Abstract
The relativistic NMR module of the Amsterdam Density Functional (ADF) package, which is frequently utilised in studies of heavy atom NMR chemical shifts, is extended to calculate a hitherto neglected term from the response of the exchange-correlation (XC) potential. The term vanishes in the absence of spin-orbit coupling. Further, corrections to the shielding arising from scaling factors in the zeroth-order regular approximation (zora) relativistic framework are investigated. The XC response markedly improves calculated proton chemical shifts for hydrogen halides. Mercury chemical shifts for mercury dihalides are also noticeably altered. Contributions from density-gradient dependent terms in the response kernel contribute about 30–40%. New fully relativistic density functional theory (DFT) benchmark data are compared with zora and literature reference values. In line with previous work, it is found that absolute shielding constants for Hg are not accurately predicted with zora. However, chemical shifts agree well with fully relativistic calculations. The application of ‘scaled-zora’ scaling factors deteriorates the shielding constants and is therefore not recommended. The scaling hardly affects chemical shifts. zora calculations are not suitable for absolute shielding of heavy atoms but they can be used safely for chemical shifts in most application scenarios.
Acknowledgements
This research was supported by the US Department of Energy, Office of Basic Energy Sciences, Heavy Element Chemistry program, under grant DE-FG02-09ER16066. The author thanks the Center for Computational Research (CCR) at the University at Buffalo for providing computational resources and Vaida Arcisauskaite (University of Copenhagen) for sharing ADF input settings and geometries for the Hg systems to facilitate the comparison with data from [Citation38]. The author thanks Professor Trond Saue (Toulouse) for providing a developer’s version of the Dirac programme for the 4c GIAO NMR calculations, and Mr. Ben Pritchard (Buffalo) for help with the calculations.
Notes
aCalculations with different functionals. Present work: un-scaled zora (Z) and 4c (D = Dirac) DFT calculations excluding and including (+f XC ) the contribution from the DFT part of the XC response kernel.
Figure 1 Left: Difference between calculated and experimental proton shielding for the hydrogen halide (HX) series: present zora calculations incl. f XC and selected literature data from Table . Right: Calculated versus experimental proton shifts for the HX series. zora PBE/50 with and without (‘no f XC ’) DFT contributions from the exchange-correlation response kernel
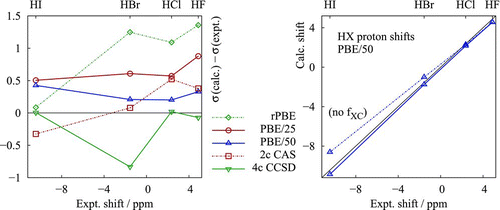
b4c DFT with BP functional (Becke-88/Perdew-86), XC kernel evaluated by numerical differentiation [Citation29].
c2c complete active space SCF (CASSCF) data from [Citation73].
d4c coupled-cluster singles and doubles CCSD data from [Citation74].
eExperimental data for HCl, HBr, and HI from [Citation75]. Experimental data for HF converted from experimental chemical shift difference between HBr and HF as given in [Citation76] and the experimental HBr shielding and shift from [Citation75]. A proton shielding for TMS of 33.485 ppm from [Citation75] was used to calculate chemical shifts.
aCalculations with different functionals. Present work: un-scaled zora (Z) and 4c (D) DFT calculations excluding and including (+f XC ) the contribution from the DFT part of the XC response kernel. See also footnotes in Table .
b4c DFT with BP functional (Becke-88/Perdew-86), XC kernel evaluated by numerical differentiation [Citation29].
aCalculations with different functionals. Present work: un-scaled zora (Z) and 4c (D) DFT calculations excluding and including (+f XC ) the contributions from the DFT part of the XC response kernel.
brPBE response kernel.
cPBE/25 calculation using only the LDA part of the XC response kernel.
d4-component DFT data from [Citation38].