ABSTRACT
Twenty-two Colletotrichum strains were isolated from anthracnose symptoms or leaf spots on leaves of various wild Poaceae and Cyperaceae plants collected in three provinces of Iran and tentatively identified as belonging to the Graminicola species complex based on morphology. All strains were studied via a polyphasic approach combining colony characteristics, morphology and phylogeny inferred from multi-locus sequences, including the nuc rDNA ITS1-5.8S-ITS2 (ITS), partial sequences of the β-tubulin (tub2), actin (act), manganese superoxide dismutase 2 (sod2), DNA lyase 2 (apn2) genes, a 200-bp intron of the glyceraldehyde-3-phosphate dehydrogenase (gapdh), and the intergenic spacer between the apn2 gene and the mat1 idiomorph (apn2/mat1). Six species were distinguished, including three new species, namely C. caspicum, C. persicum, and C. sacchari, and three previously described species, C. cereale, C. nicholsonii and C. sublineola. Comprehensive morphological descriptions and illustrations are provided for all species. Furthermore, this study provided new insights into the distribution and host range of known species.
GRAPHICAL ABSTRACT
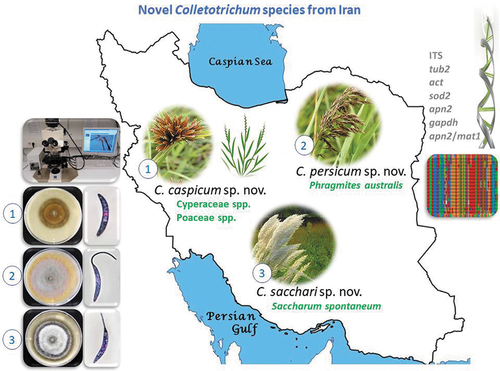
INTRODUCTION
Colletotrichum species are mainly known as pathogens of major importance causing anthracnose diseases of a wide range of plant families in tropical, subtropical, and temperate climates, but also of crown and stem rots, red rots, ripe rot, damping-off of blossoms, seedling blights and brown blotch diseases (Alizadeh et al. Citation2015; Arzanlou et al. Citation2015; Cannon et al. Citation2012; Lenné Citation2002; Marin-Felix et al. Citation2017). Some species are responsible for significant yield losses of economically important fruits such as strawberry, citrus, and banana, as well as many other crops including coffee, cereals, legumes, vegetables, forage plants and ornamentals (Atghia et al. Citation2015; Backman et al. Citation1982; Cannon et al. Citation2012; Liu et al. Citation2015; Phoulivong et al. Citation2010; Prihastuti et al. Citation2009; Smith and Black Citation1990; Yan et al. Citation2015).
Accurate identification of plant pathogenic fungi is a key step to study species diversity and epidemiology and therefore of uttermost importance for the development of effective control strategies for plant diseases (Alizadeh et al. Citation2015; Cai et al. Citation2009). Traditionally, the host-plant association has been used as the basis for identification and defining species in the genus Colletotrichum. Accordingly, several hundred species were described assuming that Colletotrichum species were specific to their host plants (Cannon et al. Citation2012). Von Arx (Citation1957) treated many of these species as synonyms and reduced the number of Colletotrichum species to 11 based on morphological characters with little or no attention to host specificity, which represented a drastic move in Colletotrichum systematics. Consequently, Colletotrichum species have been identified and delimited on morphological characters for many years (Simmonds Citation1965; Sutton Citation1992; Than et al. Citation2008a, Citation2008b; Thaung Citation2008). Delineation of Colletotrichum species based on morphology alone however is nearly impossible due to the lack of reliable morphological characters (Cai et al. Citation2009; Cannon et al. Citation2012; Hyde et al. Citation2010, Citation2009b).
In recent years, multi-locus sequence analyses combined with geographical, ecological, pathological and morphological data have been used for species differentiation within the genus Colletotrichum (Cai et al. Citation2009; Marin-Felix et al. Citation2017). This strategy led to significant progress in the systematics of Colletotrichum and revealed about 250 species within the genus, most of them belonging to large species complexes, namely the Acutatum, Agaves, Boninense, Dematium, Destructivum, Dracaenophilum, Gigasporum, Gloeosporioides, Graminicola, Magnum, Orbiculare, Orchidearum, Spaethianum and Truncatum species complexes (Bhunjun et al. Citation2021; Crouch et al. Citation2009c; Damm et al. Citation2009, Citation2012a, Citation2012b, Citation2013, Citation2014, Citation2019; Liu et al. Citation2013, Citation2014; Weir et al. Citation2012).
Colletotrichum species associated with more than 40 genera of Poaceae as well as with Bletilla ochracea (Orchidaceae) belong to the Graminicola species complex (Crouch Citation2014; Crouch et al. Citation2009a, Citation2009c, Citation2009d; Crouch and Tomaso-Peterson Citation2012; Moriwaki and Tsukiboshi Citation2009; Tao et al. Citation2013). Seven species from Poaceae hosts and the orchid Bletilla ochracea that are closely related to C. caudatum, form falcate conidia with a filiform appendage, except for one species, C. ochraceae, and are either regarded as the Caudatum subclade of the Graminicola species complex (Bhunjun et al. Citation2021; Crouch Citation2014; Tao et al. Citation2013; Zhang et al. Citation2020) or as Caudatum species complex (Jayawardena et al. Citation2016; Marin-Felix et al. Citation2017).
Within a survey, Colletotrichum species were isolated from wild plants with anthracnose symptoms or leaf spots collected in Northern Iran; some of them were previously reported (Alizadeh et al. Citation2015). Iran is a country with a high biodiversity that includes more than 8000 recorded plant species (Owfi Citation2020), of which many are considered as endemic or native plants (Noroozi et al. Citation2016). Wild Poaceae and Cyperaceae (Poales) species are regarded as the most important weeds in Iran (Keshavarzi Citation2021). However, the knowledge of Colletotrichum species associated with these plants in Iran is very low, and there is no report of a Colletotrichum species from any Poaceae or Cyperaceae in Iran (Farr and Rossman Citation2021), except for a recent report of C. karsti on Cyperus sp. in Iran originating from the same survey as this study (Alizadeh et al. Citation2015). Colletotrichum karsti forms straight conidia and belongs to the C. boninense species complex (Damm et al. Citation2012b). However, the majority of strains from the two plant families formed curved conidia and were closely related to the Graminicola species complex based on preliminary blastn searches on NCBI GenBank.
Therefore, the aim of this study is to characterize the Colletotrichum species from wild Poaceae and Cyperaceae plants in Northern Iran, based on a combination of morphology, culture characteristics, and multigene analyses.
MATERIALS AND METHODS
Sample collection and fungal isolation.—
Samples were collected from wild Poaceae and Cyperaceae plants with anthracnose symptoms or leaf spots in the Alborz, Golestan and Guilan provinces of Iran during 2011–2013. Leaf samples (approximately 1 cm diam) were washed in tap water, surface-disinfected in 2% sodium hypochlorite solution for 1 min, rinsed in sterile distilled water and moist incubated in glass petri dishes on autoclaved paper towels soaked with sterile tap water. Petri dishes were kept at 20–25℃ in the dark. Conidial masses or mycelia indicative of Colletotrichum were transferred to water agar (WA, 2%) plates supplemented with chloramphenicol (50 mg/L). Single spore or single hyphae isolates were obtained on potato dextrose agar (PDA, Merck, Darmstadt, Germany) (Goh Citation1999) and deposited in the Mycology Laboratory of the College of Agriculture and Natural Resources, University of Tehran, Karaj, Iran (UTFC) and the Iranian Fungal Culture Collection (IRAN) at the Iranian Research Institute of Plant Protection, Tehran, Iran. The ex-type strains of new species and some additional strains were deposited in the culture collections of the Westerdijk Fungal Biodiversity Institute, Utrecht, The Netherlands (CBS) and the Senckenberg Museum of Natural History Görlitz, Germany (GLMC), as well as the German Collection of Microorganisms and Cell Cultures, Braunschweig, Germany (DSMZ). Type specimens were deposited in the herbarium of the Iranian Research Institute of Plant Protection (IRAN) ().
Table 1. Strains of Colletotrichum spp. studied, with collection details and GenBank accession numbers
Morphological analysis.—
Both cultural and microscopic features were studied on synthetic nutrient agar (SNA, Nirenberg Citation1976), and on oatmeal agar (OA, Crous et al. Citation2019) according to Alizadeh et al. (Citation2015). Cultures were inoculated with 5-mm diam plugs from 4–7 d old cultures. To enhance sporulation, isolates were transferred to SNA amended with autoclaved filter paper and double-autoclaved stems of Anthriscus sylvestris. SNA and OA cultures were incubated at 25℃ under near-UV light with a 12-hours photoperiod for 10 d. Measurements and photomicrographs of fungal structures (conidia, conidiophores, conidiomata, setae and appressoria) were made according to Damm et al. (Citation2007). Appressoria were observed on the reverse side of SNA plates. Conidia were taken from acervuli. Microscopic preparations were made in clear lactic acid or methylene blue, with at least 40 measurements per structure and observed with a Nikon SMZ1000 dissecting microscope (DM) or with an Olympus BX53 microscope with differential interference contrast illumination (DIC). Colony characters and pigment production on SNA and OA were documented after 10 d. Growth rates were measured after seven and ten days. The descriptions provided were based on ex-type strains; data of strains with distinct morphological differences were added.
Phylogenetic analysis.—
Fungal isolates on PDA were incubated at 25℃ for 7–10 d. Genomic DNA was extracted using a standard phenol-chloroform extraction protocol (Sambrook and Russel Citation2001). The 5.8S nuclear ribosomal RNA gene with the two flanking internal transcribed spacers (ITS), a partial sequence of the manganese superoxide dismutase gene (sod2), the 3ʹ end of the DNA lyase gene (apn2), the 5ʹ end of the DNA lyase gene and mating type protein genes-like gene (apn2/mat1), partial sequences of the beta-tubulin (tub2) and actin (act) gens and a 200-bp intron of the glyceraldehyde-3-phosphate dehydrogenase (gapdh) were amplified using the primer pairs ITS-1 + ITS-4 (White et al. Citation1990), SOD625F/R, Apn1W1F/R and mat1M72F/R (Crouch et al. Citation2009c), GDF1 + GDR1 (Guerber et al. Citation2003), Act-512F + Act-783R (Carbone and Kohn Citation1999) and T1 (O’Donnell and Cigelnik Citation1997) + Bt-2b (Glass and Donaldson Citation1995), respectively, following the protocols explained in the respective references, except for tub2, act and gapdh. The PCR for these three loci was performed as following: an initial step of 5 min at 98°C, 35 cycles of 10 s at 98°C, 20 s at 65℃ and 20 s at 72°C, followed by 10 min at 72°C. PCR was performed in a TProfessional Thermocycler (Biometra, Germany) in a total volume of 25 μL. The PCR mixture contained 1 μL genomic DNA, 0.2 μM of each primer, 1 × HF Phusion PCR buffer (Thermo Scientific, Germany), 2 mM MgCl2, 20 μM of each dNTP, 0.75μ DMSO and 0.25 U Phusion High-Fidelity polymerase (Thermo Scientific, Germany). The PCR products were purified by the Wizard Genomic DNA Purification Kit (Promega, USA) and sequenced by Macrogen (Amsterdam, the Netherlands) with the amplifying primers.
The DNA sequences were used to obtain consensus sequences using BioNumerics 7.6.3 (Applied Math, St-Marthens-Lathem, Belgium); alignments were assembled and manually adjusted using BioNumerics and Sequence Alignment Editor 2.0a11 (Rambaut Citation2002). The sequences of the examined Iranian Colletotrichum isolates were compared with other fungal DNA sequences using the blast tools of the NCBI GenBank database (www.ncbi.nlm.nih.gov/genbank/) and the EPPO-Q-bank Fungi database (https://qbank.eppo.int/fungi/). Sequence data from ex-type and reference strains of known Colletotrichum species of the Graminicola species complex including its Caudatum subclade and of the outgroup C. gloeosporioides strain CBS 112999 (Cannon et al. Citation2012; Crouch Citation2014; Crouch et al. Citation2009a, Citation2006, Citation2009c; Crouch and Tomaso-Peterson Citation2012; Crouch et al. Citation2009d; Damm et al. Citation2012b; Moriwaki and Tsukiboshi Citation2009; O’Connell et al. Citation2012; Prihastuti et al. Citation2010; Rojas et al. Citation2010; Tao et al. Citation2013; Weir et al. Citation2012; Zhang et al. Citation2020) were obtained from NCBI GenBank ().
Maximum parsimony analyses were performed on multi-locus alignments 1 (ITS, sod2, apn2, apn2/mat1) following the studies of Crouch et al. (Citation2009c) and Crouch (Citation2014), and 2 (ITS, tub2, act, gapdh) following Tao et al. (Citation2013) as well as for each locus separately with PAUP (Phylogenetic Analysis Using Parsimony) 4.0b10 (Swofford Citation2003) using the heuristic search option with 100 random sequence additions and tree bisection and reconstruction (TBR) as the branch-swapping algorithm. The individual gene trees were assessed for clade conflicts between phylogenies. Alignment gaps were treated as missing and all characters were unordered and of equal weight. No more than 10 trees of score (length) greater than or equal to 10 were saved in each replicate. Tree length, consistency index (CI), retention index (RI), rescaled consistency index (RC) and homoplasy index (HI) were calculated for the resulting trees. The robustness of the trees obtained was evaluated by 100 000 bootstrap replications using the fast-stepwise addition algorithm (Hillis and Bull Citation1993). A Markov Chain Monte Carlo (MCMC) algorithm was used to generate phylogenetic trees with Bayesian probabilities using MrBayes 3.2.6 (Ronquist and Huelsenbeck Citation2003) for the combined sequence datasets. Models of nucleotide substitution for each gene determined by MrModeltest 2.3 (Nylander Citation2004) were included for each gene partition. The analyses of two MCMC chains were run from random trees for 1000 000 generations and sampled every 100 generations. The likelihood score of the two runs were 3400 and 3000 for the first analysis and 1100 and 1000 for the second analysis and therefore, the first 3200 and 1050 (the averages of both) trees, respectively, were discarded as the burn-in phases of the analyses and posterior probabilities determined from the remaining trees. Sequences derived in this study have been lodged in GenBank, the alignment and trees in TreeBASE (http://purl.org/phylo/treebase/phylows/study/TB2:S28088), and taxonomic novelties in MycoBank.
RESULTS
In the analyses of multi-locus alignment 1 (gene boundaries of ITS: 1–572, sod2: 583–1 328, apn2: 1339–2182, apn2/mat1: 2193–3779), 78 strains from Poaceae and Cyperaceae as well as the outgroup, and 3779 characters including the alignment gaps were processed, of which 1379 characters were parsimony-informative, 509 parsimony-uninformative and 1891 constant. After a heuristic search using PAUP, 430 equally most parsimonious trees were retained (length = 3747 steps, CI = 0.670, RI = 0.880, RC = 0.590, HI = 0.330), of which one is shown in . The topology of these trees was similar, which was verified for a large selection of trees (not shown). They differed not only in the position of strains within species and of branch lengths, but also in the position of some of the species. Within the Caudatum subclade, especially the position of single-strain species and of the two C. caudatum sequences differed. For the Bayesian analysis, a GTR+I+G model was selected for ITS, a HKY+I+G model for sod2 and a GTR+G model for apn2 and apn2/mat1, and incorporated in the analysis. The consensus tree obtained from Bayesian analysis confirmed the tree topology obtained with parsimony (not shown). Most of the Bayesian posterior probability values agreed with bootstrap support values.
Figure 1. The first of 430 equally most parsimonious trees obtained from a heuristic search of the combined ITS, sod2, apn2, and apn2/mat1 sequence alignment of the Graminicola species complex. Bootstrap support values above 50 % and Bayesian posterior probability values above 0.70 are shown at the nodes. Colletotrichum gloeosporioides strain CBS 112999 is used as outgroup. Numbers of types or ex-type strains are emphasized with an asterisk. The Caudatum subclade is indicated with a green bracket.
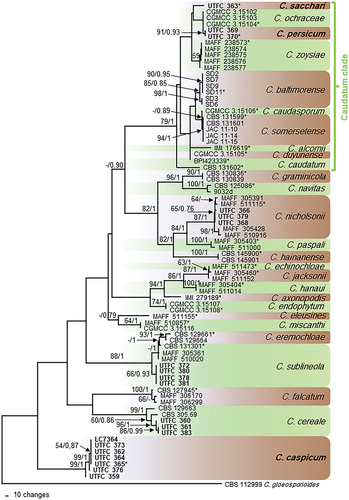
The three strains UTFC 363, UTFC 369 and UTFC 370 belong to a clade with a bootstrap support of 79% and a Bayesian posterior probability value of 1 representing the Caudatum clade of the Graminicola species complex. Within this clade, there are few supported clades; strains UTFC 369 and UTFC 370 form one of them (91/0.93), while strain UTFC 363 forms a single-strain clade. Most of the remaining species of the Graminicola species complex were represented by well supported clades. Each three strains collected in Iran cluster within C. cereale (99/1) and C. nicholsonii (87/1). Further four strains group within the C. sublineola/eremochloae clade (88/1). Seven strains from Poaceae and Cyperaceae from Iran and one strain from China form a well-supported clade basal to the other species of the Graminicola species complex (99/1). In some of the 430 most parsimonious trees, however, this clade was not basal, but clustered with the C. sublineola/eremochloae clade.
In the analyses of multi-locus alignment 2 (gene boundaries of ITS: 1–572, tub2: 583–1324, act: 1335–1632, gapdh: 1643–1973), 46 strains from Poaceae and Cyperaceae as well as the outgroup and 1973 characters including the alignment gaps were processed, of which 512 characters were parsimony-informative, 193 parsimony-uninformative and 1268 constant. After a heuristic search using PAUP, 711 equally most parsimonious trees were retained (length = 1 367 steps, CI = 0.699, RI = 0.851, RC = 0.594, HI = 0.301), of which one is shown in . The topology of these trees was similar, which was verified for a large selection of trees (not shown). They differed mainly in the position of strains within the species and branch lengths of clades. In some trees, C. duyunense was basal to the remaining species of the Caudatum subclade, and in some trees, the C. eremochloae clade was integrated in the C. sublineola clade, but separate in the others. For the Bayesian analysis, a GTR+I+G model was selected for ITS, a HKY+G model for tub2, a GTR+I model for gapdh, and a HKY+I model for act, and incorporated in the analysis. The consensus tree obtained from Bayesian analysis confirmed the tree topology obtained with parsimony (not shown). Most of the Bayesian posterior probability values agreed with bootstrap support values.
Figure 2. The first of 711 equally most parsimonious trees obtained from a heuristic search of the combined ITS, tub2, act, and gapdh sequence alignment of the Graminicola species complex. Bootstrap support values above 50 % and Bayesian posterior probability values above 0.70 are shown at the nodes. Colletotrichum gloeosporioides strain CBS 112999 is used as outgroup. Numbers of types or ex-type strains are emphasized with an asterisk. The Caudatum subclade is indicated with a blue bracket.
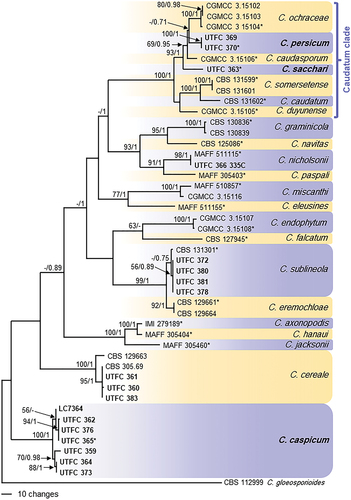
Strains UTFC 363, UTFC 369 and UTFC 370 belong to a long-branched clade with a bootstrap support of 100% and a Bayesian posterior probability value of 1, representing the Caudatum clade of the Graminicola species complex. Strains UTFC 369 and UTFC 370 form a well-supported clade (100/1), while strain UTFC 363 forms a single-strain clade on a long branch. The clade formed by strains UTFC 369 and UTFC 370 was well supported in all single-locus phylogenies, except for ITS (not shown). Both clades are sister clades of C. ochraceae and C. caudasporum. Most of the other species of the Graminicola species complex were represented by well supported clades. Seven strains from Poaceae and Cyperaceae from Iran and one strain from China form a well-supported clade basal to the other species of the Graminicola species complex (100/1). Strains UTFC 360, UTFC 361 and UTFC 363 cluster with C. cereale and strain UTFC 366 with C. nicholsonii with high support values (100/1 and 98/1, respectively); four further strains group with C. sublineola with low support (-/0.75).
The topologies of the single-locus phylogenies (not shown) did not show any conflicts, which allowed us to combine them in multi-locus alignments 1 and 2, respectively; they also agreed with the topologies of the respective multi-locus phylogenies. The placement of the strains isolated in this study was the same in the single-locus phylogenies as in the multi-locus phylogenies, and the clades formed by unidentified strains were supported in all of the single-locus phylogenies, except for the clade formed by strains UTFC 369 and UTFC 370 that was not supported in the ITS phylogeny; the branch of these two strains and that of UTFC 363 was very short.
TAXONOMY
Based on the DNA sequence data and morphology, the 22 isolates () from wild Poaceae and Cyperaceae plants in Iran belong to six Colletotrichum species, including C. cereale, C. nicholsonii and C. sublineola and three species that are new to science. All species studied in culture are characterized below.
Colletotrichum caspicum Alizadeh, Damm, F. Liu, Jav.-Nikkh. & Stukenbr. sp. nov.
Figure 3. Colletotrichum caspicum (A–C, E, G, I–T from ex-holotype strain UTFC 365. D from strain UTFC 376. F, H from strain UTFC 373). A–B. Colony on OA after 7 d, A. upper and B. reverse side. C, H. Setae. D–E. Acervuli. F–G. Conidiophores. I–N. Appressoria. O–T. Conidia. C–H, O–T. from Anthriscus stem. I–N. from SNA. D, E. DM. C, F–T. DIC. Scale bars: D, E = 50 μm, C, F–T = 10 μm.
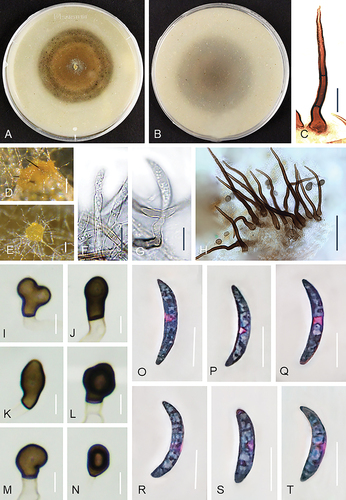
MycoBank: MB839808
Typification: IRAN. GUILAN: Rasht, from leaves of Cyperus sp., Aug 2012, A. Alizadeh (holotype IRAN 17632F). Ex-holotype culture IRAN 3709C = UTFC 365 = CBS 148570 = DSM 113396 = GLMC 2641. GenBank: ITS = MW741443; sod2 = MW822612; apn2 = MW822635; apn2/mat1 = MW915578; tub2 = MW740344.
Diagnosis: Conidia on SNA measure 14–25 × 3–4.5 μm and on Anthriscus stem 14–28 × 2.5–4.5 μm, appressoria on SNA 5–21 × 4–9 µm (all strains included). The species differs from all other Colletotrichum species by its unique DNA sequences (ITS, sod2, apn2, apn2/mat1, tub2, act) and from its closest relative C. cereale also by its slower growth and lower sporulation.
Etymology: Referring to the Caspian Sea, near which this fungus was first collected.
On Anthriscus stem: Conidiomata scarce, not observed in strains UTFC 359, UTFC 362 and UTFC 364, basal cells not observed, setae and conidiophores mostly formed directly on hyphae. Setae medium to dark brown, 3–5-septate, 58–103 μm long, smooth-walled, tapering to an acute apex, base cylindrical, conical or inflated, 4.5–7.5 μm diam, no setae observed in UTFC 359. Conidiophores rarely observed, hyaline to pale brown, simple or septate and branched, up to 60 μm long. Conidiogenous cells hyaline to very pale brown, smooth-walled, cylindrical, 5–25 × 3–4 μm, opening 1–1.5 μm, collarette and periclinal thickening not observed. Conidia enteroblastic, aseptate, hyaline, smooth-walled, falcate, almost uniformly curved, apex ± acute, base truncate, 14–25 × 3–4.5 μm, mean ± SD = 20.5 ± 2.6 × 3.7 ± 0.3 μm, L/W ratio = 5.6. Conidia of strain UTFC 376 larger and narrower, measuring 21–28 × 2.5–4 μm, mean ± SD = 25 ± 1.7 × 3.3 ± 0.28 μm, L/W ratio = 7.6. UTFC 359 not sporulating.
On SNA: Hyphae hyaline, smooth-walled, septate, branched, 1–6 μm diam. Conidiomata rarely observed, conidiophores and setae mostly formed directly on hyphae. Setae only observed in UTFC 376, medium to dark brown, smooth-walled, 2–4-septate, straight, 67–130 μm long, tapering to an acute apex, base cylindrical to conical, sometimes inflated, 5–9.5 μm diam. Conidiophores rarely observed, hyaline to pale brown, simple or septate and branched, up to 60 μm long. Conidiogenous cells hyaline to very pale brown, smooth-walled, cylindrical, 5–27 × 3–4 μm, opening 1–1.5 μm, collarette and periclinal thickening not observed. Conidia enteroblastic, aseptate, hyaline, smooth-walled, falcate, almost uniformly curved, apex ± acute, base truncate, 14.5–25 × 3–4.5 μm, mean ± SD = 21 ± 2.5 × 3.9 ± 0.3 μm, L/W ratio = 5.2. UTFC 359 not sporulating. Hyphal appressoria solitary, aseptate, smooth-walled, pale to medium brown, globose, subglobose to ellipsoidal, sometimes clavate or irregulare shaped, entire edge, more or less lobed, 5–9 × 4–6 μm, mean ± SD = 7.5 ± 1 × 5 ± 0.6 μm, L/W ratio = 1.5. Appressoria of strain UTFC 376 larger, measuring 6–21 × 4–9 μm, mean ± SD = 11.3 ± 3.9 × 6.4 ± 1.6 μm, L/W ratio = 1.8.
Culture characteristics: Colonies on OA flat and effuse with entire margin, ocher brown, with a grayish brown margin, reverse pale brown, 57–60 mm diam in 7 d. Colony color very different in strain UTFC 359, yellow, ocher brown to grayish brown at the center, reverse pale yellow to honey, yellowish gray in the center. Colonies on SNA flat with entire margin, hyaline to pale honey, reverse same colors, 55–60 mm diam in 7 d (>85 mm diam in 10 d). Conidia in mass buff to orange.
Additional specimens examined: IRAN. GUILAN: Rasht, from leaves of Cyperus sp., Aug 2012, A. Alizadeh, culture IRAN 3708C = UTFC 364; IRAN. GOLESTAN: Gorgan, Tooskestan, from leaves of a Poaceae sp., Aug 2011, A. Alizadeh, culture IRAN 4291C = UTFC 362; IRAN. ALBORZ: Karaj, from leaves of Cynodon dactilon, Jun 2012, A. Alizadeh, culture IRAN 4290C = UTFC 359 = CBS 148569 = DSM 113395 = GLMC 2642; IRAN. GUILAN: Lahijan, from leaves of a Poaceae sp., Oct 2013, A. Alizadeh, culture IRAN 4293C = UTFC 376; IRAN. GOLESTAN: Bandar Torkaman, from leaves of Cyperus sp., Oct 2013, A. Alizadeh, culture IRAN 4292C = UTFC 373.
Notes: Colletotrichum caspicum was isolated from Poaceae and Cyperaceae species, which indicates its wide host range. While several species were described from different Poaceae, no Colletotrichum species was previously described from Cyperus species (Cyperaceae). However, a few species were reported from this host genus (Farr and Rossman Citation2021), including C. fructicola on Cyperus microiria in Japan (Hirayama et al. Citation2018), C. karsti on Cyperus sp. in Iran (Alizadeh et al. Citation2015), and C. truncatum and its synonym C. dematium f. truncatum on Cyperus rotundus in Brazil and in the United States, respectively (Damm et al. Citation2009; Roy Citation1982), only the latter forms curved conidia, and all belong to other species complexes than C. caspicum.
Colonies of C. caspicum differ from those of its closest relative C. cereale that is faster growing and more strongly sporulating than C. caspicum; the orange conidial masses of C. cereale are visible in the center of the OA colony ().
Figure 4. Colletotrichum cereale (from strain UTFC 383). A–B. Colony on OA after 7 d, A. upper and B. reverse side. C. Tip of a seta. D, E, H. Acervuli with setae. F, G. Conidiophores. I. Base of a seta. J–O. Appressoria. P. Conidia. C, F–I, P. from Anthriscus stem. D–E, J–O. from SNA. D, E. DM. C, F–P. DIC. Scale bars: D, E = 50 μm, C, F–P = 10 μm.
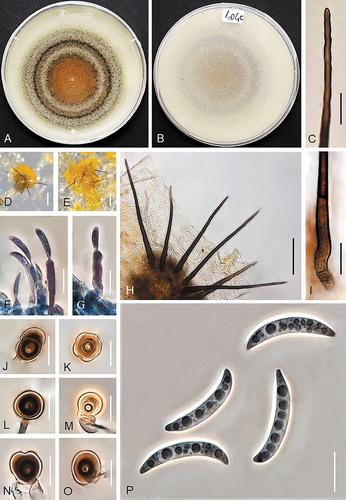
Colletotrichum caspicum is not closely related to any other Colletotrichum species and can be identified with sequences of all loci available. In blastn searches on NCBI GenBank, the ITS sequence of the ex-type strain, UTFC 365, matched with 99% identity (1 and 2 nucleotides difference, respectively) with Colletotrichum sp. 2 strain C_3_1 from leaves of Carex secalina (Cyperaceae) in Poland (MG978338, Górzyńska et al. Citation2019) and Colletotrichum sp. strain MBD_1013 from a leaf of Dalea purpurea (Fabaceae) in the USA (MK595495, M.B. DeMers and G. May, unpubl. data), while the closest ex-type strain was with 97% identity (17 nc. diff.) that of C. verruculosum, IMI 45525 (GU227806), belonging to the Spaethianum species complex (Damm et al. Citation2009). Closest matches with the act sequence of C. caspicum strain LC7364 were with 93% identity (17 nc. diff.) C. cereale strains CBS 129663 (JQ005837, O’Connell et al. Citation2012) and CGMCC 3.15110 (KC843534, Tao et al. Citation2013). Closest matches with the tub2 sequences of C. caspicum were with 89% identity several C. tofieldiae strains and with 88% identity C. graminicola strain STE-U 5298 (AY376587, Lubbe et al. Citation2004); closest ex-type strain was with 89% identity that of C. hanaui. Closest matches with the sod2, apn2 and apn2/mat1 sequences of C. caspicum were with ≤ 90% and ≤ 82% identity, respectively, those of several species of the Graminicola species complex.
Colletotrichum cereale Manns, Ohio Agric. Exp. Stn. Bull. 203: 207 (1909).
On Anthriscus stem: Conidiomata acervular, abundant. Setae abundant, medium to dark brown, 2–4-septate, straight, 120–270 μm long, smooth-walled, tip rounded to somewhat acute, base cylindrical to conical, sometimes inflated, 4–8 μm diam. Conidiophores hyaline to pale brown, simple, septate, up to 80 μm long. Conidiogenous cells hyaline to pale brown, smooth-walled, clavate or cylindrical, 5–18 × 3–6 μm, opening 1.5–2 μm, collarette 1–1.5 μm long, periclinal thickening visible. Conidia enteroblastic, aseptate, hyaline, smooth-walled, falcate, central part often with nearly parallel walls, more strongly curved toward the acute apex, base truncate, 22.5–28.5 × 4–4.5 μm, mean ± SD = 24.7 ± 1.4 × 4.5 ± 0.3 μm, L/W ratio = 5.4.
On SNA: Hyphae septate, branched, hyaline, 2–5.5 μm diam. Conidiomata acervular, abundant on agar surface. Setae abundant, medium to dark brown, smooth-walled, 2–4-septate, straight, 85–252 μm long, tip round or somewhat acute, base cylindrical to conical, sometimes inflated, 4–8 μm diam. Conidiogenous cells hyaline to pale brown, smooth-walled, clavate or cylindrical, 6–25 × 3–6 μm, opening 1.5–2 μm, collarette 1–1.5 μm long, periclinal thickening visible. Conidia enteroblastic, aseptate, hyaline, smooth-walled, falcate, central part often with nearly parallel walls, more strongly curved toward the acute ends, base truncate, 22–27 × 3.5–4.5 μm, mean ± SD = 23.4 ± 1.3 × 4 ± 0.4 μm, L/W ratio = 5.8. Hyphal appressoria solitary, aseptate, smooth-walled, medium to very dark brown, ellipsoidal to clavate, sometimes irregularly shaped, entire edge, more or less lobed, 7.5–13.5 × 6–10.5 μm, mean ± SD = 12.5 ± 1.9 × 9.5 ± 1.6 μm, L/W ratio = 1.7.
Culture characteristics: Colonies on OA flat with entire margin, orange at center due to abundant sporulation, smoky gray, pale to dark brown with darker brown spots toward the margin, partly covered by fluffy, white to smoky gray aerial mycelia, reverse buff, pale gray to cinnamon, 68–70 mm diam in 7 days at 25°C. Colonies on SNA flat with entire margin, hyaline, filter paper, Anthriscus stem and agar medium covered with white to pale olivaceous gray aerial mycelium, reverse hyaline to buff, 67–70 mm diam in 7 d (>90 mm diam in 10 d). Conidia in mass orange.
Specimens examined: IRAN. GOLESTAN: Gorgan, from leaves of Saccharum spontaneum, Jun 2013, A. Alizadeh, culture IRAN 3705C = UTFC 360 = CBS 148572 = DSM113398 = GLMC 2643; IRAN. GOLESTAN: Gorgan, from leaves of Saccharum spontaneum, Jul 2012, A. Alizadeh, living culture IRAN 3706C = UTFC 361; IRAN. GUILAN: Lahijan, from leaves of Lolium sp., Oct 2013, A. Alizadeh, living culture IRAN 3717C = UTFC 383 = CBS 148571 = DSM 113397 = GLMC 2644.
Notes: Colletotrichum cereale is known as a species with a worldwide distribution, and both as a plant pathogen of cool-season grasses (C3) of the subfamily Pooideae (Crouch et al. Citation2009d) and an endophyte of Bletilla ochracea (Orchidaceae) (Tao et al. Citation2013). Crouch et al. (Citation2006) regarded the species as a distinct taxon with highly specialized populations corresponding to ecosystem and/or host plant. The strains examined in this study have similar conidia and appressoria shapes and dimensions as C. cereale strain CBS 129663 (Crouch et al. Citation2006). All loci included in this study separate C. cereale distinctly from all other species.
Colletotrichum nicholsonii J.A. Crouch, B.B. Clarke, J.F. White et B.I. Hillman.
Figure 5. Colletotrichum nicholsonii (from strain UTFC 366). A. Colony on OA after 7 d. B. Colony on SNA amended with a double autoclaved stem of Anthriscus sylvestris and autoclaved filter paper. C. Seta. D, E. Acervuli. F, H. Conidiophores. G, O. Conidia. I–N. Appressoria. C, D. from Anthriscus stem. E. from autoclaved filter paper. F–O. from SNA. D–E. DM. C, F–O. DIC. Scale bars: D–E = 50 μm, C, F–O = 10 μm.
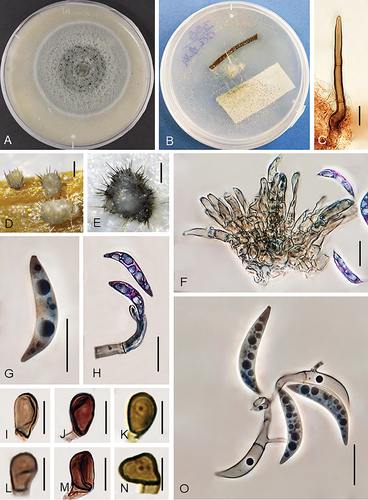
On Anthriscus stem: Conidiomata acervular, abundant. Setae abundant, medium to dark brown, 2–4-septate, straight, up to 170 μm long, smooth-walled, tapering to an acute to rounded apex, base cylindrical, sometimes bent or inflated, 6–8 μm diam. Conidiophores pale to medium brown, branched, septate, up to 70 μm long. Conidiogenous cells pale to medium brown, smooth-walled, clavate or cylindrical, up to 25 μm long, opening 1.5–2 μm, collarette 1–1.5 μm long, periclinal thickening visible. Conidia enteroblastic, aseptate, hyaline, smooth-walled, falcate, strongly bent in the upper third toward an acute apex, base truncate 18.5–23.5 × 4.5–5.5 μm, mean ± SD = 21.5 ± 1 × 5 ± 0.3 μm, L/W ratio = 4.4.
On SNA: Hyphae hyaline, smooth-walled, septate, branched, 1.5–6 μm diam. Conidiomata acervular, abundant, especially on agar surface and on filter paper. Setae abundant, medium to dark brown, smooth-walled, 2–4-septate, straight, up to 260 μm long, tip round or somewhat acute, base cylindrical to conical, sometimes inflated, 2.5–5 μm diam. Conidiophores pale to medium brown, branched, septate, up to 80 μm long. Conidiogenous cells pale to medium brown, smooth-walled, clavate to cylindrical, up to 30 μm long, opening 1.5–2 μm, collarette and periclinal thickening not visible. Conidia enteroblastic, aseptate, hyaline, smooth-walled, falcate, strongly bent in the upper third toward an acute apex, base truncate, 14.5–24.5 × 4.5–5 μm, mean ± SD = 18.6 ± 1.1 × 4.9 ± 0.2 μm, L/W ratio = 4.4. Hyphal appressoria solitary, aseptate, smooth-walled, medium to dark brown, globose, oblong, ovoid, obovoid or clavate, edges entire or more or less lobed, 10.5–18 × 5–12 μm, mean ± SD = 13.5 ± 1.3 × 10 ± 1 μm, L/W ratio = 1.4.
Culture characteristics: Colonies on OA flat with entire margin, creamy white to gray, becoming dark gray with age in the center, reverse medium to dark gray, 69–71 mm diam in 7 d. Colonies on SNA flat with entire margin, hyaline, filter paper, Anthriscus stem and agar medium partly covered with white to pale olivaceous gray aerial mycelium, reverse hyaline to pale gray, 67–73 mm diam in 7 d (>90 diam mm in 10 d). Conidia in mass white to pale gray.
Specimens examined: IRAN. GUILAN: Rasht, from leaves of Paspalum dilatatum, Aug 2013, A. Alizadeh, culture IRAN 4294C = UTFC 366; IRAN GUILAN: Rasht, from leaves of Paspalum dilatatum, Oct 2013, A. Alizadeh, culture IRAN 3710C = UTFC 368; IRAN. GUILAN: Lahijan, from leaves of Paspalum dilatatum, Oct 2013, A. Alizadeh, culture IRAN 3715C = UTFC 379.
Notes: Colletotrichum nicholsonii was isolated from leaves of Paspalum dilatatum in this study. The colony characteristics, setae, conidial shape and dimension agree with the holotype of C. nicholsonii (Crouch et al. Citation2009c). In the phylogram, our three strains confidently clustered with the ex-type strain of C. nicholsonii (MAFF 511115) and strain MAFF 305428.
Colletotrichum persicum Alizadeh, Damm, Jav.-Nikkh & Stukenbr. sp. nov.
Figure 6. Colletotrichum persicum (from ex-holotype strain UTFC 370). A–B. Colony on OA after 7 d, A. upper and B. reverse side. C. Tip of a seta. D–F. Conidiophores. G. Basis of a seta. H–L. Conidia. M–R. Appressoria. C–L. from Anthriscus stem. M–R. from SNA. C–R. DIC. Scale bars: C–R = 10 μm.
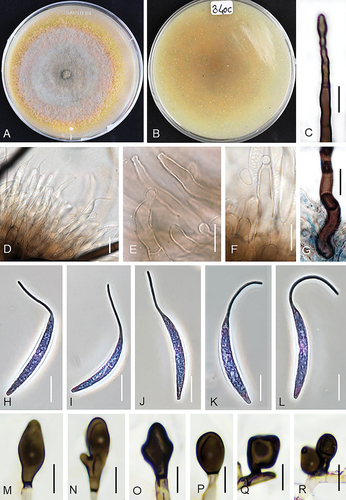
MycoBank: MB839810
Typification: IRAN. GUILAN: Kuchesfahan, from leaves of Phragmites australis, Oct 2013, A. Alizadeh (holotype IRAN 17633F). Ex-holotype culture IRAN 3712C = UTFC 370 = CBS 148574 = DSM 113400 = GLMC 2645. GenBank: ITS = MW741433; sod2 = MW822604; apn2 = MW822624; apn2/mat1 = MW915570; tub2 = MW740367; act = MW822655; gapdh = MW740228.
Diagnosis: Conidia on Anthriscus stem measure 26–35.5 × 3–5.5 μm (excluding appendages) and appressoria on SNA 10–23 × 5–13 µm. The species differs from all other Colletotrichum species by its unique DNA sequences (as far as currently comparable) and by the existence or the length, respectively, of conidial appendages, which exceeds those of all other species (11–20 μm long, mean = 15.2 μm). Value, site and direction of their curvature are very variable in contrast to the uniform appendages of C. sacchari.
Etymology: Referring to the ancient name of Iran, the country from which this fungus was first collected.
On Anthriscus stem: Conidiomata acervular, conidiophores and setae formed on a basal cushion of pale brown angular cells. Setae abundant, medium to dark brown, 3–5-septate, 75–198 μm long, smooth-walled, tapering toward a round or slightly acute, base cylindrical to conical, sometimes inflated, 4–7 μm diam. Conidiophores, hyaline, pale to medium brown, branched, septate, up to 70 μm long. Conidiogenous cells pale to medium brown, smooth-walled, clavate, cylindrical, opening 1.5–2 μm, collarette 1–1.5 μm long, periclinal thickening visible. Conidia enteroblastic, aseptate, hyaline, smooth-walled, falcate, apex prolonged into a filiform appendage, 26–35.5 × 3–5.5 μm, mean ± SD = 30.4 ± 2.09 × 4.3 ± 0.6 μm, L/W ratio = 7.1 (excluding appendage), conidial appendage 11–20 µm long, mean ± SD = 15.2 ± 2.3 μm.
On SNA: Hyphae septate, branched, hyaline to pale brown, 1–6 μm diam. Not sporulating. Setae not observed. Hyphal appressoria solitary, aseptate, smooth-walled, pale to dark brown, globose, subglobose to ellipsoidal, oblong to clavate, edges entire or more or less lobed, 10–23 × 5–13 μm, mean ± SD = 16 ± 4.1 × 9 ± 1.9 μm, L/W ratio = 1.8.
Culture characteristics: Colonies on OA flat with entire margin, surface partly covered with white to pale mouse gray cottony aerial mycelium, luteous at the margin, a yellow pigment diffusing into the agar medium, reverse luteous, 58–60 mm diam in 7 d. Colonies on SNA flat with entire, sometimes fimbriate or irregular margin, filter paper, Anthriscus stem and agar medium covered with fluffy, olivaceous gray aerial mycelium; reverse olivaceous gray, dark olivaceous gray in the center, 60–62 mm diam in 7 d (>90 diam mm in 10 d). Conidia in mass yellow.
Additional specimen examined: IRAN. GUILAN: Kuchesfahan, from leaves of Phragmites australis, Oct 2013, A. Alizadeh, culture IRAN 3711C = UTFC 369 = CBS 148573 = DSM 113399 = GLMC 2646.
Notes: No Colletotrichum species was previously described from Phragmites, but Grove (Citation1916) described a variety, namely C. lineola var. phragmitis, from culms of Phragmites in Moreton, Cheshire, UK. The spores of this fungus were oblong-fusoid, measuring 15–25 × 3–4 µm; that means, they are not only shorter than those of C. persicum, but also have a different shape. The only report of a Colletotrichum species from Phragmites based on molecular data is that of C. destructivum (Destructivum species complex) on Phragmites sp. in the USA (Damm et al. Citation2014), while the study of Wong and Hyde (Citation2001) includes unidentified Colletotrichum species on Phragmites australis in Hong Kong.
Colletotrichum persicum can be identified with sequences of all loci available. In a blastn search on NCBI GenBank, the ITS sequence of the ex-type strain UTFC 370 was 99% identical (2 nucleotides difference) with those of C. caudatum isolates MAFF 305700 and I-99 (AB042304 and AB042305, Moriwaki et al. Citation2002), closest ex-type strain was with 3 nucleotides difference that of C. caudasporum. Closest matches with the gapdh sequence of C. persicum were with 97% identity (8 nc. diff.) C. ochraceae strains CGMCC 3.15102 and CGMCC 3.15104 (ex-type); closest matches with the act and tub2 sequences with 98% (6 nc. diff.) and 99% (5 nc. diff.), respectively, the C. caudasporum strain. The sod2 sequence was 97% identical (12 nc. diff.) with those of all C. zoysiae strains (no sod2 sequence of the ex-type strain available). Closest matches with the apn2 sequences of C. persicum were those of C. caudatum isolate MAFF 305700 (JX076926, Crouch Citation2014) with 99% identity (9 nc. diff.); the closest ex-type strain that of C. zoysiae with 98% identity. Closest matches with the apn2/mat1 sequence of C. persicum were those of C. baltimorense isolates SD3 and SD6 with 97% identity (27 nc. diff.); the closest ex-type strains that of C. somersetense with 96% identity.
Like the species from Saccharum spontaneum that is described below, C. persicum belongs to the Caudatum subclade of the Graminicola species complex that is characterized by the formation of curved conidia with a filiform appendage (Crouch Citation2014). However, C. ochraceae, the closest neighbor of C. persicum, is an exception as it forms conidia without appendages (Tao et al. Citation2013). Therefore, the two species can easily be distinguished from each other by the existence of a filiform appendage. With an average of 15.2 µm (11–20 µm) the conidial appendages of C. persicum are larger than those of all other species in this clade. In contrast to C. duyunensis, in which setae are lacking, C. persicum forms abundant setae.
Colletotrichum sacchari Alizadeh, Damm, Jav.-Nikkh & Stukenbr. sp. nov.
Figure 7. Colletotrichum sacchari (from ex-holotype strain UTFC 363). A–B. Colony on OA after 7 d, A. upper and B. reverse side. C. Seta. D. Acervuli. E–G. Conidiophores. H–L. Conidia. M–R. Appressoria. C–L. from Anthriscus stem. M–R. from SNA. D. DM. C, E–R. DIC. Scale bars: D = 50 μm, C, E–R = 10 μm.
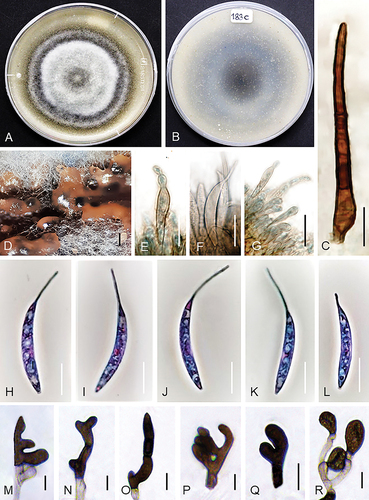
MycoBank: MB839811
Typification: IRAN. Golestan: Galugah, from leaves of Saccharum spontaneum, Sep 2012, A. Alizadeh & A. Pordel (holotype IRAN 17630F). Ex-holotype culture IRAN 3707C = UTFC 363 = CBS 148575 = DSM 113401 = GLMC 2647. GenBank: ITS = MW741431; sod2 = MW822602; apn2 = MW822622; apn2/mat1 = MW915568; tub2 = MW740368; act = MW822656; gapdh = MW740229.
Diagnosis: Conidia on Anthriscus stem measure 23–31.5 × 4.5–6 μm (excluding appendages), conidial appendages 11–16.5 µm and appressoria on SNA 11.5–50 × 6–19.5 μm. The species differs from all other Colletotrichum species by its unique DNA sequences (as far as currently comparable) and the often deeply lobed to branched and septate appressoria. Conidial appendages are uniform regarding value, site and direction of their curvature in contrast to the very variable appendages of C. persicum, while they are lacking in C. ochraceae.
Etymology: Referring to the host, Saccharum.
On Anthriscus stem: Conidiomata acervular, forming cushions of pale brown angular cells, from which setae and conidiophores are produced. Setae abundant, medium to dark brown, 2–4-septate, however septation hardly visible, straight, sometimes bent, 121–226 μm long, smooth-walled, tapering toward a round or slightly acute apex, base cylindrical to conical, sometimes inflated, 4–7.5 μm diam. Conidiophores pale to medium brown, simple, septate, up to 70 μm long. Conidiogenous cells pale to medium brown, smooth-walled, clavate or cylindrical, opening 1.5–2 μm, collarette and periclinal thickening not observed. Conidia enteroblastic, aseptate, hyaline, smooth-walled, falcate, apex prolonged to a filiform appendage, 23–31.5 × 4.5–6 μm, mean ± SD = 27.5 ± 1.8 × 4.7 ± 0.2 μm, L/W ratio = 5.8 (excluding appendage), conidial appendage 11–16.5 µm long, mean ± SD = 13.5 ± 1.6 μm.
On SNA: Hyphae hyaline to pale brown, smooth-walled, septate, branched, 1–6 μm diam. Not sporulating. Setae not observed. Hyphal appressoria smooth-walled, medium to dark brown, either aseptate, globose, subglobose, ellipsoidal to clavate with entire margin or more complex, irregularly shaped, elongate cylindrical, deeply lobed to branched and septate, 11.5–50 × 6–19.5 μm, mean ± SD = 18.8 ± 8.3 × 10.5 ± 2.8 μm, L/W ratio = 1.8.
Culture characteristics: Colonies on OA flat with entire margin, almost entirely covered with whitish to smoky gray, cottony aerial mycelium, olivaceous at the margin, reverse pale gray, pale blue or pale violet, dark gray at the center, 69–70 mm diam in 7 d. Colonies on SNA flat with entire margin, hyaline; filter paper, Anthriscus stem and agar medium covered with whitish, buff to smoky gray aerial mycelium, reverse hyaline with black spots mainly under the filter paper, 70–72 mm diam in 7 d (>90 diam mm in 10 d). Conidia in mass orange.
Notes: Colletotrichum sacchari is not closely related to C. falcatum that was also described from Saccharum, however from S. officinarum in Indonesia. The ex-type strains of C. sacchari and C. falcatum belong to different clades in the phylogenies of this study (). In contrast to C. falcatum, C. sacchari belongs to the Caudatum subclade of the Graminicola species complex that is characterized by the formation of curved conidia with appendages (Crouch Citation2014). Von Arx (Citation1957) synonymized Colletotrichum species with falcate conidia from Poaceae, including C. falcatum, with C. graminicola, however considered the existence of formae speciales on different hosts. After several pathogenicity tests on sorghum, sugar cane and rye with a strain from maize failed, Messiaen et al. (Citation1959) implemented this by naming some formae speciales of C. graminicola, including C. graminicola f. sp. sacchari, apparently referring to C. falcatum however, lacking any definite connection to it.
There is only one report of a Colletotrichum species from S. spontaneum listed in the USDA fungal database (Farr and Rossman Citation2021), that of C. falcatum on S. spontaneum in India. However, there are several reports from S. officinarum, including: C. crassipes in Malaysia (Liu Citation1977), C. exoticum in India (Mathur Citation1979), C. falcatum in many countries (Sutton Citation1980), C. gloeosporioides in India (Viswanathan et al. Citation2003), C. graminicola in several countries mostly located in south America (Farr and Stevenson Citation1963), C. graminicola f. sp. sacchari in Brazil (Mendes et al. Citation1998), C. graminicola (as C. graminicolum) in Pakistan (Ahmad et al. Citation1997) and Colletotrichum sp. in India (Alvarez Citation1976; Mathur Citation1979). Most of these reports are very old and there is no DNA data to support the identification of the reported fungi. Moreover, some of the reported species have not yet been revised in modern terms. However, none of these fungi produce conidia with a filiform appendage that is the prominent feature of the members of the Caudatum clade, including C. sacchari and it is therefore unlikely that any of these reports refers to C. sacchari. In this study, we also isolated C. cereale and C. sublineola from S. spontaneum in Iran.
Colletotrichum sacchari can be identified with sequences of all loci studied here. In a blastn search on NCBI GenBank, the ITS sequence of the ex-type strain UTFC 363 was 99% identical (3 nc. diff.) with that of C. ochraceae strains CGMCC 3.15102 and CGMCC 3.15103 and C. zoysiae strains MAFF 238573 (ex-type), MAFF 238574 and MAFF 238575 that are included in this study. Closest matches with the gapdh sequence of C. sacchari were with 97% identity (8 nc. diff.), C. ochraceae strains CGMCC 3.15102 and CGMCC 3.15104 (ex-type); closest match with the act and tub2 sequences was with 98% (5 nc. diff.) C. caudasporum strain CGMCC 3.15106. The sod2 sequence was 98% identical (11 nc. diff.) with those of C. zoysiae, including its ex-type and 98% identical (4 nc. diff.) with that of the lectotype of C. caudatum, BPI423339; the latter sequence is however very short. Closest matches with the apn2 and apn2/mat1 sequences of C. sacchari were those of C. caudatum isolate MAFF 305700 (JX076926, Crouch Citation2014) with 99% and 97% identity (8 and 34 nc. diff.), respectively; the closest ex-type strains those of C. zoysiae and C. somersetense with 98% and 96% identity, respectively.
Colletotrichum sacchari differs morphologically from C. persicum that is described above in terms of conidial dimension, shape and size of appressoria, size and conidial appendages. The L/W ratio of conidia of C. sacchari is lower than that of C. persicum (5.8 vs. 7.1). Moreover, the conidial appendages of C. sacchari are very uniform, like an extension of the conidium; the direction of their curvature is usually toward the dorsal side or longitudinal axis of the conidia. In contrast, the conidial appendages of C. persicum are very variable regarding value, site and direction of the curvature that is sometimes toward the dorsal and sometimes toward the ventral side of the conidium. They vary from nearly straight to strongly curved, are sometimes bent just at its origin at the conidium and sometimes near its tip, just as they would be flexible. The conidial appendages of C. sacchari are longer than those of C. alcornii, that measure 2.0–6.0 μm. C. sacchari apparently differs from C. duyunensis by setae formation (abundant setae in C. sacchari vs. absence of setae in C. duyunensis). Colletotrichum sacchari can easily be distinguished from all others species in the caudatum clade by shape and size of appressoria ().
Colletotrichum sublineola Henn. ex Sacc. & Trotter, Syll. Fung. (Abellini) 22: 1206 (1913). .
Figure 8. Colletotrichum sublineola (from strain UTFC 381). A–B. Colony on OA after 7 d, A. upper and B. reverse side. C. Tip of a seta. D, E. Acervuli. F. Conidiophores. G. Base of a seta. H, I, P. Conidia. J–O. Appressoria. C, G–I. from Anthriscus stem. D–F, J–P. from SNA. D, E. DM. C, F–P. DIC. Scale bars: D–E = 50 μm, C, F–P = 10 μm.
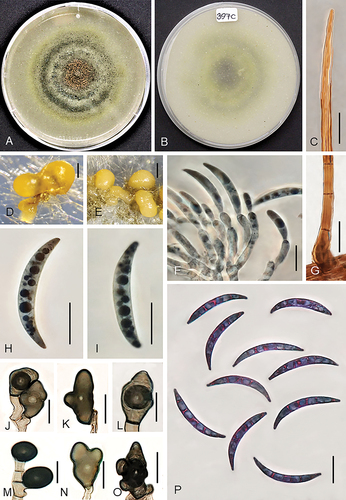
On Anthriscus stem: Conidiomata acervular, abundant. Setae abundant, medium to dark brown, 2–4-septate, straight, up to 150 μm long, smooth-walled, tapering to an ± acute apex, base cylindrical, sometimes bent or inflated, 6–7.5 μm diam. Conidiophores hyaline or hyaline to pale brown, branched, septate, up to 50 μm long. Conidiogenous cells hyaline or hyaline to pale brown, smooth-walled, clavate to cylindrical, opening 1.5–2 μm, collarette and periclinal thickening not visible. Conidia enteroblastic, aseptate, hyaline, smooth-walled, falcate, slightly more strongly curved toward the acute apexes than the truncate bases, 23–27.5 × 4–4.5 μm, mean ± SD = 25.2 ± 1.2 × 4.2 ± 0.2 μm, L/W ratio = 6.
On SNA: Hyphae septate, branched, hyaline, 1.5–6 μm, Conidiomata acervular, abundant. Setae abundant, smooth-walled, pale to medium brown, 2–4-septate, straight, up to 140 μm long, tapering toward a round or slightly acute apex, base cylindrical to conical, sometimes inflated, 2.5–7.5 μm diam. Conidiophores pale to medium brown, branched, septate, up to 50 μm long. Conidiogenous cells pale to medium brown, smooth-walled, clavate to cylindrical, opening 1.5–2 μm, collarette and periclinal thickening not visible. Conidia enteroblastic, aseptate, hyaline, smooth-walled, falcate, slightly more strongly curved toward the acute apexes than the truncate bases, 20–29.5 × 3.5–5 μm, mean ± SD = 23 ± 2.1 × 3.5 ± 0.2 μm, L/W ratio = 6.5. Hyphal appressoria solitary, aseptate, smooth-walled, pale to medium brown, globose, oblong, ovoid, obovoid to clavate, edges entire or more or less lobed, 10–21.5 × 10–17 μm, mean ± SD = 16.5 ± 1.3 × 14.8 ± 1.4 μm, L/W ratio = 1.4.
Culture characteristics: Colonies on OA flat with entire margin, pale olivaceous gray to greenish gray, partly covered with gray, felty to wooly aerial mycelium, and in the center with orange conidial masses, yellow to luteous pigment diffusing into the agar medium, reverse pale olivaceous gray to luteous, 73–75 mm diam in 7 d. Colonies on SNA flat with entire margin, hyaline, filter paper, Anthriscus stem and agar medium covered with white to pale olivaceous gray aerial mycelium; reverse hyaline, 69–70 mm diam in 7 d (>90 diam mm in 10 d). Conidia in mass orange.
Specimens examined: IRAN. GUILAN: Rasht, from leaves of Sorghum halepense, Oct 2013, A. Alizadeh, culture IRAN 4296C = UTFC 380; IRAN. GUILAN: Rasht, from leaves of Saccharum spontaneum, Oct 2013, A. Alizadeh, culture IRAN 4297C = UTFC 381 = CBS 148576 = DSM 133402= GLMC 2648; IRAN. GUILAN: Rasht, from leaves of Sorghum halepense, Oct 2013, A. Alizadeh, culture IRAN 4295C = UTFC 378; IRAN. GUILAN: Rasht, from leaves of Sorghum halepense, Oct 2013, A. Alizadeh, culture IRAN 3714C = UTFC 372.
Notes: Colletotrichum sublineola was previously only reported from Sorghum species, including S. bicolor (United States, Brazil, Burkina Faso, Japan, South Africa, Sudan, Togo, Zambia), S. halepense (United States), Sorghum sp. (Togo, United States) and S. vulgare var. technicum (United States) (Farr and Rossman Citation2021). Moreover, this species was so far only reported from S. halepense (Johnsongrass) in the United States (Alabama, Florida, Georgia, Indiana, Kentucky, North Carolina, Texas). Considering that, this species is identified from Sorghum halepense and also from Saccharum spontaneum in this study, presumably still little is known about its geographical distribution and host range. Xavier et al. (Citation2018) showed that C. sublineola populations obtained from Sorghum bicolor and S. halepense from the southeastern United States are genetically distinct, although both are capable of infecting different cultivars of Sorghum bicolor.
DISCUSSION
In this study, six Colletotrichum species were differentiated that were associated with anthracnose symptoms on leaves or leaf spot diseases of wild Poaceae and Cyperaceae plants in Iran (), including three known species, C. cereale, C. nicholsonii and C. sublineola, while three species, namely C. caspicum, C. persicum and C. sacchari were newly described using a polyphasic approach combining multi-locus phylogeny, colony characteristics, morphology, collection and host data.
Figure 9. Wild Poaceae and Cyperaceae plants with symptoms of anthracnose or leaf spots from which Colletotrichum species were isolated in this study. A–B. Cyperus sp. (C. caspicum). C. Poaceae sp. (C. caspicum). D. Cynodon dactylon (C. caspicum). E. Lolium sp. (C. cereale). F–H. Paspalum dilatatum (C. nicholsonii). I–J. Phragmites australis (C. persicum). K–L. Saccharum spontaneum (C. sacchari). M. Anthracnose symptoms on Sorghum halepense (C. sublineola). N–P. Sorghum halepense (C. sublineola).
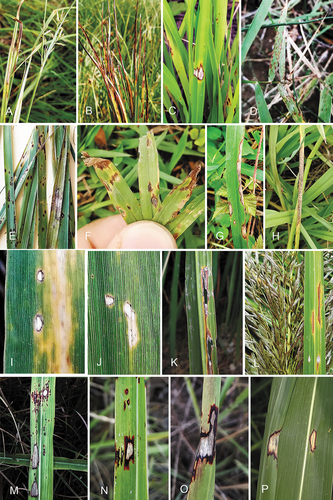
In simultaneous studies, different sets of loci had previously been used for discriminating species among the different species complexes in the genus Colletotrichum. For example, Crouch et al. (Citation2009c) and Crouch (Citation2014) used ITS, sod2, apn2 and apn2/mat1 to study the Graminicola species complex and its Caudatum subclade. In contrast, Tao et al. (Citation2013) applied ITS, gapdh, act and tub2 for studying endophytic Colletotrichum species from Bletilla ochracea, including species of the Graminicola species complex. As a result, individual species were characterized with different loci, and ITS is the only locus available for all species of the Graminicola complex described in these studies and therefore, the only locus that can be compared with all species. However, ITS sequences are known to have a resolution that is too low to resolve all species of the genus Colletotrichum (Crouch et al. Citation2009b; Damm et al. Citation2012a). Moreover, as only few morphological data of previously described Colletotrichum species from Poaceae are available for comparison, taxonomic studies of species in the Graminicola complex are challenging, and both identification and discovering of species are impeded. Some of the previously described species, especially those in the Caudatum subclade as well as C. echinochloae and C. jacksonii (Crouch et al. Citation2009c; Moriwaki and Tsukiboshi Citation2009), might be synonyms, which however, cannot be proven with certainty on this basis. We tried to solve this problem for the strains obtained from Poaceae and Cyperaceae collected in Iran in this study by calculating phylogenies from different data sets based either on the combinations of loci used by Crouch et al. (Citation2009c), Crouch (Citation2014) or those used by Tao et al. (Citation2013) and the inclusion of as many strains and taxa as possible in each of them, which was however a compromise. Missing data resulted in low or lacking support values, especially in the tree based on ITS, sod2, apn2 and apn2/mat1 sequences (). Therefore, the data sets, especially those of the ex-type strains, need to be completed in further studies and the suitability of the individual loci for species delimitation within the Graminicola complex evaluated.
The results of this study are very valuable for understanding the host-pathogen relationships in the Graminicola complex. Crouch et al. (Citation2009d) detected two distinct lineages within the Graminicola complex corresponding to the C3 and C4 photosynthetic pathways of their Poaceae hosts. The C4 lineage comprises several Colletotrichum species (including those later referred to as Caudatum subclade) that inhabit warm-season (C4) grasses, for example maize and sorghum. These species are apparently very host specific; they are exclusively associated with single host genera or species. In contrast, the C3 lineage, only consists of C. cereale, that has a wide host-range and had only been isolated from cool-season (C3) grasses. Later, Tao et al. (Citation2013) isolated six species belonging to the Graminicola complex, both from the C3 and the C4 lineage, for the first time from a plant host other than Poaceae (Bletilla ochracea, Orchidaceae). These species were however isolated as endophytes of this orchid, while they are pathogens of Poaceae hosts. In this study, C. cereale (C3 lineage) was isolated from both cool-season (C3, Lolium sp.) and warm-season (C4, Saccharum spontaneum) grasses with anthracnose symptoms or leaf spots. Moreover, the new species C. persicum was described in the C4 lineage from Phragmites australis, which is a very adaptable grass with characteristics both of the C3 and C4 pathways (Milke et al. Citation2020). These are further exceptions of the splitting of the Graminicola complex in a C3 and a C4 lineage. Moreover, the distant location of the C. cereale clade (C3 lineage) cannot be entirely confirmed with the phylogenies in this paper.
To our best of knowledge, this study comprises the first reports of C. cereale, C. nicholsonii and C. sublineola for the mycobiota of Iran. Saccharum spontaneum (C4) was identified as a common host of both C. sublineola and C. cereale. Moreover, after the differentiation of the strains from Eremochloae from C. sublineola and their recognition as a separate species, C. eremochloae by Crouch and Tomaso-Peterson (Citation2012), C. sublineola was regarded as only occurring on Sorghum species (Crouch and Tomaso-Peterson Citation2012; Xavier et al. Citation2018). In this study, C. sublineola was isolated not only from Sorghum halepense but also from Saccharum spontaneum that represents a new report of a host genus for a species of the C4 lineage of the Graminicola complex that was previously regarded as being restricted to one host genus or species each.
The newly described species C. caspicum is not restricted to Poaceae. The strains originated from at least two hosts belonging to different plant families, Poaceae and Cyperaceae, and in two countries in Asia, Iran and China. Results of blast searches based on ITS sequences indicate an even wider host spectrum and a further distribution of this species that might include Europe (Poland) and North America (USA) (Górzyńska et al. Citation2019; M.B. DeMers and G. May, unpubl. data). However, these results still need to be confirmed based on sequencing of further loci.
The systematic position of C. caspicum in the two phylogenies in this study is basal to all other species of the Graminicola complex, which also means it belongs neither to the C3 nor the C4 lineage. Blastn searches on NCBI GenBank indicate a close relationship of this species both to the Spaethianum and the Graminicola species complex. However, based on preliminary analyses, including the ex-type strains of this complex, C. caspicum does not belong to the Spaethianum complex, and the species was tentatively assigned to the Graminicola complex. However, individual species of most of the species in the Graminicola complex are not closely related; backbone support is missing or low (Marin-Felix et al. Citation2017; Bhunjun et al. Citation2021; this study). Therefore, the systematic position of all species of the Graminicola complex needs to be revised with a more complete sequence data set that would allow a better analysis of all Colletotrichum species combined.
ACKNOWLEDGMENTS
The authors highly appreciate the kind assistance of Bijan Aghapour, Department of Plant Protection, Baharan Institute of Higher Education, Gorgan, Iran and Dr. Adel Pordel, Plant Protection Research Department, Baluchestan Agricultural and Natural Resources Research and Education Centre, AREEO, Iranshahr, Iran, in collecting plant material for this study. We also exceedingly thank Dr. Vahid Khosravi, Rice Research Institute, Mazandaran Branch, Agricultural Research, Education and Extension Organization (AREEO), Amol, Iran, Dr. Mohammad Ali Aghajani, Department of Plant Pathology, Agriculture and Natural Resources Research Center of Golestan, Gorgan, Iran, Dr. Fereydoon Padasht Dehkaei, Rice Research Institute, Rasht, Guilan, Iran for assistance in accommodation in sampling trips and Dr. Rouhollah Amini, Department of Plant Ecophysiology, Faculty of Agriculture, University of Tabriz, Iran, for identifying of some of the host plants. The authors highly appreciate the great assistance of Petra Happel, Max Planck Institute for Terrestrial Microbiology, Marburg, Germany in setting up the experiments and for her helpful comments. Prof. Dr Uwe Braun, Institut für Geobotanik und Botanischer Garten, Martin-Luther-Universität Halle-Wittenberg, Germany, is thanked for verifying the Latin names. We gratefully acknowledge the Christian-Albrechts University of Kiel, Germany for financial supporting and providing materials.
DISCLOSURE STATEMENT
No potential conflict of interest was reported by the author(s).
Additional information
Funding
LITERATURE CITED
- Ahmad S, Iqbal SH, Khalid AN. 1997. Fungi of Pakistan. Sultan Ahmad mycological society of Pakistan. Lahore (Pakistan): Department of Botany, University of the Punjab.
- Alizadeh A, Javan-Nikkhah M, Zare R, Fotouhifar K-B, Damm U, Stukenbrock EH. 2015. New records of Colletotrichum species for the mycobiota of Iran. Mycol Iran. 2:95–109.
- Alvarez MG. 1976. Primer catálogo de enfermedades de plantas Mexicanas. Fitofilo. 71:1–169.
- Arzanlou M, Bakhshi M, Karimi K, Torbati M. 2015. Multigene phylogeny reveals three new records of Colletotrichum spp. and several new host records for the mycobiota of Iran. J Plant Prot Res. 55(2):198–211.
- Atghia O, Alizadeh A, Fotouhifar KB, Damm U, Stukenbrock EH, Javan-Nikkhah M. 2015. First report of Colletotrichum fructicola as the causal agent of anthracnose on common bean and cowpea. Mycol Iran. 2:139–140.
- Backman PA, Williams JC, Crawford MA. 1982. Yield losses in soybeans from anthracnose caused by Colletotrichum truncatum. Plant Dis. 66(1):1032–1034.
- Bhunjun CS, Phukhamsakda C, Jayawardena RS, Jeewon R, Promputtha I, Hyde KD. 2021. Investigating species boundaries in Colletotrichum. Fungal Divers. 13:1–21.
- Cai L, Hyde KD, Taylor PWJ, Weir BS, Waller JM, Abang MM, Zhang JZ, Yang YL, Phoulivong S, Liu ZY, et al. 2009. A polyphasic approach for studying Colletotrichum. Fungal Divers. 39:183–204.
- Cannon PF, Damm U, Johnston PR, Weir BS. 2012. Colletotrichum - current status and future directions. Stud Mycol. 73:181–213.
- Carbone I, Kohn LM. 1999. A method for designing primer sets for speciation studies in filamentous ascomycetes. Mycologia. 91(3):553–556.
- Crouch JA. 2014. Colletotrichum caudatum s. l. is a species complex. IMA Fungus. 5(1):1–30.
- Crouch JA, Beirn LA, Cortese LM, Bonos SA, Clarke BB. 2009a. Anthracnose disease of switchgrass caused by the novel fungal species Colletotrichum navitas. Mycol Res. 113(12):1411–1421.
- Crouch JA, Clarke BB, Hillman BI. 2006. Unraveling evolutionary relationships among the divergent lineages of Colletotrichum causing anthracnose disease in turfgrass and maize. Phytopathology. 96(1):46–60.
- Crouch JA, Clarke BB, Hillman BI. 2009b. What is the value of ITS sequence data in Colletotrichum systematics and species diagnosis? A case study using the falcate-spored graminicolous Colletotrichum group. Mycologia. 101(5):648–656.
- Crouch JA, Clarke BB, White JF, Hillma BI. 2009c. Systematic analysis of the falcate-spored graminicolous Colletotrichum and a description of six new species from warm season grasses. Mycologia. 101(5):717–732.
- Crouch JA, Tomaso-Peterson M. 2012. Anthracnose disease of centipedegrass turf caused by Colletotrichum eremochloae, a new fungal species closely related to Colletotrichum sublineola. Mycologia. 104(5):1085–1096.
- Crouch JA, Tredway LP, Clarke BB, Hillman BI. 2009d. Phylogenetic and population genetic divergence correspond with habitat for the pathogen Colletotrichum cereale and allied taxa across diverse grass communities. Mol Ecol. 18(1):123–135.
- Crous PW, Verkley GJM, Groenewald JZ, and Houbraken J, eds. 2019. Fungal biodiversity. Westerdijk laboratory manual series. Utrecht (The Netherlands): Westerdijk Fungal Biodiversity Institute.
- Damm U, Cannon PF, Liu F, Barreto RW, Guatimosim E, Crous PW. 2013. The Colletotrichum orbiculare species complex: important plant pathogens and mycoherbicides. Fungal Divers. 61:29–59.
- Damm U, Cannon PF, Woudenberg JHC, Crous PW. 2012a. The Colletotrichum acutatum species complex. Stud Mycol. 73:37–113.
- Damm U, Cannon PF, Woudenberg JHC, Johnston PR, Weir B, Tan YP, Shivas RG, Crous PW. 2012b. The Colletotrichum boninense species complex. Stud Mycol. 73:1–36.
- Damm U, Crous PW, Fourie PH. 2007. Botryosphaeriaceae as potential pathogens of Prunus species in South Africa, with descriptions of Diplodia africana and Lasiodiplodia plurivora spp. nov. Mycologia. 99:664–680.
- Damm U, O’Connell RJ, Groenewald JZ, Crous PW. 2014. The Colletotrichum destructivum species complex - hemibiotrophic pathogens of forage and field crops. Stud Mycol. 79:49–84.
- Damm U, Sato T, Alizadeh A, Groenewald JZ, Crous PW. 2019. The Colletotrichum draecaenophilum, C. magnum and C. orchidearum species complexes. Stud Mycol. 92:1–46.
- Damm U, Woudenberg JHC, Cannon PF, Crous PW. 2009. Colletotrichum species with curved conidia from herbaceous hosts. Fungal Divers. 39:45–87.
- Farr DF, Rossman AY. 2021. Fungal databases, U.S. National fungus collections, ARS, USDA. [Retrieved 2021 January 9]. Available online at https://nt.ars-grin.gov/fungaldatabases/.
- Farr ML, Stevenson JA. 1963. Eine Ergänzungsliste bolivianischer Pilze. Sydowia 17:37–69.
- Glass NL, Donaldson GC. 1995. Development of primer sets designed for use with the PCR to amplify conserved genes from filamentous ascomycetes. Appl Environ Microbiol. 61(4):1323–1330.
- Goh TK. 1999. Single-spore isolation using a hand-made glass needle. Fungal Divers. 2:47–63.
- Górzyńska K, Węgrzyn E, Sandecki R, Lembicz M. 2019. Endophytic fungi and latent pathogens in the sedge Carex secalina (Cyperaceae), a critically endangered species in Europe. Plant Prot Sci. 55:102–108.
- Grove WB. 1916. New or noteworthy fungi. Part V. J Bot. 54:217–223.
- Guerber JC, Liu B, Correll JC, Johnston PR. 2003. Characterization of diversity in Colletotrichum acutatum sensu lato by sequence analysis of two gene introns, mtDNA and intron RFLPs, and mating compatibility. Mycologia. 95(5):872–895.
- Hillis DM, Bull JJ. 1993. An empirical test of bootstrapping as a method for assessing confidence in phylogenetic analysis. Syst Biol. 42(2):182–192.
- Hirayama Y, Asano S, Okayama K, Ohki ST, Tojo M. 2018. Weeds as the potential inoculum source of Colletotrichum fructicola responsible for strawberry anthracnose in Nara, Japan. J Gen Plant Pathol. 84(1):12–19.
- Hyde KD, Abd-Elsalam K, Cai L. 2010. Morphology: still essential in a molecular world. Mycotaxon. 114(1):439–51.
- Hyde KD, Cai L, McKenzie EH, Yang YL, Zhang JZ, Prihastuti H. 2009b. Colletotrichum: a catalogue of confusion. Fungal Divers. 39:1–17.
- Jayawardena RS, Hyde KD, Damm U, Cai L, Liu M, Li XH, Zhang W, Zhao WS, Yan JY. 2016. Notes on currently accepted species of Colletotrichum. Mycosphere. 7(8):1192–1260.
- Keshavarzi M. 2021. An overview of ecological anatomy of Poaceae halophytes from Iran. In: Handbook of halophytes: from Molecules to ecosystems towards biosaline agriculture. Berlin/Heidelberg (Germany): Springer. p. 1035–1062.
- Lenné JM. 2002. Some major plant diseases. In: Waller JM, Lenné JM, Waller SJ, editors. Plant pathologist’s pocketbook. 3rd ed. Wallingford (UK): CABI. p. 4–18.
- Liu F, Cai L, Crous PW, Damm U. 2014. The Colletotrichum gigasporum species complex. Persoonia. 33:83–97.
- Liu F, Damm U, Cai L, Crous PW. 2013. Species of the Colletotrichum gloeosporioides complex associated with anthracnose diseases of Proteaceae. Fungal Divers. 61(1):89–105.
- Liu F, Weir BS, Damm U, Crous PW, Wang Y, Liu B, Wang M, Zhang M, Cai L. 2015. Unravelling Colletotrichum species associated with Camellia: employing ApMat and GS loci to resolve species in the C. gloeosporioides complex. Persoonia. 35:63–86.
- Liu PSW. 1977. A supplement to a host list of plant diseases in Sabah, Malaysia. Phytopathology. 21:1–49.
- Lubbe CM, Denman S, Cannon PF, Groenewald JZ, Lamprecht SC, Crous PW. 2004. Characterization of Colletotrichum species associated with diseases of Proteaceae. Mycologia. 96(6):1268–1279.
- Marin-Felix Y, et al. 2017. Genera of phytopathogenic fungi: GOPHY 1. Stud Mycol. 86:99–216.
- Mathur RS. 1979. The coelomycetes of India. Delhi (India): Bishen Singh Mahendra Pal Singh.
- Mendes MAS, Silva VL da, Dianese JC, Ferreira MAS, Santos CEN dos, Neto EG, Urben AF, Castro C. 1998. Fungos em plants no Brasil. Brasilia (Brazil): Embrapa-SPI/Embrapa-Cenargen.
- Messiaen M, Lafon R, Molot P. 1959. Nécroses de racines, pourritures de tiges et verse parasitaire du mais. Annales des Épiphyties. 10:441–474.
- Milke J, Gałczyńska M, Wróbel J. 2020. The importance of biological and ecological properties of Phragmites australis. Cav. Trin. Ex Steud., in phytoremendiation of aquatic ecosystems – the review. Water. 12:1770.
- Moriwaki J, Tsukiboshi T. 2009. Colletotrichum echinochloae, a new species on Japanese barnyard millet. Echinochloa utilis. Mycoscience. 50(4):273–280.
- Moriwaki J, Tsukiboshi T, Sato T. 2002. Grouping of Colletotrichum species in Japan based on rDNA sequences. J Gen Plant Pathol. 68(4):307–320.
- Nirenberg HI. 1976. Untersuchungen über die morphologische und biologische Differenzierung in der Fusarium-Sektion Liseola. Mitteilungen aus der Biologischen Bundesanstalt für Land- und Forstwirtschaft Berlin-Dahlem. 169:1–117.
- Noroozi J, Moser D, Essl F. 2016. Diversity, distribution, ecology and description rates of alpine endemic plant species from Iranian mountains. Alp Bot. 126(1):1–9.
- Nylander JAA. 2004. MrModeltest v2, program distributed by the author. Uppsala (Sweden): Evolutionary Biology Centre, Uppsala University.
- O’Connell RJ, et al. 2012. Lifestyle transitions in plant pathogenic Colletotrichum fungi deciphered by genome and transcriptome analyses. Nat Genet. 44(9):1060–1065.
- O’Donnell K, Cigelnik E. 1997. Two divergent intragenomic rDNA ITS2 types within a monophyletic lineage of the fungus Fusarium are nonorthologous. Mol Phylogenet Evol. 7:103–116.
- Owfi RE. 2020. Natural products and botanical medicines of Iran. Boca Raton (FL): CRC Press.
- Phoulivong S, Cai L, Chen H, McKenzie EH, Abdelsalam K, Chukeatirote E, Hyde KD. 2010. Colletotrichum gloeosporioides is not a common pathogen on tropical fruits. Fungal Divers. 44(1):33–43.
- Prihastuti H, Cai L, Chen H, McKenzie EHC, Hyde KD. 2009. Characterization of Colletotrichum species associated with coffee berries in northern Thailand. Fungal Divers. 39:89–109.
- Prihastuti H, Cai L, Hyde KD. 2010. Neotypification of Colletotrichum falcatum, the causative agent of red-rot disease in sugarcane. Sydowia. 62:283–293.
- Rambaut A. 2002. Sequence alignment editor. Version 2.0. Oxford (UK): University of Oxford.
- Rojas EI, et al. 2010. Colletotrichum gloeosporioides s.l. associated with Theobroma cacao and other plants in Panama: multilocus phylogenies distinguish pathogen and endophyte clades. Mycologia. 102:1318–1338.
- Ronquist F, Huelsenbeck JP. 2003. MrBayes 3: Bayesian phylogenetic inference under mixed models. Bioinformatics. 19:1572–1574.
- Roy KW. 1982. Seedling diseases caused in soybean by species of Colletotrichum and Glomerella. Phytopathology. 72:1093–1096.
- Sambrook J, Russel DW. 2001. Molecular cloning: a laboratory manual. New York (USA): Cold Spring Harbor Laboratory.
- Simmonds JH. 1965. A study of the species of Colletotrichum causing ripe fruit rots in Queensland. Queensland J Agric Animal Sci. 22:437–459.
- Smith BJ, Black LL. 1990. Morphological, cultural and pathogenic variation among Colletotrichum species isolated from strawberry. Plant Dis. 74:69–76.
- Sutton BC. 1980. The coelomycetes. Fungi imperfecti with pycnidia, acervuli and stromata. Kew (UK): CABI.
- Sutton BC. 1992. The genus Glomerella and its anamorph Colletotrichum. In: Bailey JA, Jeger MJ, editors. Colletotrichum: biology, pathology and control. Wallingford (UK): CABI. p. 1–26.
- Swofford DL. 2003. PAUP*: phylogenetic analysis using parsimony (*and other methods). Version 4.0b 10. Sunderland (MA): Sinauer Associates.
- Tao G, Liu ZY, Liu F, Gao YH, Cai L. 2013. Endophytic Colletotrichum species from Bletilla ochracea. Orchidaceae), with descriptions of seven new species. Fungal Divers. 61:139–164.
- Than PP, Jeewon R, Hyde KD, Pongsupasamit S, Mongkolporn O, Taylor PWJ. 2008a. Characterization and pathogenicity of Colletotrichum species associated with anthracnose on chilli (Capsicum spp.) in Thailand. Plant Pathol. 57:562–572.
- Than PP, Shivas RG, Jeewon R, Pongsupasamit S, Marney TS, Taylor PW, Hyde KD. 2008b. Epitypification and phylogeny of Colletotrichum acutatum J.H. Simmonds. Fungal Divers. 28:97–108.
- Thaung MM. 2008. Coelomycete systematic with special reference to Colletotrichum. Mycoscience. 49:345–350.
- Viswanathan R, Premachandran MN, Balamuralikrishnan M, Jothi R. 2003. A new stalk rot disease of sugarcane caused by Phaeocytostroma sacchari in India. Sugar Tech. 5:61–64.
- Von Arx JA. 1957. Die Arten der Gattung Colletotrichum Cda. Phytopathologische Zeitschrift. 29:413–468.
- Weir B, Johnston PR, Damm U. 2012. The Colletotrichum gloeosporioides species complex. Stud Mycol. 73:115–180.
- White TJ, Bruns T, Lee S, Taylor J. 1990. Amplification and direct sequencing of fungal ribosomal RNA genes for phylogenetics. In: Innis MA, Gelfand DH, Sninsky JJ, and White TJ, editors. PCR protocols: a guide to methods and applications. San Diego (USA): Academic Press. p. 315–322.
- Wong MKM, Hyde KD. 2001. Diversity of fungi on six species of Gramineae and one species of Cyperaceae in Hong Kong. Mycol Res. 105:1485–1491.
- Xavier KV, Mizubuti ESG, Queiroz MV, Chopra S, Vaillancourt L. 2018. Genotypic and pathogenic diversity of Colletotrichum sublineola isolates from sorghum (Sorghum bicolor) and johnsongrass (S. halepense) in the Southeastern United States. Plant Dis. 102:2341–2351.
- Yan JY, Jayawardena MM, Goonasekara ID, Wang Y, Zhang W, Liu M, Huang JB, Wang ZY, Shang JJ, Peng YL, Bahkali A. 2015. Diverse species of Colletotrichum associated with grapevine anthracnose in China. Fungal Divers. 71:233–246.
- Zhang W, Damm U, Crous PW, Groenewald JZ, Niu X, Lin J, Li Y. 2020. Anthracnose disease of carpetgrass (Axonopus compressus) caused by Colletotrichum hainanense sp. nov. Plant Dis. 104:1744–1750.