ABSTRACT
The complex hymenophore configuration of the oak mazegill (Daedalea quercina, Polyporales) is rarely quantified, although quantifications are important analytical tools to assess form and growth. We quantified the hymenophore configuration of the oak mazegill by manual counting of tubes and tubular branches and ends. Complementary measurements were made with the software AngioTool. We found that the number of tubular branches and ends varied substantially between specimens, with a positive correlation with hymenophore area (5–51 cm2). We then measured complexity as tubular branches and ends per area, and complexity was not correlated with the size of the basidiocarps. Basidiocarps from two locations were compared (Hald ege, N = 11; Hvidding krat, N = 7), and the prevalence of branches and that of ends were greater in the Hvidding krat hymenophores (P < 0.001 and P = 0.029, respectively). Additionally, lacunarity, a measure of complexity (“gappiness”), gave a higher score for the Hald ege hymenophores (P = 0.002). Lacunarity analysis of multiple species of Polyporales showed that the oak mazegill hymenophore is comparatively complex. Concerning factors that affect hymenophore complexity of the oak mazegill, we observed that greater hymenophore complexity was associated with abrupt boundaries between growth zones on the pileus surface. Several years of monitoring documented that basidiocarps can remodel to gravitational changes and heal from damage. In conclusion, intra- and interspecies differences of hymenophore configuration can be quantified. In oak mazegill, hymenophore complexity is not dependent on size per se, although abrupt borders between growth zones are associated with increased complexity. Some of the variation between basidiocarps may reflect aspects of the ecology of the individual fungus.
INTRODUCTION
The basidiocarp of the oak mazegill (Daedalea quercina) has been a source of tinder since the stone ages (Berihuete-Azorín et al. Citation2018), and its hymenophore has such an aesthetic configuration that it invokes the appearance of the maze of Daedalus, which hosted the Minotaur (). The oak mazegill belongs to the species-rich order Polyporales in which the macroscopic configuration of the hymenophore is typically tubular but can be lamellate, and in the oak mazegill these features coexist in the daedaleoid configuration (Linder et al. 2011; Clémençon Citation2012; Binder et al. Citation2013; Hibbett et al. Citation2014). Although the oak mazegill hymenophore has tubes that can be lamellate, these exhibit conspicuously less radial symmetry than found in most other species with lamellate hymenophores (Rathod Citation2011; Clémençon Citation2012; Læssøe and Petersen Citation2019). The loss of radial symmetry is in part due to branches between tubes, and the term daedaleoid refers to hymenophores that combines features of tubular and lamellate configuration () (Clémençon Citation2012; Hibbett et al. Citation2014).
Figure 1. The oak mazegill basidiocarp, growing on the trunk of a fallen oak, was cut into left and right halves to show the presence of growth zones on the pileus surface, the daedaleoid configuration of the hymenophore, and hymenophore zones with a particularly complex configuration. The perception of complexity comes from the loss of radial symmetry by a mixed tubular and lamellate configuration of the hymenophore and by a high prevalence of branches and ends to the lamellate tubes (see insert)
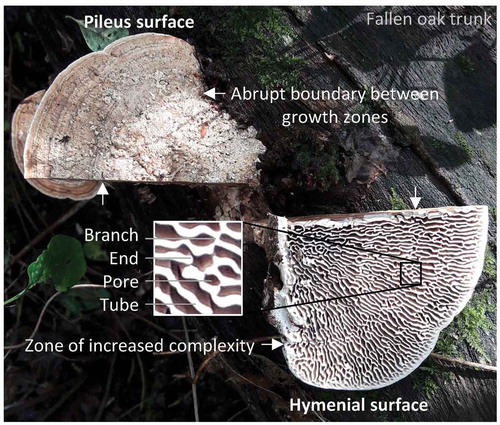
Basidiocarps of the oak mazegill can grow to some 20 cm in width (Linder et al. Citation2011; Læssøe and Petersen Citation2019). Large basidiocarps appear, to the naked eye, to be more complex than small basidiocarps (i.e., the navigation of the maze from base to margin takes more time in large basidiocarps). No previous attempt, to the best of our knowledge, has been made to quantify the daedaleoid configuration beyond the size of tubes and pores (Linder et al. Citation2011; Rathod Citation2011), although quantifications and analyses thereof are descriptive tools that may reveal otherwise obscure correlations and causations of growth (Gould Citation1966; Marshall et al. Citation2019). Thus, it is not clear whether differences in perceived complexity reflect a change in number of structures per area, or just more structures, and whether the prevalence of some structures is affected by the biology of the fungi and the environment. A related question is whether the hymenophore configuration as seen at the tubular crests (closest to the ground) corresponds to the hymenophore configuration at the base (closest to the pileus surface). While it is known that the hymenophore varies between basidiocarps (Schanzle Citation1973), it is not clear whether the hymenophore complexity is different between individuals from different locations. Therefore, although the success of molecular techniques in establishing phylogenetic relations is evident (Pegler Citation1973; Justo and Hibbert Citation2011; Lindner et al. Citation2011; Rathod Citation2011; Li and Cui Citation2013; Han et al. Citation2015; Das and Aminuzzaman Citation2017; Runnel et al. Citation2019), “morphology and anatomy” and “taxonomy and systematics” are complementary (Clémençon Citation2012) and we test multiple species of Polyporales for morphometric species differences in hymenophore configuration.
In this study, we aimed to use quantitative approaches, or morphometrics, to understand the configuration of the oak mazegill hymenophore and to identify some of the factors that may impact on the configuration. Morphometrics are commonly used as analytical tools to reveal biological and physical processes (e.g., Milinkovitch et al. Citation2013). The principle approach was to count tubular branches and ends along lines of inspection. Lines of inspection were made by successive shrinkage of the basidiocarp margin toward the base. Oak mazegill basidiocarps often have “indistinct annual zones” on the pileus surface (Lindner et al. Citation2011), “furrows” that separate “different periods of growth” (Ames Citation1913). We will refer to these as “growth zones,” and the lines of inspection had the added value of approximating the position of the pileus growth zones on the hymenophore (). As will be shown, there may be a relation between zonation on the pileus surface and complexity on the hymenophore.
The approach taken here is essentially unbiased with regard to where counting is performed. It is adopted from quantifications of the concentration of enzymes in liver lobules along so-called portocentral radii (Lamers et al. Citation1997; Christoffels et al. Citation1999). Secondly, for the same purposes, we used AngioTool, a freeware that was developed to quantify the organization of networks of blood vessels (Zudaire et al. Citation2011). The readouts include the counting of branches (“nodal points”) and ends and additionally give a score of lacunarity, which is used as a measure of complexity or “gappiness.” When the configuration of the hymenophore is daedeleoid, the particular configuration is highly variable between basidiocarps, possibly due to a high sensitivity to environmental or physicochemical cues (Lindner et al. Citation2011). We address the sensitivity to physical cues, specifically to damages that were experimentally induced or naturally occurring and to changes in orientation with respect to the ground (geotropic response). Therefore, the primary aim of this study was to develop and test approaches to quantify hymenophore configuration complexity in the oak mazegill. Our secondary aim was to use the morphometrics to assess species differences, differences within species (oak mazegill is used as a case), and the impact of physical cues as sources of hymenophore variation.
MATERIALS AND METHODS
Collection of basidiocarps.—
Basidiocarps of oak mazegill fungus (Daedalea quercina) were collected on two locations. The first location was approximately 4 hectares around latitude 56.408N and longitude 9.363E in a protected oak forest (Hald ege, Denmark). Quantifications were performed on 12 basidiocarps referred to as samples He01–He12 that were collected on 3 Dec 2016. They were collected from three fallen oak tree trunks (we did not catalog the exact location of the trunks). We sampled an additional 10 large basidiocarps of oak mazegill (approximately 10 cm in width) on 5 Dec 2016 to test whether conspicuous growth zone borders on the pileus surface of the basidiocarp are associated with changes in tubular complexity. In both collections, horizontally oriented, dimidiate rather than corticioid (Clémençon Citation2012), and solitary basidiocarps were favored, as they were deemed the least complex on which to perform measurements. We did not record temperature, humidity, or soil pH. As we were interested in the possible relation between basidiocarp size and its complexity, we sought to collect (nonrandom) a broad range of sizes.
The second location was approximately 8 hectares around latitude 56.543N and longitude 9.763E (Hvidding krat, Denmark) in a privately managed oak forest. Collection took place on 28 Apr 2018. We collected eight basidiocarps from three different stumps (sawn) of oak trees. These are referred to as samples HkXXXXXX (Hvidding krat-hour-minute-second of photography). Horizontally oriented and solitary basidiocarps were favored again. We did not record temperature, humidity, or soil pH, and we sought to collect (nonrandom) a range of sizes that was comparable to that of the Hald ege basidiocarps.
Magnetic resonance imaging (MRI).—
To investigate the full depth of the hymenophore configuration, one specimen (Hk115825) was imaged on a small-animal 7-T MRI scanner (MR Solutions, Guilford, UK) at an isotropic resolution of 0.5 mm using a three-dimensional (3D) gradient echo sequence.
DICOM files were imported to Amira 5.6 (FEI, Thermo Fisher Scientific, Boston, Massachusetts). Tissue was selected on the basis of thresholding and added to a single label. Excessive labeling (false positive) was removed manually. After surface generation, the resultant surface was smoothened five times at a lambda of 0.6 and constraints set to “none.” Cuts were made with the SurfaceCut module. The 3D model was imaged using Amira’s Snapshot function.
Assessment of tube configuration and complexity by AngioTool.—
Images imported to AngioTool (Zudaire et al. Citation2011) can be analyzed to give readouts on morphometrics including area, average structure length, and number of nodal points and ends, and additionally a fractal-based score of complexity called lacunarity (Plotnick et al. Citation1996). We analyzed lacunarity in downloaded photographs of hymenophores of 10 different species of Polyporales from MycoKey 4.1 (www.mycokey.com) as a comparison with lacunarity measured in the oak mazegills. From MycoKey, the following photographs were analyzed (photograph ID Genus species): JHP-99.492 Bjerkandera adusta; JHP-05.240 Trametes gibbosa; JHP-02.158 Fomitopsis pinicola; JHP-99.313 Cerrena unicolor; JHP-09.264 Daedaleopsis confragosa; JHP-99.380 Piptoporus betulinus; JHP-99.527 Aurantioporus alborubescens; JHP-92.383 Spongipellis delectans; JHP-99.534 Grifola frondosa; and JHP-99.506 Phaeolus schweinitzii. We further analyzed the oak mazegills from the two locations with the morphometrics of AngioTool. There was a good correspondence in size of the basidiocarps from the two locations; only He02 was much smaller than any of the Hvidding krat basidiocarps, and it was excluded from the comparison between the two locations. From each location, morphometrics were related to area with Spearman correlation tests, and the means of the morphometrics between locations were compared using independent-samples t-tests (IBM SPSS version 24; IBM, Armonk, New York). P values below 0.05 were considered significant.
Manual assessment of tubular configuration complexity.—
Lines of inspection were placed as nine times 10% downscaled outlines of the basidiocarp margin (). We settled on nine times 10% downscaled outlines because that offered sufficient spatial resolution for the dual purpose of assessing overall complexity and to determine whether the abrupt borders on the pileus surface are related to differences in hymenophore complexity. The number of tubular branches and ends was counted along each 10% downscaling (from 10% to 90%) within a 90° pie of the basidiocarp (). Complexity of tubular configuration was expressed as a percentage derived from the sum of branches and ends divided by the total number of crossed structures, i.e., tubes, branches, and ends (). The complexity would be 0 if only tubes were crossed and no branches or ends were counted. Conversely, the complexity would be 100 if only branches and ends were counted. The complexity of one basidiocarp was expressed as the average complexity of all inspected lines. Only in specimens He04, He06, and He11 was the pie smaller than 90°, namely, 82°, 63°, and 76°, respectively, because beyond these bounds the tubular configuration appeared affected by neighboring basidiocarps.
Figure 2. Illustration of the manner of manual analysis of oak mazegill hymenophore complexity. Inspection lines 90 to 10 represent shrinkage of the basidiocarp margin from 90% to 10% of the margin, and they were placed at equidistance between the margin and the base from which the basidiocarp emerges from the wood. Tubes and tubular branches and ends were counted along each inspection line within a quarter-circle area. The framed area of the hymenophore around line 40 is schematized at the bottom of the figure to illustrate that only structures on inspection lines, or in the immediate vicinity thereof, were counted. Counted structures are indicated by a red circle. The schematized area contained several branches and ends that were not counted (dashed circles). The complexity score for inspection line 40 in the shown area is 40% [(1 branch + 1 end)/(3 lamellate tubes + 1 branch + 1 end) × 100]
![Figure 2. Illustration of the manner of manual analysis of oak mazegill hymenophore complexity. Inspection lines 90 to 10 represent shrinkage of the basidiocarp margin from 90% to 10% of the margin, and they were placed at equidistance between the margin and the base from which the basidiocarp emerges from the wood. Tubes and tubular branches and ends were counted along each inspection line within a quarter-circle area. The framed area of the hymenophore around line 40 is schematized at the bottom of the figure to illustrate that only structures on inspection lines, or in the immediate vicinity thereof, were counted. Counted structures are indicated by a red circle. The schematized area contained several branches and ends that were not counted (dashed circles). The complexity score for inspection line 40 in the shown area is 40% [(1 branch + 1 end)/(3 lamellate tubes + 1 branch + 1 end) × 100]](/cms/asset/34e29b26-9471-4edd-b4e8-e2fb5fb6c86d/umyc_a_1785197_f0002_oc.jpg)
Relation of zone abrupt borders and tubular complexity.—
Two nails were inserted through the most conspicuous border on the pileus surface of 10 basidiocarps, and complexity was then counted in lines through the emerging nails and neighboring lines.
Response to experimental damage.—
We experimentally damaged six large basidiocarps of oak mazegill (approximately 5–10 cm in width) from Hald ege on 3 Sep 2017 and monitored these a few times a year until Dec 2019. In four basidiocarps, we cut away approximately half of the basidiocarp, in the fifth we cut away a 1-cm-wide stripe of the hymenophore but left the pileus intact, and in the sixth we made a single deep gash from the margin at a skew angle to the radial symmetry.
RESULTS
Constant vertical configuration of the hymenophore.—
shows the hymenophore of the just-collected specimen Hk115825. Most of the hymenophore in this specimen was configured as lamellate tubes, but locally the lamellate tubes took the shape of pillars (tubes that are much deeper than transverse wide and base-margin long). Three examples are indicated by arrowheads in . In the MRI-generated image series of Hk115825, finer structures such as the pillars were detected (). On the basis of the MRI series, we generated a 3D model (compare with ). The level of correspondence to the actual hymenophore was such that, for example, the three small pillars indicated with red arrowheads in were reconstructed (). We then removed the front half of the 3D model, and when this cut plane was inspected, it was seen that most tubes appeared unbranched. This indicated that the configuration of the tubes will be similar at their crest (the part closest to the ground) and at their base (the part that is closest to the pileus layer). Similar observations can be made when light is shown between the tubes, i.e., the pore spaces are rarely crossed by partial dissepiments. The relation between dissepiments is then approximately similar at any depth of the hymenophore, and the crests of the tubes are considered to represent the overall configuration of the hymenophore.
Figure 3. Vertical configuration of the hymenophore of oak mazegill. A. Hymenophore surface of specimen Hk115825. B. One image of the MRI-generated series of 64 images in the horizontal plane. Notice that the spatial resolution is sufficient to detect small macroscopic features such as 3 pillars (red arrowheads; compare A and B). C. The MRI-based 3D model corresponds well to the physical specimen, since it shows, for example, the 3 pillars (red arrowheads; compare A and C). It can be seen that there are few structures that traverse the pore space. In the cut plane, most tubes appear as pillars (an example is indicated by the dashed oval). Consequently, the relation between tubes is approximately the same at any depth of the hymenophore
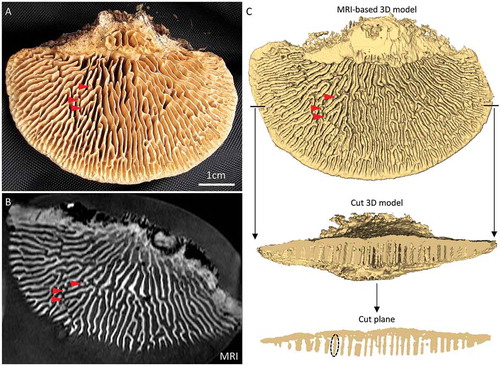
Analyses of hymenophore configuration using AngioTool.—
The readouts of the AngioTool analyses and the statistical tests thereof are listed in . In the collections from both locations, the number of tubular branches (junctions in AngioTool) and that of free ends were significantly and positively correlated with area. In contrast, the number of branches and that of ends per area, or the prevalence of these, were not related to area in either collection. We found significant differences between the collections, in that the Hvidding krat collection had a higher prevalence of both branches (40 vs. 22 per cm2, P < 0.001) and ends (14 vs. 9 per cm2, P = 0.029).
Table 1. AngioTool-derived morphometric and spatial parameters of Oak mazegill fruit bodies from Hald ege (HeXX, N = 11) and Hvidding krat (HkXXXXXX, N = 7)
Irrespective of the lower prevalence of branches and ends, the Hald ege collection had a greater lacunarity score than the Hvidding krat collection (0.15 vs. 0.11, P = 0.027). Lacunarity was not related to prevalence of branches and ends in either collection. From the publication of AngioTool, it is not clear how lacunarity is calculated, so we scored the lacunarity of species with hymenophores with quite different configurations (). Hymenophores with predominantly poroid (e.g., Fomitopsis pinicola) or lamellate (e.g., Daedaleopsis confragosa) configuration had low lacunarity, whereas hymenophores that combined poroid and lamellate features had higher scores, the oak mazegills in particular (). To further test the relation between hymenophore configuration and lacunarity, we scored the lacunarity of six MRI-generated images spaced at a fixed distance along the depth of the hymenophore (). Because the hymenophore configuration is essentially constant at any depth (), most of the difference in lacunarity between the images of is due to the decrement of the hymenophore size and margin as the section plane changes from close to the pileus surface (Sec60) to closer to the ground (Sec80). The lacunarity score varied almost as much as in , and although the two smallest sections scored the lowest lacunarity, it was an intermediate-sized section (Sec72) that had the highest lacunarity ().
Figure 4. AngioTool-derived lacunarity scores of hymenophore configuration of multiple species of Polyporales, showing the oak mazegill to have a relatively complex configuration when compared with the hymenophores of 10 other species within Polyporales (all images, except those of oak mazegill, are adapted from images from MycoKey)
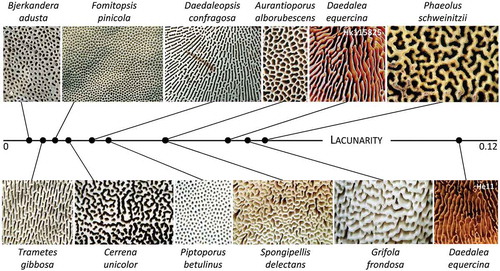
Figure 5. The AngioTool-derived lacunarity score is a compound measurement of configuration, size, and computations at the hymenophore margin. Lacunarity scores of MR images from different depths of one hymenophore (specimen Hk115825), from closest to the pileus surface (Sec60) to closest to the ground (Sec80). There is not a linear relation between lacunarity score and depth, despite a general conservation of the hymenophore configuration at the different depths (one example of a consistent feature is indicated by the red arrowhead). If the same region is measured for each depth (right-hand column), the lacunarity scores are essentially similar. Differences in lacunarity score of the total hymenophore (left-hand column) may then reflect size and computations at the margin of the hymenophore
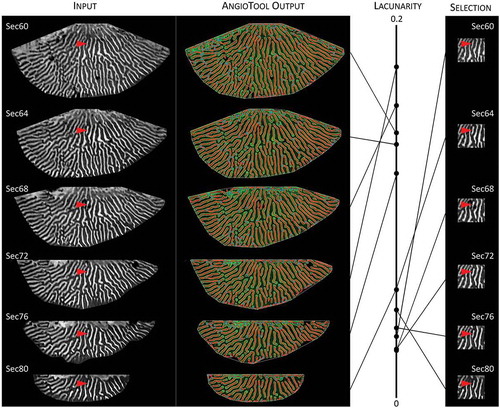
Manual analysis reveals zones of high complexity.—
shows the hymenophore of a large oak mazegill basidiocarp, and shows the same photograph with indications of all recognized tubular branches and ends. Within this hymenophore, there appeared to be three zones with approximately the same outline as the margin of the basidiocarp, i.e., semicircle-like, that were characterized by a high prevalence of branches and ends, or high complexity. We then reasoned that a manual analysis along lines of inspection that represent shrunken margins, as illustrated in , could serve the dual purpose of assessing the overall prevalence of lamellate tubes, branches, and ends to complement the AngioTool analyses, and it could additionally be used to identify zones of high complexity.
Figure 6. Manual analyses of hymenophore complexity of oak mazegill basidiocarp. A. A large horizontal basidiocarp with considerable overall radial symmetry to the hymenophore configuration and with at least three zones of greater complexity. B. Every branching on the basidiocarp of A is indicated by a red dot, and every free ending by a white dot. C. The number of transitions along the lines of inspection was strongly correlated with area (P < 0.001). D. Relative complexity in all 12 specimens was not related to the axis from base (0) to margin (10). E. A basidiocarp where nails have been inserted through the upper surface of a particularly pronounced border of growth rings, showing the nails to emerge in a zone with a line of inspection with relative great complexity (42%) compared with the proximal and distal lines of inspection, 6% and 21%, respectively. F. A basidiocarp, where the nails emerge in a line of inspection that coincides within the transition from low to great complexity
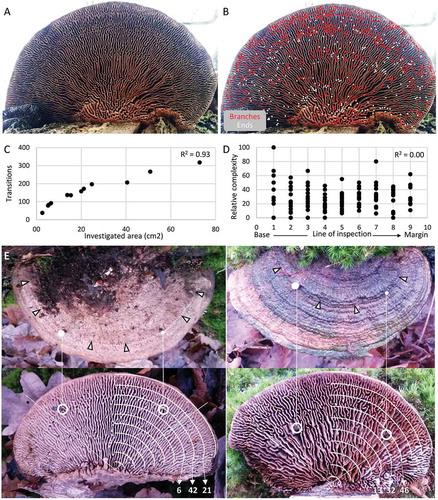
lists morphometrics for the specimens collected in Hald ege that were analyzed manually. shows that the number of transitions (lamellate tubes, branches, and ends) was highly correlated with the investigated area (P < 0.001). In all specimens, a total of 379 branches and 209 ends were counted. In 10 out of 12 basidiocarps, branches were more frequent than ends, but in specimens He09 and He11 ends were more frequent than branches and accounted for 67.1% and 69.6%, respectively, of all features contributing to complexity (). Across all inspected lines of the 12 specimens, neither preponderance of ends or that of branches was related to greater complexity (P = 0.353 and P = 0.087, respectively). For all investigated lines in all 12 specimens, relative complexity was 31.5 ± 19.3 (average ± standard deviation) and the number of branches and ends relative to transitions (i.e., complexity) was not related to the line of inspection, i.e., to the distance from the wooden trunk to the margin of the basidiocarp (). Also, there was no correlation between total complexity of the investigated area and the size of that area (P = 0.706). Any one line of inspection therefore revealed, on average, that once 10 tubes had been inspected, two would have exhibited branches and one would have displayed an end. This suggests that on average any two neighboring tubes would have a very similar distance to one another. For most basidiocarps, the distance between any two tubes would be approximately 0.23 cm (). These findings complement those of the AngioTool analyses () and further indicate that hymenophore complexity does not increase along the base-margin distance.
Table 2. Morphometric and spatial parameters of the 12 manually analysed oak mazegill fruit bodies collected from Hald ege (specimens He01 and He03-He12 were also analyzed with AngioTool; TABLE 1)
In large horizontal basidiocarps in particular, many hymenophores exhibited a few zones of greater complexity, and such zones could be identified by lines of inspection with a high relative prevalence of junctions and ends (–B). These complex zones in particular give the hymenophore the configuration of a maze. In smaller basidiocarps, there were fewer complex zones. Most often, these zones co-localized with particularly abrupt borders between growth rings on the pileus surface (). Occasionally, an abrupt border on the pileus surface could co-localize with a persistent change in hymenophore configuration (). These data suggest that the hymenophore configuration is not only programmed but also responsive to the rate of growth.
Oak mazegill basidiocarps may remodel to geotropic orientation and damage.—
On most basidiocarps of oak mazegill, the hymenophore is facing the ground, presumably because the tubes grow geotropically. It is not unusual to find on partially fallen or ground-fallen trunks, however, basidiocarps at skew angles with respect to each other (). In some cases, it even appears that basidiocarps can remodel such that the hymenophore becomes overgrown by a new pileus surface and what was the pileus surface becomes overgrown by a new hymenophore (). The new pileus does not have obvious growth rings ().
Figure 7. The oak mazegill basidiocarp has capacity to remodel. A. An oak trunk in Hald ege of which the top has fallen partially toward the ground and which has multiple basidiocarps of oak mazegill. (The white circle identifies the basidiocarp illustrated in – and –). B. A part of the top trunk shown in A has recently fallen to the ground, and this particular basidiocarp is developing a pileus surface on the old hymenophore. C. Compared with the basidiocarp in B and growing on the same trunk, this basidiocarp is further in the development of a new pileus surface on the old hymenophore (whereas the neighboring basidiocarps are unresponsive). D. The ground-facing side of the basidiocarp shown in C, showing a well-developed new hymenophore on the old pileus. E. An oak mazegill basidiocarp growing on a ground-lying trunk of oak in a different location in Hald ege from A–D. This basidiocarp was cut along the dashed black lines in Sep 2017. F. The basidiocarp of E after the cutting. G. The basidiocarp of E, 2 years later, showing pronounced growth along the intact margin and the cut surface of the basidiocarp, but not at the stem of the basidiocarp. H. This oak mazegill basidiocarp was cut also in Sep 2017 and is much like the basidiocarp in E–G but exhibits only faint new growth on the cut surface (found on a ground-lying trunk of oak in a different location in Hald ege from E–G)
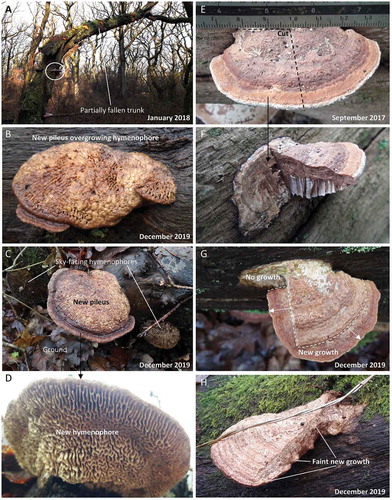
Next, we experimentally damaged six basidiocarps and photographed them at multiple times during a 2-year period to test whether growth can occur from damaged parts. In four of these, chunks of the basidiocarps were cut off. One of these exhibited sustained growth, even out of the cut parts (), and a second grew a thin layer on the cut surface (), whereas the remaining three did not showed any obvious growth on the cut surface. It was not clear whether the latter ones were alive and unresponsive, or dead. The sixth basidiocarp received a single deep cut but nothing was removed, and over time the cut became partially filled with tissue (). A seventh basidiocarp was incidentally noted to have a gash of unknown origin near its margin (). Its gash became overgrown with tissue, and this tissue stood out at a skew angle to the overall radial configuration. Over the course of 2 years, the gash-filling tissue remodeled such that its thickness became like that of the surrounding tubes while the skew angle could still be made out (). Taken together, these observations suggest that the hymenophore configuration is not only programmed and responsive to the rate of growth, but also responsive to externalities such as physical damage and orientation relative to the ground.
Figure 8. The damaged oak mazegill basidiocarp has capacity to heal. A. Basidiocarp with experimentally given gash. B. Two years after A, the gash has been filled with tissue that resembles the surrounding tubes, i.e., the gash has healed, except that some of the skewed orientation with respect to the overall radial symmetry is still visible and the hymenophore therefore has not regenerated. C. This basidiocarp was found to have a gash, the cause of which is unknown, near its margin. D. Less than a year after C, the gash appears to have been overgrown with new context. E. Two years after D, the tissue that filled the gash has remodeled to resemble the surrounding tubes, the gash has healed, except that the new context retains the skewed orientation of the gash with respect to the over radial symmetry and the hymenophore therefore has not regenerated
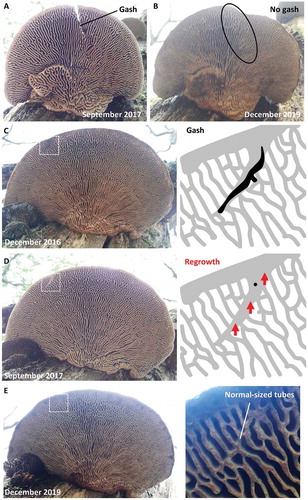
DISCUSSION
The primary aim of this study was to develop morphometric analyses of the hymenophore configuration of the oak mazegill, and we show with such analyses that it is possible to discriminate between basidiocarps from different locations and from different species. Hymenophore configuration can be analyzed much faster by freeware such as AngioTool than by hand. Effectively this means that the use of automated analyses, for example, by AngioTool, allows for a greater number of hymenophores to be analyzed in one project. The output from AngioTool is sensitive to changes to the settings of parameters before the computation is done (Zudaire et al. Citation2011), and, as we show here, it is also sensitive to the size of the images or the area that is being measured. It is then important to use standardized images, including bringing them to the same scale before the computation. One convenient feature of AngioTool is that it gives a visual representation of the computation of the tubes, branching points, and ends, and these computations can then be verified by eye. In contrast, the fractal-based score of lacunarity is not visually presented, and lacunarity, in our experience, is a difficult readout to make use of. In fact, there may be a discrepancy in increments in lacunarity and perceived complexity to the naked eye, and we therefore encourage readers to consult fig. 10 of Smith et al. (Citation1996), which gives examples of structures with lacunarity between 0 and 0.5.
When addressing the primary aim of the study, we hoped to be able to articulate which features make the oak mazegill hymenophore complex. Firstly, the relative complexity, or relative prevalence of tubular branches and ends, was independent of the investigated area. In other polypores, Leiotrametes menziesii, for instance, the configuration of the hymenophore is dependent on size and appears to progress from poroid to lamellate with age (Welti et al. Citation2012). Secondly, there is an essentially fixed distance between tubes. The number of tubes per distance in the oak mazegill fungus has previously been estimated in specimens collected in India (Rathod Citation2011). The estimate reported by Rathod (Citation2011), 6–7 tubes per centimeter, reveals a somewhat denser configuration than the approximately 5 tubes per centimeter measured here, but both estimates are within the range previously given (pores are “mostly 1–4 mm wide measured tangentially” and “walls 1–3 mm thick” (Lindner et al. Citation2011). Thirdly, the relative complexity does not change with distance from basidiocarp base to margin. We conclude that if bigger basidiocarps appear more complex, it is primarily because there is more of the complex structure, not that the structure is more complex.
We document through observations and experimental damaging that the oak mazegill basidiocarp may undergo pronounced changes in response to orientation relative to the ground, growth rate, and damage. Also, the oak mazegill, like other polypores, may overgrow obstacles such as twigs (Clémençon Citation2012). The sensitivity of morphometric comparisons, then, will likely be greatest when basidiocarps are compared that show a minimum of signs of having responded to such externalities and care is taken to use standardized images. By analogy to medical sciences, this corresponds to strive for case-control studies of healthy individuals of different populations. The daedaleoid configuration of the hymenophore varies between species and may additionally reflect phylogeny (Seelan et al. Citation2015; Runnel et al. Citation2019). It remains to be shown whether quantifications, such as the ones used here, could make analysis of hymenophore configuration a more powerful complement to molecularly inferred phylogenetics. Pore size must reflect to some extent the compromise between maximal hymenial surface and the distance covered by the spore when released (Clémençon Citation2012). Also, pores likely have a microenvironment (airflow and humidity) that can be different from the air immediately beneath the hymenophore. Such factors, and possibly numerous additional ones (e.g., Butler and Wood Citation1988), likely drive the hymenophore configuration. In phylogenetically distant polypores, the sum of these factors may attain a similar value, although the magnitude of each factor (e.g., force of spore release) is dissimilar in the compared species. What is clear, at least, is that similar hymenophore configurations have evolved by convergence multiple times (Butler and Wood 1982; Clémençon Citation2012).
Morphometric scoring and modeling has been used to assess aging and desiccation of pilei of Agaricus bisporus (Braaksma et al. Citation1998; González-Pérez et al. Citation2019), but we do not know of studies that quantify hymenophore complexity. Complexity is often quantified by fractal analysis of, for example, soil (Torre et al. Citation2018), bone (Doube et al. Citation2010), heart structure (Captur et al. Citation2015), and epidermal netting of the muskmelon (Li et al. Citation2012), which has some resemblance to lamellate tubes. Our scoring of hymenophore complexity, both manual and automated, showed substantial variation between basidiocarps, and the hymenophore configuration is also highly variable in other species of Daedalea (Lindner et al. Citation2011) and in some other genera of Polyporales (Welti et al. Citation2012; Hibbett et al. Citation2014).
In the oak mazegill, the hymenophore is mostly poroid at the margin of the basidiocarp, as in other polypores (Corner Citation1932; Clémençon Citation2012). For most of the distance between the margin and the base, however, the tubes are predominantly lamellate in a radial orientation. This suggests that the lamellate configuration grows out of cylindrical dissepiments. In the analysis of Ames (Citation1913), radially oriented dissepiments (“walls”) grow the most and the transverse dissepiments grow only when “branches” form. At least in some polypores, the cylindrical dissepiments are patterned in the “pore field,” a zone immediately behind the margin, which is without dissepiments and is several pore widths deep (Corner Citation1932; Clémençon Citation2012). Concerning the oak mazegill, however, there is no obvious pore field and certainly not one that is several cylindrical pore widths deep. Also, it is remarkable that the flipped basidiocarps can grow a new (largely poroid) hymenophore on the old pileus surface. For the oak mazegill, this strongly suggests that the hymenophore configuration can be patterned outside the pore field. A test of this conjecture, which we have not performed, could be to flip basidiocarps and assess the extent to which the configuration of the old hymenophore is replicated in the new hymenophore growing through the old pileus.
We show that hymenophore zones of relatively high complexity are associated with abrupt borders between growth zones on the pileus. Complexity of the hymenophore configuration therefore may not be an entirely stochastic phenomenon and may reflect rate of growth and, like in other fungi, how well the fungus is established in its substrate and the physical features of the environment such as temperature, humidity, and light (Kauserud et al. Citation2008; Büntgen et al. Citation2013; Kües and Navarro-González Citation2015; Andrew et al. Citation2016; Halbwachs et al. Citation2016; Pelkmans et al. Citation2016). Whether these zonal increases in hymenophore complexity are adaptive, for instance leading to a greater and farther spore release, remains to be shown. The lamellate, or gill-like, configuration is likely to have a greater hymenial surface than a tubular or hydnoid configuration (Clémençon Citation2012), and it may be that the zonal increases in hymenophore complexity are associated with a reduction in hymenial surface area. Further impacting on the hymenophore configuration is the capacity of the oak mazegill basidiocarps, like many other polypores, to grow in clusters, incorporate obstacles, adapt to changes in gravity (displacements), and heal from damage (Butler and Wood 1982; Clémençon Citation2012). Although this makes for a bewildering list of determinants of hymenophore configuration, many of these factors can be controlled for by focusing on relatively simple basidiocarps and by monitoring over periods of time, as we have attempted here. More importantly, because the oak mazegill basidiocarp is perennial and the configuration of its hymenophore appears exceptionally sensitive, it may be one of the best candidates for a fungal bioindicator with a multiyear record.
CONCLUSION
This study focused on basidiocarps of the oak mazegill that were perceived as being relatively simple, i.e., oriented close to the horizontal plane and with little or no fusion with other basidiocarps. On the basis of the studied basidiocarps and the morphometrics we adapted, it is clear that the incidence of tubular branches and ends, or complexity, is similar across a wide range of basidiocarp sizes. One important qualification of this conclusion is that the number of growth zones increases with the size of the basidiocarp and that abrupt borders between growth zones are associated with increased hymenophore complexity. The impact of growth on hymenophore configuration gives the oak mazegill basidiocarp the potential to be a bioindicator with a multiyear record. This perspective corroborates the value of morphometric studies beyond the use for the intra- and interspecies characterizations we document here.
ACKNOWLEDGMENTS
We thank Jan M. Ruijter for suggesting the use of multiple lines of inspection and Jens H. Petersen for critiquing an earlier version of the manuscript. We owe a special thanks to the residents of Nonbo hede 6 for facilitating this study.
LITERATURE CITED
- Ames A. 1913. A consideration of structure in relation to genera of the Polyporaceae. Annales Mycologici 11:211–253.
- Andrew C, Heegaard E, Halvorsen R, Martinez-Peña F, Egli S, Kirk PM, Bässler C, Büntgen U, Aldea J, Høiland K, Boddy L. 2016. Climate impacts on fungal community and trait dynamics. Fungal Ecology 22:17–25.
- Berihuete-Azorín M, Girbal J, Piqué R, Palomo A, Terradas X. 2018. Punk’s not dead. Fungi for tinder at the Neolithic site of La Draga (NE Iberia). PLoS ONE 13:e0195846.
- Binder M, Justo A, Riley R, Salamov A, Lopez-Giraldez F, Sjökvist E, Copeland A, Foster B, Sun H, Larsson E, Larsson K-H, Townsend J, Grigoriev IV, Hibbett DS. 2013. Phylogenetic and phylogenomic overview of the Polyporales. Mycologia 105:1350–1373.
- Braaksma A, Van Doorn AA, Kieft H, Van Aelst AC. 1998. Morphometric analysis of ageing mushrooms (Agaricus bisporus) during postharvest development. Postharvest Biology and Technology 13:71–79.
- Butler GM, Wood AE. 1988. Effects of environmental factors on basidiome development in the resupinate polypore Phellinus contiguus. Transactions of the British Mycological Society 90:75–83.
- Büntgen U, Peter M, Kauserud H, Egli S. 2013. Unraveling environmental drivers of a recent increase in Swiss fungi fruiting. Global Change Biology 19:2785–2794.
- Captur G, Syrris P, Obianyo C, Limongelli G, Moon JC. 2015. Formation and malformation of cardiac trabeculae: biological basis, clinical significance, and special yield of magnetic resonance imaging in assessment. Canadian Journal of Cardiology 31:1325–1337.
- Christoffels VM, Sassi H, Ruijter JM, Moorman AFM, Grange T, Lamers WH. 1999. A mechanistic model for the development and maintenance of portocentral gradients in gene expression in the liver. Hepatology 29:1180–1192.
- Clémençon H. 2012. Cytology and plectology of the Hymenomycetes. 2nd rev. ed. Stuttgart, Germany: Gebrüder Borntraeger Verlagsbuchhandlung. 520 p.
- Corner EHJ. 1932. The fruit-body of Polystictus xanthopus Fr. Annals of Botany 46:71–111.
- Das K, Aminuzzaman FM. 2017. Morphological and ecological characterization of Xylotrophic fungi in mangrove forest regions of Bangladesh. Journal of Advances in Biology & Biotechnology 11:1–15.
- Doube M, Kłosowski MM, Arganda-Carreras I, Cordelières FP, Dougherty RP, Jackson JS, Schmid B, Hutchinson JR, Shefelbine SJ. 2010. BoneJ: free and extensible bone image analysis in ImageJ. Bone 47:1076–1079.
- González-Pérez JE, López-Méndez EM, Ochoa-Velasco CE, Ruiz-López II. 2019. Mass transfer and morphometric characteristics of fresh and osmodehydrated white mushroom pilei during convective drying. Journal of Food Engineering 262:181–188.
- Gould SJ. 1966. Allometry and size in ontogeny and phylogeny. Biological Reviews 41:587–638.
- Halbwachs H, Simmel J, Bässler C. 2016. Tales and mysteries of fungal fruiting: how morphological and physiological traits affect a pileate lifestyle. Fungal Biology Reviews 30:36–61.
- Han M-L, Vlasák J, Cui B-K. 2015. Daedalea americana sp. nov. (Polyporales, Basidiomycota) evidenced by morphological characters and phylogenetic analysis. Phytotaxa 204:277–286.
- Hibbett DS, Bauer R, Binder M, Giachini AJ, Hosaka K, Justo A, Larsson E, Larsson KH, Lawrey JD, Nagy LG, Nilsson RH, Weiss M, Thorn RG. 2014. Agaricomycetes. In: McLaughlin DJ, Spatafora JW, eds. Systematics and evolution. Berlin and Heidelberg, Germany: Springer-Verlag. p. 373–429
- Justo A, Hibbert DS. 2011. Phylogenetic classification of Trametes (Basidiomycota, Polyporales) based on a five-marker dataset. Taxon 60:1567–1583.
- Kauserud H, Stige LC, Vik JO, Økland RH, Høiland K, Stenseth NC. 2008. Mushroom fruiting and climate change. Proceedings of the National Academy of Sciences of the United States of America 105:3811–3814.
- Kües U, Navarro-González M. 2015. How do Agaricomycetes shape their fruiting bodies? 1. Morphological aspects of development. Fungal Biology Reviews 29:63–97.
- Lamers WH, Geerts WJ, Jonker A, Verbeek FJ, Wagenaar GT, Moorman AF. 1997. Quantitative graphical description of portocentral gradients in hepatic gene expression by image analysis. Hepatology 26:398–406.
- Li H-J, Cui B-K. 2013. Two new Daedalea species (Polyporales, Basidiomycota) from South China. Mycoscience 54:62–68.
- Li L, Chang L, Ke S, Huang D. 2012. Multifractal analysis and lacunarity analysis: a promising method for the automated assessment of muskmelon (Cucumis melo L.) epidermis netting. Computers and Electronics in Agriculture 88:72–84.
- Lindner DL, Ryvarden L, Baroni TJ. 2011. A new species of Daedalea (Basidiomycota) and a synopsis of core species in Daedalea sensu stricto. North American Fungi 6:1–12.
- Læssøe T, Petersen JH. 2019. Fungi of temperate Europe. Volumes 1–2. Princeton, New Jersey: Princeton University Press. 1715 p.
- Marshall AF, Bardua C, Gower DJ, Wilkinson M, Sherratt E, Goswami A. 2019. High-density three-dimensional morphometric analyses support conserved static (intraspecific) modularity in caecilian (Amphibia: Gymnophiona) crania. Biological Journal of the Linnean Society 126:721–742.
- Milinkovitch MC, Manukyan L, Debry A, Di-Poï N, Martin S, Singh D, Lambert D, Zwicker M. 2013. Crocodile head scales are not developmental units but emerge from physical cracking. Science 339:78–81.
- Pegler DN. 1973. The polypores: with keys to world genera and British species. Bulletin of the British Mycological Society 7:3–43.
- Pelkmans JF, Lugones LG, Wösten HAB. 2016. Fruiting body formation in basidiomycetes. In: Wendland J, ed. The Mycota, Volume I. Growth, differentiation and sexuality. Cham, Switzerland: Springer. p. 387–405
- Plotnick RE, Gardner RH, Hargrove WW, Prestegaard K, Perlmutter M. 1996. Lacunarity analysis: a general technique for the analysis of spatial patterns. Physical Review E 53:5461–5468.
- Rathod MM. 2011. Taxonomic studies on the daedaloid and hexagonoid polypores form the forest of Western Maharasta. Recent Research in Science and Technology 3:50–56.
- Runnel K, Spirin V, Miettinen O, Vlasák J, Dai YC, Ryvarden L, Larsson KH. 2019. Morphological plasticity in brown-rot fungi: Antrodia is redefined to encompass both poroid and corticioid species. Mycologia 111:871–883.
- Schanzle RW. 1973. Daedalea quercina forma trametea in Illinois. Mycologia 65:689–690.
- Seelan JSS, Justo A, Nagy LG, Grand EA, Redhead SA, Hibbett D. 2015. Phylogenetic relationships and morphological evolution in Lentinus, Polyporellus and Neofavolus, emphasizing southeastern Asian taxa. Mycologia 107:460–474.
- Smith TG Jr, Lange GD, Marks WB. 1996. Fractal methods and results in cellular morphology—dimensions, lacunarity and multifractals. Journal of Neuroscience Methods 69:123–136.
- Torre IG, Losada JC, Heck RJ, Tarquis AM. 2018. Multifractal analysis of 3D images of tillage soil. Geoderma 311:167–174.
- Welti S, Moreau P-A, Favel A, Courtecuisse R, Haon M, Navarro D, Taussac S, Lesage-Meessen L. 2012. Molecular phylogeny of Trametes and related genera, and description of a new genus Leiotrametes. Fungal Diversity 55:47–64.
- Zudaire E, Gambardella L, Kurcz C, Vermeren S. 2011. A computational tool for quantitative analysis of vascular networks. PLoS ONE 6:e27385.