Abstract
Lake Brunner, located on the west coast of the South Island of New Zealand, has been identified as threatened by phosphorus (P) inputs from neighbouring dairy farms. A two-year study was undertaken to determine if the application of reactive phosphate rock (RPR) with or without the addition of aluminium (Al) sulphate (alum) at 20 kg Al ha−1 could decrease P losses in surface runoff from dairy pastures compared to the same rate of P applied as superphosphate (30 kg P ha−1). The study was conducted on nine humped and hollowed plots (1100–3500 m2). Frequent and reliable rainfall year round (totalling 4000–5000 mm yr−1) translated into more than 50 surface runoff events per year and large losses of P from superphosphate-treated plots (flow-weighted mean filterable reactive P (FRP) = 0.072 mg l−1; mean load 8.2 kg P ha−1 yr−1). In contrast, the flow-weighted mean FRP concentration in the RPR-treated plots was significantly less (0.034 mg l−1; mean load 4.0 kg P ha−1 yr−1). No effect was found on other P forms. Alum did not decrease P losses, most likely due to Al being washed off in surface runoff before it could bind to the soil. Enhanced P losses from superphosphate-treated plots were attributed to the greater availability of P within 21 days of application (water solubility 80–95%) compared with RPR (≈1% water soluble), and the occurrence of surface runoff during this period (especially in year 1). The use of RPR is recommended as one management practice to decrease FRP inputs to Lake Brunner and may be useful in other regions where rainfall and soil pH allow.
Introduction
Lake Brunner (42 36.975S, 171 26.126E) is an iconic lake, 30 km inland from Greymouth on the West Coast of New Zealand's South Island. Local residents, tourists and government have expressed concerns over declining water quality in the lake (Horrox Citation2008). The decline in water quality has been due to increases in phytoplankton biomass associated with increasing phosphorus (P) concentrations (Horrox Citation2005). At the same time, concentrations of nitrogen (N) have stabilised. Consequently, the management of water quality in the lake has focused attention on mitigating P inputs. It is thought that a considerable proportion of P inputs to the lake are associated with losses from dairy farms that feed the Crooked River and Orangipuku Stream. This is due to several factors, but foremost is ample rainfall (4000–5000 mm yr−1) and the reshaping of paddocks with humps that lie about 1–2 m higher than hollows to promote drainage. The hollows are spaced about 10–20 m apart and drain into channels that ultimately connect with the lake. Humping and hollowing creates a very effective conduit for P from the dairy farms to travel into Lake Brunner with decreased opportunity for attenuation in stream beds or riparian areas. The quantity of P lost from dairy farms in the region is estimated to range from 2 to 9 kg ha−1 yr−1 (Rutherford et al. Citation2008; Wilcock et al. Citation2007). The five-year average load in Pigeon Creek, a tributary of the Orangipuku Stream, is estimated to be about 5 kg P ha−1 yr−1 (Wilcock Citation2006).
The P lost from dairy farms comes from a number of sources. McDowell (Citation2008a ,Citationb) determined that the proportion lost to waterways varied according to how soon runoff followed the application of fertiliser or a grazing event. Using the equations generated from this work, it was estimated that fertiliser and dung contributed about 10–40 and 30–50%, respectively, of total P lost from the paddock. The remaining P was thought to come from treading, via crushed and denuded pasture and displacement of soil particles, and dissolution from the soil. The ability of soils within the Lake Brunner region to retain P is poor due to a lack of aluminium (Al) and iron (Fe) oxides (McDowell Citation2008a). This means that for every unit increase in soil test P concentration (i.e. Olsen P in New Zealand (Olsen et al. Citation1954)), the potential for P loss is greater than for soils with greater P retention.
Of the factors that could be managed on dairy farm paddocks, decreasing the P lost from fertiliser and improving the P retention capacity of the soil are two that have been highlighted as simple and potentially cost-effective (McDowell Citation2008b). Methods to decrease P lost from fertilisers have focused on using fertilisers with little water-soluble P (e.g. Hart et al. Citation2004). This implies that low water-soluble P fertilisers are safe to apply in areas where runoff is frequent, but no data exist to confirm this within a grazed systems context. To improve soil P retention, some work has indicated that adding Al or Fe oxides or calcium (Ca) to soils may decrease P loss (McDowell & Houlbrooke Citation2009; Stout et al. Citation1999). However, no field work has been done in grazed pastures. An additional benefit may arise from surface-applying these materials instead of incorporating them into the soil (e.g. when reseeding), thereby acting to decrease P losses from freshly deposited dung.
Due to toxicity concerns about grazing pasture with alkaline Ca-containing compounds that alter rumen pH (Dewes et al. Citation1995), only materials containing Fe and Al would appear suitable. Of these, aluminium sulphate (alum) has been extensively evaluated as an additive for poultry and swine manure in the USA and was shown to decrease P loss from a grassland catchment with moderate annual rainfall (1500 mm) compared with a catchment where untreated manure was spread (Moore & Edwards Citation2005 ,Citation2007). However, alum has not been tested on grazed pastures or in high-rainfall environments where any benefit could be negated if alum is lost in surface runoff.
The primary objective of this research was to determine if the load of P lost in surface runoff from humped and hollowed pastures grazed by dairy cattle was less when fertiliser-P was applied as reactive phosphate rock (RPR; <1% water soluble) than with the same rate of P (30 kg ha−1) applied as superphosphate (≈80–90% water soluble). A secondary objective was to determine if spraying alum onto pastures could further decrease P losses further in a high-rainfall environment.
Materials and methods
Site
The site was located on a dairy farm (>20 years old) within the Inchbonnie catchment, which in turn drains into Lake Brunner on the West Coast of the South Island of New Zealand. The chosen paddock had humps that were 10 m apart, with hollows that fell to a maximum depth of 1 m below humps. The soil at the site, a Hari Hari silt loam (New Zealand classification, Mottled Fluvial Recent; USDA classification, Fluvent (Hewitt Citation1998)), supported a permanent ryegrass (Lolium perenne) and white clover (Trifolium repens) pasture that had been established eight years prior to use in this trial. It was grazed as part of the farm's rotational grazing regime every 20–60 days, depending on the time of year and pasture yield. The overall farm stocking rate was 2.3 cows ha−1. The paddock received RPR at a rate of 18 kg P ha−1 in October each year. Sufficient lime was applied every three to four years to keep soil pH between 5.6 and 5.8.
Installation, trial design and sample analyses
In April 2006, nine of the 13 hollows in the paddock were surveyed and contributing areas quantified (mean area, 1684 m2; range 952–3623 m2). The following month, a standard 22.86 cm high H-flume (Grant Citation1979) was installed at the outlet of each of the nine hollows, subsequently called plots. Three months later, the nine plots were assigned a number, randomised and one of three treatments applied each with three replicates. The three treatments were:
-
30 kg ha−1 of P broadcast as superphosphate
-
30 kg ha−1 of P broadcast as RPR
-
30 kg ha−1 of P broadcast as RPR, followed by 20 kg ha−1 of Al applied as a liquid by dissolving alum in 100 l of deionised water as per McDowell & Houlbrooke (Citation2009).
Soil samples were taken at a depth of 0–7.5 cm one month before and one and six months after treatments were imposed. The one- and six-month samplings were repeated in the second year of the trial. Soils were air-dried, crushed and passed through a 2 mm sieve before being sent to a commercial laboratory for analysis of:
-
Olsen P (Olsen et al. Citation1954)
-
pH (in water; 1:2.5 soil to water ratio (Hendershot et al. Citation1993))
-
water-extractable P (an estimate of P in surface runoff (McDowell & Condron Citation2004))
-
resin-extractable P (bicarbonate form—shown to better estimate plant available P in soils with RPR applied than Olsen P (Amer et al. Citation1955; Menon et al. Citation1989))
-
total P (Crosland et al. Citation1995)
-
exchangeable Al (0.01M CaCl2 method; (Bertsch & Bloom Citation1996)) ().
Table 1 Mean soil chemical concentrations (ASC = anion storage capacity) for soil samples taken before and one and six months after treatments. The least significant difference (LSD05) at the P <0.05 level is given. The asterisk indicates the LSD05 is significant, otherwise not significant.
Statistical analyses
For treatment comparisons, events were summed to generate an annual load (kg ha−1) for each year, beginning in June 2007. This load was divided by annual flow (l ha−1) volume to get annual flow-weighted concentrations (mg l−1). Loads could not be used for comparison among treatments due to the different plot sizes that would bias P loads towards larger plots due to the influence on surface runoff volumes. The concentrations were tested for normality and, if necessary, log10 transformed before an analysis of variance was used to indicate a significant difference (P<0.05) for different P fractions between treatments. Additional comparisons were made of the flow-weighted mean annual concentrations and flow-weighted concentrations during the seven days during and after grazing periods, or the 21 days following the application of fertiliser treatments.
Results and discussion
Soil chemical properties
No significant difference was noted between treatment means of most soil chemical measurements, but resin-extractable P and water-extractable P were both greater one and six months after treatments were imposed as compared with one month beforehand (). The enhanced responsiveness of resin-extractable P over Olsen P was probably a reflection of the inability of the Olsen test to account for P in minerals such as apatite found in RPR (Rajan et al. Citation1996; Saggar et al. Citation1990). Schindler et al. (Citation2009) have recently suggested that for predicting P loss in surface runoff, agronomic tests like Olsen P (Olsen et al. Citation1954) are as good as water-soluble P tests (McDowell & Condron Citation2004), which were designed specifically for the task. However, the Olsen P data in suggest that no difference in surface runoff P concentrations would exist between treatments. In contrast, water-soluble P concentrations indicated a significant difference between treatments one month after application ().
Compared with agronomic guidelines, pH appeared to be slightly above levels recommended for optimal production for most ryegrass–clover pastures (range 5.8–6.0 (Roberts & Morton Citation1999)), while Olsen P was within the range considered optimal for sedimentary soils (20–30 mg l−1 (Roberts & Morton Citation1999)). Past work has shown that for the same Olsen P concentration, the potential P concentration in surface runoff varies widely by soil type (McDowell & Condron Citation2004). While the soil in the Inchbonnie catchment is poor at retaining P (McDowell Citation2008a), agronomically there is little scope for cost-effectively decreasing Olsen P concentrations to reduce P loss from the soil. However, McDowell (Citation2008b) estimated that up to 40% of P losses from dairy-farmed soils in the catchment came from the application of superphosphate, which other studies (e.g. McDowell & Catto Citation2005; Nguyen et al. Citation1999) suggest could be decreased with the use of a low water-soluble P fertiliser like RPR.
Surface runoff data
Rainfall during years 1 and 2 was about 3500 mm and 3000 mm, respectively. A similar pattern was noted for surface runoff, which tended to be about one-third of rainfall (). Mean daily flows during the year varied from 0 mm to just below 180 mm (). The number of surface runoff events paralleled rainfall events in excess of 5 mm between May and October and 15 mm from November to April. During year one, 5 out of 11 grazing events coincided with surface runoff events, but decreased to 4 out of 10 in year 2 (). Similarly, in year 1, a much greater volume of surface runoff occurred within 21 days of treatments being applied (58 mm) than occurred in year 2 (5 mm; ).
Fig. 1 Example mean daily flow (flume 1, RPR treatment, 3623 m2) and grazing times (grey bars, average 290 cows ha−1) during the study. The arrows indicate when fertiliser treatments were imposed and the hatched bars indicate the 21-day period following fertiliser application.
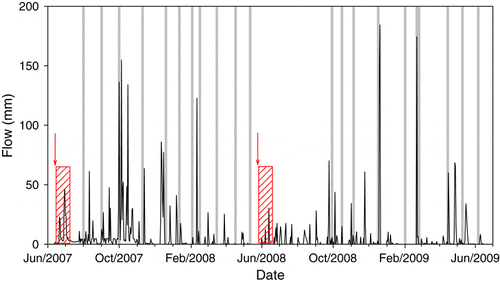
Table 2 Surface runoff and flow-weighted mean concentration of filterable reactive phosphorus (FRP), filterable unreactive P (FUP), particulate P (PP) and total P (TP) for each treatment in both years of the trial. The least significant difference (LSD05) at the P <0.05 level is given. An asterisk indicates the LSD05 is significant.
The mean loads of all three P fractions comprising TP (FRP, FUP and PP) were greatest in the superphosphate treatment, followed by the RPR and RPR + alum treatments (). McDowell & Wilcock (Citation2008) surveyed contaminant losses from different pastoral land uses. Losses of P from grazed dairy land ranged from 0.2 to 10.5 kg P ha−1 yr−1 with a mean of 1.9 kg P ha−1 yr−1, less than the lowest mean loss recorded in the present study (). Although least significant differences are given in between treatments as an indication of variation, due to the dependence of loads on volumes of surface runoff that vary greatly between the plots, treatment comparisons in the present study were made with flow-weighted mean concentrations. No significant differences were found among treatments for FUP and PP fractions. However, flow-weighted mean concentrations of FRP for data from both years combined were greater in the superphosphate treatment than either the RPR or RPR + alum treatments. This was largely due to the greater mean concentration of FRP lost in surface runoff in year 1 compared with year 2 (). The treatment differences for FRP parallel the relative water solubility of RPR (<1%) and superphosphate (85–95%), and support the hypothesis that P losses from fertilisers are associated with dissolution and loss in surface runoff of dissolved or filterable reactive P (Hart et al. Citation2004).
Fig. 2 Mean loads of filterable reactive phosphorus (FRP), filterable unreactive P (FUP) and particulate P (PP) for each treatment in year 1 and 2. The least significant difference at the P <0.05 level is given for the comparison of treatment differences for each P fraction within each year.
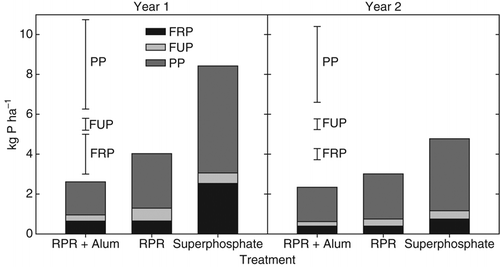
Some studies have indicated that the potential for fertiliser-P loss decreases exponentially with time since application (Nash et al. Citation2004). The rate of decrease and initial loss is dependent on the fertiliser's water solubility (McDowell & Catto Citation2005). For superphosphate, Nash et al. (Citation2004) defined a 21-day period after application as when the greatest potential for P loss from superphosphate occurs. In examining the 21-day period following the application of fertiliser treatments over both years, concentrations of all P fractions (and total P) were significantly greater in the superphosphate treatment than in the RPR and RPR + alum treatments (P <0.001). Furthermore, although concentrations of all fractions were less in year 2 than in year 1 (P <0.001), the flow-weighted mean concentration during the 21-day period after application was greater than the concentrations for the remainder of the year (compare and ). The greater concentrations in year 1 compared with year 2 can be explained by the tenfold greater volume of surface runoff within 21 days of application (, ). However, the enriched losses within 21 days of application for all treatments indicate that, while the risk for RPR is significantly less than for superphosphate (on average over both years 77% less for FRP), some P is still lost, and that there is also benefit in trying to apply RPR when surface runoff is unlikely (as happened in year 2).
Table 3 Flow-weighted mean concentration and percentage contributions (in parentheses) to annual surface runoff and load in each year for P fractions among the three fertiliser treatments for the 21-day period after fertiliser applied. The F-statistic represents the significant difference between annual and fertiliser-only flow-weighted concentrations for each P fraction over the two-year study period.
In contrast, no significant differences were found between flow-weighted mean concentrations for any fraction among the fertiliser treatments for the 7 days during and after grazing events for both years of the study. However, the greater frequency of grazing (compared with fertiliser applications) means that there was a greater likelihood of P from dung being lost in a surface runoff event (11 and 10 grazings in year 1 and 2, respectively). Although the availability of dung-P for loss in surface runoff declines exponentially with time since deposition (McDowell Citation2006; Smith et al. Citation2001), frequent grazing translated into a greater significant difference than that for fertiliser () in P concentrations between the 7-day periods following grazing and the rest of the year. Frequent grazing probably also caused the lack of any difference between year 1 and 2 concentrations during grazing periods.
Table 4 Flow-weighted mean concentration and percentage contributions (in parentheses) to annual surface runoff and load in each year for P fractions among the three fertiliser treatments for the seven-day periods after grazing (n=11 in year 1 and 10 in year 2). The F-statistic represents the significant difference between annual and grazing-only flow-weighted concentrations for each P fraction over the two-year study period.
Of those studies examining the loss of P associated with superphosphate applied to grazed pastures, the majority have measured large losses that, on a load basis, were similar to those measured here (for a summary see Hart et al. (Citation2004)). One example is the 4.36 kg P ha−1 (50% filterable) measured by Sharpley & Syers (Citation1979) in surface runoff from a grazed pasture plot with 50 kg P ha−1 applied. In a later study, Sharpley & Syers (Citation1983) measured P losses to be about 10% less in ungrazed pasture plots—a decrease commensurate with the percentage contributed by grazing in year 1 of this study (). The key similarity is that, although Sharpley & Syers’ work applied P in June whereas P was applied in April in this research, both were applied when surface runoff was likely. While data for small plot (0.1–0.5 m2) studies of ungrazed pastures tested with simulated rainfall (e.g. Nguyen et al. Citation1999 ,Citation2002) and the present study of grazed pasture field plots both show that application of RPR instead of superphosphate decreases P losses, if superphosphate is applied at a time of year when surface runoff is unlikely, P losses from fertiliser would probably comprise about 10% of paddock losses (Nash et al. Citation2004). Agronomically, RPR can produce similar pasture production as superphosphate, but requires rainfall of >850 mm a year, a soil pH <6.0 and up to four years of repeated applications to do so (Sinclair et al. Citation1990). Hence, the decision to use RPR should consider agronomic and environmental benefits, especially if climate or soil conditions are less than ideal or P losses from soils receiving superphosphate are likely to be low.
While the use of RPR compared with superphosphate can decrease flow-weighted mean FRP concentrations at this high-rainfall site, the application of alum with RPR to decrease P losses had no effect. Several reasons could explain this, but chief is the likelihood that the alum was washed away before binding to the soil. Similar to soil samples, no significant increase in Al concentration was noted in pasture samples taken one month after the application of alum in year 1 (means±standard deviations for total Al concentration in pasture shoots from RPR, RPR + alum and superphosphate treatments were 440±174, 726±214 and 738±234 mg kg−1 dry weight, respectively). Insufficient Al was also present in the soil one or six months after application to have changed exchangeable Al concentrations (). It is also possible that Al was present, but ineffective, in this grazed pasture soil. Although some work has shown that alum decreases P losses from grazed winter forage crops (McDowell & Houlbrooke Citation2009) or when incorporated with manure sprayed onto pastures (Moore & Edwards Citation2007), both of these studies concerned areas with annual rainfall (including irrigation) of about 1100 mm. Additional work is required to determine the right application method and the effectiveness of alum in decreasing P losses from grazed pastures in higher rainfall environments.
Conclusions
Due to high rainfall and soil contouring (with humps and hollows) to assist the removal of surplus water, surface runoff losses of P from dairy pastures in the Inchbonnie catchment can be large. Of the factors that can be easily controlled, losses arising from the addition of superphosphate appeared the simplest. The application of 30 kg P ha−1 RPR resulted in less FRP loss in surface runoff compared with the application of superphosphate. This decrease is most likely associated with the lower water-soluble P concentration of RPR, meaning that if a surface runoff event was to occur soon after application, less fertiliser-P would be solubilised and lost. Differences in FRP between treatments were dominated by the tenfold greater depth of surface runoff within 21 days of application in year 1 compared with year 2. Given there is little or no cost difference between the two fertilisers (per kilogramme of fertiliser-P applied), the use of RPR in this high-rainfall environment appears to make economic and environmental sense.
Acknowledgements
The assistance of Rosalie and Des Shaffrey in allowing access to the farm and in sample collection and Peter Carey in site maintenance is gratefully acknowledged. This trial was funded by the Ministry of Agriculture and Forestry's Sustainable Farming Fund with contributions from Dairy New Zealand, Westland Milk Products and the West Coast Regional Council.
References
- Amer , F , Bouldin , DR , Black , CA and Duke , FR . 1955 . Characterization of soil phosphorus by anion exchange resin adsorption and P32-equilibration . Plant and Soil , 6 : 391 – 408 .
- Bertsch , PM and Bloom , PR . 1996 . “ Aluminum ” . In Methods of Soil Analysis, Part 3: Chemical Methods , Edited by: Sparks , DL . 517 – 574 . Madison, WI : Soil Science Society of America .
- Crosland , AR , Zhao , FJ , McGrath , SP and Lane , PW . 1995 . Comparison of aqua regia digestion with sodium carbonate fusion for the determination of total phosphorus in soils by inductively coupled plasma atomic emission spectroscopy (ICP) . Communications in Soil Science and Plant Analysis , 26 : 1357 – 1368 .
- Dewes , HF , McLeay , LM and Harfoot , CG . 1995 . Fly ash, basic slag and Glenbrook slag toxicity in cattle . New Zealand Veterinary Journal , 43 : 104 – 109 .
- Eisenreich , SJ , Bannerman , RT and Armstrong , DE . 1975 . A simplified phosphorus analysis technique . Environmental Letters , 9 : 43 – 53 .
- Grant , DM . 1979 . ISCO Open Channel Flow Measurement Handbook , Lincoln, NE : Instrumentation Specialities Company .
- Hart , MR , Quin , BF and Nguyen , ML . 2004 . Phosphorus runoff from agricultural land and direct fertilizer effects: a review . Journal of Environment Quality , 33 : 1954 – 1972 .
- Hendershot , WH , Lalande , H and Duquette , M . 1993 . “ Soil reaction and exchangeable acidity ” . In Soil Sampling and Methods of Analysis , Edited by: Carter , MR . 141 – 146 . Boca Raton, FL : Lewis publishers .
- Hewitt , AE . 1998 . New Zealand soil classification . Landcare Research Science Series , 1 : 1 – 133 .
- Horrox , J . 2005 . State of the environment report: West Coast surface water quality , Greymouth, , New Zealand : West Coast Regional Council .
- Horrox , J . 2008 . State of the environment report: West Coast surface water quality , Greymouth, , New Zealand : West Coast Regional Council .
- McDowell , RW . 2006 . Contaminant losses in overland flow from cattle, deer and sheep dung. Water . Air and Soil Pollution , 174 : 211 – 222 .
- McDowell , RW . 2008a . Phosphorus in humped and hollowed soils of the Inchbonnie catchment, West Coast. II: Accounting for losses by different pathways . New Zealand Journal of Agricultural Research , 51 : 307 – 316 .
- McDowell , RW . 2008b . Phosphorus in humped and hollowed soils of the Inchbonnie catchment, West Coast. I: Variation with age and distribution . New Zealand Journal of Agricultural Research , 51 : 299 – 306 .
- McDowell , RW and Catto , W . 2005 . Alternative fertilizers to prevent incidental phosphorus loss . Environmental Chemistry Letters , 2 : 169 – 174 .
- McDowell , RW and Condron , LM . 2004 . Estimating phosphorus loss from New Zealand grassland soils . New Zealand Journal of Agricultural Research , 47 : 137 – 145 .
- McDowell , RW and Houlbrooke , DJ . 2009 . Management options to decrease phosphorus and sediment losses from irrigated cropland grazed by cattle and sheep . Soil Use and Management , 29 : 224 – 233 .
- McDowell , RW and Wilcock , RJ . 2008 . Water quality and the effects of different pastoral animals . New Zealand Journal of Veterinary Research , 50 : 289 – 296 .
- Menon , RG , Chien , SH and Hammond , LL . 1989 . Comparison of the Bray I and Pi tests for evaluating plant-available phosphorus from soils with different partially acidulated phosphate rocks . Plant and Soil , 114 : 211 – 216 .
- Moore , PA Jr and Edwards , DR . 2005 . Long-term effects of poultry litter, alum-treated litter, and ammonium nitrate on aluminium availability in soils . Journal of Environmental Quality , 34 : 2104 – 2111 .
- Moore , PA Jr and Edwards , DR . 2007 . Long-term effects of poultry litter, alum-treated litter, and ammonium nitrate on phosphorus availability in soils . Journal of Environmental Quality , 36 : 163 – 174 .
- Nash , D , Hannah , M , Clemow , L , Halliwell , D , Webb , B and Chapman , D . 2004 . A field study of phosphorus mobilisation from commercial fertiliser . Australian Journal of Soil Research , 42 : 313 – 320 .
- Nguyen ML , Cooper AB , Thorrold BS 1999 . Phosphorus losses in surface runoff from field plots receiving soluble and slow-release phosphatic fertilisers . National Institute of Water and Atmospheric Research , Hamilton , New Zealand NIWA Client Report SQF90201
- Nguyen ML , Quin BF , Sukias JPS 2002 . Potential losses of phosphorus and nitrogen in runoff and drainage from pastoral soils applied with superphosphate and reactive phosphate rock In Currie LD , Loganathan P Dairy Farm Soil Management. Occasional Report 15 . Fertilizer and Lime Research Centre, Massey University , Palmerston North, , New Zealand 137 153 .
- Olsen SR , Cole CV , Watanabe FS , Dean LA 1954 . Estimation of available phosphorus in soils by extraction with sodium bicarbonate . DC : United States Department of Agriculture Washington Circular No. 939
- Rajan , SSS , Watkinson , JH and Sinclair , AG . 1996 . Phosphate rock for direct application to soils . Advances in Agronomy , 57 : 78 – 159 .
- Roberts , AHC and Morton , JD . 1999 . Fertiliser use of New Zealand dairy farms , Auckland : New Zealand Fertiliser Manufacturers’ Association .
- Rutherford , AJ and Monaghan , RM . 2005 . Inchbonnie catchment farm survey. Report prepared for West Coast Regional Council , Mosgiel, , New Zealand : AgResearch .
- Rutherford K , Chagué-Goff C , McKerchar A 2008 . Nutrient load estimates for Lake Brunner . NIWA Client Report HAM2008-060, prepared for West Coast Regional Council . National Institute of Water and Atmospheric Research , Hamilton, , New Zealand .
- Saggar , S , Hedley , MJ and White , RE . 1990 . A simplified resin membrane technique for extracting phosphorus from soils . Fertilizer Research , 24 : 173 – 180 .
- Schindler , FV , Guidry , AR , German , DR , Gelderman , RH and Gerwing , JR . 2009 . Assessing extractable soil phosphorus methods in estimating phosphorus concentrations in surface runoff-off from Calcic Hapludolls . Soil Use and Management , 25 : 11 – 20 .
- Sharpley , AN and Syers , JK . 1979 . Effect of aerial topdressing with superphosphate on the loss of phosphate from a pasture catchment . New Zealand Journal of Agricultural Research , 22 : 273 – 277 .
- Sharpley , AN and Syers , JK . 1983 . Transport of phosphorus in surface runoff as influenced by liquid and solid fertilizer phosphate addition . Water, Air and Soil Pollution , 19 : 321 – 326 .
- Sinclair , A , Dyson , CB and Shannon , PW. 1990 . The long-term effectiveness of reactive phosphate rock as a phosphate fertiliser for New Zealand pastures . Proceedings of the New Zealand Grassland Association , 51 : 101 – 104 .
- Smith , KA , Jackson , DR and Withers , PJA . 2001 . Nutrient losses by surface run-off following the application of organic manures to arable land. 2. Phosphorus . Environmental Pollution , 112 : 53 – 60 .
- Stout , WL , Sharpley , AN , Gburek , WJ and Pionke , HB . 1999 . Reducing phosphorus export from croplands with FBC fly ash and FGD gypsum . Fuel , 78 : 175 – 178 .
- Wilcock , RJ . 2006 . “ Inchbonnie (Lake Brunner) catchment study water quality in Pigeon Creek ” . In Lake Brunner Project Update: December 2006 , Edited by: Washington , S , Derks , J and Cotton , S . 3 – 4 . Christchurch : NZ Landcare Trust .
- Wilcock , RJ , Monaghan , RM , Thorrold , BS , Meredith , AS , Betteridge , K and Duncan , MJ . 2007 . Land–water interactions in five contrasting dairying watershed: Issues and solutions . Land Use and Water Resources Research , 7 : 2.1 – 2.10 .