ABSTRACT
The importance of mushrooms as a food source is continually increasing. To investigate how environmental factors affect the nutritional value of mushrooms, we harvested them in eastern Poland, south-central Germany, and northwestern Belgium in plots with similar environmental conditions but varying in tree species composition and richness. We used gas chromatography–mass spectrometry (GC-MS) to analyze the fatty acid (FA) content of the mushrooms. Fungal species identity explained the largest part (40%) of the total variation in FA concentration and composition. Environmental factors accounted for 1–12% of variation. The concentration of FA, especially saturated fatty acids, decreased with increasing understory cover and increasing nitrogen concentration in the topsoil. The effect of tree species richness or tree species identity was negligible. Our results suggest that the nutritional value of mushrooms depends mainly on the species identity of fungi, but that their FA content is slightly higher in forests with less undergrowth and in nitrogen-poor soils.
INTRODUCTION
Mushrooms are a key source of food and income in many parts of the world due to their wide availability and significant nutritional value (Monarrez-Gonzalez et al. Citation2020; Valverde et al. Citation2015). Mushroom picking is also an important cultural/traditional practice (Bernaś et al. Citation2022). In recent years, the mushroom market has been thriving and has grown more than 30-fold since 1978 and in 2013 exceeded 4.7 kg of mushrooms consumed per capita (compared, e.g., with 1 kg in 1997) (Royse et al. Citation2017). Due to growing awareness of the health benefits of mushrooms, positive attitudes toward natural products, and environmental concerns, it is estimated that the market of mushroom-based products will continue to grow (Kumar et al. Citation2022; Niazi and Ghafoor Citation2021; Veljović and Krstić Citation2020).
Wild mushrooms are also considered a valuable source of nutrition due to their high concentration of vitamins and antioxidants, complete amino acid profile, and high fiber content (Valverde et al. Citation2015). They also have a low dry matter content, estimated at 5–12% of their fresh mass (Bano and Rajarathnam Citation1988; Kalač Citation2009), active chemicals, and low content of lipids, making them ideal element of antidiabetic diet (Dubey et al. Citation2019).
Two to six percent of mushroom dry mass are lipids—mainly fatty acids (FA), which regulate intracellular signaling pathways and expression of bioactive lipid mediators in the human body (Calder Citation2015; Nagy and Tiuca Citation2017). Despite of their low FA content in dry mass, mushrooms can contain even 37 types of fatty acids (Doğan and Akbaş Citation2013), with palmitic (16:0), oleic (18:1), linoleic (18:2), linolenic (C18:3), and stearic (C18:0) acids occurring in significant amounts (Kalač Citation2013; Sande et al. Citation2019).
Unsaturated fatty acids (UFA), characterized by at least one double bond in their structure, make up an average of 70% of all fatty acids contained in mushrooms (mainly by linoleic, linolenic, and oleic acids) (Sande et al. Citation2019). UFA take part in many metabolic processes and regulate the antioxidant signaling pathway and inflammatory processes (Calder Citation2015). Polyunsaturated FA (PUFA), which contain two or more double bonds in their structure, play an important role in the formation of high-density lipoprotein (HDL) in the human body, which transports fat from the blood to the liver, reducing the risk of cardiovascular disorders, incidence of stroke, and atherosclerosis and fighting against obesity and diabetes mellitus type 2 (Sokoła-Wysoczańska et al. Citation2018). For these reasons, it is worth considering mushrooms as an important source of unsaturated fatty acids, especially for persons concerned about high caloric values or high cholesterol content in the diet.
Many studies demonstrate high variability in the content of fatty acids among mushroom species (Ayaz et al. Citation2011; Günç Ergönül et al. Citation2013; Isik Citation2020; Ribeiro et al. Citation2009). Dimitrijevic et al. (Citation2018) analyzed the composition of fatty acids in 12 edible mushroom species and showed that percentage of linoleic acid fluctuated between 20% and 72%, oleic acid between 0.11% and 41%, and stearic acid between 0.8% and 57%, depending on the species.
Most studies concerning FA content in mushrooms concentrate on cultivated mushrooms, with the aim of enhancing their nutritional value (among them FA content) in controlled conditions (e.g., Mandeel et al. Citation2005; Patil et al. Citation2010; Vidhyalakshmi et al. Citation2017). According to these studies, substrate traits modify the FA content in mushrooms. Vidhyalakshmi et al. (Citation2017) demonstrated the highest lipid content in mushrooms grown on straw, and Patil et al. (Citation2010) found soybean straw to be the best substrate for enhancing fat content in mushrooms. Diamantopoulou et al. (Citation2016) demonstrated the importance of C/N ratio of the substrate for UFA content in sporocarps of Volvariella volvacea (Bull.) Singer. They revealed that mushrooms grown on a substrate with higher C/N ratios (C/N = 40 or 60 compared with C/N = 20 or 30) contain more UFA (mainly linoleic acid). Further, the content of stearic acid increased during fungal growth in conditions of high C/N ratio (C/N = 60). Zhang et al. (Citation2022) showed that factors such as incubation temperature, light, pH, and C/N ratio affect fatty acid production in cultivated oleaginous fungi. Similar factors may also influence the synthesis of FA in wild-growing mushrooms, but despite a number of papers demonstrating large differences in the content of specific fatty acids between regions, substrates, and species, knowledge of environmental factors responsible for this variability remains scarce.
Among poorly explored factors are tree stand characteristics. Tree species can affect the quality of the forest floor by altering litter characteristics, e.g., C/N ratio or pH, due to differences in elemental and biochemical contents of tree foliage (Binkley and Giardina Citation1998; Joly et al. Citation2016; Kooch et al. Citation2017; Tajik et al. Citation2019). Tree species also influence symbiotic relationships through the transfer of carbohydrates (products of photosynthesis) to mycorrhizal fungi (Seyfried et al. Citation2021). Forest canopy exerts a buffering effect on under-canopy microclimate: temperature and water availability (Zellweger et al. Citation2020), but also limits access to light, which potentially may influence FA content in mushrooms.
In our study, we collected 10 species of mushrooms from forests differing in tree species richness and identity, growing in similar habitat conditions, and analyzed their FA content composition. We investigated how (i) species of mushroom, (ii) tree species richness and composition, and (iii) type of site (experimental tree plantation vs. natural forest) affect content and composition of fatty acids in mushrooms. We hypothesized that (i) FA content and composition will be affected mostly by mushroom species identity (Dimitrijevic et al. Citation2018); (ii) content of FA will be lower in deciduous stands than in coniferous due to lower C/N ratio of the deciduous litter (Jacob et al. Citation2009); (iii) composition of FA in mushrooms derived from plots characterized by higher tree richness will be more similar to each other than composition of FA of mushrooms from monocultures, as monocultures of different species create more specific environmental conditions than do mixtures of trees.
MATERIALS AND METHODS
Study area.—
Our study was conducted in three European regions: in old-growth Białowieża Primeval Forest (eastern Poland) (52.70°N, 23.85°E) and in two tree diversity experiments—Kaltenborn (central Germany) (50.41°N, 7.02°E) and Zedelgem (northeastern Belgium) (51.13°N, 3.13°E). Białowieża Primeval Forest (BPF) is one of the best-preserved forests in Europe, stretching over the border between Poland and Belarus. It lies in the zone of hemiboreal and mixed broadleaved-coniferous forests (Jaroszewicz et al. Citation2019). Our plots in BPF were established as part of the European Union (EU)-funded FunDivEUROPE project (Baeten et al. Citation2013) to study the effect of tree diversity on ecosystem multifunctionality. Study plots were set in mature mixed deciduous forests of the oak-lime-hornbeam type (Tilio-Carpinetum), without signs of silvicultural activities for the last 10–20 years. The 43 study plots (30 × 30 m) varied in tree species richness (1–5 tree species), including various mixtures of Pinus sylvestris L., Quercus robur L., Picea abies L., Betula pendula Roth, and Carpinus betulus L., but were similar in terms of other environmental conditions (i.e., topography, geology, soil type) to minimize the impact of confounding factors (Baeten et al. Citation2013). In this study, we used a subset of 20 plots (1–4 tree species), excluding all plots containing P. abies, which was subject to intensive dieback caused by the spruce bark beetle (Ips typographus L.) outbreak during the years 2015–2019.
The two experimental tree plantations: Kaltenborn (part of the BIOTREE experiment; Scherer-Lorenzen et al. Citation2007) and Zedelgem (part of the FORBIO experiment; Verheyen et al. Citation2013), are young forest plantations established on postagricultural land to study the effect of tree species diversity on ecosystem multifunctionality (Kaltenborn in 2003/2004; Zedelgem between 2009 and 2012). Different tree communities were planted to create a gradient of tree species richness under homogeneous environmental conditions to minimize the effect of uncontrolled factors.
In Kaltenborn, the main species are Fagus sylvatica L., Pseudotsuga menziessii (Mirb.) Franco, Quercus petraea (Matt.) Liebl., and Picea abies H. Karst. Mushrooms were collected from eight plots without management treatment—four monocultures of these species and four three-species mixtures (Scherer-Lorenzen et al. Citation2007).
In Zedelgem, mushrooms were harvested from 20 plots—10 plots covered by monocultures of B. pendula, F. sylvatica, Pinus sylvestris, Tilia cordata Mill., and Q. robur and 10 plots covered by three-species mixtures of these species. In this site, there is no management treatment (Verheyen et al. Citation2013).
Sampling design.—
In Białowieża Forest, mushrooms were collected over two years (2020–2021) of mushroom monitoring (Stojek et al. Citation2022). In Kaltenborn and Zedelgem, mushrooms were sampled twice, during the peak production of sporocarps: in October and November 2020. At all sites, we collected sporocarps of all soil-dwelling and epixylic macrofungi, excluding bracket fungi and those with sporocarp size <0.5 cm. They were brought to the laboratory for cleaning, weighting, and species identification. The mushroom species were determined to the lowest possible taxonomic level, using available online keys and atlases (www.grzyby.pl and www.nagrzyby.pl), a book, Fungi of temperate Europe, Volumes 1 and 2 (Laessoe and Petersen Citation2019), and expertise of local professionals. Based on these atlases, we categorized mushrooms into edible and nonedible species. All species of Armillaria genus were aggregated into the collective species Armillaria mellea agg. due to difficulties in their determination based just on morphological traits. Edible mushrooms were dried in a food dehydrator at 50 C for approximately 24 h, and samples of species present in five or more plots at each site were sent to the laboratory of the Department of Bromatology of Warsaw Medical University for estimation of content and composition of fatty acids.
Forest stand and soil characteristics.—
The plot-level forest inventory data for plots in Białowieża and Kaltenborn were obtained from FunDivEUROPE and SoilForEurope projects data portal (https://data.botanik.uni-halle.de/fundiveurope). Data on tree species and tree diameter at breast height were collected in 2018. C/N ratio and nitrogen concentration of the soil were measured in 2012 (Dawud et al. Citation2016). We used data from 0–10 cm depth. Soil parameters in Zedelgem were defined using the same method in 2018–2019.
In June 2020, we visually estimated percentage tree canopy cover and carried out the understory vegetation survey in BPF and Kaltenborn plots using the six-step Braun-Blanquet cover-abundance method (Braun-Blanquet Citation1928). The understory was defined as a vegetation layer consisting of all herbaceous plants irrespective of their height, and trees and shrubs lower than 0.5 m. Data on understory and tree canopy cover in Zedelgem were collected in 2018 and 2020, respectively.
Based on stem basal area, the share of each tree species in plots was estimated. Based on the same data, the proportion of deciduous trees at plot level was calculated.
Analysis of fatty acid content.—
Powdered dried mushrooms in the amount of 100 mg were extracted for 30 min in an ultrasonic bath using 9 mL of chloroform and with the addition of 25 µL of internal standard (C19:0 in toluene, 1 mg/mL). The resulting mixture was evaporated to dryness with nitrogen.
Extracted samples were hydrolyzed with 1 mL of 0.5 M NaOH methanolic solution at 80 C for 15 min, and then a complete derivation was carried with 1 mL of BF3 solution in methanol (14% w/v) at 80 C for 15 min. Then, 2 mL of NaCl was added to the sample. The methyl esters were extracted with 2 mL of hexane. The upper layer was taken and dried with an anhydrous Na2SO4. The pure liquid was transferred to inserts for gas chromatography–mass spectrometry (GC-MS) analysis. Analyses were performed on a gas chromatograph with time-of-flight mass spectrometry (GC-TOF-MS) (Pegasus BT; LECO, St. Joseph, Michigan). Chromatographic separations were conducted on a TR-FAME capillary column (120 m/0.25 mm ID/film thickness 0.25 µm; Thermo Fisher Scientific, Göteborg, Sweden). Helium was used as the carrier gas (flow: linear velocity at 1 mL/min); the injection was 1 µL, in the splitless mode. The injector and transfer line were heated to 240 C.
The temperature program: initial temperature was set at 110 C for 16 min, then increased 6 C/min to 190 C, 1 C/min to 210 C, and 0.4 C/min to 220 C, and finally held for 10 min at 220 C. Ion source temperature was 250 C and energy 70 eV. To determine the FA types contained in the samples, the fatty acid methyl ester (FAME) standards (Supelco 37 Component FAME Mix; Sigma, St. Louis, Missouri) were applied. TChromaTOF software was then used for the identification and quantitative analysis of fatty acids.
In total, we identified 34 fatty acids (from C8:0 to C24:1), which were grouped as saturated fatty acids (SFA), monounsaturated fatty acids (MUFA), and polyunsaturated fatty acids (PUFA).
Statistical analysis.—
Detrended correspondence analysis (DCA) was employed to explore the patterns in FA composition between samples. We used the vegan::decorana() function to conduct DCA (Oksanen et al. Citation2020). To assess the correlations of the main gradient of FA composition between samples, revealed by DCA, through a passive fit of the examined environmental factors (N concentration, C/N ratio, canopy cover, understory cover, tree species richness deciduous trees proportion), we used the vegan::envfit() function (Oksanen et al. Citation2020). The strength of these relationships was evaluated based on the determination coefficient (R2) and P-values, computed for each vector representing the environmental factor, obtained from a permutation test with 999 iterations (vegan::envfit() function; Oksanen et al. Citation2020).
To explore the relationship between concentration of FA (ALL FA [total FA], PUFA, MUFA, SFA) in samples of mushrooms and environmental factors (N concentration, C/N ratio, understory cover, tree richness, canopy cover, deciduous trees proportion) for each group of FA, we built one global linear regression model (LM) (lme4::lm()) with all hypothesized predictors. The mushroom species collected from particular site (species_site) was used as one of the predictors, as mushroom species was a key variable explaining differences between mushrooms and results of the same species but collected in different sites were significantly different. At the same time, mushroom species rarely repeated between sites, making it impossible to use “site” as a separate factor.
All response variables have close-to-normal distribution, except MUFA concentration—for this variable we applied log10 transformation. The collinearity among predictors was inspected, using car::vif. It revealed that in all models, except model explaining content of SFA, C/N ratio was collinear (variance inflation factor [VIF] > 5) and was rejected (Fox et al. Citation2017). Outliers were inspected using car::outlierTest (Fox and Weisberg Citation2017). From all model combinations, we chose the model with change in corrected Akaike’s information criterion (ΔAICc) < 2 as equivalent, using the MuMIn::dredge() function (Bartoń Citation2020). For each group of FA (ALL FA, SFA, PUFA, MUFA, UFA), we performed one final LM regression composed of all predictors included in the subset of LMs with ΔAICc < 2. We evaluated the final model using analysis of variance (ANOVA) (car::Anova(); Fox and Weisberg Citation2017). The results of final LMs were visualized based on marginal responses (predicted values, assuming that other explanatory variables are at a constant level) and Tukey posteriori tests, using ggeffects::ggpredict() (Lüdecke et al. Citation2018), emmeans::emmeans() (Lenth et al. Citation2023), and multcomp::cld (Hothorn, Torsen et al. Citation2008). To avoid the risk of treating ecologically relevant patterns as statistically not significant, we accounted for the effect size and biological significance of the obtained results rather than for statistical significance (Nakagawa and Cuthill Citation2007; Wasserstein and Lazar Citation2016). Furthermore, we estimated the independent contribution of each variable in a multivariate model, using hierarchical partitioning of marginal R2 for generalized mixed-effect models (glmm.hp::glmm.hp(); Lai et al. Citation2022).
All statistical analyses were carried out in R 4.1.2 (R Core Team Citation2021), using the RStudio 2022.2.0.44 environment (RStudio Team Citation2022).
RESULTS
Composition of FA in mushrooms.—
DCA ordination revealed that the composition of FA in mushrooms was predominantly influenced by mushroom species. Cohesive clustering was observed among samples from the same mushroom species, and the variability was primarily explained by this factor (; ).
Figure 1. Results of detrended correspondence analysis (DCA) visualizing differences in the concentration of 34 fatty acids in sporocarps of 10 mushrooms species from particular sites and plots. Points represent samples of mushroom species from a particular plot and site. Different symbols represent particular mushroom species collected at different sites: Am = Armillaria mellea; Lv = Lactarius vellereus; Lp = Lycoperdon perlatum; Mp = Macrolepiota procera; Rb = Rhodocollybia butyracea; Rn = Russula nigrescens; Rc = Russula cyanoxantha; Xch = Xerocomellus chrysenteron; Ll = Laccaria laccata; Lpr = Laccaria proxima). Sites: B = Białowieża (Poland); Z = Zedelgem (Belgium); K = Kaltenborn (Germany). Black arrows show the passive projection of soil and tree stand characteristics correlated with DCA result: canopy_cov = canopy cover; C_N = carbon to nitrogen ratio; DeciProp = deciduous trees proportion; tree_rich = tree species richness; Nconc = Nitrogen concentration; understory_cov = understory cover.
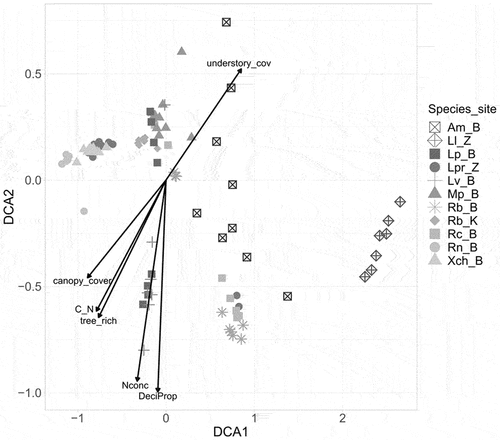
Table 1. Environmental factors explaining differences in the composition of 34 fatty acids in sporocarps of 10 mushroom species collected in Białowieża Primeval Forest (eastern Poland), Kaltenborn (central Germany), and Zedelgem (northeastern Belgium).
Of the studied environmental factors, understory cover and canopy cover emerged as statistically significant contributors, explaining the highest proportion of variation (14% and 7%, respectively) in the sporocarp FA composition. The influence of C/N ratio, N concentration, deciduous trees proportion, and tree richness were comparatively modest, each explaining less than 5% of the observed variation ().
Factors affecting content of FA.—
The differences in the concentration of all of the studied groups of FA (ALL FA, SFA, PUFA, MUFA, UFA) were best explained by the mushroom species (explaining close to 40% of variation in ALL FA, SFA, PUFA, and UFA and 83% of variation in MUFA), whereas soil and tree stand characteristics played a much smaller role ().
Table 2. Linear models explaining concentration of fatty acids (ALL FA, SFA, PUFA, MUFA; µg/100 g dry mass) in sporocarps of 10 mushroom species collected in Białowieża Primeval Forest (eastern Poland), Kaltenborn (central Germany), and Zedelgem (northeastern Belgium).
Concentration of ALL FA differed highly between mushroom species, with the highest average content observed in Xerocomellus chrysenteron and Armillaria mellea (7597 ± 588 [SE] and 7599 ± 738 [SE] µg/100 mg dry mass) and the lowest in Lactarius vellereus (3432 ± 959 [SE] µg/100 mg dry mass) and Lycoperdon perlatum (2187 ± 750 [SE] and 2794 ± 959 [SE] µg/100 mg dry mass in samples collected in Białowieża and Zedelgem, respectively). L. perlatum (Zedelgem and Białowieża) and Rhodocollybia butyracea (Kaltenborn and Białowieża) were each collected at two sites. L. perlatum from both sites contained similar total amount of FA, whereas samples of R. butyracea from Kaltenborn contained nearly 2 times more FA than samples from Białowieża (7184 and 4027 µg/100 mg dry mass, respectively) (). Apart from mushroom species, soil nitrogen concentration and understory cover negatively influenced concentration of ALL FA (). The total content of FA increased with decreasing nitrogen concentration from 6992 µg/100 mg at 2.2 mg N/g of soil dry mass to 8292 µg/100 mg at 1.1 mg N/g. Increasing understory cover from 0% to 100% decreased the total content of FA from 8180 to 6370 µg/100 mg dry mass ().
Figure 2. Partial regression plots visualizing mean (+ SE) differences in the concentration of five groups of fatty acids (ALL FA = all fatty acids; SFA = saturated fatty acids; PUFA = polyunsaturated fatty acids; MUFA = monounsaturated fatty acids; UFA = unsaturated fatty acids [PUFA = MUFA]; µg/100 g dry mass) between 10 mushroom species collected at different sites: Am = Armillaria mellea; Lv = Lactarius vellereus; Lp = Lycoperdon perlatum; Mp = Macrolepiota procera; Rb = Rhodocollybia butyracea; Rn = Russula nigrescens; Rc = Russula cyanoxantha; Xch = Xerocomellus chrysenteron; Ll = Laccaria laccata; Lpr = Laccaria proxima). Sites: B = Białowieża (Poland); Z = Zedelgem (Belgium); K = Kaltenborn (Germany). Groups marked by the same letter do not differ significantly at P = 0.05, according to pairwise comparisons of least-squares means (lsmeans::cld). For the remaining partial regression plots visualizing influence of soil and tree stand characteristics on the concentration of groups of FA in sporocarps, see and SUPPLEMENTARY FIGS. 1 and 2.
![Figure 2. Partial regression plots visualizing mean (+ SE) differences in the concentration of five groups of fatty acids (ALL FA = all fatty acids; SFA = saturated fatty acids; PUFA = polyunsaturated fatty acids; MUFA = monounsaturated fatty acids; UFA = unsaturated fatty acids [PUFA = MUFA]; µg/100 g dry mass) between 10 mushroom species collected at different sites: Am = Armillaria mellea; Lv = Lactarius vellereus; Lp = Lycoperdon perlatum; Mp = Macrolepiota procera; Rb = Rhodocollybia butyracea; Rn = Russula nigrescens; Rc = Russula cyanoxantha; Xch = Xerocomellus chrysenteron; Ll = Laccaria laccata; Lpr = Laccaria proxima). Sites: B = Białowieża (Poland); Z = Zedelgem (Belgium); K = Kaltenborn (Germany). Groups marked by the same letter do not differ significantly at P = 0.05, according to pairwise comparisons of least-squares means (lsmeans::cld). For the remaining partial regression plots visualizing influence of soil and tree stand characteristics on the concentration of groups of FA in sporocarps, see FIGS. 3–5 and SUPPLEMENTARY FIGS. 1 and 2.](/cms/asset/a2d0c497-7e0b-4346-bd22-879f7f1c9423/umyc_a_2325045_f0002_b.gif)
Figure 3. Partial regression plots visualizing influence of soil and tree stand characteristics on the concentration of all fatty acids (ALL FA; µg/100 g dry mass) in sporocarps of Armillaria mellea, Lactarius vellereus, Lycoperdon perlatum, Macrolepiota procera, Rhodocollybia butyracea, Russula nigrescens, Russula cyanoxantha, Xerocomellus chrysenteron, Laccaria laccata, and Laccaria proxima collected in Białowieża (Poland), Zedelgem (Belgium), and Kaltenborn (Germany). For partial regression plots showing mean (+ SE) differences in the content of fatty acids among fungi species, see .
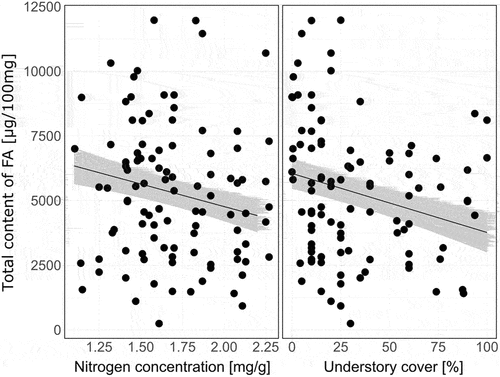
Figure 4. Partial regression plots visualizing influence of soil and tree stand characteristics on the concentration of saturated fatty acids (SFA; µg/100 g dry mass) in sporocarps of Armillaria mellea, Lactarius vellereus, Lycoperdon perlatum, Macrolepiota procera, Rhodocollybia butyracea, Russula nigrescens, Russula cyanoxantha, Xerocomellus chrysenteron, Laccaria laccata, and Laccaria proxima collected in Białowieża (Poland), Zedelgem (Belgium), and Kaltenborn (Germany). For partial regression plots showing mean (+ SE) differences in the content of fatty acids among fungi species, see .
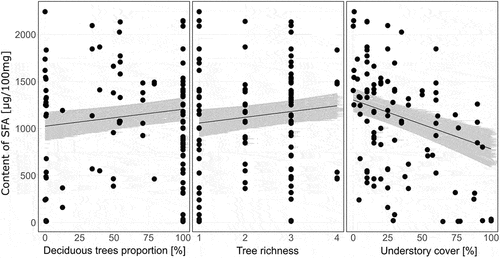
Understory cover, and in a much smaller extent proportion of deciduous trees, and tree richness partially explained differences in concentration of SFA between samples. The highest effect size was revealed for understory cover: the SFA content increased from 784 µg/100 mg dry mass at 100% of understory cover to 1305 µg/100 mg dry mass at 0% of understory cover. Effect size of other factors was faint. The SFA content increased from 1059 µg/100 mg dry mass in monoculture to 1242 µg/100 mg dry mass in four-species mixtures and from 1026 µg/100 mg dry mass in coniferous stand to 1205 µg/100 mg dry mass in purely deciduous stand ().
The concentration of PUFA increased with decreasing nitrogen concentration (from 2554 µg/100 mg at 2.2 mg N/g to 3514 µg/100 mg at 1.1 mg N/g) and with decreasing understory cover (from 2548 µg/100 mg dry mass at 100% of understory cover to 3216 µg/100 mg dry mass at 0%) (; ). Effect of environmental factors on MUFA and UFA concentrations was negligible. Deciduous tree proportion and understory cover explained less than 1% of variation in MUFA. N concentration and understory cover also explained less than 2% of variation in UFA.
Figure 5. Partial regression plots visualizing influence of soil and tree stand characteristics on the concentration of polyunsaturated fatty acids (PUFA; µg/100 g dry mass) in sporocarps of Armillaria mellea, Lactarius vellereus, Lycoperdon perlatum, Macrolepiota procera, Rhodocollybia butyracea, Russula nigrescens, Russula cyanoxantha, Xerocomellus chrysenteron, Laccaria laccata, and Laccaria proxima collected in Białowieża (Poland), Zedelgem (Belgium), and Kaltenborn (Germany). For partial regression plots showing mean (+ SE) differences in the content of fatty acids among fungi species, see .
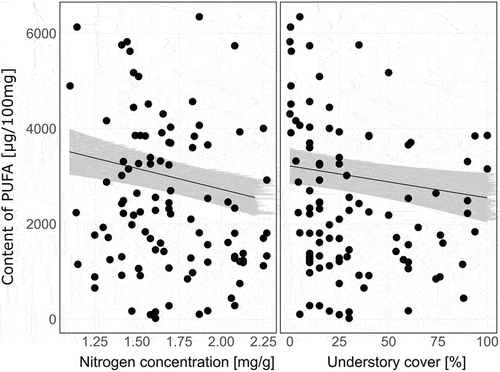
DISCUSSION
Fungal species was the key factor explaining most of the variation in mushroom fatty acid concentration (82% of variation of MUFA, around 40% of other types of FA). This is in accordance with many studies (Ayaz et al. Citation2011; Barros et al. Citation2008; Günç Ergönül et al. Citation2013; Isik Citation2020; Ribeiro et al. Citation2009), reporting high differences between mushroom species. Ribeiro et al. (Citation2009) showed 10 times higher total concentration of FA in Suillus luteus than in Amanita rubescens, whereas Kalač (Citation2013) reported even higher differences—more than 80-fold difference in the total content of fatty acids between mushroom species, with the lowest FA content in Agaricus campestris L. (1.1 g/kg dry mass) and the highest in Lactarius deliciosus (L.) Pers. (80.2 g/kg dry mass). In our study, the differences were not so large: the lowest content of ALL FA was obtained from samples of L. perlatum (21.3 g/kg dry mass) and the highest from samples of A. mellea (75.1 g/kg dry mass). These values were comparable to those reported in the review by Kalač (Citation2013) for another Lycoperdon species (L. echinatum, 12.2 g/kg dry mass) and for A. mellea (55.6 g/kg dry mass). L. perlatum was not included in the review.
Environmental factors explained much less of the variability in fatty acid content of mushrooms than the mushroom species effect. Among the studied factors, understory cover had the strongest (negative) effect on the content of all studied groups of FA (ALL FA, SFA, PUFA, MUFA, UFA) and modulated their composition in the studied mushrooms (see ). There are very few studies about the influence of any environmental factors on the FA content of fungi, and none have investigated possible interactions between forest understory cover and FA content. However, we also found that the levels of ALL FA, PUFA, and UFA, the types of FA that were negatively affected by understory cover, were also negatively affected in parallel by soil nitrogen concentration. Thus, we hypothesize here that the revealed negative interaction between understory cover and content of FA in sporocarps could be due to competition for nitrogen between yielding sporocarps of ectomycorrhizal fungi and understory plants. Most of the understory plants form arbuscular mycorrhizal associations (Luginbuehl et al. Citation2017; Pfeffer et al. Citation1999) with fungal species not developing fruiting bodies (Perez-Moreno et al. Citation2021); therefore, they were not considered in our study. This hypothesis is, in our opinion, supported by the studies on environmental factors affecting lipid content in microorganisms. There are very few of them, and most of these studies have been conducted on oleaginous microalgae, cultivated as renewable oil sources; however, they report that nitrogen limitation induces lipid production as the carbon and energy reserves (antistress response) (Jacobsen et al. Citation2008; Yu et al. Citation2019). This may explain our findings on the negative influence of nitrogen concentration on FA concentration in mushrooms, if there is sufficient similarity in the metabolism of microalgae and fungi. However, further field and experimental research is needed to explain the negative influence of nitrogen concentration and the negative influence of understory plant cover on FA concentration in mushrooms.
The environmental factors examined in our analyses affected only slightly the content of FA, explaining 1–12% of total variance (depending on the group of FA). This low proportion of variance explained can be caused by high differences in the content of FA between mushroom species, but also by impact of many other factors such as humidity or season (changes in day length and exposure to the light), which were not taken into account in our study. The importance of these factors for FA production was described in some yeast and microalgae experiments (Amini Khoeyi et al. Citation2012; Aussant et al. Citation2018; Robertson et al. Citation2013). Unfortunately, these factors were not controlled in our study, as mushrooms were collected over several months of the mushroom season and pulled together into one collective sample within a plot. Consequently, some samples could contain more sporocarps collected during the part of the season with higher temperatures and longer light period (end of a summer), whereas the others contain more sporocarps collected during the season with lower temperatures and shorter light period (beginning of autumn). This topic needs further research, taking into account weather- and season-dependent variables. Despite the fact that our study plots differed mainly in tree species richness and composition, we did not detect significant effects of these factors on the FA composition. The very limited effects of tree species richness and proportion of deciduous trees were visible only in case of SFA content.
Most of the published studies on factors influencing the FA content of mushrooms are the results of experiments aimed at improving the efficiency of mushroom cultivation. Our study is the first, or one of the very few, field studies to investigate environmental factors affecting the fatty acid content of wild mushrooms. Our results suggest that the most nutritious sporocarps, with the highest fatty acid content, grow in forest patches with low understory cover. However, due to fact that the level of FA content is highly dependent on the mushroom species identity, which is a much more important factor than any environmental variable, mushroom consumers looking for the most nutritionally valuable food should pay more attention to the species than to the place of sporocarp collection. In our previous study (Stojek et al. Citation2022), mixed forests with the highest number of tree species were found to have the highest sporocarp production. The lack of effect of this factor on FA content suggests a trade-off between mushroom productivity and quality.
Supplementary Figures.docx
Download MS Word (246.4 KB)ACKNOWLEDGMENTS
We thank the technical staff of the Białowieża Geobotanical Station (University of Warsaw) and Department of Toxicology and Bromatology (Medical University of Warsaw), especially those involved in the field and laboratory work: Ł. Dawidziuk-Iwacik, I. Smerczyński, A. Wiktoruk, and E. Syta. Stand characteristics and increment of trees were measured by the team of O. Bouriaud from the Forestry Faculty of the University of Suceava (Romania) in the framework of FunDivEUROPE (http://project.fundiveurope.eu) and Soil4EUROPE (https://www.biodiversa.org/977) projects.
DISCLOSURE STATEMENT
No potential conflict of interest was reported by the author(s).
SUPPLEMENTARY MATERIAL
Supplemental data for this article can be accessed online at https://doi.org/10.1080/00275514.2024.2325045
Additional information
Funding
LITERATURE CITED
- Amini Khoeyi Z, Seyfabadi J, Ramezanpour Z. 2012. Effect of light intensity and photoperiod on biomass and fatty acid composition of the microalgae, Chlorella vulgaris. Aquacult Int. 20(1):41–49. doi:10.1007/s10499-011-9440-1.
- Aussant J, Guihéneuf F, Stengel DB. 2018. Impact of temperature on fatty acid composition and nutritional value in eight species of microalgae. Appl. Microbiol. Biotechnol 102(12):5279–5297. doi:10.1007/s00253-018-9001-x.
- Ayaz FA, Chuang LT, Torun H, Colak A, Sesli E, Presley J, Smith BR, Glew RH. 2011. Fatty acid and amino acid compositions of selected wild-edible mushrooms consumed in Turkey. Int J Food Sci Nutr. 62(4):328–335. doi:10.3109/09637486.2010.533160.
- Baeten L, Verheyen K, Wirth C, Bruelheide H, Bussotti F, Finér L, Jaroszewicz B, Selvi F, Valladares F, Allan E, et al. 2013. A novel comparative research platform designed to determine the functional significance of tree species diversity in European forests. Perspect Plant Ecol Evol Syst. 15(5):281–291. doi:10.1016/j.ppees.2013.07.002.
- Bano Z, Rajarathnam S. 1988. Pleurotus mushrooms. Part II. Chemical composition, nutritional value, post-harvest physiology, preservation, and role as human food. Crit Rev Food Sci Nutr. 27(2):87–158. doi:10.1080/10408398809527480.
- Barros L, Venturini BA, Baptista P, Estevinho LM, Ferreira ICFR. 2008. Chemical composition and biological properties of portuguese wild mushrooms: a comprehensive Study. J Agric Food Chem. 56(10):3856–3862. doi:10.1021/jf8003114.
- Bartoń K. 2020. MuMIn: multi-Model inference. R package version 1.43.17.
- Bernaś E, Słupski J, Gębczyński P. 2022. Edible mushrooms of the polish carpathians. In: Hernik J, Walczycka M, Sankowski E, Harris BJ, editors. Cultural heritage – possibilities for land-centered societal development. Environmental History. 13. Cham: Springer Nature Switzerland AG. p. 259–268. doi:10.1007/978-3-030-58092-6_16
- Binkley D, Giardina C. 1998. Why do tree species affect soils? The warp and woof of tree-soil interactions. Biogeochemistry. 42(1):89–106. doi:10.1023/A:1005948126251.
- Braun-Blanquet J. 1928. Die Pflanzengesellschaften und ihre Untersuchung, and Braun-Blanquet J, editor. Pflanƶensoƶiologie: grundƶüge der Vegetationskunde. Wien: Springer: p. 18–319. doi:10.1007/978-3-662-02056-2_3.
- Calder PC. 2015. Functional roles of fatty acids and their effects on human health. J Parenteral Enteral Nutr. 39(1S):18S–32S. doi:10.1177/0148607115595980.
- Dawud SM, Raulund-Rasmussen K, Domisch T, Fineer L, Jaroszewicz B, Vesterdal L. 2016. Is tree species diversity or species identity the more important driver of soil carbon stocks, C/N ratio, and PH? Ecosystems. 19(4):645–660. doi:10.1007/s10021-016-9958-1.
- Diamantopoulou P, Papanikolaou S, Aggelis G, Philippoussis A. 2016. Adaptation of Volvariella volvacea metabolism in high carbon to nitrogen ratio media. Food Chem. 196:272–280. doi:10.1016/j.foodchem.2015.09.027.
- Dimitrijevic MV, Mitic VD, Jovanovic OP, Jovanovic VPS, Nikolic JS, Petrovic GM, Stojanovic GS. 2018. Comparative study of fatty acids profile in eleven wild mushrooms of boletacea and russulaceae families. Chem. Biodivers 15(1):e1700434. doi:10.1002/cbdv.201700434.
- Doğan HH, Akbaş G. 2013. Biological activity and fatty acid composition of Caesar’s mushroom. Pharm Biol. 51(7):863–871. doi:10.3109/13880209.2013.768272.
- Dubey SK, Chaturvedi VK, Mishra D, Bajpeyee A, Tiwari A, Singh MP. 2019. Role of edible mushroom as a potent therapeutics for the diabetes and obesity. 3 Biotech. 9(12):450. doi:10.1007/s13205-019-1982-3.
- Fox J, Weisberg S. 2017. Package “Car.” companion to applied regression. http://cran.nexr.com/web/packages/car/car.pdf.
- Günç Ergönül P, Akata I, Kalyoncu F, Ergönül B. 2013. Fatty acid compositions of six wild edible mushroom species. Sci World J. doi:10.1155/2013/163964.
- Hothorn T, Bretz F, Westfall P. 2008. Package “Multcomp”: simultaneous inference in general parametric models. Biometrical J. 50(3):346–363. doi:10.1002/bimj.200810425.
- Isik H. 2020. Fatty acid contents of three wild edible mushroom species. Chem. Nat. Compd 1–3. doi:10.1007/s10600-020-03239-0.
- Jacob M, Weland N, Platner C, Schaefer M, Leuschner C, Thomas FM. 2009. Nutrient release from decomposing leaf litter of temperate deciduous forest trees along a gradient of increasing tree species diversity. Soil Biol Biochem. 41(10):2122. doi:10.1016/j.soilbio.2009.07.024.
- Jakobsen AN, Aasen IM, Josefsen KD. 2008. Strom AR accumulation of docosahexaenoic acid-rich lipid in thraustochytrid aurantiochytrium sp. strain T66: effects of N and P starvation and O2 limitation. Appl Microbiol Biotechnol. 80:297–306. doi:10.1007/s00253-008-1537-8.
- Jaroszewicz B, Cholewińska O, Gutowski JM, Samojlik T, Zimny M, Latałowa M. 2019. Białowieża forest—a relic of the high naturalness of European forests. Forests. 10(10):849. doi:10.3390/f10100849.
- Joly FX, Fromin N, Kiikkilä O, Hättenschwiler S. 2016. Diversity of leaf litter leachates from temperate forest trees and its consequences for soil microbial activity. Biogeochemistry. 129(3):373–388. doi:10.1007/s10533-016-0239-z.
- Kalač P. 2009. Chemical composition and nutritional value of European species of wild growing mushrooms: a review. Food Chem. 113(1):9–16. doi:10.1016/j.foodchem.2008.07.077.
- Kalač P. 2013. A review of chemical composition and nutritional value of wild-growing and cultivated mushrooms. J Sci Food Agric. 93(2):209–218. doi:10.1002/jsfa.5960.
- Kaşık G, Nurullahoğlu ZU, Öztürk C, Öztürk R, Eroğlu G, Aktaş S, Özcan MM. 2020. Oil content and fatty acid compositions of some edible macrofungi. J Agroaliment Processes Technol. 26(3):217–222.
- Kooch Y, Samadzadeh B, Hosseini SM. 2017. The effects of broad-leaved tree species on litter quality and soil properties in a plain forest stand. CATENA. 150:223–229. doi:10.1016/j.catena.2016.11.023.
- Kumar H, Bhardwaj K, Kuča K, Sharifi-Rad J, Verma R, Machado M, Kumar D, Cruz-Martins N. 2022. Edible mushrooms’ enrichment in food and feed: a mini review. Int. J. Food Sci. 57(3):1386–1398. doi:10.1111/ijfs.15546.
- Laessoe T, Petersen JH. 2019. Fungi of temperate Europe. Vol. 1, 2. Princeton: Princeton University Press. p. 1–1715.
- Lai J, Zhu W, Zhang S, Zhang X, Lingfeng M. 2022. Glmm.Hp: an R package for computing individual effect of predictors in generalized linear mixed models. J Plant Ecol. 15(6):1302–1307. doi:10.1093/jpe/rtac096.
- Lenth RV 2023. Emmeans: estimated marginal means, aka least-squares means. https://cran.r-project.org/web/packages/emmeans/index.html.
- Lüdecke D. 2018. ggeffects: tidy data frames of marginal effects from regression models. J Open Source Software. 3(26):772. doi:10.21105/joss.00772.
- Luginbuehl LH, Guillaume NM, Kurup S, Van Erp H, Radhakrishnan GR, Breakspear A, Oldroyd GED, Eastmond PJ. 2017. fatty acids in arbuscular mycorrhizal fungi are synthesized by the host plant. Science. 356(6343):1175–1178. doi:10.1126/science.aan0081.
- Mandeel QA, Al-Laith AA, Mohamed SA. 2005. Cultivation of oyster mushrooms (Pleurotus spp.) on various lignocellulosic wastes. World J Microbiol Biotechnol. 21(4):601–607. doi:10.1007/s11274-004-3494-4.
- Monarrez-Gonzalez JC, Gonzalez-Elizondo MS, Marquez-Linares MA, Gutierrez-Yurrita PJ, Perez-Verdin G. 2020. Effect of forest management on tree diversity in temperate ecosystem forests in northern Mexico. PLoS One. 15(5):e0233292. doi:10.1371/journal.pone.0233292.
- Nagy K, Tiuca ID. 2017. Importance of fatty acids in physiopathology of human body. In: Catala A, editor. In: Fatty Acids. IntechOpen: p. 2–21. doi:10.5772/67407.
- Nakagawa S, Cuthill IC. 2007. Effect size, confidence interval and statistical significance: a practical guide for biologists. Biol Rev. 82(4):591–605. doi:10.1111/j.1469-185X.2007.00027.x.
- Niazi AR, Ghafoor A. 2021. Different ways to exploit mushrooms: a review. All Life. 14(1):450–460. doi:10.1080/26895293.2021.1919570.
- Oksanen J, Blanchet FG, Friendly M, Kindt R, Legendre P, McGlinn D, Minchin P, O’Hara R, Simpson G, Solymos, et al. 2020. Vegan community ecology package version 2.5-7 November 2020.
- Patil S, Ahmed S, Telang S, Mmv B. 2010. The nutritional value of pleurotus ostreatus (JACQ.:FR.) Kumm cultivated on different lignocellulosic agrowastes. Innov Rom Food Biotechnol. 7:66–76.
- Pérez-Moreno J, Guerin-Laguette A, Rinaldi AC, Yu F, Verbeken A, Hernández-Santiago F, Martínez-Reyes M. 2021. Edible mycorrhizal fungi of the world: what is their role in forest sustainability, food security, biocultural conservation and climate change? Plants People Planet. 3:471–490. doi:10.1002/ppp3.10199.
- Pfeffer PE, DD D, Bécard G, Shachar-Hill Y. 1999. Carbon uptake and the metabolism and transport of lipids in an arbuscular mycorrhiza1. Plant Physiology. 120(2):587–598. doi:10.1104/pp.120.2.587.
- R Core Team. 2021. R: a language and environment for statistical computing. Vienna (Austria): R Foundation for Statistical Computing. https://www.R-project.org/.
- Ribeiro B, Guedes de Pinho P, Andrade PB, Baptista P, Valentão P. 2009. Fatty acid composition of wild edible mushrooms species: a comparative study. Microchem J. 93(1):29–35. doi:10.1016/j.microc.2009.04.005.
- Robertson R, Guihéneuf F, Schmid M, Stengel D, Fitzgerald G, Ross P, Stanton C. 2013. Algae-derived polyunsaturated fatty acids. In: Catala A, editor. Polyunsaturated Fatty Acids: Sources, Antioxidant Properties and Health Benefits. Hauppauge, USA: Nova Sciences Publishers, Inc. p. 1–54.
- Royse DJ, Baars J, Tan Q. 2017. Current overview of mushroom production in the world. In: Zied DC, Pardo-Gimenez A, editors. Edible and medicinal mushrooms. Blackwell Publishing, Wiley John & Sons Inc. p. 5–13. doi:10.1002/9781119149446.ch2.
- RStudioTeam. 2022. RStudio: integrated development environment for R. Boston (MA): RStudio, PBC. http://www.rstudio.com/
- Sande D, Pereira de Oliveira G, Fidelis E, Moura MA, de Almeida Martinsmartins B, Silva Lima MTN, Takahashi JA. 2019. Edible mushrooms as a ubiquitous source of essential fatty acids. Food Res Int. 125:108524. doi:10.1016/j.foodres.2019.108524.
- Scherer-Lorenzen M, Schulze ED, Don A, Schumacher J, Weller E. 2007. Exploring the functional significance of forest diversity: a new long-term experiment with temperate tree species (BIOTREE). Perspect Plant Ecol Evol Syst. 9(2):53–70. doi:10.1016/j.ppees.2007.08.002.
- Seyfried GS, Canham CD, Dalling JW, Yang WH. 2021. The effects of tree-mycorrhizal type on soil organic matter properties from neighborhood to watershed scales. Soil Biol Biochem. 161:108385. doi:10.1016/j.soilbio.2021.108385.
- Sokoła-Wysoczańska E, Wysoczański T, Wagner J, Czyż K, Bodkowski R, Lochyński S, Patkowska-Sokoła B. 2018. Polyunsaturated fatty acids and their potential therapeutic role in cardiovascular system disorders—a review. Nutrients. 10(10):1561. Article 10. doi:10.3390/nu10101561.
- Stojek K, Gillerot L, Jaroszewicz B. 2022. Predictors of mushroom production in the European temperate mixed deciduous forest. For Ecol Manage. 522:120451. doi:10.1016/j.foreco.2022.120451.
- Tajik S, Ayoubi S, Khajehali J, Shataee S. 2019. Effects of tree species composition on soil properties and invertebrates in a deciduous forest. Arabian J Geosci. 12(11):368. doi:10.1007/s12517-019-4532-8.
- Valverde ME, Hernández-Pérez T, Paredes-López O. 2015. Edible Mushrooms: improving human health and promoting quality life. Int J Microbiol. 2015:e376387. doi:10.1155/2015/376387.
- Veljović S, Krstić J. 2020. Elaborating on the potential for mushroom-based product market expansion: consumers’ attitudes and purchasing intentions. In: Singh J, Meshram V, Gupta M, editors. Bioactive natural products in drug discovery. Springer Nature Singapore. p. 643–663. doi: 10.1007/978-981-15-1394-7_23.
- Verheyen K, Ceunen K, Ampoorter E, Baeten L, Bosman B, Branquart E, Carnol M, De Wandeler H, Grégoire JC, Lhoir P, et al. 2013. Assessment of the functional role of tree diversity: the multi-site FORBIO experiment. Plant Ecol & Evol. 146(1):26–35. doi:10.5091/plecevo.2013.803.
- Vidhyalakshmi R, Priya DL, Sumithira P. 2017. Influence of substrate in the nutritive value of oyster mushroom. Indian J Appl Microbiollied. 20(1):47–54. doi:10.46798/ijam.2017.v20i01.006.
- Wasserstein RL, Lazar LA. 2016. The ASA statement on p-values: context, process, and purpose. Am Stat. 70(2):129–133. doi:10.1080/00031305.2016.1154108.
- Yu XJ, Huang CY, Chen JL, Wang ZP, Chen JL, Li HJ, Liu XY, Wang ZP, Sun J, Wang ZP. 2019. High-throughput biochemical fingerprinting of oleaginous aurantiochytrium sp. strains by fourier transform infrared spectroscopy (FT-IR) for lipid and carbohydrate productions. Molecules. 24(8):1593. doi:10.3390/molecules24081593.
- Zellweger F, De Frenne P, Lenoir J, Vangansbeke P, Verheyen K, Bernhardt-Römermann M, Baeten L, Hédl R, Berki I, Brunet J, et al. 2020. Forest microclimate dynamics drive plant responses to warming. Science. 368(6492):772–775. doi:10.1126/science.aba6880
- Zhang XY, Li B, Huang BC, Wang FB, Zhang YQ, Zhao SG, Li M, Wang HY, Yu XJ, Liu XY, et al. 2022. Production, biosynthesis, and commercial applications of fatty acids from oleaginous fungi. Frontiers in Nutrition. 9:873657. doi:10.3389/fnut.2022.873657.