmark tests are listed in . The test library JENDL-5β1 that revised the actinide data mentioned above was used as prior data.
shows the C/E of the prior (JENDL-5β1) and the expected posterior (adjusted) results. The adjustment significantly improves the underestimation seen in the criticality and Na-void benchmark tests. The suggested changes of the group cross sections are shown by black lines in . Most of the data points were changed by less than about 1%. The changes for 235U() and 238U(
) cross sections were the largest in wide energy region, and those for 235U(
) and 238U(
) were the next.
According to the suggestion, the evaluated value of the cross sections was modified. Above the resonance region, the data points in the library were modified according to the change of the corresponding energy group. In the resonance region, the factors to the resonance widths of ,
,
and
were introduced for each group. The sensitivities of the group cross sections to each factor were calculated. Using these sensitivities, the resonance parameters were modified using the adjustment results. The group cross sections calculated by the revised resonance parameters are shown by red lines in . Since the resonance parameter correlates all kind of cross sections, some deviations from the suggested changes are observed. However, most of the revised data indicate reasonably good agreements.
2.1.19. Prompt neutron multiplicity above 20 MeV
The evaluated values of of JENDL-4.0/HE above 20 MeV were significantly smaller than the experimental data. They were re-evaluated using a function proposed by Ethvignot et al. [Citation116] which was given by
where is the incident neutron energy;
is the
at zero incident energy;
is the high energy limit of
;
is the increasing rate parameter. For the JENDL-5 evaluation,
was taken from the evaluated thermal values. From the comparison with the experimental data,
and
were nuclei-independently determined to be 12.5 and 0.016 (1/MeV), respectively. shows comparison of the systematics with the experimental data. Since for 237Np, the systematics somewhat overestimates the experimental data of Taieb et al. [Citation117], there might be a possibility for the improvement of present systematics. However, the determined parameters give reasonably good agreement with the experimental data in general. These parameters were applied to the
estimation above 20 MeV for 235,238U, 237Np, 237,239,240,241,242Pu, 241,242,242mAm.
The secondary neutron data of actinides at the incident energy above 20 MeV were given for fission (MT = 18) and the other reactions (MT = 5). The neutron emission multiplicity of MT = 5 was modified so as to keep the same amount of total neutron emission as JENDL-4.0/HE.
2.2. Structural materials
2.2.1. Ti
The resonance parameters were mostly taken from JENDL-4.0, except for 48Ti, whose parameters were adopted from ENDF/B-VIII.0. The total cross sections of 48Ti in the region of 0.4 to 7 MeV were directly obtained from the measured data of Rapp et al. [Citation118], in which the contributions of the other Ti isotopes were subtracted by using their total cross sections calculated by the optical model. The elastic scattering angular distributions of Smith et al. [Citation119] were taken into account in the region of 1.5 to 3.8 MeV.
The evaluation was made with CCONE [Citation74] in the region above the resolved resonance region. illustrates the total cross section of natural Ti in JENDL-5, together with JENDL-4.0 and measured data. The upper limit of energy region is 200 MeV, calculated by the optical model with the potential form of Koning and Delaroche [Citation120]. The potential parameters were modified as follows:
. The region below 7 MeV is the resolved and unresolved resonance ones as mentioned above.
The production cross sections for residual nuclides are included above 20 MeV in JENDL-5, rather than the cross sections for each reaction channel. shows the example of production cross sections for residual nuclides, which have the cross sections larger than 1 mb up to 200 MeV. Many nuclides are produced with increasing incident energy. In this case Si appears as the lightest nuclide.
The covariance data for 48Ti were evaluated with the CCONE-KALMAN system [Citation121], based on measured data of total, inelastic scattering, (), (
), (
), and (
) reactions. The covariance data were compiled for cross sections of all reaction channels below 20 MeV, except for partial cross sections of the (
), (
), (
), (
He), and (
) reactions.
2.2.2. Cr
The resolved resonance parameters of minor stable isotopes 50,54Cr, which have the natural abundances of 4.35% and 2.37%, respectively, were replaced with ENDF/B-VIII.0, in which the parameters were extracted by SAMMY.
For 50,52–54Cr the partial cross sections and energy-angle differential cross sections of (), (
), (
), (
He), and (
) reactions, which were removed in JENDL-4.0, are newly added in JENDL-5. New measured data of (
), (
) and (
) reactions on 50,52,53Cr were published after the release of JENDL-4.0. JENDL-5 still reasonably explains those data for (
) and (
) reactions. shows the present (
) reaction cross sections (i.e. same as JENDL-4.0) on 50,52,53Cr, together with measured data [Citation122]. There were no measured data for 52,53Cr before Khromyleva et al. published their data. The present data of 50,53Cr are consistent with them. On the other hand, it is found that JENDL-5 is slightly smaller than the data of Khromyleva et al. for 52Cr.
The new evaluations for 48,49,51Cr were performed by CCONE [Citation74]. The three unstable isotopes have half-lives of 21.6 h, 42.3 m and 27.7 d, respectively. Their nuclear data are mainly prepared for the activation estimation.
2.2.3. 55Mn
The resonance parameters were employed from JEFF-3.3, in which the parameters of the first three resonances were adjusted to reproduce the transmission and capture data of Fe-Mn alloy samples from the original data [Citation123]. In the region between 0.125 and 3.73 MeV which covers unresolved resonance region, the total cross section consists of the cross section derived from the unused and pseudo resonance parameters between 0.125 and 0.177 MeV, the cross section calculated by the optical model between 0.177 and 0.50 MeV and the data measured by Cierjacks et al. [Citation124] between 0.50 and 3.73 MeV.
The nuclear data above the resonances were evaluated with CCONE [Citation74]. The total cross sections and angular distributions of elastic scattering were reproduced by the coupled channel optical model. The potential form of Kunieda et al. [Citation125] was used with five coupled levels. The cross section of inelastic scattering to the first excited state was adopted from Lashuk et al. [Citation126].
In the total cross section of 55Mn in JENDL-5 is compared with JENDL-4.0 and measured data. The difference between JENDL-5 and JENDL-4.0 can be seen in the region between 0.125 and 0.50 MeV, where JENDL-4.0 is based on the data of low resolution measurements. On the other hand, the difference above 3.73 MeV comes from different methodologies in the JENDL-5 and JENDL-4.0 evaluations. In JENDL-5 the total cross section was derived by the coupled channel optical model explained above, while in JENDL-4.0 it was based on measured data [Citation124].
The covariance data were evaluated with the CCONE-KALMAN system, based on the measured data of total, capture, (), (
), (
) reactions. The covariances for all reaction channels were included.
2.2.4. Fe
The resonance parameters were taken from ENDF/B-VIII.0 for 54,57Fe and JEFF-3.3 for 58Fe. For 56Fe the resonance parameters were taken from JENDL-4.0, except for the negative resonance which was modified to reproduce the measured data of especially the capture cross section at thermal energy. This modification was made due to addition of the direct capture cross section calculated by the formulation [Citation127]. The valley of resonance cross section increases above 1 keV, compared to JENDL-4.0 in . The direct capture component was considered to be important to well explain the criticality benchmark of the HEU-MET-INTER-001 in ICSBEP, which is highly sensitive to the capture cross section around 24 keV [Citation128].
The cross section evaluations with CCONE [Citation74] were made by using available measured data. The optical model calculations were based on the potential form of Koning and Delaroche [Citation120]. The potential parameters were optimized to reproduce measured total cross sections and elastic scattering angular distributions of stable isotopes and natural Fe.
illustrates the comparison of neutron emission double differential cross sections in JENDL-5 with JENDL-4.0 and the data of Soda et al. [Citation129], in which the data at 30 to 150 deg. were obtained by using 18 MeV neutrons. The present results are in reasonable agreement with the measured data. JENDL-4.0 has large cross sections in the secondary energy range between 5 and 12 MeV. This comes from the large component of continuum inelastic scattering of 56Fe. The contributions of discrete inelastic scattering seen between 12 and 15 MeV in JENDL-4.0 are not appropriate to explain the measured data. The better reproduction of elastic component at 150 deg is attributed to the large elastic scattering angular distribution at backward angles in JENDL-5.
In the resolved resonance region the covariance data of JENDL-4.0 and JEFF-3.3 were adopted for 56,58Fe, respectively. The covariance evaluations for 56,58Fe were done with the CCONE-KALMAN system above the resolved resonance region. The used data were total, inelastic scattering, capture, (), (
), (
) and (
) reactions for 56Fe and total, capture, (
) and (
) reactions for 58Fe.
2.2.5. Co
In the resolved resonance region of stable 59Co the resonance parameters were reevaluated with the data analyzed by de Saussure et al. [Citation130]. The thermal capture cross section was evaluated to be 37.31 (21) b with available measured data. The present values are comparable with the data of JENDL-4.0, ENDF/B-VIII.0 and JEFF-3.3 within uncertainties. The total cross section in the unresolved resonance region was taken from the data measured with thick and thin samples by de Saussure et al. [Citation130].
The evaluation of 59Co was performed with CCONE [Citation74] above the resolved resonance region. The detailed evaluation methods and results are explained in Ref [Citation131]. The covariance was estimated with the CCONE-KALMAN system and the measured data of total, elastic scattering, inelastic scattering, capture, (), (
), (
), and (
) reactions.
For 60Co with half-life of 5.27 yr the resonance parameters were employed from Anufriev et al. [Citation132]. The elastic scattering and capture cross sections at the thermal energy were reproduced to be 4.4 b [Citation83] and 2 b [Citation133], respectively, with the modifications of neutron and gamma widths of negative resonance and scattering radius. shows the evaluated total, elastic scattering and capture cross sections. Above the resolved resonance region, the total cross section was evaluated by the coupled channel optical model, coupled with the ground and 1.22 MeV (spin-parity )-states. The potential form of Kunieda et al. [Citation125] was used.
2.2.6. Ni
The resonance parameters of 58,60,62Ni were replaced with the data of ENDF/B-VIII.0. For 61,64Ni the data of JENDL-4.0 carried over into JENDL-5.
The nuclear data other than the resonance parameters were evaluated with CCONE [Citation74], based on the measured data. The calculations of the total cross section and elastic scattering angular distributions were based on the coupled-channel optical model with the potential form of Kunieda et al. [Citation125] up to 200 MeV.
shows the () reaction cross section of 58Ni in JENDL-5, compared with JENDL-4.0, JENDL/A-96 [Citation134] and measured data. The present data show a good agreement with measured data. To the contrary, JENDL-4.0 and JENDL/A-96 underestimate the measured data between 6 and 9 MeV and between 7 and 12 MeV, respectively. The isomer production cross section of 58Co (half-life 9.10 h) via the (
) reaction of 58Ni is shown in . JENDL-5 and JENDL/A-96 reproduce the measured data well.
The covariance data of 58Ni were estimated with the CCONE-KALMAN system for the measured data of total, elastic scattering, inelastic scattering, capture, (), (
), (
), (
), (
), (
), (
) and (
) reactions. The obtained uncertainties of the (
) reaction cross section are also shown as hatched area in , in which the uncertainties of JENDL-4.0 are also drawn.
Figure 38. Comparison of () reaction cross section of 58Ni in JENDL-5 (solid line) with JENDL-4.0 (long-dashed line), JENDL/A-96 (short-dashed line) and measured data. Uncertainties of JENDL-5 and JENDL-4.0 are depicted with hatched areas. Asterisks stand for the data corrected for the cross sections of monitor reaction and/or gamma-ray branching ratio.
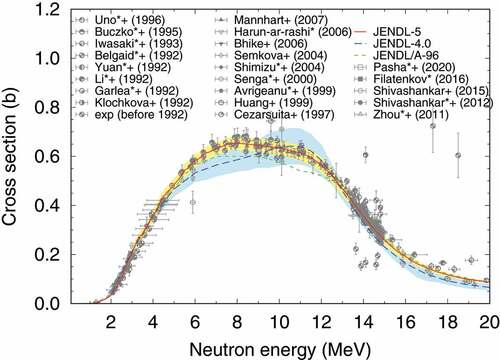
2.2.7. Cu
The resolved resonance parameters were taken from JEFF-3.3. The upper limits of the resolved resonance regions are 99.5 keV for both 63Cu and 65Cu. The angular distributions of elastic scattering up to 400 keV were also taken from JEFF-3.3. The negative resonance parameters of 63Cu were adjusted to reproduce the experimental capture cross sections by Weigand et al. [Citation135]. As illustrated in , the evaluated values of JENDL-5 are smaller than those of JENDL-4.0 around 100 eV. The revision improves results of the shielding benchmark test in copper system [Citation136], which has a large sensitivity for cross sections in low incident energies [Citation137].
Figure 40. Comparison of capture cross sections on 63Cu. the solid and dashed lines represent the data of JENDL-5 and JENDL-4.0, respectively.
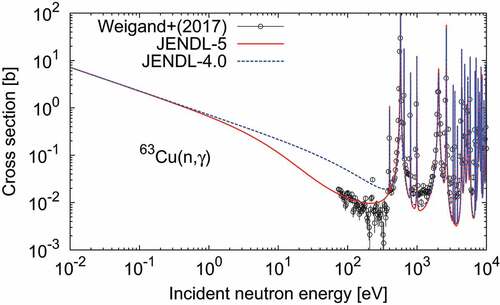
The data above the resolved resonance regions are based on our evaluation results already published [Citation138]. Three points were updated from Ref [Citation138]. First, the elastic scattering cross sections on 63,65Cu were decreased about 5% in the region from 100 keV to 5 MeV from the results of criticality benchmark tests in copper-containing systems. Second, the internal ratios of inelastic scattering for the first, second, and third excited states of 63,65Cu were improved. In Ref [Citation138], the evaluation was performed based on the experimental data given as the summation from the first to the third excited states [Citation139]. The ratios of transitions to each state were evaluated more correctly with reference to the experimental angular distributions of inelastic scattering for each state by Schmidt et al. [Citation140]. Finally, the reaction cross section on 63Cu was revised. As shown in , the data were re-evaluated so as to reproduce the experimental data around 14 MeV while maintaining an incident energy dependence similar to that in JENDL-4.0 below 10 MeV.
Figure 41. Comparison of reaction cross sections on 63Cu. the dash-dotted line is the previous evaluated result in Ref [Citation138]. The solid and dashed lines represent the data of JENDL-5 and JENDL-4.0, respectively.
![Figure 41. Comparison of (n,p) reaction cross sections on 63Cu. the dash-dotted line is the previous evaluated result in Ref [Citation138]. The solid and dashed lines represent the data of JENDL-5 and JENDL-4.0, respectively.](/cms/asset/f59d4930-a794-43ff-8445-e9d0261efbe8/tnst_a_2141903_f0041_oc.jpg)
2.2.8. Zn
The nuclear data of 62–72Zn above the resonance region were updated by CCONE [Citation74]. The resolved resonance parameters were taken from JENDL-4.0, which were newly evaluated at the time of JENDL-4.0 development.
For the simulation of radioisotope production (e.g. 64,67Cu), the cross sections in the energy region higher than 20 MeV are requisite, since the neutron source by the natC() reaction with 40 MeV-incident deuterons is considered [Citation141]. shows the (
) reaction cross sections of JENDL-5 and JENDL-4.0 compared with measured data published since the 1980s. This reaction produces 64Cu (half-life 12.7 h). The difference between JENDL-5 and JENDL-4.0 appears around 11 MeV. The cross section in JENDL-5 is in better agreement with the data of Mannhart and Schmidt [Citation142].
shows the production cross section of 67Cu for the 68Zn target in JENDL-5 with measured data, JENDL-4.0 and JENDL/HE-2007 [Citation143]. The evaluated cross sections below 20 MeV are consistent with the measured data. In contrast, there are no measured data above 20 MeV. The cross section in JENDL-5 is largely different from JENDL/HE-2007. The present cross section is favorable to explain the measured production yield of 67Cu [Citation141].
2.3. Fission products
2.3.1. Ga
The 69,71Ga data evaluated by Shibata et al. [Citation144] were adopted. The parameters of the negative resonance of JENDL-4.0 were adjusted to get better agreement with the thermal capture cross sections based on the evaluation with the available experimental data. Above the resonance region, the cross sections and secondary particle emissions were evaluated by the statistical model code POD [Citation145] taking into account the measured cross section of total, (), (
), (
) reactions as well as neutron emission spectra for natural Ga.
For the other unstable isotopes 67,72Ga, the cross sections, angular distribution of elastic scattering, and emission spectra of -ray and light-particles were evaluated by CCONE [Citation74] without resonance parameters.
2.3.2. Sr
The cross sections, angular distribution of elastic scattering, and emission spectra of -ray and light-particles of 82–90Sr above the resonance region were updated by CCONE [Citation74]. The resolved resonance parameters were taken from JENDL-4.0.
In JENDL-4.0 the evaluations were done by POD, and thus, the reaction channels included in the ENDF-6 format were limited. In JENDL-5 the partial or individual reaction cross sections and energy-angle double differential cross sections for charged particle emissions are stored, instead of using the inclusive production cross sections and energy-angle double differential cross sections for charged particle emissions.
2.3.3. 89Y
The presence of a new -wave resonance at resonance energy of 19.7 eV was reported by the capture measurement at ANNRI, using the NaI detector [Citation146]. The resonance parameter (neutron width
eV, gamma width
meV and spin
) analyzed by REFIT [Citation146] was newly added to the ones of JENDL-5.
2.3.4. Zr
The resonance parameters were updated by adopting available ones (e.g [Citation147,Citation148]) and the Reich-Moore form. The detailed evaluations are found in Ref [Citation149]. The whole evaluation methodology and evaluated results above the resolved resonance region for the stable Zr isotopes were published [Citation150]. The same methodology was applied to the evaluation of 93Zr.
For 90Zr covariance data in the resolved resonance region (up to 171 keV) were evaluated with the kernel approximation method [Citation151]. The uncertainty of resonance parameters was taken from Ref [Citation147]. Above the resolved resonance region, the CCONE-KALMAN system was employed to estimate the covariance data for all the cross sections and angular distribution in the form of average scattering angle cosine () [Citation121]. shows the average cross section, uncertainty and its correlation matrix for capture reaction of 90Zr in the energy range of 0.01 eV to 20 MeV, in which three regions are present: (a) below 2 keV, (b) between 2 and 171 keV, and (c) above 171 keV. The region (a) is evaluated by the uncertainties of measured thermal capture cross sections. The region (b) is evaluated by the uncertainties of resonance parameters. The region (c) is evaluated by the CCONE-KALMAN system with measured data. The region (b) has smaller uncertainties, especially between 2 and 70 keV, relative to the regions (a) and (c), due to small uncertainties of resonance parameters.
2.3.5. 93Nb
The parameters of five -wave and six
-wave resonances below 400 eV were revised from JENDL-4.0, by adopting the analyzed data of capture measurements at ANNRI [Citation152]. The resonance parameters above 400 eV are unchanged, except for 3.14 keV resonance. The thermal capture cross section at 300 K is adjusted to be 1.06 b, by taking into account two negative resonance parameters with spins
and 5, which is different from the analyzed results of Endo et al. [Citation152]. The present value is 7% smaller than JENDL-4.0 (1.14 b) but is consistent with the data of Krane [Citation153].
The nuclear data above the resonance region were updated by adopting the results [Citation154] evaluated by CCONE [Citation74]. In those evaluation, the cross section of the isomer productions for ,
,
and
reactions were taken into account.
2.3.6. 99Tc
A new capture measurement with a 99Tc sample by the TOF method was made by the NaI detector of ANNRI [Citation155]. Using these data, the resolved resonance parameters were evaluated with the REFIT code [Citation79,Citation156], in which the resolution function of ANNRI was taken into account [Citation157]. In this analysis the resonance parameters up to 540 eV were determined. Nevertheless, in the case that resonances with small peak cross sections cannot be identified due to high background level, the resonance parameters remained unchanged from those of JENDL-4.0.
shows the comparison of the capture cross section in JENDL-5 with that in JENDL-4.0 and measured data [Citation155] below 550 eV. Both cross sections are broadened by the resolution function of ANNRI. The capture cross section below 200 eV is almost the same as that of JENDL-4.0. The energy difference becomes visible with increasing energy, although the flight length and initial time delay in the present resonance analysis were fixed by the resonance energies of 197Au. It is found that the resonance energies of JENDL-4.0 are shifted to higher energies.
2.3.7. Ru
The evaluation for 96,98–106Ru was performed by Shibata et al. [Citation158]. The resonance parameters of 96,102Ru were updated. Above the resonance region, the evaluated data by POD were adopted for all isotopes. JENDL-5 adopted those data with merging the data of JENDL/ImPACT-2018 [Citation159] above 20 MeV.
2.3.8. 107,108Pd
The resolved resonance parameters of 107Pd were revised with the measured data of Nakamura et al. [Citation160], who reported that the first two resonances (3.9 and 5.2 eV) in JENDL-4.0 correspond to 105Pd and 109Ag, respectively. Then, the two resonances are removed in JENDL-5. In JENDL-5 and JENDL-4.0 broadened by the resolution function of ANNRI are depicted with the measured data of Nakamura et al. [Citation160], from which the contributions of the two resonances were subtracted. For the thermal capture cross section, prompt -ray analysis revealed that the lower limit is 9.16 (27) b [Citation161]. The TOF measurement provided the cross section around 10 b [Citation160]. The thermal value of Terada et al. [Citation162] normalized at the large 44-eV resonance peak of JENDL-4.0 was also larger than 10 b. It was reasonable compared to the data of Nakamura et al. [Citation161], and was adopted for JENDL-5. The data of Terada et al. [Citation162] were reproduced by adjusting the parameter of the negative resonance. The present thermal capture cross section results in 11 b.
The 2.9-eV -wave resonance of 108Pd in JENDL-4.0 was reported to be large, compared with the TOF data of Nakamura et al. [Citation160]. The parameters of JENDL-4.0 are 10
eV and 92 meV for neutron and gamma widths, respectively. The analysis of 2.9-eV resonance was performed with REFIT [Citation79,Citation156]. The resulting neutron and gamma widths are 1.92
eV and 114.5 meV, which are comparable to the values of 2.52
eV and 91.8 meV adopted in ENDF/B-VIII.0.
2.3.9. 113,115In
The meta-state production cross sections of inelastic scattering, and capture reactions for 113In and of inelastic scattering, capture and () reactions for 115In are known to be important for metrology applications such as dosimetry.
The cross sections of inelastic scattering, capture, () and (
) reactions for 113In and of inelastic scattering, (
), and (
) reactions for 115In were revised, together with ground- and meta-state production ones. In these revisions the ground- and meta-state and total production cross sections for each reaction were carefully evaluated with the measured data.
compares the meta-state production cross sections by inelastic scattering of In with JENDL/AD-2017 and available measured data. JENDL/AD-2017 has extremely large cross sections (over 400 mb) between 4 and 10.5 MeV, which are not consistent with the measured data. JENDL-5 resolves the discrepancy.
2.3.10. 112,118,124Sn
The TOF and pulse-height (PH) spectra measured at ANNRI [Citation163,Citation164] revealed that some resonances in JENDL-4.0 were missing (21- and 46-eV -wave resonances for 112Sn, 289-eV
-wave resonance for 118Sn, and 579- and 950-eV
-wave resonances for 124Sn). The 240-eV
-wave resonance for 112Sn was observed by the measurements at ANNRI. Nevertheless, it was not considered in JENDL-4.0. In the present evaluation the resolved resonance parameters of 112,118,124Sn were revised, based on the reported information. For the addition of the 240-eV resonance, the parameters were adopted from ENDF/B-VIII.0. The data above the resonance region were the same as those of JENDL-4.0.
2.3.11. Sb, Te and I
New evaluations for Sb, Te and I isotopes were made and their details have already been published elsewhere [Citation165–167]. The outline of the evaluations were briefly described in this section.
The data of Sb isotopes of JENDL-4.0 were substantially based on the old evaluations before JENDL-3.2 except 126Sb whose gamma-ray production data were missing. Shibata made new evaluations for Sb isotopes taking account of the latest experimental and theoretical knowledge [Citation165]. The evaluated data of 121,123–126Sb Shibata were adopted. While the resonance parameters of 121,123Sb were updated from JENDL-4.0, those of the other isotopes were unchanged. Above the resonance region, the whole of the data were reevaluated with POD [Citation145], resulting in the better agreements with the experimental data of the capture and cross sections as well as the neutron emission spectrum.
Regarding Te, the resolved resonance parameters were updated for JENDL-4.0. However, most of the other data of Te isotopes were based on the measurement and theoretical models more than 20 years ago. The new evaluation for was performed by Shibata [Citation166]. The thermal capture cross sections of
were updated based on the latest experimental data or a systematics from JENDL-4.0. CCONE [Citation74] was used for the revision of whole of the data above the resonance region. The inclusion of the direct reaction contribution in the nuclear reaction modeling lead to the remarkable improvement of the neutron emission spectrum for elemental Ta at 14 MeV.
The data of the I isotopes were also based on the old evaluation before JENDL-3.2 except the resolved resonance parameters that were updated for JENDL-4.0 and the gamma-ray emission data were missing. Shibata performed a new evaluation for 127–131,135I [Citation167]. While the resonance parameters were not changed, the cross sections and particle emission spectra were revised by the CCONE calculations. In addition to a reasonable result for the gamma-ray spectrum, the neutron emission spectra were significantly improved.
2.3.12. 133,135Cs
For production of 134Cs (half-life 2.1 y), which is one of the important indicators in post-irradiation experiments, the capture cross section of 133Cs has been focused on. The resolved resonance parameters of 133Cs below 600 eV were replaced with the data of Block et al. [Citation168]. The thermal capture cross section at 300 K is 32.2 b, which is larger than that of JENDL-4.0 by 11%.
For 135Cs, which is one of the well-known long-lived fission products, the resolved resonance parameters of the second -wave resonance were revised by REFIT [Citation79,Citation156], based on the capture cross section measurement at ANNRI [Citation169]. The thermal capture cross section was modified from JENDL-4.0 by adjusting the negative resonance parameter so as to reproduce the result (8.57 b) of activation measurement at the Kyoto University Research Reactor (KUR) [Citation170].
2.3.13. Ba
The resolved resonance parameters were the same as those of JENDL-4.0. The optical model calculations were performed with the coupled-channel method up to 200 MeV. The potential form of Kunieda et al. [Citation125] was employed with the parameter modifications to reproduce measured total cross sections, and elastic scattering cross sections and angular distributions for natural Ba. The evaluation of nuclear data for 128–140Ba above the resolved resonance regions was made with available measured data [Citation18] by using CCONE [Citation74]. The nuclear data of unstable isotopes () were prepared mainly for the purpose of activation estimation.
shows the evaluated () reaction cross sections of 138Ba for the total and meta-state productions of 135Xe. The total (
) reaction cross section of JENDL-5 is larger than that of JENDL-4.0 by 20% around 14 MeV. The production cross section of meta-state (half-life 15.3 m) was evaluated with data by activation measurements. The present result is in better agreement with the data of Filatenkov [Citation171] than JENDL/A-96.
Gamma-ray emission data of Ba isotopes are important for gamma-ray dose estimations in shielding design because Ba can be a constituent element of heavy concrete. Nevertheless, they are not available in JENDL-4.0. Gamma-ray emission data were evaluated together with the other nuclear data, and newly added to JENDL-5. illustrates the comparison of evaluated gamma-ray emission double differential cross sections (DDXs) for natural Ba at emission angle of 90 deg. and incident neutron energies of 5.5 to 8.5 MeV with the data measured by Perkin [Citation172]. The measured data of gamma-rays mainly produced by the inelastic scattering are well reproduced by the present evaluation.
Figure 48. Comparison of () reaction cross sections of 138Ba in JENDL-5 (total cross section by solid line and meta-stable state production cross section by short-dashed line) with JENDL-4.0 (total cross section by long-dashed line) and JENDL/A-96 (meta-stable state production cross section by dotted line). The measured total and meta-state production cross sections are drawn in black and gray, respectively.
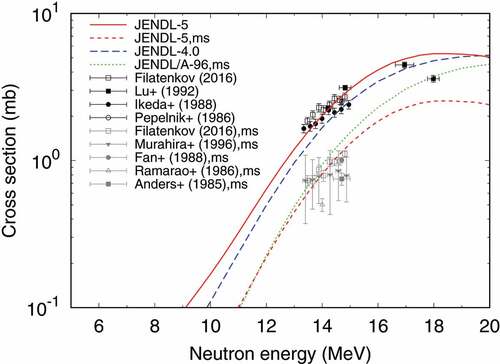
Figure 49. Comparison of evaluated double differential cross sections of gamma-ray emissions for natural Ba with the data of Perkin [Citation172]. The measurements were done with incident neutron energies of 5.5 to 8.5 MeV and emission angle of 90 deg.
![Figure 49. Comparison of evaluated double differential cross sections of gamma-ray emissions for natural Ba with the data of Perkin [Citation172]. The measurements were done with incident neutron energies of 5.5 to 8.5 MeV and emission angle of 90 deg.](/cms/asset/8e664939-ff33-40e6-bdde-7f0dad035dcc/tnst_a_2141903_f0049_oc.jpg)
2.3.14. La
The new evaluations of 135,137–141La were performed with CCONE [Citation74] above the resonance region, in which the resolved resonance parameters of 138,139La were taken from JENDL-4.0. The optical model with coupled-channel method was used with the potential form of Kunieda et al. [Citation125]. The potential parameters were modified by using measured total cross sections and elastic scattering data of natural La and 139La [Citation173–179]. The optical model calculations of La isotopes other than 139La were performed by the adjusted values and the mass-number dependence of potential parameters. The gamma-ray production data such as double differential cross sections were included for all reaction channels, though 138,139La in JENDL-4.0 do not have the gamma-ray production data. The other unstable La isotopes (135,137,141La) were evaluated mainly for activation estimation.
2.3.15. Pr
The neutron-induced reactions of 141,143Pr were evaluated [Citation180] after the release of JENDL-4.0 [Citation3]. In JENDL-5, the cross sections in the continuum region were revised by using a newer version of CCONE [Citation74] with corrections of parameters in level densities and -strength functions for M1 and E2 transitions to reproduce the experimental data.
2.3.16. 142Nd
The -wave resonances at 218, 235, 636, 956, 1119 and 1485 eV for 142Nd are assigned in JENDL-4.0. However, Katabuchi et al. [Citation181] elucidated the resonances come from 141Pr. In JENDL-5 the above six resonances were, therefore, removed.
2.3.17. 155,157Gd
Gadolinium is used as well-known burnable poison for the control of reactivity in nuclear reactors. Much attentions should be paid to the large capture cross sections of 155,157Gd for the research of reactor physics.
A new capture measurement of 155,157Gd samples with the TOF method was made by the NaI detector of ANNRI [Citation182]. The enriched samples were used, but impurities of 157Gd by 1.1% and 155Gd by 0.3% were contained in the 155,157Gd samples, respectively. Therefore, the resonance analyses of 155,157Gd below 1 eV were simultaneously done with REFIT, based on those data [Citation156]. The evaluated capture cross sections of 155,157Gd at 0 K are compared with JENDL-4.0 in , respectively, in which the bottom panels represent the capture cross section ratio of JENDL-5 to JENDL-4.0. The thermal capture cross sections (60.7 kb and 253.9 kb) are almost the same as those (60.9 kb and 254.1 kb) of JENDL-4.0 for 155,157Gd. Nevertheless, the cross section of 155Gd in JENDL-5 is smaller than JENDL-4.0 between the thermal energy and 1 eV. On the contrary, 157Gd in JENDL-5 has a larger cross section than in JENDL-4.0. Such an opposite change makes the effects to reactivity benchmark results small. In the region below the thermal energy the capture cross sections of both isotopes are smaller than those in JENDL-4.0. The resonance parameters other than the first resonance for 155,157Gd were taken from the data of Kang et al. [Citation183]. The data above the resonance regions were the same as those of JENDL-4.0.
2.4. Light nuclei
Nuclear data of the light nuclei are important not only for nuclear engineering but also for scientific/fundamental researches, e.g. on the stellar nucleosynthesis. Although new experimental and theoretical knowledge had been accumulated over the decades, the neutron cross sections had not been revised in the previous library, JENDL-4.0, for most of the light nuclei. For the present library, neutron cross sections were re-evaluated through the R-matrix [Citation184,Citation185] analysis with the AMUR code [Citation20,Citation21] in the resolved resonance region for 16O, 12,13C, 15N and 19F. The level structure information of the compound nuclei (such as excitation energy, spin and parity for each level) was taken from ENSDF [Citation186], but those data are re-examined for a number of cases so as to give a best fit to the measured cross sections listed in . We also estimated the covariance data of cross sections in the same energy region with a deterministic approach [Citation17].
Table 2. Measured cross sections used in the R-matrix analysis for the light nuclei (experimental data of angular distribution are marked with ).
2.4.1. 1H
JENDL-4.0 adopted the data of ENDF/B-VII.0 that did not include covariance data. Since data of 1H of ENDF/B-VIII.0 were updated with including covariance data, JENDL-5 adopted the data of ENDF/B-VIII.0 for 1H. In addition, the energy range was extended to 200 MeV by merging the data of JENDL-4.0/HE above 20 MeV.
2.4.2. 12C
An R-matrix analysis was performed for 13C compound system with AMUR by fitting experimental cross section of the 12C(,
) reaction [Citation187,Citation188] up to
MeV, at which the inelastic scattering to the first excited state initiates. During the analysis of the R-matrix, we also monitored the Legendre coefficients for the elastic scattering so as to reproduce experimental data of the differential cross sections with respect to angle. The direct and semi-direct neutron captures were taken into account with a potential model [Citation127]. The cross sections and differential cross sections in
MeV were replaced with those of natC of JENDL-4.0 considering a small contribution from the other isotope 13C (the natural abundance is 1.1%). From 20 MeV to 200 MeV, the evaluated data of JENDL-4.0/HE were merged into the data below 20 MeV. compares the evaluated and measured data of 12C(
,
) and 12C(
,
)13C cross sections. JENDL-5 reasonably follows the experimental data. For 12C(
,
)13C, JENDL-5 provides more realistic cross sections by considering the direct capture process above
keV than JENDL-4.0, which shows almost constant cross sections from
to
keV.
Figure 52. Comparison of the evaluated and measured 12C(,
) [Citation187,Citation188] and 12C(
,
)13C [Citation192,Citation200–202] cross sections.
![Figure 52. Comparison of the evaluated and measured 12C(n,tot) [Citation187,Citation188] and 12C(n,γ)13C [Citation192,Citation200–202] cross sections.](/cms/asset/8067b74c-8e99-4696-bf42-b87415228a5c/tnst_a_2141903_f0052_oc.jpg)
2.4.3. 13C
The isotopic data evaluation had not been performed until now in our general purpose libraries as cross section data had been given only for the natural element natC in, e.g. JENDL-4.0. To overcome such a drawback of the previous library, the data evaluation was newly performed for +13C from the resolved resonance energy region to 200 MeV.
In the case of 13C, the most important reaction may be 13C()14C which produces an unwished radioactive isotope 14C (
y) in nuclear engineering. The reaction is also known as ‘neutron poisons’ in the s-process – a neutron absorber in the nucleosynthesis. In the present study, the 13C(
)14C cross section was estimated with the Reich-Moore approximation [Citation203] putting the distant poles as pseudo resonances for p-waves to mimic the direct process. As illustrated in , we found that such a technical approach to the direct reaction, together with estimation of the
-ray width
for the first resonance (
) at
keV, was necessary to obtain evaluation consistent measured data [Citation204,Citation205] as shown in the top panel. Since those experimental data are given sparsely, we also used Maxwellian-Averaged Cross Sections (MACS) estimated by Wallner et al. [Citation205] to determine the resonance parameter values as shown in the bottom panel. Although MACS is not a pure measurements, we thought it could be a useful guide for the data evaluation in this case.
Figure 53. Comparison of the evaluated and measured/estimated [Citation204,Citation205] 13C()14C cross sections near the first resonance, where the present evaluation without the distant poles is also plotted for a reference purpose.
![Figure 53. Comparison of the evaluated and measured/estimated [Citation204,Citation205] 13C(n,γ)14C cross sections near the first resonance, where the present evaluation without the distant poles is also plotted for a reference purpose.](/cms/asset/d96b155d-beef-4fb2-a055-a930f5dc015e/tnst_a_2141903_f0053_oc.jpg)
For the capture cross section the uncertainties of 2.4% and 15% were assumed in the energy ranges of eV to 10 keV and 10 keV to 10 MeV, respectively, taking account of the uncertainties of available measured data [Citation192,Citation206,Citation207].
The new evaluation of nuclear data was made with CCONE [Citation74] between 10 and 200 MeV. The covariance was estimated by the CCONE-KALMAN system up to 20 MeV. The total cross section (neutron transmission coefficient) was calculated by the coupled-channel optical model. The coupled levels were ground state (spin-parity 1/2), 3.68 MeV (3/2
) and 7.55 MeV (5/2
) excited states with deformation parameters of
and
. The evaluated results of total, capture, (
) and (
) reaction cross sections with uncertainties are shown in , in which available measured data for each reaction are depicted. Since the neutron emission from excited levels of 13C was taken into account in the CCONE [Citation74] calculation, multiplicities in some of inelastic scattering data (e.g. MF6 and MT54) were different from unity, following neutron emission probabilities.
2.4.4. 15N
The nitride fuel is usually considered in a conceptual design of the ADS [Citation97] where 15N is assumed to be enriched to minimize the amount of 14C produced from the 14N()14C reaction. However, there exist discrepancies among the evaluated libraries in the world. For example, there is
difference between JENDL-4.0 and ENDF/B-VIII.0 in the elastic scattering cross section below 100 keV. We carried out an R-matrix analysis for
N up to
MeV to solve such an issue of inconsistency among the evaluated cross sections. We fitted measured total cross sections [Citation193] and angular distributions of elastic scattering [Citation194], simultaneously, where the recommendation value of Mughabghab was also included for elastic scattering cross section at
eV. Example results of the R-matrix fit are plotted in in which the present curves are consistent with both the measured data and the Mughabghab’s value, which may result in the most reasonable estimation of cross sections. For JENDL-5, the cross sections and differential cross sections of JENDL-4.0 were replaced with those new data up to
MeV.
Figure 55. Example comparisons of neutron total cross sections of 15N among the evaluations together with recommendation value by Mughabghab [Citation83] at eV and experimental data [Citation193] in a fast energy region.
![Figure 55. Example comparisons of neutron total cross sections of 15N among the evaluations together with recommendation value by Mughabghab [Citation83] at En=0.025 eV and experimental data [Citation193] in a fast energy region.](/cms/asset/a4fa2fec-44ab-40b1-9508-5cf822fc6c84/tnst_a_2141903_f0055_oc.jpg)
2.4.5. 16O
The cross section and covariance data were taken from works of one of the authors and his co-authors [Citation20,Citation21], in which an R-matrix analysis was performed for 17O compound system with AMUR by fitting experimental cross sections of the 16O(,
), (
,
), (
,
) and 13C(
,
)16O reactions, simultaneously. For JENDL-5, the cross sections and differential cross sections of JENDL-4.0 were replaced with those new data up to 6 MeV, where the (
,
) cross section was larger by
than that in the previous library (taken from ENDF/B-VII.0) due to a unitarity constraint from the R-matrix theory [Citation20,Citation21]. This approach itself is essentially the same as that taken in the ENDF/B-VIII.0 [Citation13] and one of the CIELO project [Citation14].
2.4.6. 17,18O
The nuclear data of 17,18O are not contained in JENDL-4.0 since the natural abundances of both isotopes are very small (0.038% and 0.205% for 17O and 18O, respectively). However, the 17O() reaction, for example, has an interest in neutron recycling from 16O in the
-process of extremely metal-poor asymptotic giant branch stars.
The new evaluations were performed for both isotopes. For 17O the resonance parameters were adopted from Mughabghab [Citation83], Wagemens et al. [Citation208], and Schatz et al. [Citation209]. The neutron width of negative -wave resonance was adjusted to reproduce the (
) reaction cross section in the energy range of 1 to 10 keV. The gamma widths of negative
-wave resonances were also fixed to match the capture cross section (0.67 mb) of Firestone et al. [Citation192]. The thermal (
) reaction cross section was fitted to be 244 mb [Citation210], which was obtained by the adjustment of alpha widths in negative
-wave resonances. A fictitious
-wave resonance was set at 2.38 MeV. The resonance is needed to take into account the
-wave resonance at 85 keV [Citation211].
The evaluated () reaction cross section of 17O in JENDL-5 is depicted with measured data in . The present data show a good agreement with measured data.
For 18O the resonance parameters were based on Mughabghab [Citation148]. They were, however, modified so as to reproduce the measured data of total cross section.
Above the resonance region the nuclear data of both isotopes were evaluated by CCONE [Citation74]. The spherical optical model potential was selected for 18O, for which measured data of elastic scattering angular distributions were available, and the same potential as 18O was applied to 17O. The adjustment of deformation parameters in the DWBA model was performed for 18O to match measured partial inelastic scattering data.
2.4.7. 19F
Fluorine is an important material for the nuclear fuel management, viz. the criticality safety. The element is also drawing attention for the design of the molten salt reactors as a constituent of the moderator/coolant materials. Therefore, it is meaningful to look into the existing data again to raise reliability of the evaluation up to the fast energy region. An R-matrix analysis was performed to evaluate neutron cross sections up to MeV. For the present evaluation, a new function was incorporated into the AMUR code so as to calculate angular distribution with an experimental energy resolution. It was because the 19F
differential cross sections measured by Elwyn et al. [Citation197] had been given with a large energy resolution (
keV). Indeed, it was impossible to analyze angular data for this isotope without such a new function. Although the consistent analysis to all the measurements listed in is still ongoing, we certainly achieved reasonable fits for the differential cross sections as illustrated in . The cross section data in JENDL-5 are the same as in JENDL-4.0 except for angular distributions for
,
and
up to 1 MeV which were taken from the present (but preliminary) R-matrix analysis.
Figure 57. Evaluated differential cross sections of the 19F reaction from different libraries which are compared with measured data [Citation197] where the estimated values were broadened by experimental resolution of 100 keV.
![Figure 57. Evaluated differential cross sections of the 19F(n,n0−2) reaction from different libraries which are compared with measured data [Citation197] where the estimated values were broadened by experimental resolution of 100 keV.](/cms/asset/bf62e30a-fd3a-4f3b-9734-922b3617dddb/tnst_a_2141903_f0057_oc.jpg)
2.4.8. Ne
JENDL-4.0 does not have the nuclear data of Ne isotopes, since neon is not considered to be so important for nuclear engineering. On the other hand, noble gas such as neon as well as helium may be usable as a coolant in a high-temperature gas-cooled reactor. Therefore, new evaluations of 20–22Ne were made to generate the nuclear data.
The resonance parameters of 20Ne were taken from the data [Citation148,Citation212–214]. The thermal capture cross section (36.8 mb) was fixed, referring to Bellmann et al. [Citation215]. The direct and semi-direct capture cross sections calculated by the formulation [Citation127] were added in the energy region of 10 eV to 20 MeV.
The resonance parameters of 21,22Ne were adopted from Heil et al. [Citation214]. The negative resonance parameters were changed to reproduce the thermal capture cross sections of 692 mb [Citation215] and 52.7 mb [Citation216] for 21,22Ne, respectively. The () reaction of 21Ne has a positive
-value. The cross section in the resonance region was given by
law, which explains the thermal cross section (0.18 mb [Citation217]).
The coupled-channel optical model with potential form of Kunieda et al. [Citation125] was adopted. The potential parameters were fixed by using measured total cross sections and elastic scattering angular distributions of natural Ne and 20Ne [Citation213,Citation218–220]. Applying the neutron transmission coefficients obtained above, the cross sections, angular distributions and energy-angle differential cross sections of emitted particles and gamma-ray for 20–22Ne above the resonance region were evaluated by CCONE [Citation74] up to 200 MeV.
2.4.9. 23,24Na
An R-matrix analysis was performed for Na system with AMUR by fitting experimental cross sections of the 23Na(
,
) reaction [Citation195,Citation221] and the 23Na(
,
) reaction [Citation222] up to
MeV. The cross sections of the 23Na(
,
) reactions above
MeV were replaced with the experimental data of Rouki et al. [Citation222]. For the cross section of the 23Na(
,
) reaction below
MeV, the evaluated data of JENDL-4.0, which are based on the experimental data, were used and for those above
MeV, cross sections calculated with CCONE [Citation74] were smoothly connected. The Legendre coefficients for the elastic scattering were taken from those evaluated by CCONE [Citation74]. This is because a more consistent result was obtained for benchmark calculations of the JASPER experiment [Citation223–225] and fast reactor systems discussed in Section 2.1.18 and when the Legendre coefficients of CCONE [Citation74] were used. The average elastic scattering angle obtained by the R-matrix analysis at the fast neutron energy region was slightly forward as compared with that by CCONE [Citation74], and this worsened the benchmark result. The other cross sections and differential cross sections in
MeV were replaced with those calculated with CCONE [Citation74]. Optical potentials and parameters in the statistical model were adopted from Ref [Citation226]. The direct and semi-direct neutron captures were taken into account with a potential model [Citation127]. Covariance data of total, elastic, inelastic, (
,
), and (
,
) reactions were taken from JENDL-4.0, while those of other reactions were newly evaluated by CCONE [Citation74] and KALMAN [Citation17].
Since no experimental data were available for 24Na, the cross sections were evaluated by using theoretical models. Below 30 keV, the cross sections were estimated with randomly generated resonance parameters [Citation227]. Above 30 keV, the cross sections were evaluated with CCONE [Citation74]. The direct and semi-direct neutron captures were taken into account with a potential model [Citation127]. The elastic and neutron capture cross sections at thermal neutron energy were determined so as to approximately reproduce b and
b, which were estimated from a scattering length
fm and a systematics given in Ref [Citation165], respectively, where
is the ratio of the target mass to that of neutron.
2.4.10. Cl
Much attention was paid to the nuclear data of Cl, which is one of the important elements for the fuel development of molten-salt reactors.
The resonance parameters of 35,37Cl were adopted from ENDF/B-VIII.0, which includes the data by the R-matrix limited format. The parameters of 35Cl have the channel widths of neutron, gamma, and proton (related to the ground-state production of 35S). The cross sections for the () reaction were calculated by AMUR together with five pseudo resonances, and added to JENDL-5 since there were no resonance data available in the neutron energy between 0.95 and 1.2 MeV. The (
) reaction cross section of 35Cl in the thermal energy region was expressed by
law and the thermal cross section was fixed to be 0.08 mb [Citation228].
For 36Cl the resonance parameters of Koehler et al. [Citation229] and de Smet et al. [Citation230] were adopted as the initial ones, in which -wave resonance was assumed in the case that Koehler et al. do not provide the orbital angular momentum. The gamma width was taken from the average
-wave value calculated by CCONE [Citation74]. The sum cross section of the (
) and (
) reactions measured by de Smet et al. was reproduced by AMUR as shown in . The sum widths were separated into proton and
ones to follow the analysed results of PH spectra [Citation229,Citation230]. The negative resonance parameters were adjusted to match the measured thermal cross sections of 0.59 mb and 47 mb for the (
) and (
) reactions, respectively [Citation231].
Above the resolved resonance region the CCONE code [Citation74] was used to calculate the nuclear data up to 200 MeV. The optical model with the potential form of Kunieda et al. [Citation125] was applied to evaluate the total cross sections and elastic scattering angular distribution. The DWBA model was also used for 35–37Cl to add the direct components to partial inelastic scattering cross sections.
2.4.11. Ar
From eV to
MeV, new evaluations were carried out for 36–39Ar and 41,42Ar. For 40Ar, the data below
MeV were taken from JENDL-4.0 (same as JENDL-3.3) and those from
to
MeV were from JENDL-4.0/HE [Citation232]. For 37Ar, the resonance parameters of Mughabghab [Citation83] were adopted up to
keV and the cross sections above
keV were calculated with CCONE [Citation74]. For the 37Ar(
,
) and (
,
) reactions, the cross sections were evaluated so as to reproduce the experimental data of Bieber [Citation233] and Goeminne [Citation234], respectively. The cross sections of 36,38,39,41,42Ar in the resonance region were estimated with randomly generated resonance parameters [Citation227]. The most likely parameter sets were determined by imposing a condition that the calculated cross sections reproduce either experimental data, evaluated data of Mughabghab [Citation83], or those estimated from a systematics [Citation165] as follows. For 36Ar, the experimental data of 36Ar(
,
) [Citation235] and the evaluated data of 36Ar(
,
)37Ar [Citation83] were used for the parameter determination. The cross sections were assumed to become continuum above 16 keV and those calculated with CCONE [Citation74] were used up to 200 MeV. For 38,39Ar, the evaluated data of elastic and capture cross sections at thermal neutron energy of Ref [Citation83] were used. The cross sections above
and
keV were evaluated with CCONE [Citation74] for 38Ar and 39Ar, respectively. For 41,42Ar, the elastic scattering cross sections at thermal neutron energy were estimated from the scattering length and the capture cross sections were from Mughabghab [Citation83] for 41Ar and a systematics [Citation165] for 42Ar. Above
keV, the cross sections were evaluated with CCONE [Citation74]. We also took into account the direct and semi-direct neutron captures, which were calculated with a potential model [Citation127]. There are experimental data of 36Ar(
,
)36Cl [Citation236] and 36Ar(
,
)33Si [Citation237,Citation238] reactions at thermal neutron energy. We estimated those cross sections by using
-law and smoothly connected them with those calculated with CCONE [Citation74] at
keV.
2.4.12. K
The resolved resonance parameters were taken from ENDF/B-VIII.0 for 39,41K. The () reaction of 39K has a positive
-value (1.36 MeV), and hence, the cross section in the resonance region is assumed to be
law, which was fixed to be 4.3 mb at thermal energy [Citation239].
No resolved resonance parameters were reported for 40K. The thermal cross sections of capture, () and (
) reactions, of which
-values are positive, were fixed to be 90, 4.4 and 0.39 b, respectively [Citation83,Citation240], following the
law up to 597 eV.
The CCONE code [Citation74] was used to evaluate the nuclear data above the resonance region for 39–43K. The total cross sections were evaluated up to 200 MeV by the coupled channel optical models coupled with the ground and a few excited states. It should be noted that the () reaction cross section of 41K in JENDL-4.0 is extremely smaller by a factor of 10 than in JENDL-5. JENDL-4.0 might fit the data of yrast isomers produced by the (
) reaction.
shows the () reaction cross section of 39K in JENDL-5, together with JENDL-4.0 and measured data. JENDL-4.0 reveals an increasing cross section above 6.55 MeV, which is the threshold energy of the (
) reaction. However, it is matched with the measured data having cross sections larger than 300 mb around 14 MeV. The present cross section drops above 9 MeV due to the increase of the (
) reaction one. The decrease gives a consistent result with the data of Foland et al. [Citation241] measured by mass separation of products. The large cross section in JENDL-4.0 may be attributed to that the data of Foland et al. [Citation241] were not available in the evaluation before 1987.
2.4.13. Covariance data
Since the AMUR code is able to estimate the covariance matrices of cross section data with a deterministic approach – the Kalman filtering method [Citation17] which is coupled to the R-matrix theory, we compiled the resulting matrices for those isotopes above, except for 19F for which a consistent resonance analysis is still ongoing. For 16O and 15N, our previous data were replaced with the present results in the same energy range of cross section described above, while the covariance of 13C we obtained is a new evaluation. illustrates the evaluations of the uncertainty and correlation matrices for the elastic scattering cross sections on 15N as an example, where those for JENDL-4.0 and JENDL-5 are compared in the energy range from eV to 8 MeV. The difference between the previous and present libraries is clearly seen: The present data shows a fundamental feature of the resonance theory while the previous data had been estimated only based on the experimental information in this case. Let us emphasize that the stripe pattern which is seen in correlation matrix we obtained certainly comes from the unitarity of the scattering matrix in the quantum mechanics, where correlation is minimized at the peak positions in principle due to constraints from the R-matrix theory [Citation20,Citation21].
2.5. Other nuclei
2.5.1. Er
The data of Er had been substantially based on the evaluation for JENDL-3.3. Only minor modification was made for JENDL-4.0 in the resonance region. To update the data not only for the resonance region but also for the fast neutrons, a new evaluation for 162,164,166,167,168,170Er was performed with the current nuclear reaction model code POD [Citation145] by Shibata [Citation242], in addition to revision of the resonance parameters of 166,167,168,170Er. Comparing with JENDL-4.0, the new evaluation provides better agreement with the experimental data of the cross sections of the reaction with charged particle emission such as and
.
2.5.2. Ho and Lu
The nuclear data of any Ho and Lu isotopes are not included in JENDL-4.0. The new evaluations of 161–166Ho and 169–177Lu were performed. The isotopes with stable and half-lives longer than 100 d are and
Lu, in which the isotopes with known resolved resonance parameters were limited to
and
. Their resonance parameters were taken from Mughabghab [Citation148] for 165Ho and 175Lu, Masyanov et al. [Citation243] for 166mHo, Erban et al. [Citation244] for 173Lu, Roig et al. [Citation245] for 176Lu, and Roig et al. [Citation246] for 177mLu.
For the other isotopes the law and constant cross sections were assumed for the capture reaction and elastic scattering, respectively, below half of the average
-wave level spacing calculated by level density models [Citation247,Citation248].
The nuclear data above the resonance region were evaluated by CCONE [Citation74] based on available measured data. The neutron transmission coefficients for targets were derived by using the coupled-channel optical model with the potential form of Kunieda et al. [Citation125]. As an example, shows that the () reaction cross section of 175Lu is compared with measured data. The production cross sections of the ground-state (half-life 3.31 yr) and meta-state (half-life 142 d) are also illustrated with corresponding measured data. The evaluated data give a good agreement with the measured data.
Figure 61. Comparisons of () reaction cross sections of 175Lu for ground-state (gs), meta-state (ms), and their summation with measured data. The data of Frehaut et al. [Citation249] are multiplied by 1.078 recommended by Vonach et al. [Citation250]. Asterisks stand for the data corrected for the cross sections of monitor reaction and/or gamma-ray branching ratio.
![Figure 61. Comparisons of (n,2n) reaction cross sections of 175Lu for ground-state (gs), meta-state (ms), and their summation with measured data. The data of Frehaut et al. [Citation249] are multiplied by 1.078 recommended by Vonach et al. [Citation250]. Asterisks stand for the data corrected for the cross sections of monitor reaction and/or gamma-ray branching ratio.](/cms/asset/95be5363-c44d-4f4f-b0c6-6ce1f9a2a2e1/tnst_a_2141903_f0061_oc.jpg)
2.5.3. 181Ta
The low energy neutron resonances of 181Ta have been used for thermometry of materials. On the other hand, the care of the amount of long-lived 182Ta (half-life 115 d) produced by the capture reaction was taken for nuclear decommissioning.
The resonance parameters up to 150 eV were analyzed by REFIT [Citation79,Citation156] with the transmission ratio and capture cross section, which were measured with the TOF method by the Li-glass and Ge detectors, respectively, at ANNRI [Citation251]. The resonance parameters of JENDL-4.0 were replaced for 28 -wave resonances in the range of 4.28 to 149.6 eV, including the two negative resonances. The thermal capture cross section at 300 K is 20.5 b, which is consistent with the data of activation measurements (e.g [Citation252]).
shows the neutron transmission of 181Ta in JENDL-5 with measured data and JENDL-4.0, where the transmission is calculated with areal density of (at/b) at temperature of 300 K. The total cross section of the first 4.28-eV resonance at 300 K is 17% larger than JENDL-4.0 at the peak. The second 10.3-eV resonance is shifted to smaller energy. It is found that the 4.28- and 10.3-eV resonances in JENDL-5 are in better agreement with the measured data than JENDL-4.0.
Figure 62. Comparison of neutron transmission of 181Ta in JENDL-5 (solid lines) with JENDL-4.0 (dashed line) and measured data. Transmission is calculated with the areal density of (at/b) at temperature of 300 K. The measured data were obtained by a natural sample, but the influence of 180mTa is negligible.
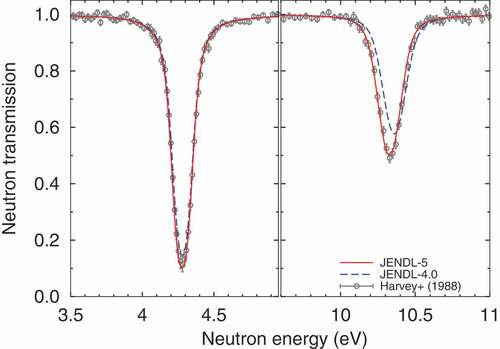
For the data above resonance region for 181Ta, the evaluation by Shibata [Citation253] was adopted, in which cross sections and emission spectra were obtained by CCONE [Citation74]. The evaluated data by Shibata were adopted for as well.
2.5.4. W
The nuclear data of tungsten had been updated in JENDL-4.0. In JENDL-5 partial data (cross sections and energy-angle differential cross sections) on the (), (
), (
), (
He) and (
) reactions were added. On the other hand, the angular distributions of elastic scattering were revised for 182,184,186W. These have an impact on criticality benchmarks of HEU-MET-FAST in ICSBEP, in which reactor cores are shielded by tungsten. The measured angular distributions of elastic scattering were fitted by the Legendre function, based on the data of Guenther et al. [Citation254] and Lister et al. [Citation255], who provided those at fine energy grids between 0.3 and 4 MeV. illustrates that the average scattering angle cosine (
) for angular distributions of elastic scattering has slightly stronger forward scattering than that of JENDL-4.0 in the region of 0.3 to 4 MeV. The representative results of fitted angular distributions for 186W at the incident energies of 2.3 and 3.37 MeV are shown in . The present angular distributions are in good agreement with the measured data of Guenther et al. [Citation254] well.
2.5.5. Re and Ir
The nuclear data of rhenium and iridium isotopes are not in JENDL-4.0. Nevertheless, they are important for nuclear engineering. The Re-included alloy is considered in terms of space and fusion reactor design. Iridium has been employed to monitor neutron fluence for thermal, fission (a few MeV), and 14-MeV neutrons [Citation256]. New evaluations of Re and Ir isotopes were performed for JENDL-5 [Citation257]. The resolved resonance parameters of 185,187Re and 191,192,193Ir were adopted from Mughabghab [Citation148] with modifications. The nuclear data above the resonance region were made by CCONE [Citation74]. The coupled-channel optical model was used for the calculations of the total cross sections and elastic scattering angular distributions up to 200 MeV with the potential form of Kunieda et al. [Citation125]. Some details for the evaluations of Re are found in Ref [Citation257].
represents the evaluated () reaction cross sections of 193Ir, compared to measured data. Radioactive 192Ir has two meta-states with half-lives of 1.45 m (m1) and 241 yr (m2). JENDL-5 explicitly gives the production cross sections of the three states, including the ground-state. The activation measurements reported the sum production cross sections of ground (g) and m1-states, and production cross section of m2-state. The (
) reaction cross sections of g+m1-state and m2-state productions in JENDL-5 reasonably explain the measured data within uncertainties between 11 and 16 MeV, while the production cross section of g+m1-state was overestimated below 11 MeV and slightly underestimated above 16 MeV.
Figure 65. Comparison of () reaction cross sections of 193Ir with measured data. The symbol g+m1 stands for the sum production of ground and meta-state (half-life 1.45 m). The symbol m2 represents the production of meta-state (half-life 241 yr). The evaluated data for total, g+m1 and m2-states are illustrated by solid, short-dashed and long-dashed lines, respectively. Asterisks stand for the data corrected for the cross sections of monitor reaction and/or gamma-ray branching ratio.
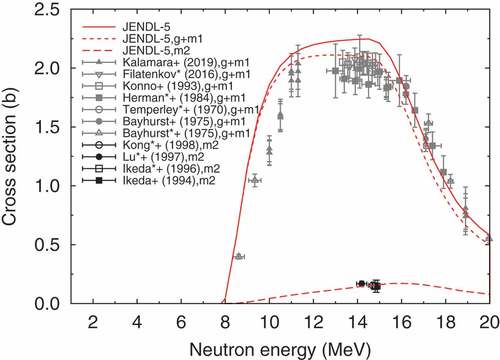
2.5.6. Pt
Due to the low priority until JENDL-4.0, the nuclear data of Pt isotopes had not been evaluated. Because JENDL-5 was intended to broaden the applicability, a new evaluation of Pt isotopes was performed by Shibata [Citation258]. The resonance parameters for 190,192,194,195,196,198Pt were evaluated based on the experimental data with modification for thermal capture cross sections. The CCONE code [Citation74] was applied to the evaluation for 189–198Pt above the resonance region by taking into account of the experimental data of the cross sections of ,
,
and
reactions as well as the gamma-ray emission spectrum.
2.5.7. 197Au
Natural gold consists of only 197Au. It is easily accessible for any measurements. Hence, the resonance energies of 197Au are frequently used to convert a TOF channel into a neutron energy. In this use the accuracy improvement of resonance energy is important for the resonance analysis.
It is confirmed that the capture cross section calculated with the resolved resonance parameters of JEFF-3.3 [Citation27] was consistent with that measured at ANNRI. Therefore, in JENDL-5 the resonance parameters were taken from JEFF-3.3, in which the upper energy for calculating cross sections from the resonance parameters is 2 keV. The resolved resonance region was slightly extended to 2.3 keV since its upper limit was 2.3 keV in JENDL-4.0. shows that the capture cross section averaged with group structure up to 2.6 keV is not drastically changed between 2 and 2.3 keV, compared with that of JENDL-4.0, although missing of resonances might be apparent. It seems that the overall change of averaged cross section between JENDL-5 and JENDL-4.0 is small, except for the valley of resonances in the region of 10 to 100 eV. The nuclear data above the resonance region remain unchanged from JENDL-4.0. The present capture cross section is also compared with the data of IAEA neutron standards 2017 [Citation28] at the thermal energy and in the energy region of 0.2 to 2.5 MeV. The difference exceeding the uncertainty in the IAEA neutron standards is found around 2 MeV.
Figure 66. Comparison of capture cross section of 197Au in JENDL-5 (solid line) with that in JENDL-4.0 (dashed line) and IAEA neutron standards 2017 [Citation28]. The capture cross sections are averaged with group structure in the energy region of 0.32 eV to 2.6 keV.
![Figure 66. Comparison of capture cross section of 197Au in JENDL-5 (solid line) with that in JENDL-4.0 (dashed line) and IAEA neutron standards 2017 [Citation28]. The capture cross sections are averaged with group structure in the energy region of 0.32 eV to 2.6 keV.](/cms/asset/5d8bd735-4679-413a-908f-f70b233a505e/tnst_a_2141903_f0066_oc.jpg)
2.5.8. Hg and Tl
Shibata evaluated 10 Hg isotopes of A = 195 to 204 for JENDL-5 to reflect the current experimental and theoretical knowledge [Citation259]. The resonance parameters were updated for 200,202,204Hg. Above the resonance region, CCONE [Citation74] was used for the evaluation of reaction cross sections and particle-emission spectra. The evaluated data of JENDL-4.0/HE were adopted above 20 MeV.
Shibata also made an evaluation for 203,204,205Tl [Citation260] whose data were missing in JENDL-4.0. While the resonance parameters of ENDF/B-VII.1 were adopted, the cross sections above the resonance region were evaluated with CCONE [Citation74] with parameter adjustments taking into account of the experimental data of natTl(), 203,205,natTl(
), 203,205Tl(
), 203Tl(
), 203,205Tl(
) and 203,205Tl(
).
2.5.9. 206,207,208Pb
Lead is one of the important elements for the development of target in an ADS [Citation77]. For 206Pb the thermal capture cross section was revised to be 29 mb by adjusting the gamma width of the negative resonance [Citation261]. The whole evaluation was made above the resonance region. Special care was paid to the cross sections of inelastic scattering, which are a large uncertainty factor in the analyses of reactor physics parameters (e.g. void reactivity) [Citation77]. The cross sections of inelastic scattering to excited-state in a lower neutron energy region were taken from the data of Negret et al. [Citation262]. shows the production cross sections of 803-keV gamma-ray by inelastic scattering in JENDL-5 with JENDL-4.0 and the data measured by Negret et al. [Citation262]. The 803-keV gamma-ray comes from the transition from the first excited state to the ground one. The measured data are production cross sections without the contribution from the decay of meta-state where the excitation energy is 2.20 MeV, the spin-parity is 7, and half-life is 125
s. The corresponding cross section evaluated by CCONE [Citation74] is also depicted by short-dashed line and is in reasonable agreement with the measured data. It should be noted that the cross section without the contribution from meta-state is not included in JENDL-5.
For 207Pb LEU-COMP-THERM benchmark analysis in ICSBEP with covariance data pointed out that the cross section of elastic scattering (11.5 b) at thermal energy in JENDL-4.0 was large. In the present evaluation the scattering radius and neutron width of the negative resonance were changed to decrease the cross section. As a result, its cross section is 10.8 b in JENDL-5. The thermal capture cross section was also updated to the data of Schillebeeckx et al. [Citation261]. The present value is 649 mb, which is 4.7% larger than JENDL-4.0.
For 208Pb the partial cross sections of inelastic scattering were replaced with the data measured by Mihailescu et al. [Citation263].
Figure 67. Comparison of 803 keV gamma-ray production cross sections for inelastic scattering of 206Pb in JENDL-5 (solid line) with JENDL-4.0 (long-dashed line). The measured data have no contribution from meta-state (excitation energy 2.20 MeV, spin-parity 7, and half-life 125
s). The corresponding cross section is shown by short-dashed line.
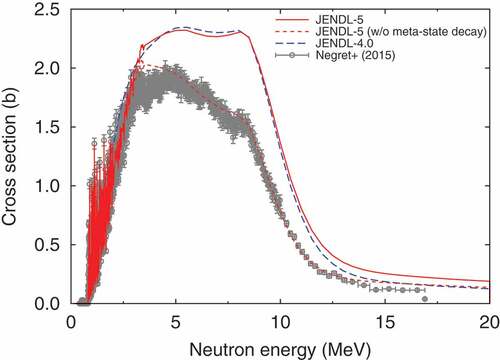
2.5.10. 209Bi
The nuclear data of 209Bi have received great interest from the target development of an ADS, as in the case of lead.
The resolved resonance parameters were updated in JENDL-4.0 and were kept unchanged in JENDL-5. The evaluation of 209Bi was performed by CCONE [Citation74] above the resonance region. Much attention was paid to the neutron production cross sections by inelastic scattering and () reaction, together with the gamma-ray production data from each reaction channel which are not contained in former JENDL general-purpose files, except for capture reaction.
compares the () reaction cross sections of JENDL-5 with JENDL-4.0 and measured data. The radioactive nuclide of 208Bi with long half-life of 0.37 Myr is produced by this reaction. The data measured by Frehaut et al. [Citation249] was multiplied by 1.077, which is recommended by Vonach et al. [Citation250]. It is found that the cross section in the region below 14 MeV is larger than that of JENDL-4.0, and the difference increases by 0.35 b at 10 MeV. The production cross section of meta-state (half-life of 2.58 msec) is also depicted with the corresponding measured data.
Figure 68. Comparison of () reaction cross section of 209Bi in JENDL-5 (solid line) with that in JENDL-4.0 (long-dashed line). The production cross section of meta-state (short-dashed line) is shown with measured data. The total and meta-state production cross sections are drawn in black and gray, respectively. The data of Frehaut et al. [Citation249] are multiplied by 1.077 recommended by Vonach et al. [Citation250]. Asterisks stand for the data corrected for the cross sections of monitor reaction and/or gamma-ray branching ratio.
![Figure 68. Comparison of (n,2n) reaction cross section of 209Bi in JENDL-5 (solid line) with that in JENDL-4.0 (long-dashed line). The production cross section of meta-state (short-dashed line) is shown with measured data. The total and meta-state production cross sections are drawn in black and gray, respectively. The data of Frehaut et al. [Citation249] are multiplied by 1.077 recommended by Vonach et al. [Citation250]. Asterisks stand for the data corrected for the cross sections of monitor reaction and/or gamma-ray branching ratio.](/cms/asset/4e6a5bc1-20c7-436d-b5b9-836f6daf6fb4/tnst_a_2141903_f0068_oc.jpg)
2.6. CCONE-based nuclear data
There are many nuclides listed in , for which resonance parameters were not available. In the low energy region of those nuclides the -shaped cross sections for reactions with positive
-values were adopted, except for elastic scattering whose cross section was assumed constant, calculated by
, where
is the scattering radius and its value was deduced from neighboring isotopes or
fm. The upper energy of the
-shaped cross section is determined to be half of average level spacing of
-wave resonances (
) [Citation264]. Above
the cross sections calculated by CCONE [Citation74] were discontinuously connected. The
-shaped cross section was fixed at the thermal energy. In the case of capture cross section, the values were derived by the formulation of Shibata [Citation165]. For fission, (
), and (
) reactions the ratio of each reaction cross section to capture one at
was assumed to the same as one at the thermal energy. The thermal cross sections of their reactions were calculated on the basis of thermal capture one. It should be noted that when measured thermal cross sections were available, the values were adopted.
Table 3. Nuclides containing CCONE-based nuclear data.
2.7. High energy region above 20 MeV
The upper energy limit of incident energy was extended to 200 MeV (partially 20 MeV) by merging JENDL-4.0/HE [Citation232] and JENDL/ImPACT-2018 [Citation159], or by evaluating newly.
Since the high energy files were mainly developed for the simulation of radiation transport, the recoil nucleus spectra, which were important for energy deposition to materials, were not provided in the evaluated files such as JENDL-4.0/HE and JENDL/ImPACT-2018. In addition, existence of the difficulty in the calculation of recoil spectra was also a reason of the lack of the data. A novel method to calculate the recoil spectra was developed [Citation265] and applied to the nuclides for all stable isotopes and the nuclides for the data exist in JENDL-4.0/HE and JENDL/ImPACT-2018 with the mass number larger than or equal to 10. The calculations were performed with CCONE [Citation266] with the default parameters and the results of the recoil spectra were added to residual nuclei in the evaluated files.
3. Thermal scattering law sublibrary
In JENDL-4.0 [Citation3], the thermal neutron scattering data were taken from ENDF/B-VI.8 [Citation267,Citation268] and ENDF/B-VII.0 [Citation269]. Similarly, the JENDL-5 sublibrary was partly based on ENDF/B-VIII.0 [Citation13]. The scattering data for 17 materials (aluminum, beryllium, beryllium oxide, crystalline graphite, reactor grade graphite with porosity concentration of , reactor grade graphite with porosity concentration of
, iron, light water ice, lucite, polyethylene, silicon carbide, silicon dioxide in alpha phase, silicon dioxide in beta phase, uranium dioxide, uranium mononitride, yttrium hydride, and zirconium hydride) were taken from ENDF/B-VIII.0. Besides, the data for 4 materials (liquid ortho-hydrogen, liquid para-hydrogen, liquid ortho-deuterium, and liquid para-deuterium) were taken from JEFF-3.3 [Citation27].
In JENDL-5, we generated the thermal scattering law data for 16 materials (light water, heavy water, liquid methane, solid methane, liquid ethanol, solid ethanol, liquid benzene, solid benzene, liquid toluene, solid toluene, liquid meta-xylene, solid meta-xylene, liquid mesitylene, solid mesitylene, liquid triphenylmethane, and solid triphenylmethane). The scattering data of organic molecules were evaluated mainly for the design of cold neutron sources. These data were obtained with the molecular dynamics (MD) simulations. In total, the thermal neutron scattering data for 37 materials were stored. Overviews of our evaluations are mentioned below.
3.1. Light water
The thermal scattering laws of H and O in light water were calculated by the incoherent scattering approximation with the self-scattering law represented by the Gaussian approximation [Citation8]. The density states for H and O were analyzed using the MD simulation code, GROMACS [Citation270] with the potential model, TIP4P/2005f [Citation271] under the constant temperature and pressure condition. The MD simulations were conducted at 56 temperatures between 270 and 800 K with the interval of 10 K including additional temperatures of 293.6 and 296 K. The temperature and pressure conditions are listed in . On calculating the scattering law, light water was treated as liquid at all temperatures (including supercritical phase above 650 K). Thus, the scattering law includes only incoherent inelastic scattering. Scattering law data were generated by our developed code, KUNSCA [Citation272], and calculated at 801 points of
between
and
and 402 points of
between
and
(including
) with equally logarithmic intervals. All the values of
and
were normalized by the thermal energy of 25.3 meV, and logarithm data of the symmetrized scattering law were stored in the ENDF-6 format [Citation12]. In , the total cross section for light water at room temperature is shown together with the experimental data [Citation273,Citation274], ENDF/B-VIII.0 [Citation13] and JENDL-4.0 [Citation3]. The present result is in good agreement with the experimental data and ENDF/B-VIII.0, and shows an improvement in the energy region below several meV as compared with JENDL-4.0. This is due to more appropriate estimation of the molecular motion of light water by using MD simulation in the present calculation.
Table 4. The temperature and pressure conditions for H2O.
3.2. Heavy water
The thermal scattering law data were generated for the deuterium and oxygen atoms in heavy water [Citation9,Citation275]. The neutron scattering by D consists of the incoherent and coherent components, while the scattering by O is entirely coherent. The incoherent scattering components were calculated with the atomic frequency distribution functions obtained from the MD simulations. To compute the coherent scattering, the Sköld approximation [Citation276] was applied. The coherent scattering laws were calculated with the atomic structure factors obtained from the MD simulations. The simulations were performed at 14 temperatures using the GROMACS [Citation270] code with the TIP4P/2005f [Citation271] force field with the pressure conditions in .
Table 5. The temperature and pressure conditions for D2O.
shows the total cross section for the D2O molecule at room temperature. The experimental data by Kropff et al. [Citation277] and the evaluated cross sections for ENDF/B-VIII.0 and JENDL-4.0 are also shown in . The present result almost overlaps the ENDF/B-VIII.0 evaluation by Damián et al. [Citation278,Citation279]. The main difference between the ENDF/B-VIII.0 and present calculation methods is that we did not employ the diffusion model and did not treat the intra-molecular vibrations as discrete oscillators. In , the present result is in good agreement with the experimental data as well as the ENDF/B-VIII.0 evaluation. The JENDL-4.0 evaluation, which was taken from the ENDF/B-VI.8 evaluation based on the incoherent approximation, is larger than other evaluations, for the neutron energy below 2 meV.
3.3. Organic molecules
The scattering laws for each element in seven organic molecules (methane
, ethanol
, benzene
, toluene
, meta-xylene
, mesitylene
, triphenylmethane
) were calculated by the incoherent scattering approximation with the self-scattering law represented by the Gaussian approximation [Citation8]. The density states for each element in the molecule were analyzed using the MD simulation code, GROMACS [Citation270] with the force field, CHARMM36 [Citation280] under the constant temperature and pressure conditions. The input topology of each molecule was generated using CHARMM-GUI [Citation281]. The MD calculations for each molecule were conducted at the temperature and pressure conditions listed in . On calculating the scattering law, each molecular system was treated as liquid (including the supercritical phase for benzene above 600 K) or as solid depending on the temperature. Thus, the scattering laws for liquid phase contain only incoherent inelastic scattering while those for solid phase also include incoherent elastic scattering in addition to the incoherent inelastic component. Scattering law data were generated by KUNSCA [Citation272]. The conditions for generating scattering law data such as the sampling of
and
were the same as light water.
Table 6. The temperature and pressure conditions for organic molecules.
In , total cross sections for methane, ethanol, benzene, toluene, meta-xylene, mesitylene and triphenylmethane are shown respectively. Experimental data [Citation273,Citation282–290] and the evaluated cross sections for ENDF/B-VIII.0 [Citation13] and JEFF-3.3 [Citation27] are also shown if their comparable data are available. As for the comparison with experiments, the present results of methane, ethanol, benzene, toluene, mesitylene and triphenylmethane are in reasonable agreement with the corresponding experimental data. As for the comparison with the evaluated cross sections, the present result of methane at 20 K shows an improvement below thermal energies as compared with ENDF/B-VIII.0, which apparently underestimates the experiment (). The result of benzene at 300 K is in consistent with ENDF/B-VIII.0 below 2 eV, which is the upper limit of the incident neutron energy for benzene in ENDF/B-VIII.0 (). The results of toluene and mesitylene at 20 K show an improvement above several hundreds of meV as compared with JEFF-3.3, which exhibits an apparent dip around 600 meV ().
4. Fission product yield sublibrary
A nucleus is disintegrated into two or more pieces by the neutron-induced and spontaneous fission, generating a peculiar fragment distribution. The information on the fission product distribution is important for estimating a composition in nuclear fuels during reactor operations, and is also related to the stable operation of reactors, the back-end of spent fuels, and so on. In particular, decay heat, which occupies about 7% of total energy generated in reactors and is a main issue of spent fuels, can be estimated from this information and decay data, which is introduced in the latter section. In the JENDL-5 fission product yield sublibrary, neutron-induced fission product yields of 227,229,232Th, 231Pa, 232–238U, 237,238Np, 238–242Pu, ,
, 249,251Cf, 254Es, and 255Fm (31 nuclei) at thermal and fast neutrons, and spontaneous fission product yields of 238U, 244,246,248Cm, 250,252Cf, 253Es, and 254,256Fm (9 nuclei) were newly evaluated [Citation7]. Neutron-induced fission product yields at 14 MeV were taken from JENDL/FPY-2011 [Citation291]. In addition, the spontaneous fission product yields of 242Cm were taken from JEFF-3.3 [Citation27].
The evaluation was carried out based on ‘real’ experimental data of independent fission product yields that are retrieved from EXFOR database [Citation18]. For unmeasured fissioning systems or data points, the independent fission product yields of JENDL/FPY-2011 or JEFF-3.1.1 were used as ‘pseudo’ experimental data. By assuming the mass chain yields evaluated by England and Rider [Citation292] as the initial guess, we determined the parameters concerning the most probable charge, damping factor of the shell correction with the real and pseudo experimental data. The parameter fitting was carried out through the generalized least squares (GLS) method. The characteristic points of the JENDL-5 fission product yield data were the introduction of the theoretically estimated nuclear shell correction in the independent fission product yields and of the Hauser-Feshbach statistical model in calculating the fraction of isomer states. The evaluated fission product yield data were also validated by comparing calculated results of decay heat and delayed neutron yield simulations of fission bursts, reactor anti-neutrino spectra, and PIE data of Mihama-3 and Takahama-3 examined by burn-up simulations [Citation293–295] with the experimental data, which showed that the calculated results with JENDL-5 were better than those of the previously evaluated data of JENDL/FPY-2011 [Citation291]. More details of the evaluation and comparison with JENDL/FPY-2011 can be found in [Citation7].
The JENDL-5 fission product yields are given in the ENDF-6 format (MF8), in which the cumulative yields (MF8 MT459) are calculated to ensure consistency between the independent yield data (MF8 MT454) and the JENDL-5 decay data. In this newly evaluated fission product yield data, the covariance data were also evaluated based on physical constraints, that is (1) conservation of mass number, (2) conservation of charge number, (3) normalization of independent yields, (4) normalization of heavier mass yields, and (5) constraints on England and Rider’s evaluation [Citation292]. Since no description rule of covariance data of fission yields is provided in the ENDF-6 format, we generated the data files in an arbitrary format, which can be found in the JENDL-5 fission product yield sublibrary. It should be noted that the uncertainties given in MF = 8/MT = 454, 459 correspond to the ones derived from the square root of diagonal elements of the covariance data.
5. Decay data sublibrary
Unstable nuclei are generated by nuclear reactions and fission, and decay toward stable nuclei. Accompanying the decay of unstable nuclei, various radioactive rays such as ,
, and
rays are emitted. Such decay information is of importance for estimating a radioactivity and decay heat of spent fuels, radioactive protections, and so on. The JENDL-5 decay data sublibrary is made so that up-to-date nuclear decay information can be obtained, and the decay heat and the radioactivity can be numerically estimated together with the JENDL-5 fission product yield sublibrary. Nuclei compiled in the JENDL-5 decay data range from neutron to Oganesson (
), amounting to 4071, which exceeds the number (3137) of nuclei compiled in the previous version JENDL/DDF-2015 [Citation296].
The feature of the JENDL-5 decay data is as follows. The major part is based on the evaluation of ENSDF [Citation297]. Careful attention was paid to the issues found in JENDL/DDF-2015, such as missing of nuclides (e.g. 235mU generated by the -decay of 239Pu) and radioactive-rays (e.g.
-ray generated after the
-decay of 239Np), and they are corrected in the JENDL-5 decay data. X-rays and internal conversion electrons are newly evaluated by considering the atomic relaxation. Theoretically calculated
,
, and delayed neutron spectra are newly added. The evaluated data of the IAEA coordinated research project (CRP) on ‘Reference Database of Beta-Delayed Neutron Emission Data’ [Citation298] and total absorption gamma-ray spectroscopy (TAGS) data [Citation299–302] are also taken into account. The verification is carried out by comparing the calculated radioactivities with those computed with other decay data libraries.
The outline of the development process of the decay data is as follows. We first transformed ENSDF (4 October 2019 version) to the ENDF-6 format with the RADLST code [Citation303] with several corrections. Since the original RADLST code does not distinguish decays to the ground and isomeric states when transforming to the ENDF-6 format, we reconstructed the decay probabilities by reading the ENSDF directly. There existed some nuclei that were not successfully processed via the RADLST code due to insufficient nuclear structure information. In this case, the missing data were newly evaluated or the decay data itself was taken from either ENDF/B-VIII.0 [Citation13] or JENDL/DDF-2015 [Citation296]. The numbers of nuclei imported from ENDF/B-VIII.0 and JENDL/DDF-2015 are 416 and 5, respectively. We have checked that the decay chain continues until stable nuclei or ends up with spontaneous fission for nuclei with . After the transformation to the ENDF-6 format, the following corrections are further carried out for the generated data.
• The newly evaluated data of delayed neutron emission and its spectra studied in the CRP [Citation298] were added.
• The average energies of photon and charged particle were replaced with those of the TAGS data [Citation299–302]. Those of JNDC committee [Citation304] for nuclei with a half-life less than s and greater than
year were also used.
• Discrete spectra were corrected or continuum spectra were inserted if energies obtained by integrating over and
-ray spectra do not meet the average energy.
• The decay probability of spontaneous fission and the number of fission neutron taken from ENDF/B-VIII.0 were inserted to the ENDF-6 format data.
• Spectra of X-rays and discrete electrons were re-calculated.
• Theoretically calculated -decay half-lives, delayed neutron branching ratios and delayed-neutron spectra were inserted for nuclei which have no experimental data.
• Recent half-life data measured at RI beam facilities [Citation305] were put into the data.
For predicting the -decay half-lives and
spectra, we adopted the Skyrme Hartree-Fock-Bogolibov (SHFB) and proton-neutron quasiparticle random-phase-approximation (
QRPA) [Citation306]. To calculate
spectra, delayed neutron branching ratios and its spectra, the Hauser-Feshbach statistical model implemented in CCONE [Citation266] was used. For the calculation of X-rays and discrete electron emissions, we used the RELAX code [Citation307] that computes the atomic relaxation. The production probability of atomic holes was evaluated from ENSDF [Citation297] and Table of Isotopes [Citation308].
The evaluated data of X-ray spectrum of 137mBa and discrete electron spectrum of 36K in the energy range of to
eV are illustrated in . The JENDL-5 decay data shows a reasonable agreement with the X-ray spectra at
keV of ENDF/B-VIII.0 and JEFF-3.3 and discrete electron spectra at
keV of ENDF/B-VIII.0. Several low-lying peaks less than
keV, which result from the RELAX code, are given for the JENDL-5 decay data, while they do not appear in ENDF/B-VIII.0 and JEFF-3.3. In JENDL/DDF-2015 [Citation296], no X-ray and discrete electron spectra are given in this energy range.
Figure 78. X-ray spectrum for 137mBa (top) and discrete electron spectrum for K (bottom). The spectra are smoothed with an arbitrary width.
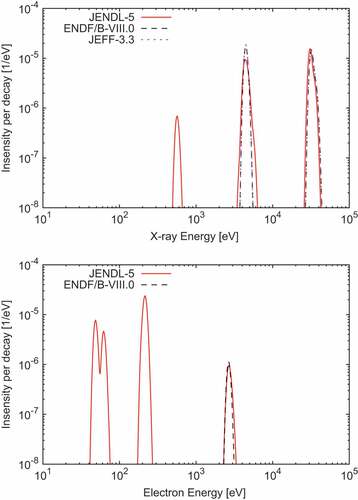
Decay heat of neutron-induced fission of instantaneous irradiation is calculated with the JENDL-5 fission product yield and decay data. Beta-ray decay heat for the 235U() and 239Pu(
) reactions are shown in as a function of time after the fission. The result of JENDL-5 is close to that calculated by JENDL/FPD-2011 and JENDL/FPY-2011 (FPD11-FPY11), reproducing the experimental data measured at the YAYOI reactor [Citation309]. shows the result of gamma-ray decay heat. The decay heat of JENDL-5 for
s becomes higher than that of FPD11-FPY11. We found that this is mainly due to the new TAGS data [Citation299–301]. In spite of that, JENDL-5 reproduces the experimental data of the YAYOI reactor [Citation310] almost in the same accuracy as FPD11-FPY11, although it slightly overestimates the gamma-ray decay heat of 239Pu(
) around
s.
Figure 79. Evaluated beta-ray decay heat for the 235U() (top) and 239Pu(
) (bottom) reactions. The result calculated by FPD11-FPY11 is also drawn for comparison. The experimental data is taken from Ref [Citation309].
![Figure 79. Evaluated beta-ray decay heat for the 235U(nfast,f) (top) and 239Pu(nfast,f) (bottom) reactions. The result calculated by FPD11-FPY11 is also drawn for comparison. The experimental data is taken from Ref [Citation309].](/cms/asset/9e10dcc6-44fb-42a4-96b4-7751975d7bd0/tnst_a_2141903_f0079_oc.jpg)
Figure 80. Same as , but of the gamma-ray. The experimental data is taken from Ref [Citation310]. .
![Figure 80. Same as Figure 79, but of the gamma-ray. The experimental data is taken from Ref [Citation310]. .](/cms/asset/5bc12213-f6a0-4b07-8fa4-cd3e280fb1c4/tnst_a_2141903_f0080_oc.jpg)
A noticeable improvement is obtained for the delayed neutron yield estimations. The results of instantaneous neutron irradiation for the 235U() and 239Pu(
) reactions are shown in as a function of time after fission burst. The delayed neutron yields of FPD11-FPY11 significantly overestimate the experimental data of Keepin et al. [Citation311], while JENDL-5 suppresses the overestimations, reproducing the experimental data within its uncertainty. We accept the overestimation for the gamma-ray decay heat seen in because a more consistent result to the experimental data through the decay heats and delayed neutron yields is obtained for JENDL-5.
Figure 81. Delayed neutron yields for the 235U() (top) and 239Pu(
) (bottom) reactions. The result calculated by FPD11-FPY11 is also drawn for comparison. The experimental data (the black solid line) and its uncertainty (shaded area) are taken from Ref [Citation311].
![Figure 81. Delayed neutron yields for the 235U(nfast,f) (top) and 239Pu(nfast,f) (bottom) reactions. The result calculated by FPD11-FPY11 is also drawn for comparison. The experimental data (the black solid line) and its uncertainty (shaded area) are taken from Ref [Citation311].](/cms/asset/5ab34882-a37d-4bb9-89f1-11a37bdeee9b/tnst_a_2141903_f0081_oc.jpg)
We also studied radioactivities and photon intensities from debris of the Fukushima-Daiichi nuclear power plant with different decay data libraries. The result of radioactivity is shown in the top panel of as a function of time after the accident, where the fuel compositions are taken from Ref [Citation313]. The radioactivity of JENDL/DDF-2015 is lower than those of other decay data from to
y because 235mU produced by
-decay from 239Pu is not included in it. The JENDL-5 decay data fixed this issue and shows a consistent result to ENDF/B-VIII.0 and JEFF-3.3. The calculated photon intensity of 14 energy groups is shown in the bottom panel of . The result of JENDL/DDF-2015 is lower than those of other decay data at the energy region less than
MeV and at energy from
to
MeV. The reasons of the deviation of the first energy group from
to
MeV and the second energy group from
to
MeV are due to the omission of X-rays of 137mBa and
-rays of 241Am, respectively. The underestimation at energy from
to
MeV is due to the omission of some
-rays of 106Rh. The JENDL-5 decay data fixed these issues, showing almost the same result as ENDF/B-VIII.0 and JEFF-3.3.
Figure 82. Radioactivity (top) and photon intensity of 14 energy groups (bottom) after the accident of Fukushima-Daiichi nuclear power plant calculated with different decay data libraries [Citation312]. Fuel compositions are taken from Ref [Citation313].
![Figure 82. Radioactivity (top) and photon intensity of 14 energy groups (bottom) after the accident of Fukushima-Daiichi nuclear power plant calculated with different decay data libraries [Citation312]. Fuel compositions are taken from Ref [Citation313].](/cms/asset/70a2796d-bbe2-480a-9d3a-193b5d65f062/tnst_a_2141903_f0082_oc.jpg)
6. Proton sublibrary
The most of the data are taken from our special purpose library JENDL-4.0/HE [Citation232] and JENDL/ImPACT-2018 [Citation159] for incident protons except for 9Be, 27Al, 93Nb and 197Au in which we included recent knowledge on the data evaluation.
6.1. Neutron production from9Be
The double differential cross section (DDX) of outgoing neutrons from the Be reaction is highly important for design of neutron sources by small accelerators. However, evaluated data in the world’s libraries do have a large uncertainty because measured data sets are scarce and discrepant whereas nuclear modeling is still on the stage of a scientific challenge for the light nuclei. Recently, Wakabayashi et al. [Citation314] proposed a function to estimate DDX of outgoing neutrons based on the available experimental data sets up to 12 MeV for incident protons on 9Be.
Firstly, we compiled calculated values by the function to the ENDF-6 format with reasonable numbers of the angle-energy nodes and suitable interpolation schemes. Secondly, the ACE (A Compact ENDF) format [Citation315] file was produced with the NJOY2016 code [Citation316] which was modifiedFootnote2 for JENDL-5. Through neutronics analysis with MCNP/PHITS to thick target yield (TTY) measurements (e.g. Ref [Citation319]), the function was reconfirmed to give more reasonable data than JENDL-4.0/HE and ENDF/B-VIII.0. We finally replaced the DDX data of 9Be in JENDL-4.0/HE with the ENDF format data of the function below 12 MeV, reducing the absolute cross sections by
from the function as illustrated in , which resulted in a better agreement with TTY measurements [Citation319]. The details of those analyses are reported elsewhere [Citation321].
Figure 83. Comparison of the evaluated cross sections of the 9Be(p,xn) reaction below 15 MeV together with experimental data taken from the EXFOR database [Citation18] where Bair’s data [Citation320] is a relative measurement.
![Figure 83. Comparison of the evaluated cross sections of the 9Be(p,xn) reaction below 15 MeV together with experimental data taken from the EXFOR database [Citation18] where Bair’s data [Citation320] is a relative measurement.](/cms/asset/7cba7de7-4de0-4208-afb7-0675336b11e9/tnst_a_2141903_f0083_oc.jpg)
6.2. Evaluation with machine learning technique
Quite recently, some of the authors developed a hybrid nuclear data estimator (G-HyND) [Citation76,Citation322] that is based on a machine learning technique with the Gaussian processes. It enables us to estimate cross sections from even the limited experimental data with a supplemental knowledge on nuclear model. Since it is not easy to fully understand nuclear reaction mechanisms that involve complicated processes (e.g. the statistical decay from highly excited states), isotope production cross sections of the previous high-energy libraries in the world had shown a large uncertainty or discrepancies from available measurements. In the present library, we adopted results of G-HyND for cross section of 9Be(,
)7Be, 27Al(
,
)7Be, 93Nb(
,
)7Be and 197Au(
,
)194Au on a trial basis. illustrates the 27Al(
,
)7Be cross sections estimated with our new code/approach as an example, where the present result shows overall fits to the available measurements [Citation324–330] and seems to give the most reasonable curve among the libraries. Although we are now struggling to seek a practical evaluation scheme with the G-HyND code, this workFootnote3 is the first-ever attempt to include evaluations by the machine learning technique in national nuclear data libraries. We understood that such a data-scientific method could be a powerful tool for producing an unprecedentedly elaborate library, and will expand such a new evaluation technique to data evaluation on other projectiles, isotopes and so forth.
Figure 84. Evaluated results of the 27Al(,
)7Be cross sections together with those from the other libraries [Citation232,Citation323] and the experimental data [Citation324–330] taken from the EXFOR database [Citation18].
![Figure 84. Evaluated results of the 27Al(p,x)7Be cross sections together with those from the other libraries [Citation232,Citation323] and the experimental data [Citation324–330] taken from the EXFOR database [Citation18].](/cms/asset/6513b093-947d-4aac-b980-a5217d2d6445/tnst_a_2141903_f0084_oc.jpg)
7. Deuteron sublibrary
The deuteron sublibrary provides the data of various reactions, the secondary particle emission, and residual nucleus production on 6,7Li, 9Be, 12,13C, 27Al, 63,65Cu, and 93Nb. The upper limit of incident energy is 200 MeV. The data for Li, Be and C isotopes were taken from JENDL/DEU-2020 [Citation331] and partially revised. The data for Al, Cu, and Nb isotopes were newly evaluated with the DEURACS code [Citation332], which was employed in the evaluation of JENDL/DEU-2020.
7.1. Li, Be, and C
Intensive fast neutron sources using deuteron accelerators have been proposed for various applications including fusion material irradiation facilities such as IFMIF [Citation333] and production of medical radioisotopes [Citation334]. In such accelerator-based neutron sources, the reactions on Li, Be, or C are generally employed to generate neutrons.
To contribute to the design study of neutron sources using deuteron accelerators, we have recently developed JENDL/DEU-2020, which is a deuteron nuclear data library for 6,7Li, 9Be, and 12,13C up to 200 MeV. In Ref [Citation331], it is shown that the transport simulations based on JENDL/DEU-2020 well reproduce the experimental data on neutron production for various combinations of target nuclei and incident energies. Moreover, the recent comprehensive benchmark test on a lithium target concludes that the transport simulations with JENDL/DEU-2020 are useful for the neutron yield estimation of IFMIF and similar facilities [Citation335]. From these results, almost all the data of JENDL/DEU-2020 were adopted as the data for Li, Be and C isotopes of the deuteron sublibrary.
In the deuteron sublibrary, the data of JENDL/DEU-2020 were modified for the following two points. Neither affects neutron emission data at incident energies below 50 MeV, which are of general interest in the design study of neutron sources. The first point is the 6Li reaction cross sections at low incident energies. As shown in , JENDL/DEU-2020 contains the non-physical small values from 3 to 5 MeV. This is because the
pickup reaction cross sections calculated by the Kalbach semi-empirical formula [Citation336] becomes too large in this energy region. In the deuteron sublibrary, the cross section was modified to have a smooth energy dependence as shown in . The second point is the double-differential cross sections (DDXs) for the 12C
reaction in the high incident energy region above 50 MeV. In JENDL/DEU-2020, the data at lower incident energies than intended were wrongly stored due to an error associated with data compiling. shows the comparison of the DDXs for the 12C
reaction at 50 MeV. The emission angle is 0 deg. The highest energy peak in the DDXs corresponds to the 12C
13Ng.s. reaction, and the
-value of the reaction is
MeV. The highest energy peak of JENDL/DEU-2020 is located at the lower energy than expected from the two-body kinematics. In the deuteron sublibrary, these data were revised to ones corresponding to the correct incident energies, as shown in .
7.2. Al, Cu, and Nb
Deuteron-induced neutron emission data from materials used for beam dumps and superconducting cavities are important from the viewpoint of shielding design of the accelerator facilities. Therefore, we newly evaluated the deuteron nuclear data for 27Al, 63,65Cu, and 93Nb up to 200 MeV with the DEURACS code.
Upon the evaluation of the data on 27Al, 63,65Cu, and 93Nb in the deuteron sublibrary, particular attention was paid to the neutron emission data. The spectroscopic factors (SFs) as the normalization factors for the transfer reaction cross sections were determined based on the evaluated values in Refs [Citation337-340] for each residual nucleus and its bound states. As claimed in Ref [Citation341], SF has ambiguity due to the calculation conditions for the transfer reaction. Therefore, the ratios among SFs corresponding to each bound state were obtained from the literatures, and then each SF was uniformly multiplied by a normalization factor so that the calculated neutron emission spectra reproduced the experimental ones. The model parameters such as level density parameters were also adjusted so that the calculation results reproduced experimental data.
As an example of the simulations using the deuteron sublibrary, neutron spectra from a thick natural copper target bombarded by a 5-MeV deuteron are shown in . The solid and dash-dotted lines represent the calculated results with PHITS-3.26 [Citation318] using the ACE files based on the deuteron sublibrary and TENDL-2021 [Citation323], respectively. The ACE files of the deuteron sublibrary were generated using the same modified NJOY2016 code as described in Section 6.1. TENDL-2021 is a nuclear data library evaluated using the TALYS code. As for the ACE files of TENDL-2021, the official ones available from the web page of TENDL-2021 were used. The dashed lines are the calculated results with the nuclear reaction models implemented in the PHITS code, namely, INCL-4.6 [Citation342] for the dynamical processes including the breakup processes and GEM [Citation343] for the evaporation process that subsequently occurs. The experimental data are taken from Ref [Citation344].
Figure 87. Experimental and calculated neutron spectra from a thick natural copper target bombarded by a 5-MeV deuteron. The number at the top of each plot denotes the emission angle.
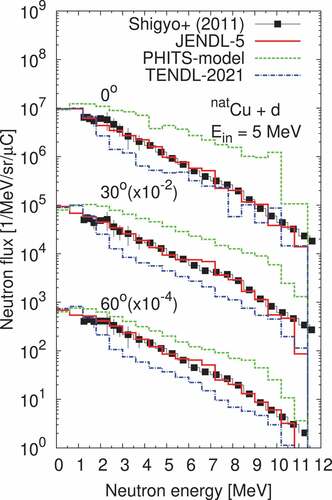
As illustrated in the figure, the simulations based on the deuteron sublibrary reproduce the experimental data better than those using the other models or data. These results are mainly attributed to the differences in the calculation for the breakup processes and the transfer reactions. This suggests the application limit of the INCL model implemented in PHITS for a low incident energy, and also indicates that the empirical model by Kalbach [Citation345] implemented in TALYS to calculate the breakup reactions does not work well under the present condition.
shows the results for a thick niobium target bombarded by a 9-MeV deuteron. The experimental data are taken from Ref [Citation344]. As is the case for a copper target presented in , the simulations based on the deuteron sublibrary are in good agreement with the experimental data. Moreover, it has been confirmed that the PHITS simulations using the deuteron sublibrary well reproduce the experimental neutron spectra for other incident energies. From these results, it is expected that the deuteron sublibrary makes a large contribution to applications such as shielding design of the deuteron accelerator facilities.
8. Alpha-particle sublibrary
We have revised JENDL/AN-2005 [Citation10] and adopted it as the alpha-particle sublibrary. The alpha-particle sublibrary provides the data of various reactions, the secondary particle emission, and residual nucleus production on 18 light nuclides from Li to Si isotopes, that is, 6,7Li, 9Be, 10,11B, 12,13C, 14,15N, 16,17,18O, 19F, 23Na, 27Al, and 28,29,30Si. The upper limit of incident energy is 15 MeV, which is the same as JENDL/AN-2005. The data on 16O, which are not contained in JENDL/AN-2005 since no neutron emission channel is open up to 15 MeV, were newly added. The remainder of this section describes the revision of JENDL/AN-2005 for the development of the alpha-particle sublibrary. The validation results of the alpha-particle sublibrary through the Monte Carlo transport simulations are also presented.
8.1. Revision of JENDL/AN-2005
The reaction data on light nuclides play an important role in the radiation shielding and criticality safety of backend facilities. In JENDL/AN-2005, neutron emission data of the
reactions were evaluated for 17 light nuclides from Li to Si in the energy region up to 15 MeV, which is a sufficiently large value as the energy of
-ray from the decay of trans-uranium nuclides. The evaluated
reaction cross sections show good agreement with the available experimental data, including the resonance structure. Therefore, the data of JENDL/AN-2005 were adopted as the
reaction cross sections in the alpha particle sublibrary.
As for individual exclusive reactions in the reactions, that is,
,
,
reactions, etc., their cross sections were mostly taken from JENDL/AN-2005. Exceptionally, only the cross sections on 9Be were modified based on the findings in Ref [Citation346] as described below. In JENDL/AN-2005, the
cross sections on 9Be are given as the sum of the
and
cross sections, and the ratios of the
underestimate the experimental data up to 7.9 MeV by Geiger et al. [Citation347]. Thus, the
cross sections were obtained by multiplying the
cross sections by the experimental ratios. The
cross sections were re-calculated by subtracting the new
cross sections from the
ones. The partial cross sections of the
reaction, that is, the
,
,
, and
reaction cross sections were also re-calculated so that the sum of them matches the new
cross section. The ratios among the partial cross sections were unchanged. As reported in Ref [Citation346], this modification is expected to improve the prediction accuracy of the neutron spectrum from the Am-Be neutron source. In this modification, the incident energy grids were increased as needed so that the sum of the
and
cross sections is equal to the evaluated
cross section of JENDL/AN-2005 in all incident energy ranges. Comparison of the
and
reaction cross sections of the alpha-particle sublibrary and JENDL/AN-2005 is presented in .
In JENDL/AN-2005, the partial cross sections of the reaction such as the
,
, and
cross sections are given explicitly. However, the outgoing neutron energy and angular distribution data are given using the Kalbach-Mann systematics [Citation348] and then only for the total
reaction. This choice gives a poor representation of the outgoing spectra of the reactions corresponding to low-lying excited states and according to Ref [Citation349], this was the cause of some poor simulation results based on JENDL/AN-2005 for the neutron spectra from thick targets.
To solve this problem, we gave the energy and angular distributions calculated with the CCONE code [Citation266] for all the partial reactions. The original energy and angular distribution data for the total
reaction were deleted. In JENDL/AN-2005, there are some exceptional cases where the energy and angular distribution are evaluated based on the experimental data (e.g. the
reaction). The above revisions were not made in these cases.
Comparison of the double-differential cross sections for the reactions on 11B is presented in . A significant difference is seen in the energy distributions between the alpha-particle sublibrary and JENDL/AN-2005. Note that the integrated values with respect to energy and angle are conserved in both. Three discrete peaks corresponding to the
,
, and
reactions are seen in the data of the alpha-particle sublibrary. These peaks are widened with a width of 0.1 MeV. On the other hand, the data of JENDL/AN-2005 have a continuous distribution. This is because the continuous energy distributions are given to the total
reaction. Validation of these spectra will be shown later.
Figure 90. Comparison of double-differential cross sections for the reaction on 11B at 6 MeV. Emission angle is 0 deg.
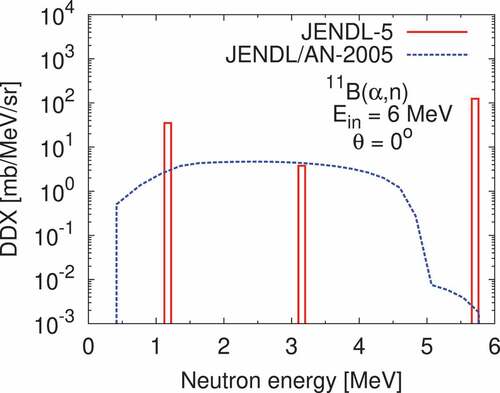
Moreover, we performed the CCONE calculation for reaction channels other than neutron emission channel, and added the calculated data. This allows the alpha-particle sublibrary to provide data for various reaction channels including -ray and charged particle emission. Since the elastic scattering data and the outgoing
-particle spectra from the
reaction are included, the sublibrary can be processed by the NJOY code to create a robust ACE file.
8.2. Validation by transport simulation
In order to investigate the validity of the alpha-particle sublibrary especially with respect to neutron emission spectrum, we perform a transport simulation for the neutron spectrum from -particle bombardment on a thick target.
illustrates experimental and calculated neutron spectra from a thick boron nitride (BN) target bombarded by a 5.5-MeV -particle. 5.5 MeV is a typical value for
-ray energy from trans-uranium nuclides. The experimental data are taken from Ref [Citation350]. The solid and dashed lines represent the calculated results with PHITS-3.26 using the ACE files based on the alpha-particle sublibrary and JENDL/AN-2005, respectively. As is the case with the proton and deuteron sublibraries, the ACE files were generated using the modified NJOY2016 code.
As shown in the figure, the prediction accuracy of the simulation is significantly improved by using the alpha-particle sublibrary. Since the threshold energies of the reactions on 14,15N are greater than 5.5 MeV, the neutron spectrum is a contribution from the
reactions on 10B(19.9%) and 11B(80.1%). This result demonstrates the validity of the revised neutron spectrum data.
Figure 91. Experimental and calculated neutron spectra from thick boron nitride (BN) target bombarded by a 5.5-MeV -particle.
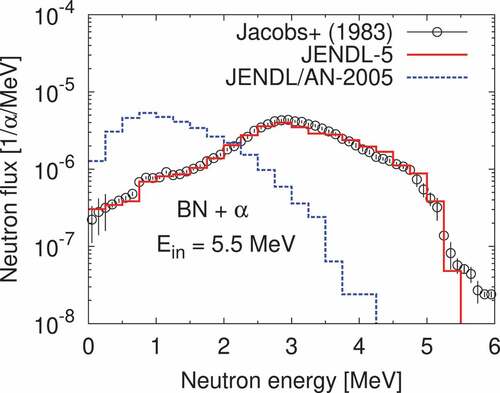
In conclusion, the alpha-particle sublibrary provides improved emitted neutron spectrum data while keeping the reaction cross section data of JENDL/AN-2005. Furthermore, the completeness has been enhanced by including data such as elastic scattering, production of
-rays and secondary particles making it a unique general purpose alpha-particle library.
9. Photonuclear sublibrary
The photonuclear sublibrary includes the nuclear data on photon-induced nuclear reactions for 2684 nuclides from 2H () to 266Lr (
). The upper limit of incident gamma-ray energy is 140 or 200 MeV. The data have photoabsorption, photofission, particle and residual-nuclide production cross sections, and energy-angle differential cross sections of emission-particles and gamma-rays. The photonuclear data in JENDL/PD-2016.1 carried over into JENDL-5 except for the nuclides mentioned below. In JENDL-5 the data modifications were focussed on three points.
The angular and energy distributions of emitted neutrons for the fission reaction were compiled in MF4 and MF5 of the ENDF-6 format, respectively, in JENDL/PD-2016.1. However, MF5 should be used for the neutron-incident case. In JENDL-5 the data were recompiled as energy-angle differential cross sections of emitted neutrons in MF6. It should be noted that the emission spectrum of delayed neutrons is left in MF5.
Some of emission-particle multiplicities of the nonelastic scattering reaction (MF6 and MT5 in the ENDF-6 format) except for the fission reaction in JENDL/PD-2016.1 were reported to be very large [Citation351]. This issue is attributable to the fission cross section comparable to the nonelastic scattering one with a prescription in which all of the neutron emitted before scission were stored as the nonelastic scattering reaction due to the difficulty in the calculation to separate them into fission or non-fission contributions. The large multiplicities did not have so important influences for practical use because the corresponding cross sections are very small. Nevertheless, the large neutron multiplicities were revised by an assumed function. shows the neutron multiplicity of 237Np in JENDL-5 compared with JENDL/PD-2016.1, which has large neutron multiplicity exceeding 60. The present values are smaller and more reasonable than JENDL/PD-2016.1.
Figure 92. Comparison of neutron emission multiplicity of 237Np in JENDL-5 (solid line) with JENDL/PD-2016.1 (dashed line).
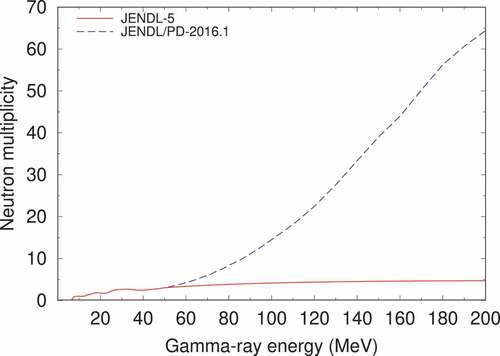
New nuclear data evaluations were made with CCONE [Citation74] for 89Y, 103Rh, 159Tb, 165Ho, 169Tm, 181Ta, 197Au and 209Bi. compares the total photoneutron cross section of 209Bi in JENDL-5 with JENDL/PD-2016.1 and the measured data. The cross section is the sum of the reaction cross sections related to neutron production. However, it is usually regarded as the sum cross sections of the (), (
), (
) reactions, and so on. The cross section in JENDL-5 corresponds to the latter case. The data of Gheorghe et al. [Citation352] were obtained with laser inverse-Compton scattering photons at the NewSUBARU facility. The data of Harvey et al. [Citation353] were multiplied by a correction factor of 1.22 recommended by Berman et al. [Citation354]. It is found that the present evaluation reproduces the data of Gheorghe et al., and JENDL-5 produces larger amounts of neutrons than JENDL/PD-2016.1.
10. Atomic sublibraries
The atomic sublibraries consist of photo-atomic, electro-atomic, and atomic relaxation data for -
, which are produced for transport calculations of photons and electrons. All the atomic sublibraries in JENDL-5 are taken from ENDF/B-VIII.0 library [Citation13] with a minor correction of the numerical representation in terms of the ENDF-6 format.
The photo-atomic sublibrary contains the total and subshell photoionization cross sections (MF23), coherent and incoherent scattering form factors, and so on (MF27). The electro-atomic sublibrary contains the total and subshell electron interaction cross sections, bremsstrahlung cross sections, and energy of the residual atom (MF23). In addition to them, the distribution of the secondary photon and electron emitted after electro-atomic reactions, and electron average energy loss are included (MF26). The atomic relaxation sublibrary deals with the radioactive information when an atom is ionized by a photon or an electron interactions (MF28). The ionized atom generally releases the energy by emitting photons (X-rays) and electrons until all the released energy becomes equal to the ionization energy. This file contains the information on subshell energies, emission energies of X-rays and electrons, and the transition probabilities. To estimate X-ray and electron spectra in the JENDL-5 decay data (Section 5), the information in the atomic relaxation sublibrary is applied.
11. Conclusion
A new general purpose nuclear data library JENDL-5 has been developed. The neutron data for a wide range of nuclides are intensively updated from JENDL-4.0 with increasing the number of nuclides to 795; JENDL-5 contains the data of almost all nuclides with half-lives longer than 1 day. The originally evaluated data of thermal scattering law and fission product yield are included for the first time in the JENDL project. JENDL-5 integrates special purpose files that have been released in the JENDL project so far to extend the applicable fields with updating and adding the data to reflect new experimental data and to satisfy requirements for particle transport calculations. JENDL-5 includes various kind of data such as for neutron activation, charged-particle and photon-induced reactions, etc. JENDL-5 provides those data as sublibraries of neutron, thermal scattering law, fission product yield, decay data, proton, deuteron, alpha-particle, and photonuclear as well as atomic related data.
Acknowledgement
We would like to thank Drs. Keiichi Shibata and Akira Ichihara of JAEA Nuclear Data Center for their contributions to the JENDL-5 evaluations and their documentations. We are grateful for the contributions on nuclear data evaluations and benchmark tests by working groups (WGs) of JENDL committee: Activation Cross Section Evaluation WG, Reactor Integral Test WG, Shielding Integral Test WG, WG on Evaluation of Nuclide Generation and Decay Heat.
FM acknowledges the advices and contributions from Drs. Masashi Kaneko, Yuta Kumagai of JAEA Research Group for Radiochemistry, Hideki Iimura of JAEA Nuclear Data Center, Masami Nakada, Manabu Fujita, Taichi Matsumura, Keisuke Okumura of JAEA CLADS, Takahiro Tachibana of Waseda University Senior High School, and Tadashi Yoshida of Tokyo Institute of Technology.
We would like to thank Drs. Yasuo Wakabayashi and Yujiro Ikeda of RIKEN for an useful discussion on the evaluation of the 9Be reaction.
The evaluation of the deuteron sublibrary was partially supported by JSPS KAKENHI Grant Number 19K15483.
Disclosure statement
No potential conflict of interest was reported by the author(s).
Additional information
Funding
Notes
1. The neutron width of the 4.26-eV resonance was mistakenly published, and then, corrected to be 0.0349 meV.
2. The code was modified to convert the LAW = 7 data in the ENDF format to not tabular energy-angle distribution data (LAW = 61 data) but laboratory energy-angle law data (LAW = 67 data) in the ACE format, because MCNP [Citation317] and PHITS [Citation318] calculations with charged particle incident ACE files with LAW = 61 data provide wrong results.
3. We also applied the equivalent machine learning approach to the evaluation of from 238U(n,f) as described in Sec. 2.1.5.
References
- Igarashi S, Nakagawa T, Kikuchi Y, et al. Japanese evaluated nuclear data library, version-1 – JENDL-1 –. Japan Atomic Energy Research Institute; 1979. JAERI 1261.
- Shibata K. JENDL: nuclear databases for science and technology. J Nucl Sci Technol. 2013;50(5):449–469. DOI:10.1080/00223131.2013.785282.
- Shibata K, Iwamoto O, Nakagawa T, et al. JENDL-4.0: a new library for nuclear science and engineering. J Nucl Sci Technol. 2011;48(1):1–30. DOI:10.1080/18811248.2011.9711675
- JENDL Japanese Evaluated Nuclear Data Library [Internet]. [cited. 2022 Mar 21]. Available from: https://wwwndc.jaea.go.jp/jendl/jendl.html.
- Advisory subcommittee on development of JENDL, JENDL committee. Final report of advisory subcommittee on development of JENDL; Guideline for developing next JENDL. JAEA-Review 2014-046, January. 2015, Japan Atomic Energy Agency. (in Japanese).
- JENDL-4.0u JENDL-4.0 updated files [Internet]. [cited. 2022 Mar 28]. Available from: https://wwwndc.jaea.go.jp/jendl/j40/update/.
- Tsubakihara K, Okumura S, Ishizuka C et al. Evaluation of fission product yields and associated covariance matrices. J Nucl Sci Technol. 2021;58:151–165.
- Abe Y, Tsuboi T, Tasaki S. Evaluation of the neutron scattering cross-section for light water by molecular dynamics. Nucl Instrum Methods Phys Res A. 2014;735:568–573.
- Ichihara A, Abe Y. Theoretical evaluation of neutron thermal scattering laws of heavy water for JENDL-5. Proc. 2021 Symposium on Nuclear Data; 2021 Nov 18–19; On line; 2022. p. 175–180.
- Murata T, Matsunobu H, Shibata K. Evaluation of the (α, xn) reaction data for JENDL/AN-2005. Japan Atomic Energy Agency. 2006. JAEA-Research 2006-052.
- Shibata K, Iwamoto N, Kunieda S, et al. Activation cross-section file for decommissioning of LWRs. JAEA-Conf 2016-004; 2015 Nov 19–20; Tokai-mura, Ibaraki, Japan; p. 47–52.
- Members of the Cross Sections Evaluation Working Group. ENDF-6 Formats Manual: Data Formats and Procedures for the Evaluated Nuclear Data Files ENDF/B-VI, ENDF/B-VII and ENDF/B-VIII. Upton (USA): Brookhaven National Laboratory; 2018, CSEWG Document ENDF-102, BNL-203218-2018-INRE.
- Brown D, Chadwick M, Capote R, et al. ENDF/B-VIII.0: the 8th major release of the nuclear reaction data library with CIELO-project cross sections, new standards and thermal scattering data. Nucl Data Sheets. 2018;148:1–142. Special Issue on Nuclear Reaction Data. doi:10.1016/j.nds.2018.02.001.
- International Co-operation in Nuclear Data Evaluation, an Extended Summary of the Collaborative International Evaluated Library Organisation (CIELO) Pilot Project. OECD 2019, NEA No. 7498, NUCLEAR ENERGY AGENCY ORGANISATION FOR ECONOMIC CO-OPERATION AND DEVELOPMENT.
- Igashira M, Kiyanagi Y, Oshima M. Nuclear data study at J-PARC BL04. Nucl Instrum Methods Phys Res A. 2009;600(1):332–334. DOI:10.1016/j.nima.2008.11.085.
- Schmittroth F, Schenter RE. Finite element basis in data adjustment. Nucl Sci Eng. 1980;74(3):168–177.
- Kawano T, Matsunobu H, Murata T, et al. Simultaneous evaluation of fission cross sections of uranium and plutonium isotopes for JENDL-3.3. J Nucl Sci Technol. 2000;37(4):327–334. DOI:10.1080/18811248.2000.9714902
- Otuka N, Dupont E, Semkova V, et al. Towards a more complete and accurate experimental nuclear reaction data library (EXFOR): international collaboration between Nuclear Reaction Data Centres. Nucl Data Sheets. 2014;120:272–276.
- Otuka N, Iwamoto O. EXFOR-based simultaneous evaluation of neutron-induced uranium and plutonium fission cross sections for JENDL-5. J Nucl Sci Technol. 2022;59(8):1004–1036.
- Kunieda S, Kawano T, Paris M, et al. R-matrix analysis for n +16O cross-sections up to En=6.0 MeV with covariances. Nucl Data Sheets. 2014;118:250–253. DOI:10.1016/j.nds.2014.04.050
- Kunieda S, Kawano T, Paris M, et al. Covariance of neutron cross sections for 16O through R-matrix analysis. Nucl Data Sheets. 2015;123:159.
- Harvey JA, Moore CL, Hill NW. Neutron total cross section of 233U from 0.01 to 1.0 eV. In: Fowler JL, Johnson CH, Bowman C, editors. Conf. on Nucl. Cross Sections F. Techn. Knoxville: Forgotten Books; 1979. p 690.
- Guber KH, Spencer RR, Leal LC, et al. High-resolution transmission measurements of 233U using a cooled sample at the temperature T = 11 K. Nucl Sci Eng. 2001;139(2):111–117. DOI:10.13182/NSE01-A2226
- Berthoumieux E, Abbondanno U, Aerts G, et al. Simultaneous measurement of the neutron capture and fission yields of 233U. In: ND 2007 - International Conference on Nuclear Data for Science and Technology, Nice, France, April 22-27 2007; 2007. p. 571–574.
- Calviani M, Praena J, Abbondanno U, et al. (n_TOF Collaboration). High-accuracy 233U(n, f) cross-section measurement at the white-neutron source n_TOF from near-thermal to 1 MeV neutron energy. Phys Rev C. 2009 Oct;80:044604. doi:10.1103/PhysRevC.80.044604.
- Weston LW, Gwin R, de Saussure G, et al. Neutron fission and capture cross-section measurements for uranium-233 in the energy region 0.02 to 1 eV. Nucl Sci Eng. 1970;42(2):143–149. DOI:10.13182/NSE70-A19495
- Plompen AJM, Cabellos O, De Saint Jean C, et al. The joint evaluated fission and fusion nuclear data library, JEFF-3.3. Eur Phys J A. 2020 Jul;56(7):181.
- Carlson A, Pronyaev V, Capote R, et al. Evaluation of the neutron data standards. Nucl Data Sheets. 2018;148:143–188. Special Issue on Nuclear Reaction Data. doi:10.1016/j.nds.2018.02.002.
- Nefedov VN, Starostov BI, Boytsov AA. High precision spectra measurements for neutrons arising from the fission of Cf-252, U-233, U-235, Pu-239 in the energy range 0.04 - 5 MeV, Proceedings of Neutron Physics Conference, Kiev, 2-6 Oct 1983, Vol.2, 1983 p.285, USSR.
- Boytsov AA, Semenov AF, Starostov BI, et al. Relative measurements of the spectra of prompt fission neutrons for thermal neutron fission of the nuclei U-233, U-235, Pu-239 in the energy range 0.01 - 5 MeV, Proceedings of Neutron Physics Conference, Kiev, 2-6 Oct 1983, Vol.2, 1983 p.294, USSR.
- Starostov BI, Nefedov VN, Boytsov AA. Precision measurements of 252Cf, 233U+nth, 235U+nth and 239Pu+nth prompt fission neutron spectra (PFNS) in the energy range 2 - 11 MeV. Proceedings of Neutron Physics Conference, Kiev, 2-6 Oct 1983, Vol.2, 1983 p.290, USSR. ( English translation INDC(CCP)-0485).
- Starostov BI, Nefedov VN, Bojtsov AA Prompt neutron spectra from fission of 233U, 235U and 239Pu by thermal neutrons and from spontaneous fission of 252Cf in the 0.01 - 12 MeV energy range Vop. At. Nauki i Tekhn, Ser. Yaderno-Reak. Konstanty, Issue.3, p.16, 1958 Russia.
- Lajtai A, Kecskemeti J, Safar J, et al. Energy spectrum measurements of neutrons for energies 30 keV - 4 MeV from thermal fission of main fuel elements, Conf. on Nuclear Data for Basic and Appl. Sci., Santa Fe 1985, Vol.1, 1985 p.613, USA.
- Kornilov N, Hambsch F-J, Fabry I et al. The 235U(n, f) prompt fission neutron spectrum at 100 K input neutron energy. Nucl Sci Eng. 2010;165(1):117–127.
- Iwamoto O. Systematics of prompt fission neutron spectra. J Nucl Sci Technol. 2008;45(9):910–919. DOI:10.1080/18811248.2008.9711492.
- Gwin R, Spencer RR, Ingle RW. Measurements of the energy dependence of prompt neutron emission from 233U, 235U, 239Pu, and 241Pu for En = 0.005 to 10 ev relative to emission from spontaneous fission of 252Cf. Nucl Sci Eng. 1984;87(4):381–404.
- LaBauve RJ Highly enriched uranium sphere (GODIVA), International Handbook of Evaluated Criticality Safety Experiments (ICSBEP), HEU-MET-FAST-001; 2014. NEA/NSC/DOC(95)03, 32p.
- Mosteller RD Water-reflected, highly enriched uranium sphere, International Handbook of Evaluated Criticality Safety Experiments (ICSBEP), HEU-MET-FAST-004; 2014. NEA/NSC/DOC(95)03, 40p.
- Brewer RW Uranium-235 sphere reflected by normal uranium using FLATTOP, International Handbook of Evaluated Criticality Safety Experiments (ICSBEP), HEU-MET-FAST-028; 2014. NEA/NSC/DOC(95)03, 22p.
- Sapir JL, Krohn BJ, Mosteller RD.Big ten: a large, mixed-uranium-metal cylindrical core with 10% average 235U enrichment, surrounded by a thick 238U reflector, International Handbook of Evaluated Criticality Safety Experiments (ICSBEP), IEU-MET-FAST-007; 2014. NEA/NSC/DOC(95)03, 145p.
- Favorite JA, Brewer RW. Bare sphere of plutonium-239 metal (4.5 at.% 240Pu, 1.02 wt.% Ga), revision 4, International Handbook of Evaluated Criticality Safety Experiments (ICSBEP), PU, International Handbook of Evaluated Criticality Safety Experiments (ICSBEP), PUMETFAST001; 001; 2014. NEA/NSC/DOC(95)03, 167p.
- O’Dell RD. 240Pu JEZEBEL: bare sphere plutonium-239 metal (20.1 at.% 240Pu, 1.01 wt.% Ga), International Handbook of Evaluated Criticality Safety Experiments (ICSBEP), PU-MET-FAST-002; 2014. NEA/NSC/DOC(95)03, 22p.
- Brewer RW. Plutonium sphere reflected by normal uranium using FLATTOP, International Handbook of Evaluated Criticality Safety Experiments (ICSBEP), PU-MET-FAST-006; 2014. NEA/NSC/DOC(95)03, 23p.
- Brewer RW. Benchmark critical experiment of a thorium reflected plutonium sphere, International Handbook of Evaluated Criticality Safety Experiments (ICSBEP), PU-MET-FAST-008; 2014. NEA/NSC/DOC(95)03, 23p.
- Brewer RW. Benchmark critical experiment of a water reflected alpha-phase plutonium sphere, International Handbook of Evaluated Criticality Safety Experiments (ICSBEP), PU-MET-FAST-011; 2014. NEA/NSC/DOC(95)03, 34p.
- Ikegami T. ZPPR-9 experiment: a 650 MWe-class sodium-cooled MOX-fueled FBR core mock-up critical experiment with clean core of two homogeneous zones, International Handbook of Evaluated Reactor Physics Benchmark Experiments (IRPhE), ZPPR-LMFR-EXP-002; 2010. NEA/NSC/DOC(2006)1.
- Ishikawa M, Ikegami T, Sanda T. ZPPR benchmarks for large LMFBR core physics from JUPITER cooperative program between United states and japan. Nucl Sci Eng. 2014;178(3):335–349. DOI:10.13182/NSE14-9.
- Yokoyama K, Shono A, Ishikawa M. Reevaluation of experimental data and analysis on experimental fast reactor JOYO MK-I performance tests. Nucl Sci Eng. 2007;157(3):249–263. DOI:10.13182/NSE06-109.
- Yokoyama K, Shono A. Japan’s experimental fast reactor Joyo MK-I core: sodium-cooled uranium-plutonium mixed oxide fueled fast core surrounded by UO2 blanket, International Handbook of Evaluated Reactor Physics Benchmark Experiments (IRPhE), JOYO, International Handbook of Evaluated Reactor Physics Benchmark Experiments (IRPhE), JOYOLMFRRESR001; 001; 2010. NEA/NSC/DOC(2006)1.
- Yokoayama K, Numata K, Shono A, et al. Reevaluation of experimental data and analysis with the latest reactor physics calculation method on fast experimental reactor “JOYO” MK-I perfomance tests. Japan Nuclear Cycle Development Institute; 2005. JNC TN9400 2005024. (in Japanese).
- Manturov G, Kochetkov A, Semenov M, et al. BFS-62-3A experiment: fast reactor core with u and U-Pu fuel of 17% enrichment and partial stainless steel reflector, International Handbook of Evaluated Reactor Physics Benchmark Experiments (IRPhE), BFS2-LMFR-EXP-001; 2010. NEA/NSC/DOC(2006)1.
- Hazama T, Shono A, Iwai T, et al. Analyses on the BFS critical experiments –an analysis on the BFS-62-3A and 62-4 cores–. Japan Nuclear Cycle Development Institute; 2002. JNC TN9400 2002036. (in Japanese).
- Kochetkov A, Matveenko I, Matveev V, et al. BN-600 hybrid core mock-up at BFS-2 critical facility. In: PHYSOR 2002, 7C-03, Seoul, Korea, October 7-10, 2002; 2002.
- Shono A, Sugino K, Hazama T, et al. Experimental analysis results on BN-600 mock-up core characteristics at the BFS-2 critical facility. In: PHYSOR 2002, 7C-04, Seoul, Korea, October 7-10, 2002; 2002.
- Ishikawa M, McKnight RD. ZPPR-10A experiment: a 650 MWe-class sodium-cooled MOX-fueled FBR homogeneous core mock-up critical experiment with two enrichment zones and nineteen control rod positions, International Handbook of Evaluated Reactor Physics Benchmark Experiments (IRPhE), ZPPR-LMFR-EXP-001; 2010. NEA/NSC/DOC(2006)1.
- Sanda T. ZPPR-10B experiment: a 650 MWe-class sodium-cooled MOX-fueled FBR homogeneous core mock-up critical experiment with two enrichment zones, seven control rods and twelve control rod positions, International Handbook of Evaluated Reactor Physics Benchmark Experiments (IRPhE), ZPPR-LMFR-EXP-005; 2010. NEA/NSC/DOC(2006)1.
- Sanda T. ZPPR-10C experiment: a 800 MWe-class sodium-cooled MOX-fueled FBR homogeneous core mock-up critical experiment with two enrichment zones and nineteen control rod positions, International Handbook of Evaluated Reactor Physics Benchmark Experiments (IRPhE), ZPPR-LMFR-EXP-006; 2010. NEA/NSC/DOC(2006)1.
- Sanda T. ZPPR-13A experiment: a 650 MWe-class sodium-cooled MOX-fueled FBR radial heterogeneous core mock-up critical experiment with central blanket zone and two internal blanket rings, International Handbook of Evaluated Reactor Physics Benchmark Experiments (IRPhE), ZPPR-LMFR-EXP-007; 2010. NEA/NSC/DOC(2006)1.
- Sanda T. ZPPR-18A experiment: a 1,000 MWe-class sodium-cooled MOX-fueled FBR core mock-up critical experiment with two-homogeneous zones and control-rod withdrawal, where enriched uranium is used with the shape of a sector in the outer core, International Handbook of Evaluated Reactor Physics Benchmark Experiments (IRPhE), ZPPR-LMFR-EXP-003; 2010. NEA/NSC/DOC(2006)1.
- Sanda T. ZPPR-18C experiment: a 1,000 MWe-class sodium-cooled MOX-fueled FBR homogeneous core mock-up critical experiment in the state of removal of one of eighteen half-inserted control rods, where enriched uranium is used with the shape of a sector in the outer core, International Handbook of Evaluated Reactor Physics Benchmark Experiments (IRPhE), ZPPR-LMFR-EXP-008; 2010. NEA/NSC/DOC(2006)1.
- Sanda T. ZPPR-19B experiment: a 1,000 MWe-class sodium-cooled MOX-fueled FBR core mock-up critical experiment with two-homogeneous zones and control-rod withdrawal, where plutonium and enriched uranium are used mixing in the outer core, International Handbook of Evaluated Reactor Physics Benchmark Experiments (IRPhE), ZPPR-LMFR-EXP-004; 2010. NEA/NSC/DOC(2006)1.
- Sugino K, Shono A, Iwai T, et al. Analyses on the BFS critical experiments –an analysis on the BFS-62-1 and 62-2 cores–. Japan Nuclear Cycle Development Institute; 2002. JNC TN9400 2002008. (in Japanese).
- Hazama T, Iwai T, Shono A. BFS critical experiment and analysis –an analysis on BFS-62-5 and 66-1 cores–. Japan Nuclear Cycle Development Institute; 2004. JNC TN9400 2005011. (in Japanese).
- Hazama T, Sato W. Evaluation of nuclear characteristics of minor actinide loaded core –analyses of BFS-69 and BFS-66-2 critical experiments–. Japan Atomic Energy Agency; 2010. JAEA. JAEAResearch 2010028. (in Japanese).
- Ishikawa M, Hazama T. Validation of Np-237 cross sections by analysis of BFS critical experiments with massively neptunium-loaded cores. In: International Conference on Nuclear Data for Science and Technology 2007; 2007. p. 817–820. DOI:10.1051/ndata:07210.
- Hazama T, Sato W, Ishikawa M, et al. Evaluation of nuclear characteristics of minor actinide loaded core –an analysis of BFS-67 critical experiment–. Japan Nuclear Cycle Development Institute; 2003. JNC TN9400 2003035. (in Japanese).
- Rowlands J. The ZEBRA MOZART programme. part 1. MZA and MZB ZEBRA assemblies 11 and 12, International Handbook of Evaluated Reactor Physics Benchmark Experiments (IRPhE), ZEBRA-LMFR-EXP-002; 2010. NEA/NSC/DOC(2006)1.
- Chiba G. Analysis of MOZART critical experiment using the IRPhEP handbook data. Japan Atomic Energy Agency; 2010. JAEA. JAEAResearch 2010042. (in Japanese).
- Hazama T, Kitano A, Kishimoto Y. Criticality evaluation for the monju restart core. Nucl Technol. 2012;179(2):250–265. DOI:10.13182/NT12-A14097.
- Kim KS, Wieselquist WA. Neutronic characteristics of ENDF/B-VIII.0 compared to ENDF/B-VII.1 for light-water reactor analysis. J Nucl Eng. 2021;2(4):318–335.
- Wright T, Guerrero C, Billowes J, et al. (The n_TOF Collaboration). Measurement of the 238U(n, γ) cross section up to 80 keV with the total absorption calorimeter at the CERN n_TOF facility. Phys Rev C. 2017 Dec;96:064601. DOI:10.1103/PhysRevC.96.064601.
- Slovacek RE, Cramer DS, Bean EB, et al. 238U(n, f) measurements below 100 keV. Nucl Sci Eng. 1977;62(3):455–462. DOI:10.13182/NSE77-A26984
- Mannhart W Response of activation reactions in the neutron field of californium-252 spontaneous fission. International Atomic Energy Agency; 2006. Technical Reports Series No. 452, p. 30–45. International Reactor Dosimetry File 2002 (IRDF2002).
- Iwamoto O. Development of a comprehensive code for nuclear data evaluation, CCONE, and validation using neutron-induced cross sections for uranium isotopes. J Nucl Sci Technol. 2007;44(5):687–697. DOI:10.1080/18811248.2007.9711857.
- Capote R, Trkov A, Sin M, et al. IAEA CIELO evaluation of neutron-induced reactions on 235U and 238U targets. Nucl Data Sheets. 2018;148:254–292. Special Issue on Nuclear Reaction Data. doi:10.1016/j.nds.2018.02.005.
- Iwamoto H, Iwamoto O, Kunieda S. G-HyND: a hybrid nuclear data estimator with Gaussian processes. J Nucl Sci Technol. 2022;59(3):334–344.
- Iwamoto H, Nishihara K, Sugawara T, et al. Sensitivity and uncertainty analysis for an accelerator-driven system with JENDL-4.0. J Nucl Sci Technol. 2013;50(8):856–862. DOI:10.1080/00223131.2013.811954
- Rovira G, Katabuchi T, Tosaka K, et al. Neutron capture cross-section measurement and resolved resonance analysis of 237Np. J Nucl Sci Technol. 2020;57(1):24–39. DOI:10.1080/00223131.2019.1651231
- Moxon MC, Brisland JGR. A least squares fitting program for resonance analysis of neutron transmission and capture data computer code; 2008. AEA-InTec-0630, AEA Technology.
- Schillebeeckx P, Becker B, Danon Y, et al. Determination of resonance parameters and their covariances from neutron induced reaction cross section data. Nucl Data Sheets. 2012;113(12):3054–3100. Special Issue on Nuclear Reaction Data.
- Butler JP, Lounsbury M, Merritt JS. The neutron capture cross sections of Pu238, Pu242, and Am243 in the thermal and epicadmium regions. Can J Phys. 1957;35(2):147–154.
- Chyzh A, Wu CY, Kwan E, et al. Precision measurement of the 238Pu(n, γ) cross section. Phys Rev C. 2013 Oct;88:044607. DOI:10.1103/PhysRevC.88.044607.
- Mughabghab SF. Atlas of neutron resonances volume 1: resonance properties and thermal cross sections Z =1 – 60. 6th ed. Amsterdam, Netherlands: Elsevier Science; 2018.
- Fukushima M, Tsujimoto K, Okajima S. Analyses with latest major nuclear data libraries of the fission rate ratios for several TRU nuclides in the FCA-IX experiments. J Nucl Sci Technol. 2017;54(7):795–805.
- Budtz-Jørgensen C, Knitter HH, Smith DL. Neutron induced fission cross section of 238Pu in the energy range from 5 eV to 10 MeV. In: Nuclear Data for Science and Technology. Proceedings of the International Conference, Antwerp, 6-10 Sep; 1983.
- Fursov BI, Polynov VN, Samylin BF, et al. Fast neutron induced fission cross sections of some minor actinides. In: International Conference on Nuclear Data for Science and Technology. Trieste, 19-24 May 1997. Italian Physical Society, conference proceedings volume 59 part I; 1997. p. 488–490.
- Ressler JJ, Burke JT, Escher JE, et al. Surrogate measurement of the 238Pu(n, f) cross section. Phys Rev C. 2011 May;83:054610. DOI:10.1103/PhysRevC.83.054610.
- Hughes RO, Beausang CW, Ross TJ, et al. 236Pu(n, f), 237Pu(n, f), and 238Pu(n, f) cross sections deduced from (p, t), (p, d), and (p, ṕ) surrogate reactions. Phys Rev C. 2014 Jul;90:014304. DOI:10.1103/PhysRevC.90.014304.
- De Saint Jean C (Co-ordinator). Co-ordinated evaluation of plutonium-239 in the resonance region; 2014. Nuclear Science NEA/WPEC-34, NEA/NSC/WPEC/DOC(2014)447.
- Gwin R, Spencer RR, Ingle RW, et al. Measurements of the average number of prompt neutrons emitted per fission of 239Pu and 235U. Oak Ridge National Laboratory; 1978. ORNL/TM-6246.
- Gwin R, Spencer RR, Ingle RW. Measurements of the energy dependence of prompt neutron emission from 233U, 235U, and 239Pu for En = 0.0005 to 10 MeV relative to emission from spontaneous fission of 252Cf. Nucl Sci Eng. 1986;94(4):365–379.
- Bouland O, Derrien H, Larson NM, et al. R-matrix analysis of the 240Pu neutron cross sections in the thermal to 5700-eV energy range. Nucl Sci Eng. 1997;127(2):105–129. DOI:10.13182/NSE127-105
- Eastwood TA, Baerg AP, Bigham CB, et al. Radiochemical methods applied to the determination of cross sections of reactor interest. In: Second Internat. At.En. Conf., Geneva, Vol.16; 1958.
- Stamatopoulos A, Tsinganis A, Colonna N, et al. (n_TOF Collaboration). Investigation of the 240Pu (n, f) reaction at the n_TOF/EAR2 facility in the 9 meV–6 MeV range. Phys Rev C. 2020 Jul;102:014616. DOI:10.1103/PhysRevC.102.014616.
- Lerendegui-Marco J, Guerrero C, Mendoza E, et al. (n_TOF Collaboration). Radiative neutron capture on 242Pu in the resonance region at the CERN n_TOF-EAR1 facility. Phys Rev C. 2018 Feb;97:024605. DOI:10.1103/PhysRevC.97.024605.
- Yokoyama K, Sugino K, Ishikawa M, et al. Development of the Unified Cross-section Set ADJ2017, JAEA, JAEAResearch 201811, 201811, March. 2017, Japan Atomic Energy Agency. (in Japanese).
- Tsujimoto K, Sasa T, Nishihara K, et al. Neutronics design for lead-bismuth cooled accelerator-driven system for transmutation of minor actinide. J Nucl Sci Technol. 2004;41(1):21–36.
- Terada K, Kimura A, Nakao T, et al. Measurements of neutron total and capture cross sections of 241Am with ANNRI at J-PARC. J Nucl Sci Technol. 2018;55(10):1198–1211. DOI:10.1080/00223131.2018.1485519
- Mendoza E, Cano-Ott D, Altstadt S, et al. Measurement and analysis of the 241Am neutron capture cross section at the n_TOF facility at CERN. Phys Rev C. 2018;97:054616. (n_TOF Collaboration) doi:10.1103/PhysRevC.97.054616.
- Rovira G, Kimura A, Nakamura S, et al. 241Am neutron capture cross section measurement and resonance analysis. In: Proceedings of the 2021 Symposium on Nuclear Data; November 18-19, 2021, J-PARC, Japan Atomic Energy Agency, Tokai, Japan;. 2022 . JAEA-Conf 2022-001: 91–96.
- Oizumi A, Jin T, Ishikawa M, et al. Physical mechanism analysis of burnup actinide composition in light water reactor MOX fuel and its application to uncertainty evaluation. Ann Nucl Energy. 2015;81:117–124. doi:10.1016/j.anucene.2015.03.033.
- Kimura A, Nakamura S, Terada K, et al. Measurements of the 243Am neutron capture and total cross sections with ANNRI at J-PARC. J Nucl Sci Technol. 2019;56(6):479–492. DOI:10.1080/00223131.2019.1598508
- Mendoza E, Cano-Ott D, Guerrero C, et al. Measurement and analysis of the 243Am neutron capture cross section at the n_TOF facility at CERN. Phys Rev C. 2014 Sep;90:034608. (n_TOF Collaboration). DOI:10.1103/PhysRevC.90.034608.
- Kodama Y, Katabuchi T, Rovira G, et al. Measurements of the neutron capture cross section of 243Am around 23.5 keV. J Nucl Sci Technol. 2021;58(11):1159–1164. DOI:10.1080/00223131.2021.1943557
- Knitter HH, Budtz-Jørgensen C. Neutron-induced fission cross section of 243Am in the energy range from 1 eV to 10 MeV. Nucl Sci Eng. 1988;99(1):1–12. DOI:10.13182/NSE88-A23540.
- Belloni F, Calviani M, Colonna N, et al. Measurement of the neutron-induced fission cross-section of 243Am relative to 235U from 0.5 to 20 MeV. Eur Phys J A. 2011;47:160. DOI:10.1140/epja/i2011-11160-x.
- Kessedjian G, Barreau G, Ache M, et al. 243Am neutron-induced fission cross section in the fast neutron energy range. Phys Rev C. 2012 Apr;85:044613. DOI:10.1103/PhysRevC.85.044613.
- Popescu L, Heyse J, Wagemans J, et al. Cross-section measurement of thermal neutron-induced fission on 243Cm. Nucl Sci Eng. 2012;171(3):204–209.
- Kawase S, Kimura A, Harada H, et al. Neutron capture cross sections of curium isotopes measured with ANNRI at J-PARC. J Nucl Sci Technol. 2021;58(7):764–786.
- Alekseev AA, Bergman AA, Berlev AI, et al. Cross section for the subbarrier fission of 244Cm. Phys at Nuclei. 2010;73:1487–1498. DOI:10.1134/S1063778810090036.
- Poenitz WP. Data interpretation, objective evaluation procedures and mathematical techniques for the evaluation of energy-dependent ratio, shape and cross section data. In: Proc. Conf. Nuclear Data Evaluation Methodsand Procedures, Upton, USA, Sep 22-25, 1980, BNL-NCS-51363, vol. I; 1981.
- Chiba S, Smith DL. A suggested procedure for resolving an anomaly in least-squares data analysis known as “peel’s pertinent puzzle” and the general implications for nuclear data evaluation; 1991. ANL/NDM-121.
- Fomushkin EF, Novoselov GF, Vinogradov YI, et al. Cross sections of neutron-induced fission of nuclei 244Cm and 246Cm in the near-threshold energy region. Yadernaya Fizika. 1980;31:39. In Russian.
- Zhuravlev K, Zamjatnin YS, Kroshkin N. Energy spectra and average prompt neutron number for fission of curium isotopes. In: 2nd Conf. on Neutron Physics, Kiev, Vol.4, 1973 p.57, USSR. USSR.
- Hirose K, 2021. private communication.
- Ethvignot T, Devlin M, Duarte H, et al. Neutron Multiplicity in the Fission of 238U and 235U with Neutrons up to 200 MeV. Phys. Rev. Lett. 2005;94:052701.
- Taieb J, Granier T, Ethvignot T, et al. Measurement of the average energy and multiplicity of prompt-fission neutrons from 238U(n,f) and 237Np(n,f) from 1 to 200 MeV. In: ND 2007 - International Conference on Nuclear Data for Science and Technology, Nice, France, April 22-27 2007; 2007. p. 429–432.
- Rapp MJ, Barry DP, Leinweber G, et al. Tantalum, Titanium, and Zirconium neutron total cross-section measurements from 0.4 to 25 MeV. Nucl Sci Eng. 2019;193(8):903–915. DOI:10.1080/00295639.2019.1570750
- Smith A, Guenther P, Moldauer P, et al. Fast-neutron total and scattering cross sections of elemental titanium. Nucl Phys A. 1978;307(2):224–238.
- Koning A, Delaroche J. Local and global nucleon optical models from 1 keV to 200 MeV. Nucl Phys A. 2003;713(3):231–310. DOI:10.1016/S0375-9474(02)01321-0.
- Iwamoto N. Evaluation of neutron capture cross sections and covariances on 99Tc and 129I in the keV energy region. EPJ Web of Conferences; 2015 Oct 5–8; Aix-en-Provence, France; 2016;111:03002.
- Khromyleva T, Bondarenko I, Gurbich A, et al. Investigation of (n, α) reaction cross sections for a number of structural material isotopes. Nucl Sci Eng. 2018;191(3):282–290. DOI:10.1080/00295639.2018.1463746
- Chadwick M, Herman M, Obložinský P, et al. ENDF/B-VII.1 nuclear data for science and technology: cross sections, covariances, fission product yields and decay data. Nucl Data Sheets. 2011;112(12):2887–2996. Special Issue on ENDF/B-VII.1 Library.
- Cierjacks S, Forti P, Kopsch D, et al. High resolution total neutron cross-sections between 0.5-30 MeV. Kernforschungszentrum Karlsruhe; 1969. Kernforschungszentrum Karlsruhe Reports, No.1000 (SUPP.2).
- Kunieda S, Chiba S, Shibata K, et al. Coupled-channels optical model analyses of nucleon-induced reactions for medium and heavy nuclei in the energy region from 1 keV to 200 MeV. J Nucl Sci Technol. 2007;44(6):838–852. DOI:10.1080/18811248.2007.9711321
- Lashuk AI, Sadokhin IP. Gamma-quanta production cross-sections at inelastic scattering of the neutrons on the nuclei of reactor construction materials. Vop at Nauki I Tekhn, Ser Yaderno-Reak Konstanty. 1994;(1):26.
- Minato F, Fukui T. Interference effect between neutron direct and resonance capture reactions for neutron-rich nuclei. EPJ Web Conf. 2017;163:00037. DOI:10.1051/epjconf/201716300037.
- Herman M, Trkov A, Capote R, et al. Evaluation of neutron reactions on iron isotopes for CIELO and ENDF/B-VIII.0. Nucl Data Sheets. 2018;148:214–253. Special Issue on Nuclear Reaction Data. doi:10.1016/j.nds.2018.02.004.
- Soda D, Matsuyama S, Ibaraki M, et al. Measurements of double-differential neutron emission cross sections for 18 and 11.5 MeV neutrons. In: Proceedings of the 1995 Symposium on Nuclear Data, November 16-17, JAERI, Tokai, Japan;. 1995. p. 146–151. JAERI-Conf 96-008.
- De Saussure G, Larson NM, Harvey JA, et al. Multilevel resonance analysis of 59Co neutron transmission measurements. Oak Ridge National Laboratory; 1991. ORNL/TM-11762.
- Iwamoto N. Evaluation of neutron nuclear data on Cobalt-59 for JENDL-5. In: Proceedings of the 2020 Symposium on Nuclear Data, November 26-27, 2020, RIKEN Nishina Center, RIKEN Wako Campas, Wako, Saitama, Japan;. 2022. p. 126–131. JAEA-Conf 2021-001.
- Anufriev VA, Babich SI, Kolesov AG, et al. Parameters of 60Co neutron levels. Soviet Atomic Energy. 1979;46(1):57. DOI:10.1007/BF01119956
- Hogg CH, Weber LD. Neutron activation cross sections for the Co-60 isomers. Idaho Nuclear Corp; 1966. Idaho Nuclear Corp. Reports, No.1024.
- Nakajima Y. JNDC WG on Activation Cross Section Data. JENDL activation cross section file. In: Proceedings of the 1990 Symposium on Nuclear Data; Tokai-mura, Ibaraki, Japan; 1991. p. 43–57. JAERI-M 91-032.
- Weigand M, Beinrucker C, Couture A, et al. 63Cu (n, γ) cross section measured via 25 keV activation and time of flight. Phys Rev C. 2017;95:015808.
- Kwon S, Ohta M, Sato S, et al. Benchmark experiment on copper with graphite by using DT neutrons at JAEA/FNS. Fusion Eng Des. 2017;124:1161–1164.
- Konno C, Ohta M, Kwon S, et al. JENDL-5 benchmark test for shielding applications. J Nucl Sci Technol. DOI:10.3327/jnst.45.634.
- Nakayama S. Calculation of neutron cross-sections on copper-63 and -65. J Nucl Sci Technol. 2018;55:614–622.
- El-Kadi S, Nelson C, Purser F, et al. Elastic and inelastic scattering of neutrons from 54,56Fe and 63,65Cu: (I). measurements from 8 to 14 MeV and a spherical optical model analysis. Nucl Phys A. 1982;(390):509–540. DOI:10.1016/0375-9474(82)90281-0.
- Schmidt D, Mannhart W. Determination of differential elastic and inelastic and double-differential neutron scattering cross sections of elemental copper at energies between 6.95 MeV and 14.18 MeV. Physikalisch-Technische Bundesanstalt; 2006. PTB-N-52.
- Kawabata M, Motoishi S, Ohta A, et al. Large scale production of 64Cu and 67Cu via the 64Zn(n, p)64Cu and 68Zn(n, np/d)67Cu reactions using accelerator neutrons. J Radioanal Nucl Chem. 2021;330:913–922.
- Mannhart W, Schmidt D. Measurement of neutron activation cross sections in the energy range from 8 MeV to 15 MeV. Physikalisch-Technische Bundesanstalt; 2007. PTB-N-53.
- Watanabe Y, Kosako K, Kunieda S, et al. Status of JENDL high energy file. J Korean Phys Soc. 2011;59:1040–1045.
- Shibata K. Evaluation of neutron nuclear data for gallium. J Nucl Sci Technol. 2013;50(3):277–286. DOI:10.1080/00223131.2013.772447.
- Ichihara A, Iwamoto O, Chiba S, et al. Program POD; a computer code to calculate cross sections for neutron-induced nuclear reactions. Japan Atomic Energy Agency. 2007. JAEA-Data/Code 2007-012.
- Katabuchi T, Toh Y, Mizumoto M, et al. Discovery of a new low energy neutron resonance of 89Y. Eur Phys J A. 2021;57:4.
- Tagliente G, Fujii K, Milazzo PM, et al. Neutron capture cross section of 90Zr: bottleneck in the s-process reaction flow. Phys Rev C. 2008;77:035802. (n_TOF Collaboration) doi:10.1103/PhysRevC.77.035802.
- Mughabghab SF. Atlas of neutron resonances: resonance parameters and thermal cross sections. Z=1-100. 5th ed. Amsterdam: Elsevier Science; 2006.
- Ichihara A. Evaluation of neutron resonance parameters for Zr isotopes. In: Proceedings of the 2016 Symposium on Nuclear Data, November 17-18, 2016, High Energy Accelerator Reseach Organization, Tsukuba, Ibaraki, Japan;. 2018. p. 103–108. JAEA-Conf 2017-001.
- Ichihara A. Theoretical calculation of neutron cross sections for 90,91,92,94,96Zr in the incident energy range between 200 keV and 20 MeV. J Nucl Sci Technol. 2018;55(9):1087–1098.
- Obložinský P, Cho YS, Mattoon CM, et al. Formalism for neutron cross-section covariances in the resonance region using kernel approximation. Brookhaven National Laboratory; 2010. BNL-91287-2010.
- Endo S, Kimura A, Nakamura S, et al. Neutron capture and total cross-section measurements and resonance parameter analysis of niobium-93 below 400 ev. J Nucl Sci Technol. 2022;59(3):318–333. DOI:10.1080/00223131.2021.1970040
- Krane KS. Neutron capture cross sections of 93Nb. Phys Rev C. 2019;100:034613.
- Ichihara A. Calculation of cross sections for metastable state production in the (n, γ), (n, n'), (n, 2n) and (n, 3n) reactions of 93Nb. J Nucl Sci Technol. 2016;53(12):2049–2055.
- Katabuchi T, Mizumoto M, Igashira M, et al. Measurement of the neutron capture cross section of 99Tc using ANNRI at J-PARC. EPJ Web Conf. 2017;146:11050.
- Mizuyama K, Iwamoto N, Iwamoto O, et al. Application of modified REFIT code for J-PARC/MLF to evaluation of neutron capture cross section on 155,157Gd. EPJ Web Conf. 2017;146:11042.
- Kino K, Furusaka M, Hiraga F, et al. Energy resolution of pulsed neutron beam provided by the ANNRI beamline at the J-PARC/MLF. Nucl Instrum Methods Phys Res A. 2014;736:66–74.
- Shibata K. Evaluation of neutron nuclear data on ruthenium isotopes. J Nucl Sci Technol. 2013;50(12):1177–1187. DOI:10.1080/00223131.2013.838912.
- Kunieda S, Furutachi N, Minato F, et al. JENDL/ImPACT-2018: a new nuclear data library for innovative studies on transmutation of long-lived fission products. J Nucl Sci Technol. 2019;56(12):1073–1091.
- Nakamura S, Kimura A, Kitatani F, et al. Cross section measurements of the radioactive 107Pd and stable 105,108Pd nuclei at J-PARC/MLF/ANNRI. Nucl Data Sheets. 2014;119:143–146.
- Nakamura S, Harada H, Raman S, et al. Thermal neutron capture cross section of palladium-107. J Nucl Sci Technol. 2007;44(2):103–108. DOI:10.1080/18811248.2007.9711262
- Terada K, Katabuchi T, Mizumoto M, et al. Measurement of neutron capture cross sections of Pd-107 at J-PARC/MLF/ANNRI. Prog Nucl Energy. 2015;82:118–121.
- Kimura A, Hirose K, Nakamura S, et al. Measurements of neutron capture cross sections of 112Sn and 118Sn with J-PARC/MLF/ANNRI. Nucl Data Sheets. 2014;119:150–153.
- Kimura A, Harada H, Nakamura S, et al. Neutron capture cross section measurements of 120Sn, 122Sn and 124Sn with the array of Ge spectrometer at the J-PARC/MLF/ANNRI. EPJ Web Conf. 2017;146:11031.
- Shibata K. Evaluation of neutron nuclear data on antimony isotopes. J Nucl Sci Technol. 2014;51(4):425–436.
- Shibata K. Evaluation of neutron nuclear data on tellurium isotopes. J Nucl Sci Technol. 2015;52(4):490–502. DOI:10.1080/00223131.2014.956157.
- Shibata K. Evaluation of neutron nuclear data on iodine isotopes. J Nucl Sci Technol. 2015;52(9):1174–1185. DOI:10.1080/00223131.2014.999729.
- Block RC, Burke JA, Barry DP, et al. Neutron transmission and capture measurements of 133Cs from 0.01 to 600 ev. Nucl Sci Eng. 2019;193(3):269–282. DOI:10.1080/00295639.2018.1520526
- Nakamura S, Kimura A, Hales BY, et al. Progress of neutron-capture cross section measurements promoted by ImPACT Project at ANNRI in MLF of J-PARC. In: Proceedings of the 2017 Symposium on Nuclear Data; November 16-17, 2017, iVil, Tokai-mura, Ibaraki, Japan;. 2018. p. 199–203. JAEA-Conf 2018-001.
- Nakamura S, Shibahara Y, Kimura A, et al. Measurements of thermal-neutron capture cross-section of Cesium-135 by applying mass spectrometry. J Nucl Sci Technol. 2020;57(4):388–400. DOI:10.1080/00223131.2019.1691077
- Filatenkov AA. Neutron activation cross sections measured at KRI in neutron energy region 13.4-14.9 MeV. International Atomic Energy Agency; 2016. INDC(CCP)0460 Rev.(CCP)0460 Rev.
- Perkin JL. Gamma ray spectra from fast neutron interactions. Nucl Phys. 1964;60(4):561–580.
- Kellie JD, Hall SJ, Crawford GI, et al. The neutron total cross-sections of some rare earth elements between 0.7 MeV and 9.0 MeV. J. Phys. A. 1974;7:1758.
- Nereson N, Darden S. Average neutron total cross sections in the 3- to 12-MeV region. Phys. Rev. 1954 Jun;94:1678–1682.
- Miller DW, Adair RK, Bockelman CK, et al. Total cross sections of heavy nuclei for fast neutrons. Phys. Rev. 1952 Oct;88:83–90.
- Islam E, Hussain M, Ameen N, et al. Total cross sections of Y, Zr, La, Ce, Pr, Gd, Ho, Ta and Hg for 1.0 to 2.0 MeV neutrons. Nucl Phys A. 1973;209(1):189–201. DOI:10.1016/0375-9474(73)90061-4
- Graham FJD, Glasgow DW. Neutron total cross sections, 2.5-15 MeV. I. Exper. Phys. Rev C. 1971 Feb;3:576–603.
- Bernard DL, Lenz G, Reber JD. Elastic scattering of fast neutrons by praseodymium and lanthanum. In: Nuclear Cross Sections and Technology, Proceedings of a Conference, Washington, D.C. 1968; Vol. 2; p. 755.
- Tanaka S, Tomita Y, Ideno K, et al. Fast-neutron scattering from 139La, 141Pr, Er and 209Bi. Nucl Phys A. 1972;179(2):513–523. DOI:10.1016/0375-9474(72)90387-9
- Minato F. Evaluation of neutron nuclear data of praseodymium-141 and -143. J Nucl Sci Technol. 2013;50(9):873–882. DOI:10.1080/00223131.2013.810125.
- Katabuchi T, Matsuhashi T, Terada K, et al. Misassigned neutron resonances of 142Nd and stellar neutron capture cross sections. Phys Rev C. 2015;91:037603.
- Kimura A, Nakamura S, Endo S, et al. Neutron total and capture cross-section measurements of 155Gd and 157Gd in thermal neutron energy region with the Li-glass detectors and the NaI(tl) spectrometer installed in J-PARC·MLF·ANNRI. J Nucl Sci Technol. 2022. DOI:10.1080/00223131.2022.2134224.
- Kang YR, Lee MW, Kim GN, et al. Neutron capture measurements and resonance parameters of gadolinium. Nucl Sci Eng. 2015;180(1):86–116. DOI:10.13182/NSE14-80
- Wigner EP, Eisenbud L. Higher angular momenta and long range interaction in resonance reactions. Phys. Rev. 1947 Jul;72:29–41. DOI:10.1103/PhysRev.72.29.
- Lane AM, Thomas RG. R-matrix theory of nuclear reactions. Rev Mod Phys. 1958 Apr;30:257–353. DOI:10.1103/RevModPhys.30.257.
- Evaluated nuclear structure data file; 2000. https://www.nndc.bnl.gov/ensdf/.
- Auchampaugh GF, Plattard S, Hill NW. Neutron total cross-section measurements of 9Be, 10,11B, and 12,13C from 1.0 to 14 MeV using the 9Be(d,n)10B reaction as a ‘white‘ neutron source. Nucl Sci Eng. 1979;69:30. DOI:10.13182/NSE79-A21282.
- Rapp M, Danon Y, Saglime J, et al. Beryllium and graphite neutron total cross-section measurements from 0.4 to 20 MeV. J Nucl Sci Eng. 2012;172:268.
- Cohn HO, Bair JK, Willard HB. Carbon-13 neutron total cross section. Phys. Rev. 1961;122:534. DOI:10.1103/PhysRev.122.534.
- Lane RO, Knox HD, Hoffmann-Pinther P, et al. States in 14C from σT and σel(θ) for 13C + n: measurement, R-matrix analysis,and model calculations. Phys Rev Part C Nucl Phys. 1981;23:1883. DOI:10.1103/PhysRevC.23.1883.
- Resler DA, Knox HD, Koehler PE, et al. Structure of 14C via elastic and inelastic neutron scattering from13C: measurement and R-matrix analysis. Phys Rev Part C Nucl Phys. 1989;39:766. DOI:10.1103/PhysRevC.39.766.
- Firestone RB, Revay Z. Thermal neutron radiative cross sections for 6,7 Li, 9Be, 10,11B,12,13C, and 1415N. Phys Rev C. 2016 May;93:054306.
- Zeitnitz B, Dubenkropp H, Putzki R, et al. Neutron scattering from 15N (i). R-matrix and phase-shift analyses. Nucl Phys Sect A. 1971;166:443. DOI:10.1016/0375-9474(71)90900-6.
- Sikkema CP. Energy levels in 16N from 15N(n,n)15N elastic scattering. Nucl Phys. 1962;32:470. DOI:10.1016/0029-5582(62)90351-6.
- Larson DC, Johnson CH, Harvey JA, et al. Measurement of the neutron total cross section of fluorine from 5 eV to 20 MeV. Oak Ridge National Laboratory; 1976. 5612. Technical memo. doi:10.2172/7331647.
- Broder DL, Gamaliy AF, Lashuk AI, et al. Gamma-production cross sections at inelastic neutron scattering by F, Co, Sb and Ta nuclei. Fiz.-Energ Institut; 1969. 155. Obninsk Reports.
- Elwyn AJ, Monahan JE, Lane RO, et al. Neutron scattering from nuclei near A = 20 at energies below 2.2 MeV. Nucl Phys. 1964;59:113. DOI:10.1016/0029-5582(64)90117-8.
- Gabbard F, Davis RH, Bonner TW. Study of the neutron reactions 6Li(n,α)3H, 19F(n, γ)20F, and 127I(n, γ)128I. Phys. Rev. 1959;114:201. DOI:10.1103/PhysRev.114.201.
- Egorov AI, Krutova RI, Loginov YE, et al. Measurement of thermal neutron radiative capture cross-sections of the 14N and 19F by in-beam γ-spectroscopy method with reactor neutrons. Nucl Instrum Methods Phys Res Sect A. 2005;545:296. DOI:10.1016/j.nima.2005.01.315.
- Hennig G. The slow neutron absorption cross section of graphite; 1957. Brookhaven National Laboratory report series No.489, p.19.
- Nagai Y, Igashira M, Takeda N, et al. Measurement of the neutron capture rate of the 12C(n, γ)13C reaction at stellar energy. Astrophys J. 1991;372:683.
- Ohsaki T, Nagai Y, Igashira M, et al. New measurement of the 12C(n, γ)13C reaction cross section. Astrophys J. 1994;422(2):912. DOI:10.1086/173783
- Reich CW, Moore MS. Multilevel formula for the fission process. Phys Rev. 1958 Aug;111:929–933. DOI:10.1103/PhysRev.111.929.
- Shima T, Okazaki F, Kikuchi T, et al. Measurement of the 13C(n, γ)14C cross section at stellar energies. Nucl Phys Sect A. 1997;621:231C. DOI:10.1016/S0375-9474(97)00243-1.
- Wallner A, Bichler M, Buczak K, et al. Accelerator mass spectrometry measurements of the 13C(n, γ)14C and 14N(n, p)14C cross sections. Phys Rev Part C Nucl Phys. 2016;93:045803. DOI:10.1103/PhysRevC.93.045803.
- Wright T, Bennett S, Heinitz S, et al. Measurement of the 13C(n, γ) thermal cross section via neutron irradiation and AMS. Eur Phys J A. 2019;55:200.
- Shima T, Kii T, Kikuchi T, et al. Experimental studies of keV energy neutron-induced reactions relevant to astrophysics and nuclear physics. Japan Atomic Energy Research Institute; 1997. JAERI-Conf 97-004.
- Wagemans J, Wagemans C, Goeminne G, et al. The 17O(n, α)14C reaction from subthermal up to approximately 350 keV neutron energy. Phys Rev C. 2002 Mar;65:034614.
- Schatz H, Käppeler F, Koehler PE, et al. 17O(n, α)14C: closure of a primordial CNO bi-cycle? Astrophys J. 1993 Aug;413:750–755.
- Wagemans J, Wagemans C, Bieber R, et al. Determination of the thermal neutron induced 17O(n, α)14C reaction cross section. Phys Rev C. 1998 Nov;58:2840–2843. DOI:10.1103/PhysRevC.58.2840
- Guardo GL, Spitaleri C, Lamia L, et al. Assessing the near threshold cross section of the 17O(n, α)14C reaction by means of the Trojan horse method. Phys Rev C. 2017 Feb;95:025807.
- Sikkema CP. Note on the scattering of fast neutrons by Ne-20. In: Low energy nuclear interactions and nuclear structure : proceedings of the International Conference on Nuclear Physics, Paris, 7-12 July 1958; 1958. p. 541.
- Cohn HO, Fowler JL. Differential elastic scattering of neutrons from neon. Phys. Rev. 1959 Apr;114:194–200.
- Heil M, Plag R, Uberseder E, et al. Stellar neutron capture cross sections of 20,21,22Ne. Phys Rev C. 2014 Oct;90:045804. DOI:10.1103/PhysRevC.90.045804.
- Bellmann D. Gamma rays from thermal neutron capture in nitrogen and natural neon. Atomkernenergie. 1971;17:145.
- Belgya T, Uberseder E, Petrich D, et al. Thermal neutron capture cross section of 22Ne. In: Capture Gamma‐Ray Spectroscopy and Related Topics: Proceedings of the 13th International Symposium on Capture Gamma‐Ray Spectroscopy and Related Topics; 2008 Aug 25–29; Cologne, Germany; 2009. p. 367–371. AIP-1090.
- Gledenov YM, Salatskiy VI, Sedyshev PV, et al. Measurements of the thermal neutron cross-sections of the O-17(n, α), Ar-36(n, α) and Ne-21(n, α) reactions. Joint Inst. for Nucl. Res.; 1997. Joint Inst. for Nucl. Res.
- Vaughn FJ, Imhof WL, Johnson RG, et al. Total Neutron Cross Sections of Helium, Neon, Argon, Krypton, and Xenon. Phys Rev. 1960 May;118:683–686.
- MacMullin S, Kidd M, Henning R, et al. Measurement of the elastic scattering cross section of neutrons from argon and neon. Phys Rev C. 2013 May;87:054613.
- Junghans A, Beyer R, Claußner J, et al. Neutron transmission measurements at nELBE. EPJ Web of Conferences; 2019 May 19–24; Beijing, China; 2020;239:01006.
- Rahn F, Camarda H, Hacken G, et al. Neutron resonance spectroscopy.Xiii. Na to 320 keV. Phys Rev C. 1973;8:1827.
- Rouki C, Archier P, Borcea C, et al. High resolution measurement of neutron inelastic scattering cross-sections for Na-23. J Nucl Instrum Methods Phys Res Sect A. 2012;672:82.
- Committee JND. Integral test of JENDL-3.3 based on shielding benchmarks shielding integral test working group (fy2006-2010); 2019. JAEA-Research 2018-017.
- Muckenthaler F, Spencer R, Hunter H, et al. Measurements for the Jasper program in-vessel fuel storage experiment. Oak Ridge National Laboratory; 1992. 11989. Oak Ridge National Lab. technical memo; doi:10.2172/432815.
- Muckenthaler F, Spencer R, Hunter H, et al. Measurements for the Jasper program intermediate heat exchanger experiment, Oak Ridge National Lab. technical memo. Oak Ridge, Tennessee 37831-6363: Oak Ridge National Laboratory; 1992, p 12064.
- Shibata K. Evaluation of neutron nuclear data for sodium-23. J Nucl Sci Technol. 2002;39(10):1065–1071.
- Furutachi N, Minato F, Iwamoto O. Statistical properties of thermal neutron capture cross sections calculated with randomly generated resonance parameters. Phys Rev C. 2019;100:014610.
- Durham RW, Girardi F. Effective neutron activation cross-sections of some chlorine and sulphur reactions in NRX. Nuovo Cimento. 1961;19:4–17.
- Koehler PE, Graff SM, O’Brien HA, et al. 36Cl(n,p)36S cross section from 25 meV to 800 keV and the nucleosynthesis of the rare isotope 36S. Phys Rev C. 1993 May;47:2107–2112. DOI:10.1103/PhysRevC.47.2107.
- De Smet L, Wagemans C, Goeminne G, et al. Experimental determination of the 36Cl(n, p)36S and 36Cl(n, α)33P reaction cross sections and the consequences on the origin of 36S. Phys Rev C. 2007 Mar;75:034617. DOI:10.1103/PhysRevC.75.034617.
- Wagemans C, Bieber R, Geltenbort P. Determination of the thermal neutron induced 36Cl(n, p)36S and 36Cl(n, α)33P reaction cross sections. Phys Rev C. 1996 Jul;54:389–392. DOI:10.1103/PhysRevC.54.389.
- Kunieda S, Iwamoto O, Iwamoto N, et al. Overview of JENDL-4.0/HE and benchmark calculations. In: Proceedings of the 2015 Symposium on Nuclear Data; Ibaraki Quantum Beam Research Center, Tokai-mura, Ibaraki, Japan; 2016. p. 41–46. JAEA-Conf 2016-004.
- Bieber R, Wagemans C, Goeminne G, et al. Thermal neutron induced (n,p) and (n, α) reactions on 37Ar. Nucl Phys A. 1999;647:3.
- Goeminne G, Wagemans C, Wagemans J, et al. Investigation of the 37Ar(n,p)37Cl and 37Ar(n,α)34S reactions in the neutron energy range from 10 meV to 100 keV. Nucl Phys A. 2000;678:11.
- Henshaw D. Atomic distribution in liquid argon by neutron diffraction and the cross sections of Ar-36 and Ar-40. Phys. Rev. 1957;105:976.
- Jiang S, Hemmick T, Kubik P, et al. Measurement of the 36Ar(n,p)36Cl cross section at thermal energies using the ams technique. Nucl Instrum Methods Phys Res Sect B. 1990;52:608.
- Davis E, Bonner T, Worley JD, et al. The disintegration of Ar-36 and Ar-40 by neutrons. Nucl Phys. 1964;55:643.
- Hanna G, Primeau D, Tunnicliffe P. Thermal neutron cross sections and resonance integrals of the reactions O-17(n,α)C-14, Ar-36(n, α)S-33, and N-14(n,p)C-14. Can J Phys. 1961;39:1784. DOI:10.1139/p61-201
- Benisz J, Jasielska A, Panek T. Q-values and cross-section measurement of the (n, α) reaction of some nuclei. Acta Physica Polonica. 1965;28:763.
- Firestone RB, Krtička M, Révay Z, et al. Thermal neutron capture cross sections of the potassium isotopes. Phys Rev C. 2013 Feb;87:024605. DOI:10.1103/PhysRevC.87.024605.
- Foland KA, Borg RJ, Mustafa MG. The production of 38Ar and 39Ar by 14-MeV neutrons on 39K. Nucl Sci Eng. 1987;95(2):128–134. DOI:10.13182/NSE87-A20423.
- Shibata K. Evaluation of neutron nuclear data for erbium. J Nucl Sci Technol. 2012;49(8):824–835. DOI:10.1080/00223131.2012.704159.
- Masyanov SM, Anufriev VA, Korostylev VA. Resonance parameters of 165Ho and 166mHo. Atomic Energy. 1992 Aug;73:673–675.
- Ebran A, Roig O, Méot V, et al. Cross section for the neutron radiative capture on 173Lu nuclei. Phys Rev C. 2019 Jun;99:064603.
- Roig O, Jandel M, Méot V, et al. Radiative neutron capture cross sections on 176Lu at DANCE. Phys Rev C. 2016 Mar;93:034602.
- Roig O, Méot V, Rossé B, et al. Direct evidence for inelastic neutron “acceleration” by 177Lum. Phys Rev C. 2011 Jun;83:064617.
- Gilbert A, Cameron AGW. A composite nuclear-level density formula with shell corrections. Can J Phys. 1965;43(8):1446–1496.
- Mengoni A, Nakajima Y. Fermi-gas model parametrization of nuclear level density. J Nucl Sci Technol. 1994;31(2):151–162.
- Frehaut J, Bertin A, Bois R, et al. Status of (n, 2n) cross section measurements at Bruyeres-le-Chatel. In: Symposium on Neutron Cross Sections from 10-50 MeV, held at Brookhaven National Laboratory, Upton, New York 11973, May 12-14, 1980; Vol. 1; 1980. p. 399–411. S,INDC(USA)-84.
- Vonach H, Pavlik A, Strohmaier B. Accurate determination of (n, 2n) cross sections for heavy nuclei from neutron production spectra. Nucl Sci Eng. 1990;106(4):409–414.
- Endo S, Kimura A, Nakamura S, et al. Measurements of the neutron total and capture cross-sections and derivation of resonance parameters of 181Ta. to be submitted.
- Nakamura S, Shibahara Y, Endo S, et al. Thermal-neutron capture cross-section measurement of Tantalum-181 using graphite thermal column at KUR. J Nucl Sci Technol. 2021;58(10):1061–1070. DOI:10.1080/00223131.2021.1908187
- Shibata K. Evaluation of neutron nuclear data on tantalum isotopes. J Nucl Sci Technol. 2016;53(7):957–967. DOI:10.1080/00223131.2015.1083492.
- Guenther PT, Smith AB, Whalen JF. Fast-neutron total and scattering cross sections of 182W, 184W, and 186W. Phys Rev C. 1982;26:2433–2446.
- Lister D, Smith AB, Dunford C. Fast-neutron scattering from the 182, 184, and 186 isotopes of tungsten. Phys. Rev. 1967;162:1077–1087.
- Chadwick MB, Frankle S, Trellue H, et al. Evaluated iridium, yttrium, and thulium cross sections and integral validation against critical assembly and bethe sphere measurements. Nucl Data Sheets. 2007;108(12):2716–2741. DOI:10.1016/j.nds.2007.11.005
- Iwamoto N. Evaluation of neutron induced reaction cross sections on re isotopes. In: Proceedings of the 2012 Symposium on Nuclear Data; November 15-16, 2012, Research Reactor Institute, Kyoto University, Kumatori, Japan;. 2013. p. 143–148. JAEA-Conf 2013-002.
- Shibata K. Evaluation of neutron nuclear data on platinum isotopes. J Nucl Sci Technol. 2017;54(2):147–157. DOI:10.1080/00223131.2016.1223561.
- Shibata K. Evaluation of neutron nuclear data on mercury isotopes. J Nucl Sci Technol. 2016;53(10):1595–1607. DOI:10.1080/00223131.2016.1140599.
- Shibata K. Evaluation of neutron nuclear data on thallium isotopes; 2017. ( unpublished).
- Schillebeeckx P, Belgya T, Borella A, et al. Neutron capture studies of 206Pb at a cold neutron beam. Eur Phys J A. 2013;49:143.
- Negret A, Mihailescu LC, Borcea C, et al. Cross section measurements for neutron inelastic scattering and the (n, 2nγ) reaction on 206Pb. Phys Rev C. 2015;91:064618.
- Mihailescu LC, Borcea C, Baumann P, et al. A measurement of (n, xnγ) cross sections for 208Pb from threshold up to 20 MeV. Nucl Phys A. 2008;811(1):1–27. DOI:10.1016/j.nuclphysa.2008.07.016
- Iijima S, Nakagawa T, Kikuchi Y, et al. Evaluation of neutron cross section of 27 fission product nuclides important for fast reactor. J Nucl Sci Technol. 1977;14(3):161–176. DOI:10.1080/18811248.1977.9730743
- Iwamoto O. Calculation of recoil nucleus spectrum in the presence of multi-particle emission in nuclear reaction with monte carlo method as an extension of CCONE code. J Nucl Sci Technol. 2022;59(10):1232–1241. DOI:10.1080/00223131.2022.2038300.
- Iwamoto O, Iwamoto N, Kunieda S, et al. The CCONE code system and its application to nuclear data evaluation for fission and other reactions. Nucl Data Sheets. 2016;131:259–288.
- Koppel JU, Houston DH. Reference manual for ENDF thermal neutron scattering data. San Diego (USA): Gulf General Atomic; 1968. GA-8774.
- MacFarlane RE. New thermal neutron scattering files for ENDF/B-VI release 2. Los Alamos (USA): Los Alamos National Laboratory; 1994. LA-12639-MS.
- Chadwick MB, Obložinský P, Herman M, et al. ENDF/B-VII.0: next generation evaluated nuclear data library for nuclear science and technology. Nucl Data Sheets. 2006 Dec;107:2931–3060.
- Abraham MJ, Murtola T, Schulz R, et al. GROMACS: high performance molecular simulations through multi-level parallelism from laptops to supercomputers. SoftwareX. 2015 Sep;1-2:19–25.
- González MA, Abascal JLF. A flexible model for water based on TIP4P/2005. J Chem Phys. 2011 Dec;135:224516.
- Abe Y, Funama F, Tasaki S, et al. Development of a general-purpose code for analyzing thermal neutron scattering cross-sections of hydrogenous molecules by molecular dynamics. To be submitted.
- Heinloth K. Streuung subthermischer Neutronen an H2O, CH2O2 und C6H6. Zeitschrift Für Phys. 1961 Apr;163:218–229.
- Russell JL Jr., Neill JM, Brown JR. Total Cross Section Measurements of H2O. San Diego (USA): General Atomic; 1966, GA-7581.
- Ichihara A. Survey of computational methods of cross sections for thermal neutron scattering by liquids. Tokai-mura (Japan): Japan Atomic Energy Agency; 2020, JAEA-Review 2019-046.
- Sköld K. Small energy transfer scattering of cold neutrons from liquid argon. Phys Rev Lett. 1967 Oct;19:1023–1025.
- EXFOR [Internet]. International Atomic Energy Agency; 2022 Mar 7 [cited. 2022 Mar 10]. Available from: http://www-nds.iaea.org/EXFOR/30283.002/.
- Damián JIM, Malaspina DC, Granada JR. Vibrational spectra of light and heavy water with application to neutron cross section calculations. J Chem Phys. 2013 Jul;139:024504.
- Damián JIM, Granada JR, Malaspina DC. CAB models for water: a new evaluation of the thermal neutron scattering laws for light and heavy water in ENDF-6 format. Ann Nucl Energy. 2014 Mar;65:280–289.
- Klauda JB, Venable RM, Freites JA, et al. Update of the CHARMM All-Atom Additive Force Field for Lipids: validation on Six Lipid Types. J Phys Chem B. 2010 Jun;114:7830–7843.
- Kim S, Lee J, Jo S, et al. CHARMM-GUI ligand reader and modeler for CHARMM force field generation of small molecules. J Comput Chem. 2017 Aug;38:1879–1886.
- Whittemore WL. Inelastic neutron scattering in liquid methane and liquid parahydroge. Nucl Sci Eng. 1964 Feb;18:182–188.
- Rodrigues C, Vinhas LA, Herdade SB, et al. Slow-neutron scattering cross-section for methanol, ethanol, propanol, iso-propanol, butanol, ethanediol and propanetriol. J Nucl Energy. 1972 Jul;26:379–383.
- Dawidowski J, Granada JR, Blostein JJ. Neutron total cross-sections of normal and deuterated ethanol in the thermal and epithermal energy ranges. Nucl Instrum Methods B. 2000 Aug;168:462–466.
- Stepanov SB, Zhitarev VE, Zelenyuk FM, et al. Temperature dependence of total cold neutron cross section in light water and benzene. Soviet Atomic Energy. 1974 Oct;37:1094–1096.
- Lolich JV, Abbate M. Total cross section and diffusion parameters of benzene. Atomkernenergie Kerntechnik. 1980;35:23–24.
- Torres L, Granada JR, Blostein JJ. Total cross sections of benzene at 90 K and light water ice at 115 K. Nucl Instrum Methods B. 2006 Sep;251:304–305.
- Palomino LAR, Cantargi F, Blostein JJ, et al. Total cross section of solid mesitylene, toluene and a mixture of them at thermal neutron energies. Nucl Instrum Methods B. 2009 Jan;267:175–177.
- Cantargi F, Blostein JJ, Torres L, et al. Thermal neutron cross section of liquid and solid mesitylene. Nucl Instrum Methods B. 2006 Aug;248:340–342.
- Cantargi F, Dawidowski J, Helman C, et al. Validated scattering kernels for triphenylmethane at cryogenic temperatures. Proceeding of ND 2019: International Conference on Nuclear Data for Science and Technology; 2019 May 19–24; Beijing, China: EPJ Web of Conferences; 2020;239:14002.
- Katakura J. JENDL FP decay data file 2011 and fission yields data file. 2011, JAEA-Data/Code: 2011-025.
- England TR, Rider BF. Evaluation and compilation of fission product yields. Los Alamos, New Mexico, 87545: Los Alamos National Laboratory, 1994. ENDF-349, LA-UR-94-3106.
- Nakahara Y, Suyama K, Inagawa J, et al. Nuclide composition benchmark data set for verifying burnup codes on spent light water reactor fuels. Nucl Technol. 2002;137(2):111–126. DOI:10.13182/NT02-2
- Department of Chemistry JAEA Tokai Research Establishment. Dissolution study group in the department of chemistry, dissolution studies of spent nuclear fuels. Japan Atomic Energy Agency; 1991.
- Nakahara Y, Suyama K, Suzaki T. Technical development on burn-up credit for spent LWR fuels. Japan Atomic Energy Agency; 2000.
- Katakura J, Minato F. JENDL Decay Data File 2015, JAEA-Data/Code: 2015-030.
- Original data are from ENSDF (DATE: 04-Oct-2019), Version available at http://www.nndc.bnl.gov/ensarchivals/.
- Dimitriou P, Dillmann I, Singh B et al. Development of a reference database for beta-delayed neutron emission. Nucl Data Sheets. 2021:144–238. DOI:10.1016/j.nds.2021.04.006.
- Algora A, Tain JL, Rubio B et al. Beta-decay studies for applied and basic nuclear physics. Eur Phys J A. 2021;57:1–28.
- Fijalkowska A, Karny M, Rykaczewski KP et al. Impact of Modular Total Absorption Spectrometer measurements of β decay of fission products on the decay heat and reactor ν¯e flux calculation. Phys Rev Lett. 2017;119:052503
- Jordan D, Algora A, Taín JL, et al. Total absorption study of the β decay of 102,104,105Tc. Phys Rev C. 2013;87:044318
- Greenwood RC, Helmer RG, Putnam MH et al. Measurement of β–decay intensity distributions of several fission-product isotopes using a total absorption γ-ray spectrometer. Nucl Inst Meth A. 1997;390:95–154.
- Burrows TW. The program RADLST, Brookhaven National Laboratory Report BNL-NSC-52142, 1988.
- Tasaka K, Katakura J, Ihara H, et al. JNDC Nuclear Data Library of Fission Products -Second Version-, JAERI 1320; 1990, 266p.
- Wu J, Nishimura S, Möller P, et al. β-decay half-lives of 55 neutron-rich isotopes beyond the n = 82 shell gap. Phys Rev C. 2020 Apr;101:042801. DOI:10.1103/PhysRevC.101.042801
- Minato F, Niu ZM, Haozhao L. Calculation of β-decay half-lives within a Skyrme-Hartree-Fock-Bogoliubov energy density functional with the proton-neutron quasiparticle random-phase approximation and isoscalar pairing strengths optimized by a Bayesian method. Phys Rev C. 2022;106:024306/1–13. DOI:10.1103/PhysRevC.106.024306.
- Cullen DE. Program RELAX CD: A code designed to calculate atomic relaxation spectra of x-rays and electrons, Lawrence Livermore National Lab., UCRL-ID-110438, 1992.
- Table of isotopes. 8th ed. 1996 . Firestone RB, Shirley VS, editors. New York, NY 10158: John Wiley & Sons, INC.
- Akiyama M, Furuta K, Ida T, et al. Measurements of Beta-Ray Decay Heat of Fission Products for Fast Neutron Fissions of 235U, 239Pu and 233U. J Atom Ener Soc Japan. 1982;24:803–816. in Japanese DOI:10.3327/jaesj.24.803.
- Akiyama M, Furuta K, Ida T, et al. Measurements of Gamma-Ray Decay Heat of Fission Products for Fast Neutron Fissions of 235U, 239Pu and 233U. J Atom Ener Soc Japan. 1982;24:709–722. in Japanese DOI:10.3327/jaesj.24.709.
- Keepin GR, Wimett TF, Zeigler RK. Delayed neutrons from fissionable isotopes of uranium. Plutonium Thorium Phys. Rev. 1957;107:1044–1049.
- Matsumura T, Fujita M. 2021. private communication.
- Nishihara K, Iwamoto H, Suyama K. Estimation of fuel compositions in Fukushima-Daiichi Nuclear Power Plant. 2012; JAEA-Data/Code 2012-018.
- Wakabayashi Y, Taketani A, Hashiguchi T, et al. A function to provide neutron spectrum produced from the 9Be + p reaction with protons of energy below 12 MeV. J Nucl Sci Technol. 2018;55(8):859–867.
- Conlin JL, Romano P. A compact ENDF (ACE) format specification; 2019. Available from: https://www.osti.gov/biblio/1561065.
- MacFarlane RE, Kahler AC. Methods for processing ENDF/B-VII with NJOY. Nucl Data Sheets. 2010;111(12):2739–2890.
- Werner CJ, Bull JS, Solomon CJ, et al. MCNP version 6.2 release notes; 2018. Available from: https://www.osti.gov/biblio/1419730.
- Sato T, Iwamoto Y, Hashimoto S, et al. Features of particle and heavy ion transport code system (PHITS) version 3.02. J Nucl Sci Technol. 2018;55(6):684–690. DOI:10.1080/00223131.2017.1419890
- Howard WB, Grimes SM, Massey TN, et al. Measurement of the thick-target 9Be(p,n) neutron energy spectra. Nucl Sci Eng. 2001;138:145. DOI:10.13182/NSE01-A2206.
- Bair JK, Jones CM, Willard HB. Neutrons from the proton bombardment of 6Li, 7Li, 9Be, 11B and 18O. Nucl Phys. 1964;53:209. DOI:10.1016/0029-5582(64)90598-X.
- Kunieda S, Yamamoto K, Konno C, et al. Estimation of double-differential cross-sections of 9Be(p,xn) reaction for new nuclear data library, JENDL-5. J Neut Res. 2022;24;329-335. DOI:10.3233/JNR-220019.
- Iwamoto H. Generation of nuclear data using Gaussian process regression. J Nucl Sci Technol. 2020;57(8):932–938.
- Koning A, Rochman D, Sublet JC, et al. TENDL: complete nuclear data library for innovative nuclear science and technology. Nucl Data Sheets. 2019;155:1–55.
- Neuzil EF, Lindsay RH. Emission of 7Be and competition processes at 30 to 42 MeV. Phys Rev. 1963;131(4):1697.
- Lafleur MS, Porile N, Yaffe L. Formation of 7Be in nuclear reactions induced by 85-MeV protons. Can J Chem. 1966;44(22):2749–2767.
- Miyano K. The 7Be, 22Na and 24Na production cross sections with 22-to 52-MeV proton on 27Al. J Phys Soc Jpn. 1973;34(4):853–856.
- Schölten B, Qaim S, Stöcklin G. Radiochemical studies of proton induced 7Be-emission reactions in the energy range of 40 to 100 MeV. Radiochimca Acta. 1994;65(2):81–86.
- Michel R, Bodemann R, Busemann H, et al. Cross sections for the production of residual nuclides by low-and medium-energy protons from the target elements C, N, O, Mg, Al, Si, Ca, Ti, V, Mn, Fe, Co, Ni, Cu, Sr, Y, Zr, Nb, Ba and Au. Nucl Instrum Methods Phys Res A. 1997;129(2):153–193. DOI:10.1016/S0168-583X(97)00213-9
- Titarenko YE, Borovlev S, Butko M, et al. Cross sections for monitor reactions 27Al(p,x)24Na, 27Al(p,x)22Na, and 27Al(p,x)7Be at proton energies in the range 0.04–2.6 GeV. Phys at Nuclei. 2011;74(4):507–522. DOI:10.1134/S1063778811040156
- Szelecsényi F, Steyn GF, Nortier FM, et al. New cross sections for the 27Al(p,x)7Be nuclear process: monitoring proton beam energy via the 22Na/7Be cross-section ratio between 45 and 200 MeV. In: EPJ Web of Conferences; 2016 Sep 11–16; Bruges, Belgium; Vol. 146; EDP Sciences; 2017. p. 08011.
- Nakayama S, Iwamoto O, Watanabe Y, et al. JENDL/DEU-2020: deuteron nuclear data library for design studies of accelerator-based neutron sources. J Nucl Sci Technol. 2021;58:805–821.
- Nakayama S, Kouno H, Watanabe Y, et al. Theoretical model analysis of (d, xn) reactions on 9Be and 12C at incident energies up to 50 MeV. Phys Rev C. 2016;94:014618.
- Moeslang A, Heinzel V, Matsui H, et al. The IFMIF test facilities design. Fusion Eng Des. 2006;81:863–871.
- Nagai Y, Hashimoto K, Hatsukawa Y, et al. Generation of radioisotopes with accelerator neutrons by deuterons. J Phys Soc Jpn. 2013;82:064201.
- Nishitani T, Yoshihashi S, Kumagai K, et al. Benchmark calculation of d-Li thick target neutron yield by JENDL/DEU-2020 for IFMIF and similar facilities. Plasma Fusion Res. 2021;16:1405104.
- Kalbach C. The Griffin model, complex particles and direct nuclear reactions. Z Phys. A. 1977;283:401–411.
- Basunia MS. Nuclear data sheets for A = 28. Nucl Data Sheets. 2013;114:1189–1291.
- Singh B. Nuclear data sheets for A = 64. Nucl Data Sheets. 2007;108:197–364.
- Browne E, Tuli J. Nuclear data sheets for A = 66. Nucl Data Sheets. 2010;111:1093–1209.
- Abriola D, Sonzogni A. Nuclear data sheets for A = 94. Nucl Data Sheets. 2006;107:2423–2578.
- Nakayama S, Watanabe Y. Systematic investigation of spectroscopic factors from (d,p) reactions for deuteron nuclear data evaluation. J Nucl Sci Technol. 2016;53:89–101.
- Boudard A, Cugnon J, David JC, et al. New potentialities of the Liège intranuclear cascade model for reactions induced by nucleons and light charged particles. Phys Rev C. 2013;87:014606.
- Furihata S. Statistical analysis of light fragment production from medium energy proton-induced reactions. Nucl Instrum Methods Phys Res Sec B. 2000;171:251–258.
- Shigyo N, Hidaka K, Hirabayashi K, et al. Measurement of deuteron induced thick target neutron yields at 9 MeV. J Korean Phys Soc. 2011;59:1725–1728.
- Kalbach C. Preequilibrium reactions with complex particle channels. Phys Rev C. 2005;71:034606.
- Smith MS, Brown DA, Romano CE, et al. (α, n) nuclear data scoping study. Oak Ridge National Laboratory; 2020. ORNL/TM-2020/1789.
- Geiger K, Van Der Zwan L. An evaluation of the 9Be(alpha,n) cross section; 1976. NRCC-15303.
- Kalbach C, Mann FM. Phenomenology of continuum angular distributions. I. systematics and parametrization. Phys Rev C. 1981;23:112–123.
- Griesheimer DP, Pavlou AT, Thompson JT, et al. In-line (α, n) source sampling methodology for monte carlo radiation transport simulations. Nucl Eng Technol. 2017;49:1199–1210.
- Jacobs G, Liskien H. Energy spectra of neutrons produced by α-particles in thick targets of light elements. Ann Nucl Energy. 1983;10:541–552.
- Kawada H, Sublet JC, Okumura S, et al. Processing of the evaluated photonuclear data library (IAEA/PD-2019). International Atomic Energy Agency; 2020. IAEA-NDS-0232.
- Gheorghe I, Utsunomiya H, Katayama S, et al. Photoneutron cross-section measurements in the 209Bi(γ, xn) reaction with a new method of direct neutron-multiplicity sorting. Phys Rev C. 2017 Oct;96:044604. DOI:10.1103/PhysRevC.96.044604.
- Harvey RR, Caldwell JT, Bramblett RL, et al. Photoneutron cross sections of Pb206, Pb207, Pb208, and Bi209. Phys Rev. 1964 Oct;136:B126–131. DOI:10.1103/PhysRev.136.B126
- Berman BL, Pywell RE, Dietrich SS, et al. Absolute photoneutron cross sections for Zr, I, Pr, Au, and Pb. Phys Rev C. 1987 Oct;36:1286–1292. DOI:10.1103/PhysRevC.36.1286.