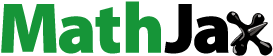
ABSTRACT
Chromium is one of the important structural materials in the process of nuclear reaction, and the precise nuclear data of n + 52Cr reaction is very important. On the basis of the spherical optical model, the unified Hauser–Feshbach theory and exciton model, all reaction cross sections, angular distributions, energy spectra, and double-differential cross sections were calculated and analysed for n + 52Cr reactions below 20 MeV. The theoretical calculations were compared with the available experimental data and other evaluation data from ENDF/B-VIII.0 and JENDL-5.
1. Introduction
The development of nuclear energy is important to solve the global energy crisis and environmental problems. Safety is the prerequisite to ensure the use of nuclear energy. Chromium is one of the most important structural materials during the nuclear reaction process. The study shows that Cr has the characteristics of thermostability, good corrosion resistance and abrasion resistance, and suitable for engineering application. It is used as a corrosive coating of zirconium alloy in the reactor [Citation1]. The study of coating on the surface of zirconium alloy cladding pipe becomes a vital goal in the development of accident resistant fuel (ATF) cladding. Chromium is currently the most researched material to improve accident resistant coating on zirconium alloy surfaces [Citation2], and it is also considered to be the most promisingly commercialized [Citation3–6]. Thus, the development of high-quality nuclear data for chromium is particularly important. Natural Cr consists of four isotopes, and they are 50Cr (4.345%), 52Cr (83.789%), 53Cr (9.501%), and 54Cr (2.365%). In this study, we obtained a set of optical model potential parameters for the n + natCr reaction below 20 MeV. We systematically calculated various cross sections, angular distributions, energy spectra, double-differential cross sections and γ-ray production cross sections for the reaction of the n+ 52Cr reactions. The calculated results were compared with the existing experimental data from EXFOR Library [Citation7], together with the evaluated data from the ENDF/B-VIII.0 [Citation8] and JENDL-5 [Citation9].
2. Theoretical models and model parameters
2.1 Optical model and the optical potential parameters
The optical model is one of the most important theories in nuclear data calculations and evaluations, which describes the nuclear reaction process using the optical model potential. The optical potentials considered here are Woods-Saxon [Citation10] form for the real part; Woods-Saxon and derivative Woods-Saxon form for the imaginary parts corresponding to the volume and surface absorption, respectively; and the Thomas form for the spin-orbit part.
The potential depths for the real part, the imaginary part of surface absorption, and the volume absorption are dependent on incident energies and are expressed as follows:
where V is the real part potential, WS and WV are the imaginary part potential of surface absorption and volume absorption. Where V0, WS0, WV0 are Constant factors, V1, WS1 and WV1 are energy-linear term factors, V2 and WV2 are energy-square term factors, V3 is charge-symmetry term factors in real potentials, WS2 is charge-symmetry term factors of surface absorption imaginary potentials. Where rr, rS, rV, rSO, and rC are the radius of the real part, the surface absorption, the volume absorption, the spin–orbit couple and the Coulomb potential, respectively.The spin–orbit couple potential is VSO, and the diffusive width of the real part, the surface absorption, the volume absorption and the spin–orbit couple potential are ar, as, av, and aso; Where Z, N and A are charge, neutron, and mass numbers of target, respectively, E is incident neutron energy in the center of mass system. The units of the potential V, WS, WV, and VSO are in MeV, the lengths rr, rS, rV, rSO, rC, ar, as, av, and aso are in fm, and the energy E is in MeV.
A new set of neutron optical potential parameters for natCr were obtained to fit the experimental data of total cross sections, nonelastic scattering cross sections, elastic scattering cross sections and elastic scattering angular distributions by the code APMN [Citation11]. The experimental data of n+ natCr were used in the fitting, because the measured data of n+ 52Cr are scarce. The optical model potential parameter set obtained for n+ natCr is given in . The global OMP parameters for proton, deuteron, 3He, triton, and alpha-particle projectiles [Citation12–16] were employed in the calculations.
Table 1. The optical model potential parameters.
2.2 Direct inelastic scattering for discrete level
The direct inelastic scattering cross sections and angular distributions to low-lying states are important in nuclear data theoretical calculations. The code DWUCK [Citation17] of the distorted wave Born approximation (DWBA) theory was used to pre-calculate the direct reaction cross sections and angular distributions in n+ 52Cr. The optical model potential parameters obtained in this work were included as input into the DWUCK code. Based on experimental data of the direct inelastic scattering cross sections and angular distributions of discrete levels, we adjusted the deformation parameters in DWUCK. The contribution to the direct inelastic scattering comes mainly from eight discrete levels, the deformation parameters of which are listed in .
Table 2. The excited energies (ε), spins (J), parities (π), and deformation parameters (β) of eight discrete levels which make the main contribution to the direct inelastic scattering.
2.3 The pre-equilibrium and equilibrium processes
The unified Hauser–Feshbach and exciton model [Citation18] is used to describe the equilibrium and pre-equilibrium emission processes for the incident energy below 20 MeV. The UNF [Citation19] code of the spherical optical model, the unified Hauser–Feshbach, and exciton model were developed for calculating fast neutron reaction data of structure materials with incident energies from about 1 keV up to 20 MeV. The angular momentum dependent exciton model was established to describe the emissions from compound nucleus to the discrete levels of the residual nuclei in pre-equilibrium processes, while the equilibrium processes were described by the Hauser–Feshbach model with width fluctuation correction. The emissions to the discrete level in the multi-particle emissions for all opened channels were included [Citation18,Citation19]. The double-differential cross sections of neutron and proton were calculated by the linear momentum dependent exciton state density [Citation20]. Since the improved pickup mechanism has been employed based on the Iwamoto-Harada model [Citation21–23], the double-differential cross sections of alpha-particle,3He, deuteron, and triton can be calculated by using a new method based on the Fermi gas model. The recoil effects in multi-particle emissions from continuum state to discrete level as well as from continuum to continuum state were taken into account strictly, so the energy balance was held accurately in every reaction channels [Citation19]. If the calculated direct inelastic scattering data and the calculated direct reaction data of the outgoing charged particles are available from other codes, one can input them, so that the calculated results will included the effects of the direct reaction processes. In this work, the calculated results of DUWCK and APMN were included as input into the UNF. To keep the energy balance, the recoil effects were taken into account for all of the reaction processes. The gamma-production data were also calculated, and the gamma-ray E1 strength function [Citation24,Citation25] is expressed as follows:
where σi, Γi, Ei are photon absorption cross section, resonance width, and resonance energy of electric dipole resonance. KE1 = 8.68 × 10−8(mb)−1(MeV)−2.
The data of discrete level, and the parameters of giant dipole resonance, were taken from Reference Input Parameter Library for Calculation of Nuclear Reactions and Nuclear Data Evaluations (RIPL) [Citation26], the initial parameters of the level densities and pair corrections were taken from RIPL, and were adjusted in order to fit the experimental data of reaction cross sections. The final parameters values are listed in . The calculated neutron reaction data can be output in the ENDF-6 format.
Table 3. The level density and pair correction parameters.
3. Theoretical results and analysis
The calculated results of the total and elastic scattering cross sections for n+52Cr reaction were compared with experimental data [Citation27–37] and evaluated data from ENDF/B-VIII.0 [Citation8] and JENDL-5 [Citation9] in .
Figure 1. Calculated (n, tot) and (n, el) cross sections (solid line) compared with experimental data (symbols) for n+52Cr reaction.
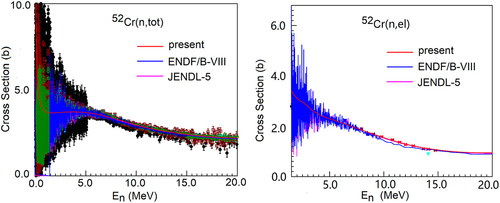
For the total cross sections, the measured data demonstrate a lot of fluctuations structure below 5.0 MeV for total cross sections of n+ 52Cr and n+ natCr reactions and it was impossible for the optical model to describe those structures, though calculated results pass reasonably through the experimental data. In order to fit the resonance structure of the experimental data, R-matrix theory must be used for energy En <5 MeV. For the elastic scattering cross-section, compared with the existing experimental data below 15 MeV, the calculated results passed through the data.
D. Schmidt et al. [Citation35] and A. B. Smith et al. [Citation38] measured the elastic scattering angular distributions at incident neutron energies 7.95~14.76 MeV and 4.5~9.9 MeV. The comparisons of the calculated results of the elastic scattering angular distributions with the experimental data are shown in .
Figure 2. Calculated neutron elastic scattering angular distributions (solid curves) compared with the experimental data (symbols) for incident energy in the range 7.95 to 14.76 MeV and 4.5 to 9.99 MeV. From 7.95 to 14.76 MeV and 4.5 to 9.99 MeV, the results were offset by factors of 10. The data at the top of the figure have not been adjusted.
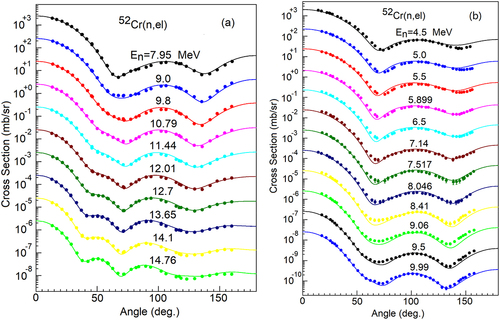
The theoretical calculation of the total cross section, elastic scattering cross section, and elastic scattering angular distributions were in good agreement with the experimental data. Therefore, the optical potential parameters determined by APMN code are reasonable, which are the basis of our theoretical calculations.
Moreover, D. Schmidt et al. [Citation35] and A. B. Smith et al. [Citation38] measured the inelastic scattering angular distributions for the first excited state. shows the comparisons of calculated results for inelastic scattering angular distributions of the first excited state with experimental data at incident neutron energies 7.95~14.76 MeV and 4.5~14.8 MeV.
Figure 3. Calculated neutron inelastic scattering angular distributions (solid curves) for the first excited state (1.434 2+) compared with the experimental data (symbols). From 7.95 to 14.76 MeV and 4.5 to 14.8 MeV, the results were offset by factors of 10. The data at the top of the figure have not been adjusted.
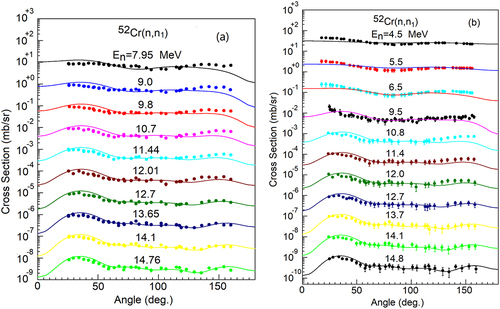
shows the comparisons of calculated results for the total inelastic scattering cross section with the experimental data. The calculated results below 5 MeV agreed well with the experimental data, and in the high-energy region were higher than the experimental data [Citation39–43] but were consistent with the evaluated data. The calculation of total inelastic scattering cross section considered of the contribution of discrete levels and continuous levels, so it was reasonable that the theoretical results were higher than the experimental data. Meanwhile, our calculation results have good consistency with ENDF/B-VIII.0 and JENDL-5.
Figure 4. Calculated (n, inl) reaction cross section (solid line) compared with experimental data (symbols) and evaluated data for n+52Cr reaction.
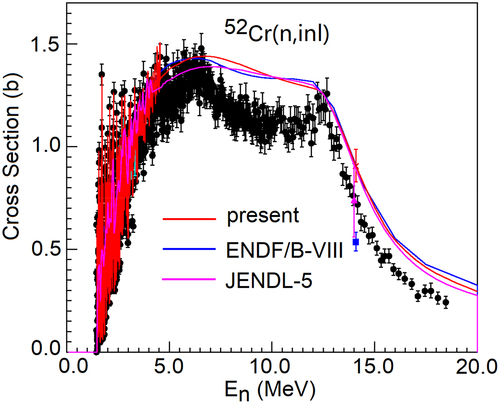
The inelastic scattering gamma production cross sections were measured mainly by L. C. Mihailescu et al. in 2007 [Citation43]. shows the comparisons of the calculated results with the existing experimental data [Citation43–49] for the transitions of the first excited state to the ground state, the second excited state to the first excited state, the third excited states to the second excited state. At relatively lower emission energies, the calculated results were in agreement with the experimental data.
Figure 5. Calculated gamma production cross sections (solid line) for the transition of the first excited state to the ground state, the second excited state to the first excited state and the third excited states to the second excited state of 52Cr compared with experimental data (symbols).
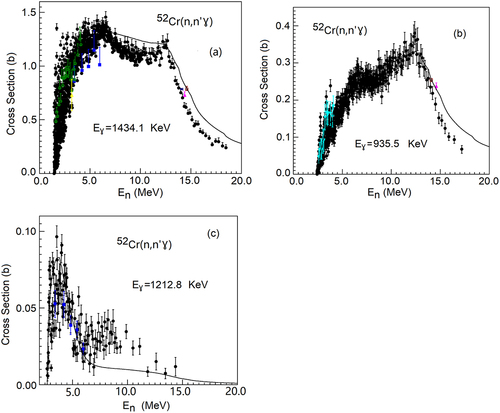
L. C. Mihailescu et al. [Citation43] also measured the discrete level inelastic scattering cross section. The comparisons of calculated results with experimental data [Citation34,Citation35,Citation41,Citation43,Citation50–54] for n+52Cr are given in . Below 5 MeV, the results of the discrete level inelastic scattering cross sections were in agreement with the experimental data.
Figure 6. Calculated neutron inelastic scattering cross sections (solid line) of the first excited state, the second excited state, and the third excited state compared with experimental data (symbols) and evaluated data for n+52Cr reaction.
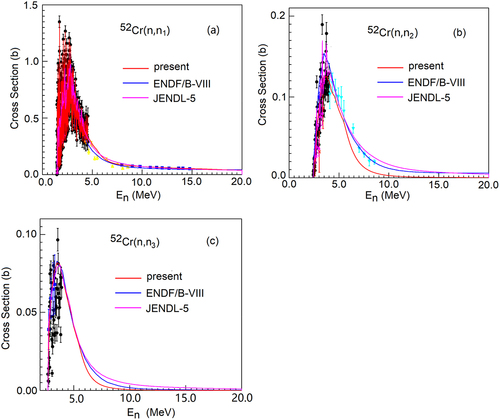
The 52Cr(n,2n) reaction cross section calculated results were in agreement with the experimental data [Citation39,Citation55–66] as shown in . In addition, (n,2nγ) reaction cross sections were also calculated. shows the comparisons between the calculated results with experimental data [Citation65] for the (n,2nγ) cross section of the transitions of the first excited state to the ground state and the third excited state to the ground excited state.
Figure 7. Calculated (n, 2n) reaction cross section (solid line) compared with experimental data (symbols) and evaluated data for n+52Cr reaction.
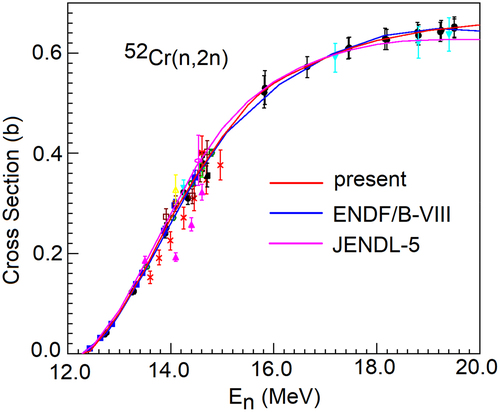
Figure 8. Calculated result of (n,2nɣ) reaction cross section (solid line) compared with the experimental data (symbols) at ɣ-ray energy of 749.1 KeV and 1164.4 KeV.
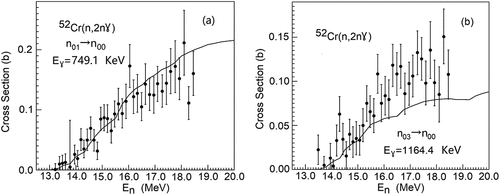
shows the comparisons of calculated results for the (n, p), (n, α), (n, d) reaction cross sections with the experimental data. For the (n, p) reaction cross section, our theoretical calculations were in good agreement with measurement of A. Fessler et al. [Citation66]. Above 8 MeV, our results were consistent with the available experimental data [Citation55,Citation56,Citation66–76].
Figure 9. Calculated (n, p), (n, α), (n, d) reaction cross section (solid line) compared with experimental data (symbols) and evaluated data for n+52Cr reaction.
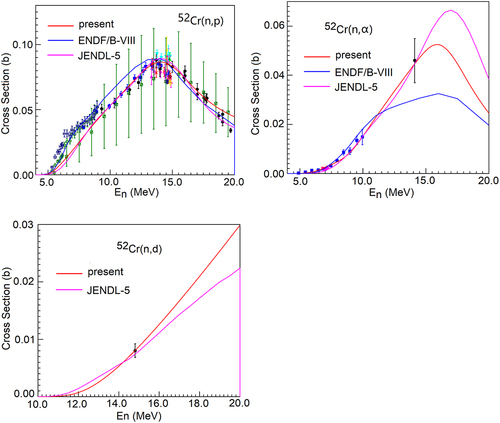
For the (n, α) reaction cross section, the experimental data of n+ natCr were used in the fitting, because the measured data of n+52Cr were scarce. The natural chromium experimental data were measured mainly by E. Wattecamps et al. and A. Paulsen et al., and the results were in good agreement with the experimental data [Citation77–79] within the error bar. For the (n, d) reaction cross section, S. M. Grimes et al. [Citation80] measured (n, d) cross sections of 52Cr at incident neutron energies 14.8 MeV, the present results and evaluated data in JENDL-5 passed through the experimental data within error bar.
D. Schmidt et al. [Citation81] measured emission neutron double-differential cross sections of natural Cr at incident neutron energies 7.95, 9.0, 9.8, 10.79, 11.44, 12.01, 12.7, and 14.1 MeV, and the contributions of elastic scattering channel were not included in measurements. The comparisons between the calculated results and measurements at incident neutron energies 10.79, 12.7 and 14.1 MeV for different angles as shown in . The double-differential neutron emission cross sections include the contributions of elastic scattering processes, direct inelastic scattering processes of discrete levels, and continuous inelastic scattering process. The calculated results of emission neutron double-differential cross sections at incident neutron energies 10.79, 12.7 and 14.1 MeV were basically in agreement with experimental data.
Figure 10. Calculated (n, xn’) double-differential neutron emission cross section (solid line) compared with experimental data (symbols) for different angles at 10.79 MeV, 12.7 MeV and 14.1 MeV incident energy. From 25.0 to 160.0 deg., the results were offset by factors of 10. The data at the top of the figure have not been adjusted.
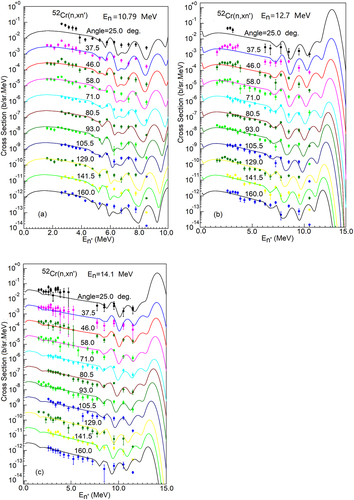
The experimental data of natural Cr for double-differential neutron emission cross sections were given in A. Takahashi [Citation82] at incident neutron energy from 13.35 to 14.83 MeV for different angles. shows the comparisons between the calculated results and measurements.
Figure 11. Calculated (n, xn’) double-differential neutron emission cross section (solid line) compared with experimental data (symbols) at incident neutron energy from 13.35 to 14.83 MeV for different angles. From 14.83 to 13.35 MeV, the results were offset by factors of 10. The data at the top of the figure have not been adjusted.
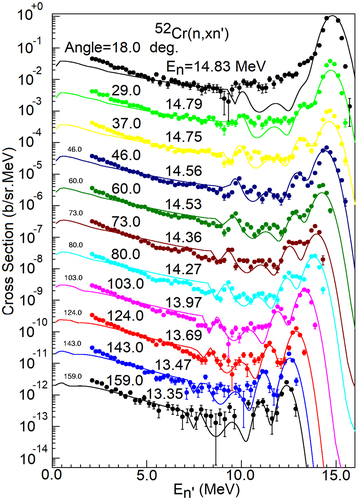
Moreover, A. Takahashi [Citation83] also measured the double-differential neutron emission cross sections at incident neutron energy 14 MeV with 16 angles. shows the comparisons between the calculated results and measurements. As shown in the figure, the part of the elastic scattering and the discrete level inelastic scattering agreed well with the experimental data, and the part of the continuous level was partly higher than the experimental data.
Figure 12. Calculated double-differential neutron emission cross section (solid lines) compared with experimental data (symbols) at incident energy of 14.1 MeV. From 15.0 to 80.0 deg. and 90.0 to 160.0 deg., the results were offset by factors of 10. The data at the top of the figure have not been adjusted.
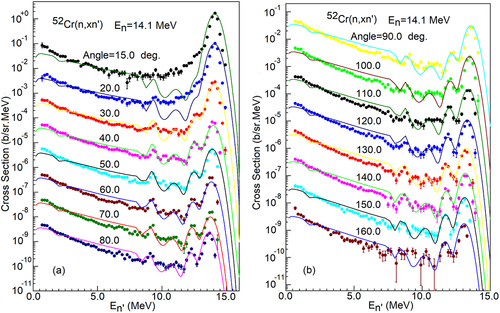
S. Matsuyama et al. [Citation84] measured double-differential neutron emission cross sections for natural Cr at incident neutron energy 14.1 MeV. The calculated results corresponded well with the experimental data as shown in . We also calculated the inelastic scattering neutron emission spectra for 52Cr at the neutron incident energy range from 7.94 to 14.1 MeV, as shown in . Experimental data were taken from the reference [Citation81]. Calculated results were in agreement with experimental data.
Figure 13. Calculated double-differential neutron emission cross section (solid lines) compared with experimental data (symbols) at incident energy of 14.1 MeV. From 20.0 to 150.0 deg., the results were offset by factors of 10. The data at the top of the figure has not been adjusted.
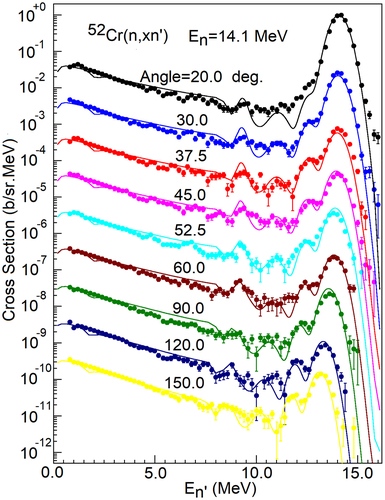
Figure 14. Calculated (n, xn’) neutron emission spectra (solid line) compared with experimental data (symbols) at 7.95, 9.0, 9.8, 10.79, 11.44, 12.01, 12.7, and 14.1 MeV incident energy. From 14.1 to 7.95 MeV, the results were offset by factors of 10. The data at the top of the figure have not been adjusted.
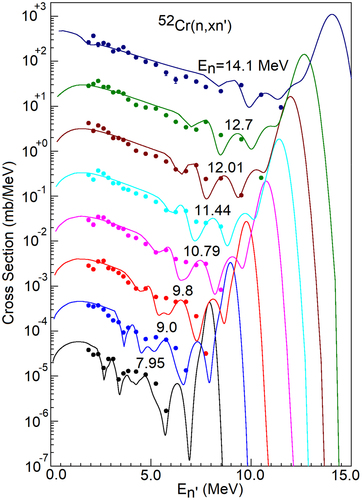
The double-differential cross sections of proton were calculated by the linear momentum dependent exciton state density. Since the improved pick up mechanism has been employed based on the Iwamoto-Harada model, the double-differential cross sections of alpha-particle and deuteron can be calculated by using a new method based on the Fermi gas model.
Therefore, The energy spectrum of proton, alpha-particle and deuteron for 52Cr at neutron incident energy 14.8 MeV. The experimental data and calculated results of proton, alpha-particle, and deuteron emission spectra are given in .
Figure 15. Calculated proton, alpha-particle, and deuteron emission spectra (solid line) compared with experimental data (symbols). From (n, xp) to (n, xd) MeV, the results were offset by factors of 10 except for (n, xp) reaction.
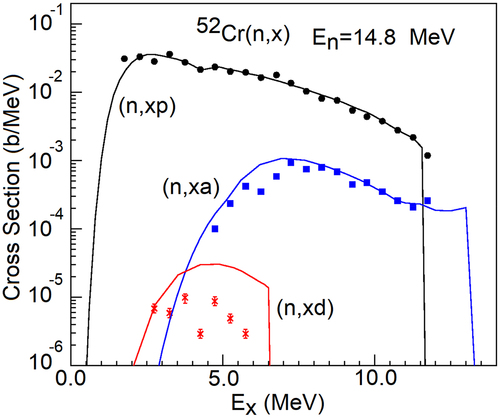
The calculated results of energy spectra for proton and alpha-particle were in agreement with experimental data. The calculation result of the energy spectrum for deuteron was higher than the experimental data [Citation80], but the (n, d) cross section were in agreement with the experimental data, so the results needed to be further discussed.
4. Conclusion
In this study, we obtained a set of optical model potential parameters for the n + natCr reaction with incident neutron energy below 20 MeV. The DWBA theory was used to calculate the direct inelastic scatterings to discrete excited states of 52Cr, and we determined the distorted parameters of the eight discrete energy levels with major contributions. On the basis of the spherical optical model, the unified Hauser–Feshbach theory and exciton model, we systematically calculated various cross sections, angular distributions, energy spectrum, double-differential cross sections, and γ production cross sections for the reaction of the n+52Cr reactions below 20 MeV. The theoretical calculation results were generally in agreement with the existing experimental data, together with the evaluated results in the ENDF/B-VIII.0, JENDL-5.
All of the present results have been transformed into the ENDF-6 format data files for application. In the present evaluated data file, the resonance parameters data of ENDF/B-VIII.0 were adopted. The evaluated results will be included in the new version of the Chinese Evaluated Nuclear Data Library (CENDL-4.0), which will be convenient for nuclear engineering application.
Disclosure statement
No potential conflict of interest was reported by the author(s).
References
- Wang ZW, Yan J, Peng ZX, et al. Experimental study of Cr-coated zirconium alloy cladding under simulated LOCA conditions. Nucl na Eng. 2023;44(2):122–128. doi: 10.13832/j.jnpe.2023.02.0122
- Chen H, Wang XM, Zhang RQ. Application and development progress of Cr-based surface coatings in nuclear fuel element: I. Selection, preparation, and characteristics of coating materials. Coatings. 2020;10(9):808. doi: 10.3390/coatings10090808
- Bischoff J, Delafoy C, Vauglin C, et al. AREVA NP’s enhanced accident-tolerant fuel developments: focus on Cr-coated M5 cladding. Nucl Eng Technol. 2018;50(2):223–228. doi: 10.1016/j.net.2017.12.004
- Kim HG, Kim IH, Jung YI, et al. Out-of-pile performance of surface-modified Zr cladding for accident tolerant fuel in LWRs. J Nucl Mater. 2018;510:93–99. doi: 10.1016/j.jnucmat.2018.07.061
- Kashkarov E, Afornu B, Sidelev D, et al. Recent advances in protective coatings for accident tolerant Zr-based fuel claddings. Coatings. 2021;11(5):557. doi: 10.3390/COATINGS11050557
- Ali A, Kim HG, Hattar K, et al. Ion irradiation effects on Cr-coated zircaloy-4 surface wettability and pool boiling critical heat flux. Nucl Eng Des. 2020;362:110581. doi: 10.1016/j.nucengdes.2020.110581
- Otuka N, Dupont E, Semkova V, et al. Towards a more complete and accurate Experimental Nuclear Reaction Data Library (EXFOR): international collaboration between nuclear reaction data centres (NRDC). Nucl Data Sheets. 2014;120:272–276. doi: 10.1016/j.nds.2014.07.065
- Brown DA, Chadwick MB, Capote R, et al. ENDF/B-VIII.0: the 8th major release of the nuclear reaction data library with CIELO-project cross sections, new standards and thermal scattering data. Nucl Data Sheets. 2018;148:1–142. doi: 10.1016/j.nds.2018.02.001
- Osamu I, Nobuyuki I, Satoshi K, et al. Japanese evaluated nuclear data library version 5: JENDL-5. J Nucl Sci Technol. 2023;60(1):1–60. doi: 10.1080/00223131.2022.2141903
- Becchetti FD, Greenlees GW. Nucleon-Nucleus Optical-Model parameters, A>40, E<50 MeV. Phys Rev. 1969;182(4):1190–1209. doi: 10.1103/PhysRev.182.1190
- Shen QB. APMN: a program for automatically searching optimal optical potential parameters in the E≤300 MeV energy region. Nucl Sci Eng. 2002;141(1):78–84. doi: 10.13182/NSE01-19CCA
- Pan H, Zhao YL, Li J, et al. Calculation and analysis for p+ 50,52,53,54, natCr reactions. Ann Nucl Energy. 2014;63:446–460. doi: 10.1016/j.anucene.2013.08.021
- Han YL, Shi YY, Shen QB. Deuteron global optical model potential for energies up to 200 MeV. Phys Rev C. 2006;74(4):044615. doi: 10.1103/PhysRevC.74.044615
- Xu YL, Guo HR, Han YL, et al. Helium-3 global optical model potential with energies below 250 MeV. Sci China Phys Mech Astron. 2011;54(11):2005–2014. doi: 10.1007/s11433-011-4488-5
- Xu YL, Guo HR, Han YL, et al. Applicability of the systematic helium-3 potential for triton-nucleus reactions. Int J Mod Phys E. 2015;24(1):1550005. doi: 10.1142/S0218301315500056
- Su XW, Han YL. Global optical model potential for alpha projectile. Int J Mod Phys E. 2015;24(12):1550092. doi: 10.1142/S0218301315500925
- Kunz P. Zero range distorted wave born approximation. Boulder (CO): University of Colorado; 2002.
- Zhang JS. A unified Hauser-Feshbach and exciton model for calculating double-differential cross sections of neutron-induced reactions below 20 MeV. Nucl Sci Eng. 1993;114(1):55–63. doi: 10.13182/NSE93-3
- Zhang JS. UNF code for fast neutron reaction data calculations. Nucl Sci Eng. 2002;142(2):207–219. doi: 10.13182/NSE02-02
- Chadwick MB, Obloinsky P. Linear momentum in the exciton model: consistent way to obtain angular distributions. Phys Rev C. 1991;44(5):R1740–R1744. doi: 10.1103/PhysRevC.44.R1740
- Iwamoto A, Harada K. Mechanism of cluster emission in nucleon-induced pre-equilibrium reactions. Phys Rev C. 1982;26(5):1821–1834. doi: 10.1103/PhysRevC.26.1821
- Zhang JS, Yan SW, Wang CL. The pick-up mechanism in composite particle emission processes. Z Physik A - Hadrons And Nuclei. 1992;344:251–258. doi: 10.1007/BF01303018
- Shen QB. Double-Differential cross section of light composite particles in the exciton model. Nucl Sci Eng. 1994;117(2):99–109. doi: 10.13182/NSE93-66
- Kalbach C. Exciton number dependence of the Griffin model two-body matrix element. Z Phys A. 1978;287(3–4):319–322. doi: 10.1007/BF01481712
- Shen QB. 低能和中能核反应理论(上册) [Theory of low and medium energy nuclear reactions (volume 1)]. Beijing: Science Press; 2005. Chinese.
- Capote R, Herman M, Obložinský P, et al. RIPL-reference input parameter library for calculation of nuclear reactions and nuclear data evaluations. Nucl Data Sheets. 2009;110(12):3107–3214. doi: 10.1016/j.nds.2009.10.004
- Carlton RF, Harvey JA, Larson DC, et al. Neutron resonance spectroscopy for n + 52Cr: total and differential elastic scattering cross sections. Phys Rev C. 2000;62(1):14608. doi: 10.1103/PhysRevC.62.014608
- Abfalterer WP, Bateman FB, Dietrich FS, et al. Measurement of neutron total cross sections up to 560 MeV. Phys Rev C. 2001;63(4):44608. doi: 10.1103/PhysRevC.63.044608
- Policroniades R, Varela A, López J, et al. An associated particle Time-of-Flight facility for neutron cross section measurement. Nucl Instrum Methods In Phys Res A. 1994;346(1–2):230–236. doi: 10.1016/0168-9002(94)90709-9
- Larson DC, Harvey JA, Hill NW. Measurement of neutron total cross sections at ORELA to 80 MeV. USA: Oak Ridge National Lab; 1981. (ORNL publication; No.5787).
- Perey FG, Love TA, Kinney WE A test of neutron total cross-section evaluations from 0.2 to 20 MeV for C, O, Al, Si, Ca, Fe, and Si02. USA: Oak Ridge National Lab; 1972. ( ORNL publication; No.4823).
- Foster DG, Glasgow DW. Neutron total cross sections, 2.5 - 15 MeV. I Experimental Phys Rev C. 1971;3(2):576–603. doi: 10.1103/PhysRevC.3.576
- Korzh IA, Mishchenko VA, Mozhzhukhin EN, et al. Study of neutron scattering in the energy region 1.5-3.0 MeV by even-even isotopes of Cr. Sov J Nucl Phys. 1977;26(6):1151.
- Kinney WE, Perey FG. Natural chromium and 52Cr neutron elastic and inelastic scattering cross sections from 4.07 to 8.56 MeV. USA: Oak Ridge National Lab; 1974. (ORNL publication; No.4806).
- Schmidt D, Mannhart W Elastic and inelastic neutron scattering on elemental chromium. Braunschweig (Ge):Phys.Techn.Bundesanst; 1998. ( Neutronenphysik Reports;No.31).
- Olsson N, Trostell B, Ramstrom E, et al. Microscopic and conventional optical model analysis of neutron elastic scattering at 21.6 MeV over a wide mass range. Nucl Phys A. 1987;472(2):237–268. doi: 10.1016/0375-9474(87)90209-0
- Takahashi A, Ichimura E, Sasaki Y, et al. Measurement of double differential neutron emission cross sections for incident neutrons of 14 MeV. J Nucl Sci Technol. 1987;25(3):215–232. doi: 10.1080/18811248.1988.9733582
- Smith AB, Schmidt D. Neutron scattering and models: chromium. USA: Argonne National Laboratory Argonne; 1987. doi: 10.1088/0954-3899/23/2/007.
- Lychagin AA, Simakov SP, Devkin BV, et al. Neutron spectra from 52Cr(N,N`G) and 208Pb(N,N`G) reactions at an incident neutron energy of 14.1 MeV; Symposiumon Nucleon Induced Reactions; Smolenice, Czechoslov. 1988; p. 272.
- Tagesen S, Hille P. Competing gamma emission in the (N,2N) reaction on 24-Cr-52. Acta Phys Austr. 1966;23:31.
- Van Patter DM, Nath N, Shafroth SM, et al. Gamma rays from inelastic neutron scattering in chromium. Phys Rev. 1962;128(3):1246. doi: 10.1103/PhysRev.128.1246
- Simakov SP, Androsenko AA, Androsenko PA, et al. 14-MeV facility and research in IPPE. Vop At Nauki I Tekhn. 1992;3-4:93.
- Mihailescu LC, Borcea C, Koning AJ, et al. High resolution measurement of neutron inelastic scattering and (n,2n) cross-sections for 52Cr. Nucl Phys A. 2007;799(1–4):1–29. doi: 10.1016/j.nuclphysa.2007.11.007
- Grozdanov DN, Fedorov NA, Kopatch YN, et al. Measurement of the yield and angular distributions of gamma rays originating from the interaction of 14.1-MeV neutrons with chromium nuclei. Phys N A Nucl. 2020;83(3):384–390. doi: 10.1134/S1063778820030096
- Lashuk AI, IP S. Gamma-quanta production cross-sections at inelastic scattering of the neutrons on the nuclei of reactor construction materials. Vop At Nauki I Tekhn. 1994;1:26.
- Oblozinsky P, Hlavac S, Maino G, et al. Gamma-ray production from 52Cr(n, xγ) reactions at 14.6 MeV. Nuovo Cimento A. 1992;105(7):965–985. doi: 10.1007/BF02730838
- Tessler G, Glickstein S. γ-ray production cross sections for neutron inelastic scattering from Cr, ni, 92Zr, and 94Zr from 3 to 6 MeV. USA: Bettis Atomic Power Lab; 1975. WAPD-T-2566; CONF-740201-7.
- Breunlich W, Stengel G, Vonach H. Bestimmung von (n n’γ)-Querschnitten für 14,4 MeV-Neutronen im Massebereich A = 46 — 88. Z Naturforschung A. 1971;26(3):451–457. German. doi: 10.1515/zna-1971-0315
- Scherrer VE, Allison BA, Faust WR. Gamma radiation from interaction of 3.2-MeV neutrons with various metals. Phys Rev. 1954;96(2):386. doi: 10.1103/PhysRev.96.386
- Olsson N, Ramstrom E, Trostell B. Neutron elastic and inelastic scattering from Mg, Si, S, Ca, Cr, Fe and niat E(n) = 21.6 MeV. Nucl Phys A. 1990;513(2):205–238. doi: 10.1016/0375-9474(90)90096-5
- Kramarovskiy YM, Nemilov YA, Pobedonostsev LA, et al. The excitation functions of neutron inelastic scattering for 52Cr, 53Cr and Al-27. Vop At Nauki I Tekhn. 1989;1:30.
- Korzh IA, Mishchenko VA, Mozhzhukhin EN, et al. Neutron scattering in energy range 1.5-3.0 MeV on the even isotopes of Cr, Ni, Zn. Yadernaya Fiz. 1977;26:234.
- Degtyarev YG, Protopopov VN. (N, n`γ) reactions on 27Al, 51V, 52Cr, 55Mn, 56Fe, 93Nb, 208Pb, and 209Bi: gamma spectra and cross sections. Ser Fiz. 1971;35(11):2341.
- Pasechnik MV, Fedorov MB, Yakovenko TI, et al. Scattering of 2.9 MeV neutrons on titanium and chromium nuclei. Ukrainskii Fizichnii Zhurnal. 1969;14(11):1874.
- Pasha I, Rudraswamy B, Santhi SY, et al. Measurement of 14.54 MeV neutron induced reaction cross sections of Cr and Mn with covariance analysis. Radiochim Acta. 2020;108(9):679. doi: 10.1515/ract-2019-3189
- Mannhart W, Schmidt D Measurement of neutron activation cross sections in the energy range from 8 MeV to 15 MeV. Germany: Phys. Techn. Bundesanst.; 2007. ( Neutronenphysik reports; No.53).
- Zhou FQ, Yi YL, Tuo F, et al. Measurement of cross sections for 50-Cr(n,2n)49-Cr and 52-Cr (n, 2n)51-Cr reactions induced by 13.5-14.6 MeV neutrons. High Energy Phys Nucl Phys. 2005;29:644.
- Molla NI, Miah RU, Basunia S, et al. Excitation functions of (n, p), (n, α) and (n, 2n) processes on some isotopes of Cl, Cr, Ge, Mo and Ce in the energy range 13.57-14.71 MeV. Con Nucl Data For Sci Techn. 1997;1:517.
- Ercan A, Erduran MN, Subasi M, et al. 14.6 MeV neutron induced reaction cross section measurements. Conf Nucl Data For Sci Technol. 1991;376. doi: 10.1007/978-3-642-58113-7_110
- Liskien H, Uhl M, Wagner M, et al. The excitation function for the reaction 52Cr(N,2N)51Cr. Ann Nucl Energy. 1989;16(11):563–570. doi: 10.1016/0306-4549(89)90010-8
- Wagner M, Winkler G, Vonach H, et al. Measurement of the cross sections for the reactions 52Cr(n,2n)51Cr,66Zn(n,2n)65Zn,89Y(n,2n)88Y and 96Zr(n,2n)95Zr from 13.5 to 14.8 MeV. Ann Nucl Energy. 1989;16(12):623–635. doi: 10.1016/0306-4549(89)90139-4
- Ikeda Y, Konno C, Oishi K, et al. Activation cross section measurements for fusion reactor structural materials at neutron energy from 13.3 to 15.0 MeV using FNS facility. Nagoya (Japan): Japan Atomic Energy Agency; 1988. ( JAERI Reports; No.1312).
- Ribansky I, Panteleev T, Stoeva L, et al. Neutron activation cross-sections for Cr isotopes at 14.6 MeV neutron energy. Ann Nucl Energy. 1985;12(11):577–581. doi: 10.1016/0306-4549(85)90010-6
- Bahal BM, Pepelnik R. Cross section measurements of Cr, Mn, Fe, Co, Ni for an accurate determination of these elements in natural and synthetic samples using a 14 MeV neutron generator. Germany: Ges. Kernen. - Verwertung; 1985. (INDC; No.85-E-11).
- Mihailescu LC, Borcea C, Koning AJ, et al. High resolution measurement of neutron inelastic scattering and (n,2n) cross-sections for 52Cr. Nucl Phys A. 2007;786(1–4):1. doi: 10.1016/j.nuclphysa.2007.01.004
- Fessler A, Wattecams P, Smith DL, et al. Excitation functions of (n, 2n), (n, p), (n, np + pn + d), and (n, α) reactions on isotopes of chromium. Phys Rev C Nucl Phys. 1998;58(2):996. doi: 10.1103/PhysRevC.58.996
- Kasugai Y, Yamamoto H, Kawade K, et al. Measurement of (n, p) cross-sections for shortlived products by 13.4-14.9 MeV neutrons. Ann Nucl Energy. 1998;25(1–3):23–45. doi: 10.1016/S0306-4549(97)00040-6
- Osman KT, Habbani FI. Measurement and study of (n, p) reaction cross sections for Cr, Ti, Ni, Co, Zr and Mo isotopes using 14.7 MeV neutrons. Sudanese (Austria): University of Khartoum; 1996. (INDC report; No.001).
- Ercan A, Erduran MN, Subasi M, et al. 14.6 MeV neutron induced reaction cross section measurements. Berlin (Germany): Nucl Data For Sci Technol. 1991. (ISBN Reports; No. 978-3-540-55100-3). doi: 10.1007/978-3-642-58113-7_110
- Pansare GR, Dighe PM, Bhoraskar VN. Cross-sections of a few (n,α) and (n, p) reactions induced at 14.7 MeV neutrons. Pramana - J Phys. 1991;37(5):425. doi: 10.1007/BF02848511
- Ikeda Y, Konno C, Oishi K, et al. Activation cross section measurements for fusion reactor structural materials at neutron energy from 13.3 to 15.0 MeV using FNS facility. Japan: Japan Atomic Energy Agency; 1988. ( JAERI Reports; No.1312).
- Smith DL, Meadows JW. Cross-section Measurement for the 52 Cr(n,p) 52 V reaction near threshold. Nuclear Science And Engineering. 1980;76(1):43. doi: 10.13182/NSE80-A19291
- Lalremruata B, Otuka N, Tambave GJ, et al. Systematic study of (n, p) reaction cross sections from the reaction threshold to 20 MeV. Phys Rev C Nucl Phys. 2012;85(2):024624. doi: 10.1103/PhysRevC.85.024624
- Yoshitomo U, Hiroshi S, Yuhri K, et al. Measurement of neutron activation cross sections for energies up to 40 MeV for natural samples of Mg, Si, Ca, V, Cr, Cu and Zn. Jour Nucl Sci Technol. 1994;31(1):1. doi: 10.3327/jnst.31.1
- Lai YC, Zasadny K. Knoll GF. Measurement of the Cr (N, X)52V neutron cross section at 14.6 MeV. Trans The American Nucl Soc. 1986;53:466.
- Sailer K, Daroczy S, Raics P, et al. The cross sections of (n, 2n), (n, p), (n, a) reactions for 14.8 MeV neutrons on isotopes of Cr and Zr. All Union Conf. on Neutron Phys; Apr 18-22; Kiev, USSR. 1977 Vol. 1, p. 246.
- Tatiana K, Ivan B, Alexander G, et al. Investigation of (n, α) reaction cross sections for a number of structural material isotopes. Nucl Sci Eng. 2018;191(3):282. doi: 10.1080/00295639.2018.1463746
- Wattecamps E, Liskien H, Arnotte F. Measurement of (N, A) cross measurement of (N, A) cross-sections for Cr, Fe and Ni at 14 MeV neutron energy. Nucl Data For Sci Technol. 1982;19(2):159. doi: 10.1007/978-94-009-7099-1_32
- Paulsen A, Liskien H, Arnotte F, et al. Measurement of (n, α) cross sections of chromium, iron, and nickel in the 5-to 10- MeV neutron energy range. Nucl Sci Eng. 1981;78(4):377. doi: 10.13182/NSE81-A21371
- Grimes SM, Haight RC, Alvar KR, et al. Charged-particle emission in reactions of 15-MeV neutrons with isotopes of chromium, iron, nickel, and copper. Phys Rev C Nucl Phys. 1979;19(6):2127. doi: 10.1103/PhysRevC.19.2127
- Schmidt D, Mannhart W, Siebert BRL. Measurement of elastic, inelastic and double-differential neutron scattering cross sections on V, Cr and Pb at energies between 8 MeV and 15 MeV. Nucl Data Sci Techn. 1997;1:407.
- Takahashi A, Yamamoto J, Oshima K, et al. Measurements of double differential neutron emission cross sections for fusion reactor candidate elements. Jour Nucl Sci Technol. 1984;21(8):577. doi: 10.1080/18811248.1984.9731087
- Takahashi A, Ichimura E, Sasaki Y, et al. Measurement of double differential neutron emission cross sections for incident neutrons of 14 MeV. J Nucl Sci Technol. 1988;25(3):215–232. doi: 10.1080/18811248.1988.9733582
- Matsuyama S, Okubo T, Baba M, et al. Measurement of double-differential neutron emission cross sections of Mo, Ta and W. Japan: Tohoku Univ; 1993. (JAERI-M Reports; No.93-046).