ABSTRACT
We investigated the possibility of estimating the effective neutron multiplication factor (keff) of the fuel debris inside the canister and primary containment vessel (PCV) of Unit 2 of the Fukushima Daiichi Nuclear Power Station (1F) using remote gas-radioactivity measurement via Monte Carlo simulation-based calculations. Our results demonstrate an almost linear correlation between keff and the 88Kr-to-135Xe activity ratio with respect to various fuel debris compositions. This correlation is maintained regardless of geometries such as the fuel debris canister and the PCV.
1. Introduction
The characteristics and composition of fuel debris at the Fukushima Daiichi Nuclear Power Station (1F) are considered diverse and complicated depending on the location of the debris in the primary containment vessel (PCV), differences in the fuel burn-up conditions before the accident [Citation1], the progression of the severe accident in each unit, and other events. Fuel debris is formed during accidents when fuel mixes with other materials, such as fuel cladding (zirconium alloy), structural material (mainly steel), control blade (B4C), air, water, concrete, and other materials inside the PCV [Citation2]. Fuel and nonfuel materials can introduce variations in the composition of fuel debris. Internal investigations of the PCV at 1F showed fuel debris in various shapes and sizes [Citation3].
A significant amount of the fuel debris is expected to accumulate in the lower part of the reactor pressure vessel (RPV) of 1F Unit 2, and some fuel debris will relocate from the RPV and accumulate in the pedestal area [Citation4].
Fuel debris removal is a crucial step in the entire 1F decommissioning process. According to the road map of 1F decommissioning [Citation5], the fuel debris retrieval method starts by inserting a special retrieval equipment through existing penetration holes in the PCV. The retrieved fuel debris is placed in a container, transferred to a storage facility in the 1F, and stored in dry conditions. Fuel debris removal will be first performed for the 1F Unit 2 as a trial.
Fuel debris must be retrieved and stored safely under stable conditions [Citation4]. For the safety management of fuel debris and establishing stable conditions, knowing the fuel debris parameters related to safety, such as the effective neutron multiplication factor (keff) and the amount of fissile material, is crucial. Research related to fuel debris composition, shapes or physical form, and distribution inside the PCV is ongoing; however, detailed information on the fuel debris with respect to these parameters is not available yet [Citation6]. Consequently, determining keff and other safety parameters related to fuel debris via calculations is difficult. In addition, very high gamma radiation inside the PCV limits the available options for measuring the necessary parameters, especially those needed for keff estimation.
The spent fuel or fuel debris contains nuclides with spontaneous fission (SF) reactions [Citation7]. A recent study shows the possibility of quantifying SF nuclides inside the storage or canister [Citation8]. The SF reactions produce neutrons and fission products (FPs) such as 88Kr and 135Xe. The neutrons subsequently trigger induced fission (IF) reactions with available fissile nuclides such as 235U and 239Pu. With the information of the quantity of SF nuclides in fuel debris, we can estimate SF reaction rate and FPs yields by relatively straightforward radioactive decay calculation. However, the estimation of IF reaction rate and FPs yields is more difficult, because we need to solve a neutron transport problem for a subcritical system with fixed neutron source (in this case from SF nuclides) by solving neutron transport equation or by particle simulation using Monte Carlo method (utilized in the current paper). Since yields of FPs such as 88Kr and 135Xe produced from SF nuclide such as 244Cm are different from the ones by IF reactions such as 235U and 239Pu [Citation9], we can define correlation between the number of FPs such as 88Kr and 135Xe and the number of IF reactions which is influenced strongly by keff.
Naito et al. derived an equation to calculate keff from an activity ratio (A(88Kr/135Xe)) of two noble-gas radioactive nuclides (88Kr and 135Xe) based on an analytical approach for a simplified fuel debris model, including 235U and 244Cm [Citation10]. Here, 88Kr and 135Xe half-life are 2.83 and 9.14 h, respectively. We consider the approach proposed by Naito et al. because of the complicated nature of fuel debris; however, it is not enough to estimate the correlation between keff and the A(88Kr/135Xe) for fuel debris.
In our previous study, we developed a new depletion calculation code to estimate the A(88Kr/135Xe) [Citation11]. This code can solve neutron transport and depletion calculations of subcritical systems with an inherent neutron external-source problem by using particle simulation (Monte Carlo method). The code contains a function for evaluating the quantity of short half-life FPs buildup due to SF and IF. In Ref [Citation11], A(88Kr/135Xe) was estimated for a canister with a hypothetical nuclear material comprising UO2 and 0.1 wt.% 244Cm for code validation.
This paper applies the depletion code to many fuel debris conditions in the decommissioning process in the fuel debris canister and the PCV [Citation12]. We model the fuel debris canister to investigate the correlation between the keff and the A(88Kr/135Xe) for many fuel debris compositions. We also model the PCV to study the keff–A(88Kr/135Xe) correlation in different geometry models.
shows the image of applications of 88Kr and 135Xe measurements to estimate the keff of the PCV and the fuel debris canisters. Noble gases such as 88Kr and 135Xe are chemically inert and will be released easily from fuel debris into the surrounding air [Citation13]. An additional advantage of short half-life FP (88Kr and 135Xe) gamma-ray emitting gas measurement is that it can be performed remotely in place with lower gamma-ray backgrounds.
In this preliminary study, it is assumed that all or the same rate of 88Kr and 135Xe released from fuel debris will be detected without time-delay of their migration times to a measurement point. The validity of this assumption and the appropriate correction methods [Citation14] will need to be confirmed in advance of 1F implementation.
This study aims to find correlations between the A(88Kr/135Xe) and the keff for the fuel debris canister and the PCV without requiring prior detailed knowledge of the fuel debris compositions and shapes.
2. Calculation methods
2.1. Geometry model
2.1.1. Fuel debris canister
We used an existing design for investigating the canister-geometry model and materials [Citation12]. The canister contains vent valves to allow hydrogen gas to flow out. However, these valves are omitted in the model geometry shown in .
Reference [Citation12]-predicted fuel debris filling rate accounts for 22% of the canister volume, including 11% water and 67% air. This is the conservative filling rate. However, we investigated the influence of the filling rate and water fraction on the keff–A(88Kr/135Xe) correlation by calculating cases involving filling rates other than the conservative filling rate.
The canister used in the calculation herein has an inner diameter of 220mm, an inner height of 800mm, and an internal volume of 0.030m3. The canister material is stainless steel SS316L with a thickness of 10mm.
2.1.2. The PCV
The PCV geometry model and material compositions of the 1F Unit 2 used in this study are based on those reported in a previous study conducted by our group [Citation15]. shows the PCV geometry model used for calculations in this study. In this model, only the fuel debris from the lower part of the pedestal is considered for calculations. The fuel debris is assumed to fill up two PCV floor sump pits having a flat surface with a depth of 40cm (excluding fuel debris in the sump pit).
2.2. Calculation case description
In this study, we perform a fixed-source subcritical depletion calculation to obtain the A(88Kr/135Xe) value of the canister and the PCV. Moreover, an eigenvalue calculation is performed to obtain the keff of the canister.
Herein, we use several general assumptions and calculation conditions as follows. (1) The fuel debris used in the calculation herein is the fuel debris from 1F Unit 2, with 10 years of storage time. (2) The subcritical depletion calculation period is two months, divided into two depletion-time intervals of one month. Therefore, the A(88Kr/135Xe) in the calculation indicates the data after the fuel debris has resided in the canister or the PCV for two months. (3) The fuel debris, water, and air are homogeneously mixed inside the canister and the PCV. (4) As in our previous paper, all horizontal boundary condition calculations are reflective, and vacuum boundary condition calculations are set at the bottom and top parts of the fuel debris canister. This model represents an infinite array of the same subcritical canister with a 300-mm lattice pitch.
We model the characteristics and composition of the fuel debris at the 1F Unit 2 using the fuel-inventory data before the accidents provided by authors [Citation16] and consider the FPs released during the accidents based on Phebus FPT4 [Citation13]. The fuel (mainly UO2) in the fuel debris contains FPs and actinides specifically modeling the 1F Unit 2 average core compositions before the accident. The A(88Kr/135Xe) of the current work was derived using a total yield of 88Kr and 135Xe produced from SF reactions of 244Cm mainly and IF reactions of 235U, 239Pu, and a trace of other fissile nuclides. The following subsections present several calculation cases to illustrate the fuel debris compositions for the canisters and the PCV.
2.2.1. Fuel debris types
The nonfuel materials included in the 1F Unit 2 PCV, such as zirconium alloy, structural material, air, water, concrete, and other materials, can be mixed with fuel and form fuel debris. We categorize the fuel debris types roughly in to four types (). The molten core–concrete interaction (MCCI) is a byproduct of the concrete interaction with the fuel.
Table 1. Fuel debris types.
We investigate the correlation among the fuel debris types, canister criticality, and the A(88Kr/135Xe) for the averaged core compositions (an average burn up of 24 GWd/t) with the conservative filling rate.
2.2.2. Canister filling rate and water contents
Since the fuel debris can have different shapes, sizes [Citation3], and water contents [Citation12], we must investigate the canister filling rate and water content influence on the keff–A(88Kr/135Xe) correlation.
We vary the canister filling rates of fuel debris from 10 vol% to 70 vol% of the canister volume with 3 vol% interval. The water volume fraction of the canister is 11 vol%, and the rest represents air. Because water is likely to be present in the fuel debris, we calculate cases where the water volume fraction varies from 1% to 15% while the fuel debris is kept constant at 22% of the canister volume. The fuel debris type used is the molten fuel in at the averaged core compositions.
2.2.3. Fuel debris inside the PCV of the 1F unit 2
We assume the fuel debris inside the PCV also has an averaged core composition, and all the fuel debris shown in could be distributed. However, with the current information, knowing the fuel debris location, fuel debris type, and distribution inside the PCV is challenging. We simulate various fuel debris compositions by varying the fraction of UO2 in the fuel debris from 1 vol% to 70 vol% and the ZrO2 from 69 vol% to 0 vol%. The water volume fraction is 10 vol%, and the rest (20 vol%) is filled with structural materials. The keff–A(88Kr/135Xe) correlation for the fuel debris in the PCV are estimated for two conditions: submerged in water or air.
2.2.4. Fuel debris with fuel components of non-average core compositions
Based on refueling and operation history before the accident, fuel burn-up in the reactor core varied roughly at 3, 15, 20, 30, and 40 GWd/t. After the accident, almost all fuels melted, mixed, and relocated. As a result, we expect fuel debris will have fuel of average core compositions. However, fuel debris stored in the canisters may not have an average core composition because of its small capacity. There is a possibility that stored fuel debris in different canisters will have different compositions (e.g. with higher or lower burn-up) compared to the average core. Therefore, to clarify the characteristics of fuel debris of non-average core compositions, we utilize fuel of varied fuel burn-up (e.g. 3.31, 15.7, 24.0, and 41.0 GWd/t) with fuel debris compositions similar to Section 2.2.1 and 2.2.2.
3. Results and discussion
3.1. Canister filling rate, water content, and types of fuel debris
shows the correlation between keff and A(88Kr/135Xe) under a fuel debris variation based on filling rate, water fraction, and fuel debris type listed in . The influence of a fuel debris type having the conservative filling rate for the core-averaged compositions on the keff–A(88Kr/135Xe) correlation is linear.
Figure 4. Canister activity ratio of 88Kr-to-135Xe versus criticality (keff) with respect to various mixing parameters at the average core composition.
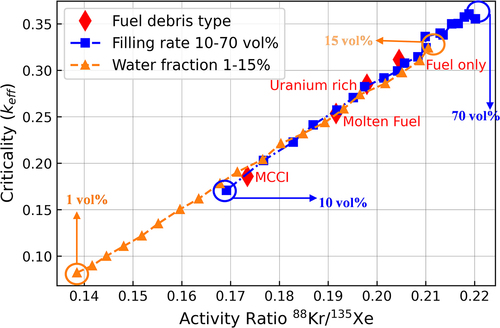
Fuel debris types with high fuel content (fuel only) exhibit high A(88Kr/135Xe) and keff, followed by uranium-rich molten fuel and MCCI (the lowest fuel content). Despite of the fuel debris type, the keff–A(88Kr/135Xe) has a linear relationship.
The filling rate of the fuel debris canisters depends on the fuel debris size and dimensions. The number of IF decreases when the quantity of nuclear material (fuel) in the fuel debris is low. Hence, the SF nuclides dominate the short half-life FPs production. For burn up>15 GWd/t, 244Cm accounts for more than 94% of the total SF sources, followed by 240Pu, accounting for<5% and 242Pu, accounting for<1% [Citation7]. Therefore, the A(88Kr/135Xe) value is determined by the 244Cm SF yields related to 88Kr and 135Xe.
Owing to the increasing filling rate of the canisters, subsequent number of IF increases. The 235U IF fission yields related to 88Kr and 135Xe differ from the one given by 244Cm. shows an increase in the filling rate from 10 vol% to 70 vol%, resulting in an approximate change in the A(88Kr/135Xe) value from 0.17 (mostly SF) to 0.22 (a combination of SF and IF).
The keff value of the canisters increases with the quantity of nuclear material that improves with the increasing filling rate of the canisters. As shown in , the keff roughly increases from 0.16 to 0.36. Hence, the correlation between the keff and A(88Kr/135Xe) values is linear.
Because of the high likelihood of water being present in the fuel debris, as mentioned in section 2.2.1, water has a significant influence on the keff–A(88Kr/135Xe) correlation of the canisters. With increasing water fraction inside the canister, the A(88Kr/135Xe) value increases, even though the nuclear material including contribution of the A(88Kr/135Xe) from SF nuclides e.g. 244Cm remained constant. Owing to the increasing quantity of the water fraction inside the canisters, the keff value of the canister increases roughly from 0.08 to 0.32. Because water acts as a moderator, increasing water quantity increases the population of thermal neutrons. These thermal neutrons are highly likely triggering IF of fissile materials (e.g.235U and 239Pu) and increase the A(88Kr/135Xe) value. shows the increase in the water fraction from 1 vol% to 15 vol%, resulting in the A(88Kr/135Xe) value changing from approximately 0.14 (influence of mostly SF) to 0.21 (influence of SF and IF).
Therefore, as shown in , under a specific burn-up degree, the variation in the composition of fuel debris based on the canister filling rate, fuel debris type, water content, or combinations of these three mixing parameters produces a linear correlation between the keff and A(88Kr/135Xe) values, with similar slopes.
3.2. Correlation among the mixing parameters of fuel debris inside the PCV of 1F unit 2
shows the correlation between the keff and A(88Kr/135Xe) values for a variety of fuel debris compositions based on the description provided in section 2.2.3.
Figure 5. Correlation between the activity ratio of 88Kr/135Xe and keff for various types of fuel debris in the PCV and the canister at the average core composition.
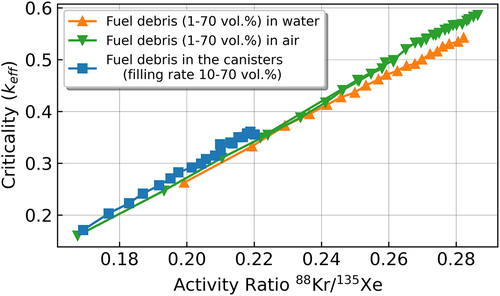
In section 2.2.3, we assumed the fuel debris had water mixed inside. We compare two cases: fuel debris exposed to air and fuel debris submerged in water, inside the pedestal. shows a graph submerged in air and water are almost similar, therefore the effect of water outside fuel debris is relatively small.
The correlations between the keff and the A(88Kr/135Xe) values are linear for both submerged in water or air. For comparison, we include the graph of the fuel debris canister presented in to demonstrate the similarity of the correlation between the keff–A(88Kr/135Xe) values with respect to the fuel debris canister and PCV. shows that the correlation between the keff and A(88Kr/135Xe) values is not sensitive to geometry.
3.3. Fuel debris with non-average core compositions
As mentioned in the Introduction and Section 2.2, the fuel components inside fuel debris contain various fissiles and SF nuclides. Each has a different yield of 88Kr and 135Xe so values of A(88Kr/135Xe) for individual fission-capable nuclides are constant and tend to be specific. The overall A(88Kr/135Xe) of the canister or the PCV is defined by the type and quantity of SF and IF.
show an intuitively simple correlation between the keff and A(88Kr/135Xe). When the keff is low, the A(88Kr/135Xe) also gives a low value and vice versa. We describe the detailed mechanism in the followings, when the keff values are relatively low, the majority of 88Kr and 135Xe yields will come from SF of mainly 244Cm (>95%). Since the A(88Kr/135Xe) of 244Cm is low (~0.1), the overall A(88Kr/135Xe) approaches 0.1. On the contrary, when the keff is relatively high, 88Kr and 135Xe from IF of mainly 235U became dominant. As a result, the A(88Kr/135Xe) will approach 0.5, because A(88Kr/135Xe) of 235U is~0.5.
239Pu in the fuel debris of the average core is~20% of 235U mass, other fissile nuclide such as 241Pu and 233U has collective mass below 2% of 235U. The A(88Kr/135Xe) of 239Pu IF is ~ 0.2, which is smaller than the one of 235U, therefore, when the keff is high, the overall A(88Kr/135Xe) is approaching value<0.5.
Difference in the correlations between keff and A(88Kr/135Xe) appear when compositions of SF nuclides and IF fissile nuclides in the fuel debris change, shows the relationship between the keff and A(88Kr/135Xe) at several different fuel burn-up (e.g. 3.31, 15.7, 24.0, and 41.0 GWd/t). Each specific burn-up graph shows a linear correlation between the canister keff and A(88Kr/135Xe) similar to .
Figure 6. Canister activity ratio of 88Kr-to-135Xe versus criticality (keff) under various conditions at different fuel burn-up.
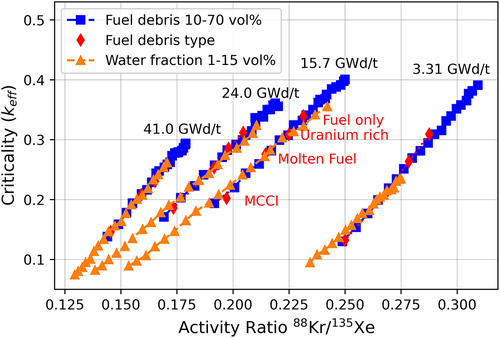
As mentioned above, each canister in this Section filled with fuel debris with a single burn-up fuel. In reality, these conditions are less probable because after all fuels melted, mixed with non-fuel material, and relocated to the bottom of RPV and inside the pedestal, the fuel with different burn-up values is likely to be mixed and its compositions will be closer to the average core conditions. also gives possibility of the A(88Kr/135Xe) measurement to predict burn-up or fissile nuclide contents in the fuel debris.
4. Conclusions and future works
We investigated the correlation between the keff and A(88Kr/135Xe) values with respect to canisters and the PCV under different fuel debris compositions using a novel depletion calculation code. The calculation results showed that the correlation between canister criticality and A(88Kr/135Xe) was linear, with similar slopes for various mixing parameters, such as fuel debris filling rates, water fractions, and fuel debris types, when the fuel isotope composition remained unchanged. Moreover, the keff–A(88Kr/135Xe) correlation is not sensitive to geometry such as in the fuel debris canister or in the PCV. These results indicate that the activity ratio A(88Kr/135Xe), which can be measured from a place nearly free from gamma ray backgrounds, can be employed for monitoring criticality.
We assume 88Kr and 135Xe migration from fuel debris to its surrounding air is relatively fast compared to its half-life, therefore 88Kr and 135Xe released from fuel debris will be detected without time-delay of their migration times to a measurement point. We also assume the similarity of chemical and physical behaviors between 88Kr and 135Xe. In future work, we will perform a detailed analysis of 88Kr and 135Xe migration in the PCV or another large container to accurately predict the keff. In addition, we will carry out experiments for code validation, assess the practicality of 88Kr and 135Xe measurements, and explore the possibility of the proposed method to predict burn-up or fissile nuclide contents in the fuel debris.
Disclosure statement
No potential conflict of interest was reported by the author(s).
References
- International Atomic Energy Agency (IAEA). The Fukushima Daiichi accident technical. In: Description and context of the accident. Vol. 1. Austria: IAEA; 2015. p. 135–137.
- TEPCO. [Internet]. https://www.tepco.co.jp/en/hd/decommission/progress/retrieval/index-e.html
- TEPCO. Fukushima Daiichi nuclear power station unit 2 primary containment vessel internal investigation results. Japan: TEPCO; 2019. https://www.tepco.co.jp/en/wp-content/uploads/handouts_190228_01-e.pdf
- Kurata M, Okuzumi N, Nakayoshi A et al. Step-by-step challenge of debris characterization for the decommissioning of Fukushima-Daiichi Nuclear Power Station (FDNPS). J Nucl Sci Technol. 2022;59(7):807–834. doi: 10.1080/00223131.2022.2040393
- Nuclear emergency response headquarters council for the decommissioning of TEPCO’s Fukushima Daiichi Nuclear Power Station. Mid-and-long-term roadmap towards the decommissioning of TEPCO’s Fukushima Daiichi Nuclear Power Station units 1-4, 2013 Jun 27. Recently revised in December 27, 2019, by The Inter-Ministerial Council for Contaminated Water and Decommissioning Issues. Available from: https://www.meti.go.jp/english/earthquake/nuclear/decommissioning/pdf/20191227_3.pdf
- International Research Institute for Nuclear Decommissioning (IRID). Development of analysis and estimation technologies for fuel debris characterization. Japan: IRID; 2020. (FY2019 Research Report).
- Richard JG, Fensin ML, Tobin SJ et al. Characterization of the neutron source term and multiplicity of a spent fuel assembly in support of NDA safeguards of spent nuclear fuel. USA: Los Alamos National Laboratory. 2010; Report no. LA-UR-10-03927.
- Nagatani T, Sagara H, Kosuge Y et al. Applicability of differential die-away self-interrogation technique for quantification of spontaneous fission nuclides for fuel debris at Fukushima Daiichi nuclear power plants. J Nucl Sci Technol. 2023;60(4):460–472. doi: 10.1080/00223131.2022.2114958
- Katakura J, JENDL FP decay data file 2011 and fission yields data file 2011. JAEA-Data/Code 2011-025. Mar 2012
- Naito Y, Azekura K. Criticality detection method based on fission product gamma radioactivity measurement. Nucl Technol. 2018;204(1):94–100. doi: 10.1080/00295450.2018.1469344
- Riyana ES, Okumura K, Sakamoto M et al. Depletion calculation of subcritical system with consideration of spontaneous fission reaction. J Nucl Sci Technol. 2022;59(4):424–430. doi: 10.1080/00223131.2021.1974970
- International research institute for nuclear decommissioning (IRID). Development of technology for containing, transfer and storage of fuel debris (FY2018 final research report). Japan: IRID; 2019.
- Bottomley PDW, Gregoire AC, Carbol P, et al. Fission product and actinide release from the debris bed test PHEBUS FPT4: synthesis of the post test analyses and of the revaporisation testing of the plenum samples. Nucl Eng Technol. 2006;38(2):163–174.
- Morimoto Y. Investigation of a realistic criticality risk assessment method. Human resource development forum. Japan: Tokyo Institute of Technology, 2018. in Japanese. 10 Jan.
- Okumura K, Riyana ES, Sato W, et al. A method for the prediction of the dose rate distribution in a primary containment vessel of the Fukushima Daiichi Nuclear Power Station. Prog Nucl Sci Technol. 2019;6:108–112. doi: 10.15669/pnst.6.108
- Sakamoto M, Okumura K, Lee K, et al. Detector light response modeling for a thick continuous slab detector. J Nucl Sci Technol. 2008;45(7):634–638. to be submitted. doi: 10.3327/jnst.45.634