Abstract
Perennial-based pasture swards potentially offer land managers the capacity for recharge control in temperate cropping zone environments to satisfy the dual role of fostering increased agricultural productivity and reduced deep drainage. This study used a neutron moisture meter to monitor levels of stored soil water to 1.70 m under pastures sown to lucerne (Medicago sativa L.), phalaris (Phalaris aquatica L.), chicory (Cichorium intybus L.), perennial veldt grass (Ehrhata calcycina Sm.), grazing brome (Bromus stamineus E. Desv.), plantain (Plantago lanceolata L.), Rhodes grass (Chloris gayana Kunth), tall fescue (Festuca arundinacea syn. Lolium arundinaceum Schreb. syn. Schedonorus phoenix (Scop.) Holub.) and cocksfoot (Dactylis glomerata L.) in two contrasting environments in the cropping zone of southern New South Wales (NSW), Australia. Performance of two cultivars with contrasting levels of summer activity of each of the latter two species was also assessed. Differences in soil water between the various treatments were small but significant throughout the 5-year study due to successive below average rainfall years. However, the study demonstrated that lucerne remains the perennial pasture species with the greatest capacity to dry the soil profile for recharge control. Lucerne was the most persistent species under drought of all species evaluated, creating the largest total dry soil deficit at both sites (214 and 138 mm at Cootamundra and Wagga Wagga, respectively). There was a significant positive correlation (P<0.05) between persistence (measured as basal frequency from year 3 onwards) and percentage decrease in soil water content at both sites (R 2=0.63 and 0.68, respectively). Kasbah cocksfoot, Fraydo tall fescue, phalaris, chicory and perennial veldt grass all persisted moderately well and created dry soil buffers that were not significantly lower than lucerne at both sites (mean 200 and 133 mm, respectively). In contrast to previous findings, summer-active cultivars of cocksfoot and tall fescue did not show any advantage in drying soils in low-rainfall environments compared with more summer-dormant cultivars. The summer-dormant cultivars of each species were shown to be more persistent, enabling them to dry the soil profile incrementally over a longer period of time. This study has demonstrated the importance of increased plant persistence on reducing the water content of the soil profile in drought-prone environments.
Introduction
Deep drainage (i.e. the leaching of water and nutrients in the soil profile to sites below the plant root zone) is having serious agricultural and environmental consequences in southern Australia. It is widely credited as the major cause of soil salinisation (Dunin & Passioura Citation2006), and is a contributing factor to soil acidification (Scott et al. Citation2000; Ridley et al. Citation2001b) and eutrophication of waterways (Ridley et al. Citation2001b; Blaesing Citation2003; Connor Citation2004). Poor utilisation of seasonal rainfall also represents inefficiency in production. Australian agricultural production systems are located in environments that typically have low natural soil fertility and dry climates. Therefore the loss of water and nutrients due to deep drainage (Heng et al. Citation2001) has the potential to reduce agricultural yields and increase input costs. Incorporating perennial pastures into farming systems within the cropping zone has been suggested as an option for reducing soil degradation attributable to deep drainage (Cransberg & McFarlane Citation1994; Cocks Citation2001; Dear et al. Citation2003; Singh et al. Citation2003). Perennial-based pastures are seen as a feasible strategy to mitigate deep drainage through their ability to create a dry soil buffer over a large portion of the landscape (Black et al. Citation1981) with minimal disruption to existing production systems.
The superior ability of lucerne (Medicago sativa L.) to dry the soil profile in a range of environments is well documented (Christian Citation1977; Lolicato Citation2000; Latta et al. Citation2001; Ridley et al. Citation2001a; Brown et al. Citation2005; Bell et al. Citation2006; Fillery & Poulter Citation2006; Sandral et al. Citation2006; Ward Citation2006). Rarely has any pasture species been reported to exceed lucerne in its capacity to dry the soil profile, although occasionally an alternative species is reported to have achieved the same level of drying as lucerne. Despite the proven capacity of lucerne to dry the soil profile, Hayes et al. (Citation2010) argued for the need to identify viable alternative perennial pasture species in order to increase the sustainability of farming systems. Put simply, this argument is centred around: (i) the need for a variety of agronomic characteristics to satisfy various environmental and enterprise-dependent niches in agriculture; (ii) the need to reduce the vulnerability of existing farming systems to invasion from pests and diseases; (iii) the limited area over which one species, even one that is as broadly adapted and productive as lucerne, can feasibly be grown in a production enterprise.
Phalaris (Phalaris aquatica L.), cocksfoot (Dactylis glomerata L.), chicory (Cichorium intybus L.), perennial veldt grass (Ehrhata calcycina Sm.), grazing brome (Bromus stamineus E. Desv.), plantain (Plantago lanceolata L.) and Rhodes grass (Chloris gayana Kunth) are all commercially available, but not currently widely utilised in the cropping zones of south-eastern Australia (Dear et al. Citation2008). Nevertheless, their commercial availability is an attractive attribute as it provides an opportunity to inject alternative perennial pasture species into the farming system almost immediately without having to first undergo the expensive and time-consuming processes of plant breeding and selection. A reinvigorated research interest in alternative perennial pasture species (Dear & Ewing Citation2008) has highlighted some attractive attributes, such as different patterns of biomass production, increased herbage quality or adaptation to niche environments such as acid and waterlogged soils, which may be attractive to production agriculture (Dear et al. Citation2008; Li et al. Citation2008; Reed et al. Citation2008).
The soil drying capacity of a species is correlated with a range of plant factors including biomass production, seasonal growth pattern, persistence and rooting depth, and it is not necessarily true to assume that all perennial pasture species convey advantages in terms of soil drying. Cocksfoot, for example, is known to be little better than annual pasture species in creating a dry soil buffer (Ridley et al. Citation1997; Lolicato Citation2000; Sandral et al. Citation2006). However, there is a need to evaluate the ability of many of these species to reduce deep drainage through the creation of a large dry soil buffer because field testing, particularly of the less common species, has been limited. Thus, the first hypothesis tested in the current study was that chicory, grazing brome, perennial veldt grass, plantain and a subtropical grass (Rhodes grass) are comparable to lucerne in their ability to dry the soil profile in medium-rainfall cropping zone environments.
There is also a reinvigorated interest in cocksfoot and tall fescue due to the increased availability of Mediterranean cultivars, particularly of tall fescue. Mediterranean cultivars are known to persist better in summer-dry environments due to increased summer dormancy (Malinowski et al. Citation2005). However, previous studies have shown that, compared with more summer-active types, Mediterranean cultivars of cocksfoot (Lolicato Citation2000; Norton et al. Citation2006a) and tall fescue (Norton et al. Citation2006b) have a reduced capacity to dry the soil profile, implying that the adoption of Mediterranean cultivars across the cropping zone would not reduce deep drainage to the same extent as traditional cultivars. Therefore, the second hypothesis was to test whether summer-active cultivars of cocksfoot and tall fescue have superior soil drying capacities than summer-dormant types in lower rainfall environments.
Sites and treatments
Experiment 1
The site was located at Cootamundra (34°40′S, 147°50′E). The soil was a Yellow Dermosol (Isbell Citation1996) with a loam texture at the surface grading to a light clay below ∼1.2 m. The soil was highly acidic at the surface () with aluminium comprising 19% of the total exchangeable cation capacity at 0–0.10 m depth. The experiment was sown to monocultures of ten perennial pasture treatments arranged in a randomised complete block design with four replicates. Plot size was 6 m×4 m and pasture species and sowing rates included: lucerne cv. Aurora (6 kg/ha); chicory cv. Grasslands Puna (5 kg/ha); grazing brome cv. Gala (25 kg/ha); phalaris cv. Landmaster (4 kg/ha); cocksfoot cv. Currie (4 kg/ha); perennial veldt grass cv. Mission (8.5 kg/ha); and two tall fescue treatments cvv. Demeter and Fraydo (12.5 kg/ha each). Seed was sown into a cultivated seed bed using a cone seeder on 26 August 2004. Lucerne seed was inoculated with commercial rhizobia and lime pelleted prior to sowing.
Experiment 2
The site was located at the Wagga Wagga Agricultural Institute, NSW (35°05′S, 147°35′E). The soil was a Red Kandosol (Isbell Citation1996) with a clay-loam texture in the surface grading to a medium-heavy clay at ∼1.5 m. The soil surface was also acidic () but aluminium only comprised 6% of effective cation exchange capacity at 0–0.10 m. Treatments sown at experiment 2 included: lucerne cv. Aurora (6 kg/ha); chicory cv. Grasslands Puna (4 kg/ha); phalaris cv. Landmaster (4 kg/ha); cocksfoot cv. Currie (4 kg/ha); and the two tall fescue treatments, cvv. Demeter and Fraydo (12.5 kg/ha each). One additional treatment was included in the second experiment, cocksfoot cv. Kasbah (4 kg/ha), to provide an additional contrast in seasonal growth patterns of cocksfoot similar to the contrast in the tall fescue cultivars. This experiment was also a randomised complete block design with four replicates and plot size was again 6 m×4 m. Seed was sown into a cultivated seedbed using a cone seeder on 21 May 2004. Each treatment was sown as a binary mixture with subterranean clover cv. Seaton Park LF (4 kg/ha). All subterranean clover and lucerne seed was inoculated with the commercial rhizobia and lime pelleted prior to sowing.
Rainfall and irrigation
Monthly rainfall data and long-term annual average rainfall (LAR) for experiment 1 were obtained from the Cootamundra township (BOM Citation2009), approximately 10 km north of the experimental site, and were considered accurate enough to represent the rainfall received at the experimental site. Rainfall data for experiment 2 were measured at the Wagga Wagga Agricultural Institute.
In 2006, experiment 2 was irrigated four times during March, April and September in an attempt to maximise the chance of deep drainage occurring. The irrigation system comprised a series of dripper lines laid on the soil surface approximately 0.3 m apart with 2 l/hr pressure compensating nozzles spaced at 0.3 m. They were connected in a sealed system that covered one replicate completely and were connected to the town water supply. Irrigation was applied to one replicate at a time, with all replicates being irrigated in the same week. A flow meter was installed on the supply line to measure the quantity of water being delivered to each replicate. It was assumed that each plot within each replicate received an equal quantity of water. All plots received 168 mm of irrigation water in March, 83 mm in April and 99 mm in September 2006 (). The total rainfall plus irrigation water was 182 mm in March, 90 mm in April and 120 mm in September, which was still 17, 52 and 13% below the maximum rainfall ever recorded for those months, respectively.
Table 1 Monthly and annual rainfall (mm) received at experiments 1 (Cootamundra) and 2 (Wagga Wagga) during the experimental period and the long-term average rainfall (LAR) at each site.
Soil water measurement
An aluminium access tube (50 mm in diameter) was inserted in the centre of each plot in both experiments in November 2004 to a depth of 1.70 m at experiment 1 and 1.40 m at experiment 2. Soil water was measured using a neutron moisture meter (NMM; CPN 503 DR Hydroprobe, CPN International, Concord, California, USA) at 0.15, 0.30, 0.45, 0.60, 0.75, 0.90, 1.15, 1.40 and 1.70 m approximately every 6–8 weeks from 2004 to 2009. The NMM was calibrated in November 2004 when the aluminium access tube was originally inserted and re-calibrated in May 2009. There was good agreement between the two separate calibrations at both experiments of the NMM and there was little difference in the calibrations at the various depths. Therefore, a calibration equation was used, which encompasses all depths of both wet and dry soil, to determine volumetric water content for experiment 1 (Y 1) and experiment 2 (Y 2):
The drained upper limit (DUL) was determined at a 4 m×4 m area of soil adjacent to each experiment; these areas were covered with clear plastic film and irrigated over a period of about 6 weeks to ensure soil moisture reached above field capacity. At the end of the 6 weeks of irrigation, three cores were extracted from around the access tube using a purpose-built manual sampling kit. Soil bulk density, gravimetric water content and DUL were estimated comprising the mean of three cores at seven depths at each site. Volumetric soil water content was determined by correlating the gravimetric moisture content and bulk density of the sampled cores to the respective readings on the NMM. Crop lower limit (CLL) was deemed to be the water content of the profile at the time the plants exhibited symptoms of moisture stress. This was determined at a separate 4 m×4 m area containing established lucerne plants adjacent to each experiment which was irrigated in the previous autumn to DUL and sampled in late spring to determine the volume of water available to lucerne. Clear plastic tents were erected over the areas to ensure late season rainfall did not confound results. Sampling occurred as moisture stress was beginning to become evident in lucerne plants in November by taking three 20 mm soil cores to 1.70 m and assessing gravimetric water content of the soil at seven depths.
In order to compare the relative effectiveness of the treatments at drying the soil profile, the soil water deficit was calculated as being DUL minus the volumetric water content of the soil (mm). Soil water deficit is presented for the initial sampling at the end of year 1, and for autumn in each subsequent year in order to determine the maximum soil water deficit under each treatment. The exception to this is February 2006 at the experiment 2 site, which was the last observation of soil water prior to irrigation being applied.
Plant persistence
The term ‘persistence’ used throughout this study refers to the capacity of individual perennial species to maintain density, mainly through plant longevity. From year 3 onwards, basal frequency was measured to estimate the relative plant density of the perennial species as many established plants were too large to reliably distinguish individuals. This was done by placing a 1 m2 quadrat (divided into squares of 0.1 m×0.1 m) over the sampling area and counting the percentage of squares occupied or partially occupied by the base of a sown perennial plant. Further details on basal frequency of the treatments are given by Hayes et al. (Citation2010).
Agronomic management
Both experiments received an application of 180 kg/ha starter fertiliser (14.9% N, 13% P, 10.5% S) at sowing. In subsequent years, both experiments were annually top-dressed with an application of 160 kg/ha of superphosphate (8.8%P, 11%S) in autumn. Nitrogen fertiliser (granulated urea, 46% N) was applied at 100 kg/ha annually at experiment 1 only.
Trifluralin (480 g/l a.i.) was applied at 2 l/ha and incorporated to the chicory and lucerne plots only in experiment 2 two weeks prior to sowing, as a pre-emergent grass weed control. Insecticide was applied one week after sowing at experiment 1 (125 ml/ha Methidathion; 400 g/l a.i.) and experiment 2 (100 ml/ha Bifenthrin; 250 g/l a.i.). No other pesticides were applied to experiment 2. Flumetsulam (800 g/kg a.i.) was applied four weeks post-sowing at 25 g/ha to control emerging wireweed (Polygonum aviculare L.) in all treatments at experiment 1 except chicory, lucerne and plantain. A second application of Flumetsulam (25 g/ha) occurred in August year 2 at experiment 1, with herbicide applied to all treatments on this occasion.
Neither experiment was grazed in the establishment year. In subsequent years, sites were grazed hard by sheep over a short time frame immediately following an assessment of herbage yield. The experiments would then be mowed to ensure herbage in all treatments was defoliated to a uniform height if required. A residual of 500–800 kg/ha of above-ground biomass remained on the plots following defoliation. Further details on herbage yields of various treatments are described by Hayes et al. (Citation2010).
Statistical analysis
A linear mixed model was used to analyse changes in stored soil water over time to 1.70 m at experiment 1 (Cootamundra) and to 1.40 m at experiment 2 (Wagga Wagga), and soil volumetric water content over time at 0.15, 0.30, 0.45, 0.60, 0.75, 0.90, 1.15, 1.40 and 1.70 m. This mixed model included treatments (species), linear components of time trend and their interactions as fixed effects, and plot, block, the spline component of the time trend and associated interactions as random effects. All terms were included in the model initially, but terms that failed to achieve statistical significance (P<0.05) were excluded from the final model. The fixed effects were tested using an approximate F-test and the random effects were tested using a chi-squared test based on the residual maximum likelihood (REML) ratio test. All data were analysed using ASReml (Gilmour et al. Citation2006).
Results
Seasonal conditions
The experimental period coincided with an extended period of drought widespread across much of south-eastern Australia. Rainfall received at Cootamundra, approximately 10 km north of experiment 1, was below average each year during the experimental period, except in 2005 when annual rainfall was slightly above the long-term average (). However, rainfall in the autumn (March–May) in 2005 was still drier than in any other year and well below the long-term average for those months. 2006 was the second driest year on record for that location, with no rainfall at all recorded during October.
At the experiment 2 site, rainfall received was also consistently below average during the experimental period (). 2006 was the fourth driest year in 108 years of records at the Wagga Wagga Agricultural Institute. No deep drainage occurred (soil moisture did not reach DUL in any month) due to the unusually dry seasonal conditions experienced despite significant amounts of water being delivered through drip irrigation in March, April and September in 2006. The irrigation in this instance served to reduce the severity of the drought on this experiment rather than to increase deep drainage. The sum of rainfall and irrigation gave an annual total of 622 mm of water received in 2006, which was 18.7% higher than the LAR.
Total soil water content
Experiment 1
Soil in the surface 0.15–0.60 m under lucerne, chicory and plantain was significantly drier (P<0.05) at the initial sampling, taken three months after sowing, compared with the perennial grass treatments that received a selective broadleaf herbicide in year 1 ((b)). These differences diminished in spring 2005 during the wettest period of the experimental period (). From March 2007, soil in the surface 0.6 m was driest under lucerne. Changes in soil water at 0.75–1.70 m due to rainfall were more subtle than observed in the surface 0.60 m ((a)).
Experiment 2
There were small but significant (P<0.05) differences in soil water content at 0.15–0.60 m throughout the experimental period. In contrast to experiment 1, soil at this depth was wetter under lucerne ((b)). There was no significant difference in soil water at 0.75–1.40 m until February 2006, approximately 22 months after sowing ((a)). From then on, there was a divergence in the soil water values with lucerne and Kasbah cocksfoot treatments becoming incrementally drier, relative to Demeter tall fescue in particular ().
Vertical soil water patterns
Experiment 1
Lucerne and chicory displayed a similar and consistent pattern of drying the soil profile to 1.70 m. In both cases, soil above 0.75 m was driest in April 2005, 8 months post-sowing. Below this depth, soil under both treatments became incrementally drier over time ((a) and (b)). Differences in soil water content over time in other treatments were less consistent, with soil generally being wettest at most depths at the initial sampling time. In the Currie cocksfoot and Rhodes grass treatments, differences in volumetric water content below 1.15 m throughout the entire experimental period were very small ((g) and (j), respectively).
Fig. 4 Changes in volumetric water content (%) through time in the surface 1.70 m under (a) lucerne, (b) chicory, (c) phalaris, (d) perennial veldt grass, (e) Demeter tall fescue, (f) Fraydo tall fescue, (g) Currie cocksfoot, (h) grazing brome, (i) plantain and (j) Rhodes grass treatments at experiment 1 (Cootamundra) (P<0.05). Horizontal bars represent LSD at P = 0.05. No significant difference was detected at any depth if no horizontal bar is shown.
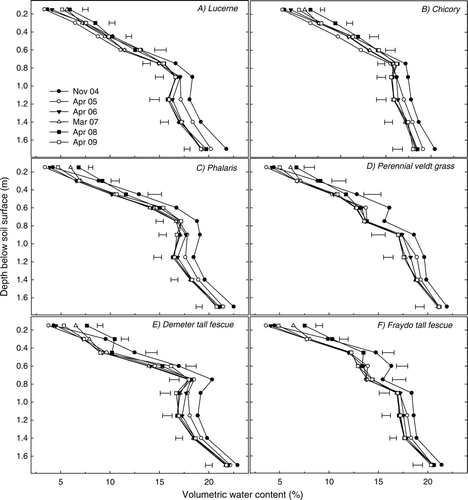
Experiment 2
Similarly to experiment 1, soil above 0.75 m under lucerne was at its driest in April 2005, approximately 11 months post-sowing. Below this depth, soil tended to become incrementally drier with time ((a)). In all other treatments, with the possible exception of Fraydo tall fescue ((d)), soil at most depths was no drier during the experimental period than in April 2005. The notable feature of the experiment 2 data was the very small change in volumetric water content below 0.9 m under most treatments.
Fig. 5 Changes in volumetric water content (%) through time in the surface 1.40 m under (a) lucerne, (b) chicory, (c) Demeter tall fescue, (d) Fraydo tall fescue, (e) Currie cocksfoot, (f) Kasbah cocksfoot and (g) phalaris at experiment 2 (Wagga Wagga). Horizontal bars represent LSD at P = 0.05. No significant difference was detected at any depth if no horizontal bar is shown.
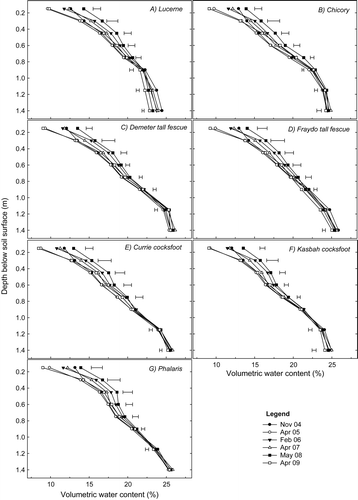
Plant persistence and soil drying
Soil under the more persistent species became incrementally drier than under the least persistent species at the maximum depth of monitoring at both locations. There was no significant difference between treatments (P<0.05) in volumetric water content at 1.70 m in experiment 1 in November 2004, but the soil profile was significantly drier under the chicory and lucerne treatments compared with the grazing brome, Rhodes grass, Demeter tall fescue and Currie cocksfoot treatments at the last date of measurement in 2008 ((a)). Similarly, in experiment 2, the soil at 1.40 m under lucerne at the end of the experiment was significantly drier (P<0.05) than all treatments except for Kasbah cocksfoot ((b)).
Fig. 6 Changes in volumetric soil water content (%) at 1.70 m and 1.40 m in experiments 1 and 2, respectively, under various perennial pasture species between November 2004 and April 2009. Error bars indicate significant differences at P = 0.05.
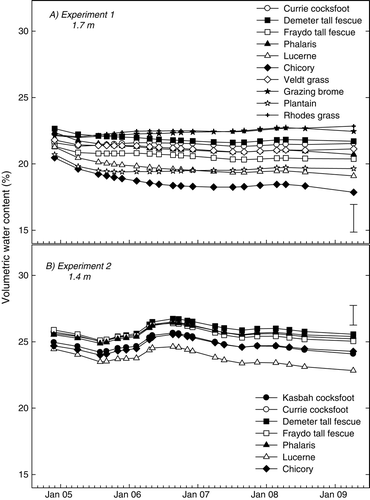
There was a significant positive correlation (P>0.05) between cumulative perennial basal frequency and decrease in soil available water in the profile between the first (November 2004) and final (April 2009) measurements at both experimental locations (). When Currie cocksfoot was omitted from the correlation at experiment 1, the coefficient of determination (R 2) increased from 0.64 to 0.86. Persistence of the various species at both locations is reported in more detail in Hayes et al. (Citation2010).
Fig. 7 Correlation between cumulative basal frequency over years 3, 4 and 5 of the pasture phase (%) and percentage decrease (%) in volumetric water content in the surface 1.70 m from November 2004 to April 2009 under various perennial pasture swards at (a) experiment 1 (Cootamundra) and (b) experiment 2 (Wagga Wagga).
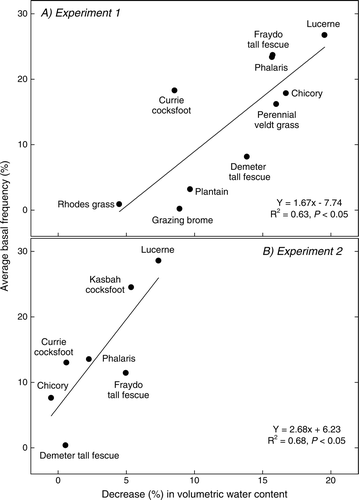
Dry soil deficit
Lucerne created a slightly larger, but significant, soil deficit than Demeter tall fescue at both locations, and Rhodes grass, grazing brome and Currie cocksfoot at experiment 1 throughout the 5-year experimental period (). There was also an apparent difference in the pattern of soil drying at the two different sites. At experiment 1, soil under lucerne was drier than many other treatments, often including cocksfoot (), in the entire 0.15–1.70 m profile, especially in the 0.15–0.60 m zone (). In contrast, at experiment 2, the soil under cocksfoot was consistently drier than other treatments at 0.15–0.60 m, and soil under lucerne at this depth was often the wettest; that is, lucerne had the lowest dry soil deficit in the surface 0.60 m at experiment 2.
Table 2 Dry soil deficit (mm) calculated for each perennial pasture treatment at 0.15–1.70 m at experiment 1 and 0.15–1.40 m at experiment 2 for six dates during the experimental period (values in parentheses represent the changes in dry soil deficit between 2004 and 2009).
Table 3 Dry soil deficit (mm) calculated for each perennial pasture treatment at 0.15–0.60 m at experiments 1 and 2 for six dates during the experimental period.
Discussion
Changes in soil water through time
A key finding of this study is the importance of increased perennial plant persistence on maximising soil water extraction in drought-prone environments. For much of the experimental period there was very little change in soil available water, particularly below 0.75 m, due to the very dry seasonal conditions experienced. Differences in the level of soil drying achieved were therefore largely attributed to incremental extraction of soil water at depth. This is illustrated clearly in where there is a gradual splaying of deep soil water data at both experiments, with volumetric water content becoming less at depth under the more persistent perennial species. There have been many studies in the past decade examining soil water under crops and pastures, particularly in Australia, due to an emphasis on research related to managing dryland salinity (Lolicato Citation2000; Angus et al. Citation2001; Heng et al. Citation2001; Latta et al. Citation2001; Ridley et al. Citation2001a; Brown et al. Citation2005; Bell et al. Citation2006; Sandral et al. Citation2006; Ward et al. Citation2006). Implicit in the findings of previous studies is the need for perennial plant persistence because, in the absence of plant survival, none of the other agronomic characteristics important in maximising water extraction such as rooting depth, ground cover, length of growing season and herbage yield can be expressed. Indeed, Christian (Citation1977) argued that the extent of soil drying depends less on the individual species and more on these agronomic traits listed. However, Christian (1977) did not mention plant persistence as being a key trait for increased soil drying, which the current study found to be a critical factor in the drought-prone environments of south-eastern Australia.
The importance of plant persistence on drying the soil profile was also demonstrated by comparing soil water under different cultivars with contrasting seasonal activities. This study showed that the summer-dormant cultivar of cocksfoot, Kasbah, was more persistent and created a dry soil deficit that was 12%, or 15 mm, greater than the less persistent cultivar Currie. The same could perhaps also be true for two of the contrasting tall fescue cultivars, although differences between cultivars of this species were not significant at (P > 0.05). The relatively low biomass production of Fraydo tall fescue may explain why differences in soil water under the two tall fescue cultivars were not significant despite significant differences in the persistence of these species (Hayes et al. Citation2010). Previous research has shown the summer-active cultivars of cocksfoot (Lolicato Citation2000; Norton et al. Citation2006a) and tall fescue (Norton et al. Citation2006b) to have superior soil drying capacities than the more summer-dormant cultivars in higher rainfall environments. The contrasting results of the present study provide confidence that the incorporation of summer-dormant grasses into lower rainfall regions will not lead to an increase in soil water recharge relative to more summer-active cultivars.
The other important aspect to consider is that increased plant persistence increases the capacity to opportunistically utilise episodic rainfall events simply by virtue of the perennial plant being present when the rainfall event occurs. Much of the environmentally degrading deep drainage that occurs is attributable to episodic rainfall events occurring outside the normal growing season (Black et al. Citation1981; Asseng et al. Citation2001; Ward Citation2006). However, the experimental sites in this research received few such episodic rainfall events. Nevertheless, had seasonal conditions been different, few perennial species other than lucerne persisted in adequate densities to utilise any rainfall that may have occurred.
Changes in soil water with depth
This research suggests that the pattern of soil drying between chicory and lucerne was similar. The dry soil deficit created under chicory and lucerne throughout the experimental period was almost identical at experiment 1 () and remained so until early 2006 at experiment 2 when chicory density declined sharply. The similarity in the pattern of soil drying between the two species is also represented graphically in (a) and (b). Visually, it appears that both chicory and lucerne are highly reliant on water from the surface 0.75 m in the first 10–12 months of growth, but thereafter appear to become more reliant upon water from deeper in the profile (below 0.75 m), which in both cases becomes incrementally drier with time. It would be useful to confirm this finding by growing the species in monocultures to avoid the confounding effects of volunteer weeds. However, given the tap root structure of both species and the similarity in seasonal growth patterns, it is reasonable to assert that the soil drying pattern of chicory is more similar to lucerne than any other species tested. This finding has some important implications in view of the fact that, previously, lucerne was considered unrivalled in its soil drying capabilities.
Much is already known about the root development of lucerne. Early lucerne root growth is largely confined to the tap root (Christian Citation1977), which can penetrate to 6 m over a number of successive years (Black et al. Citation1981; Ridley et al. Citation2001a; Fillery & Poulter Citation2006), perhaps even deeper. Its use of deep soil water for herbage production is sparing (Hoffmann et al. Citation2003), no doubt a function of its adaptation to semi-arid environments and the large plant water potential required in extracting water from depth relative to extracting freely available surface water (Bennett & Doss Citation1963). Sandral et al. (Citation2006) showed that lucerne was much quicker at drying the surface 1.70 m than perennial grasses, thus making it a much more effective species at de-watering the soil profile in shorter phased cropping rotations. Experiment 1 in the current study demonstrated that chicory also dried the soil profile very quickly, suggesting that it too would be a suitable species for this purpose. However, chicory was less persistent than lucerne, indicating that its drying influence on the soil profile is likely to be less enduring than that of lucerne. The mature chicory stand with lower plant populations would be less likely to maintain the dry soil deficit created in the first 2 years after establishment as long as lucerne in this environment, although this would be highly dependent upon the seasonal conditions experienced. Poorer plant persistence could perhaps explain why the water extraction capacity of chicory was inferior to that of lucerne, as reported by Brown et al. (Citation2005). However, reduced persistence may not necessarily be a disadvantage in short-phased farming systems. Unlike lucerne, which can reduce subsequent crop yields due to large water deficits associated with its superior soil drying capacity (Hirth et al. Citation2001), the reduced persistence of chicory potentially offers a perennial pasture alternative that provides a balance between reducing deep drainage in the short term and maximising crop yields. More work is required to investigate the practicality of using chicory in phased rotation with crops.
Evidence of initial rooting depth and pattern of soil drying of perennial species is less clear from experiment 2 for two reasons. Firstly, the earlier sowing time at this site allowed for greater plant development prior to the first observation of soil water content. Secondly, there appeared to be a substantial subsoil constraint at around 1 m below the soil surface, which limited plant access to soil water. This might be due to a physical constraint given the absence of any obvious chemical abnormality in the soil and the fact that the hydraulic soil corer used was unable to penetrate to 1.70 m in 16 of the 36 plots when installing access tubes at this site. The constraint becomes apparent by examining the bulk density of the initial site characterisation cores () as well as the soil water extraction of individual species throughout the experimental period (). Although the depth varies from species to species, in all cases volumetric water content converges in a band somewhere between 0.75 and 1.40 m, indicating an inability of plant roots to access additional water.
An interesting observation of experiment 2 was that plant growth was inhibited at this site compared with experiment 1 (Hayes et al. Citation2010) even though both locations suffered substantial droughts. In fact, experiment 2 was the site that received irrigation to alleviate moisture stress in the most severe drought year, yet cumulative yields of all perennial species were roughly half those measured at experiment 1. Plant persistence of all species other than lucerne was inferior to that observed at experiment 1. The moisture stress, most likely exacerbated by the physical constraint at experiment 2, was a major limiting factor to plant performance and plants were thus less able to survive at experiment 2 due to the reduced capacity to access deep soil water. Yet, lucerne performed significantly better than all other species at that site, most likely due to its superior capacity to penetrate this layer and access deep soil water. This could explain why the dry soil deficit in the surface 0.60 m at experiment 2 was 8–13% (or 6–9 mm) greater under Currie cocksfoot compared with lucerne (); this is in contrast to experiment 1 where soil under lucerne at 0.15–0.60 m tended to be drier.
Cocksfoot is known to be a shallower rooted perennial species (Ridley et al. Citation1997; Lolicato Citation2000; Sandral et al. Citation2006) and in the current study root penetration would have been further constrained by the subsoil in experiment 2. The entire water requirement of cocksfoot would therefore have been confined to the surface depths, in contrast to lucerne which was able to access deeper soil water ((a)). When considering the scientific literature on the root morphology and development of lucerne, Christian (Citation1977) found evidence affirming that lucerne ‘is not necessarily or genetically a deep-rooting plant, but that its roots will grow in any plane where water is available’. Based on our observations of the superior water extraction of lucerne despite a substantial physical subsoil constraint in experiment 2, it can be concluded that lucerne appears to have a more robust root system than chicory, phalaris, tall fescue or cocksfoot, which allows it to better penetrate its roots through dense subsoil.
An additional observation of the lucerne swards in this study was the more rapid decline in lucerne basal frequency between years 4 and 5 at experiment 1 compared with experiment 2. Conditions for plant growth in experiment 2 were generally less favourable than in experiment 1, which most likely explains why production of most species at experiment 2 was only half that observed in experiment 1. It was therefore unexpected that the decline in lucerne basal frequency towards the end of the experimental period was observed to be more rapid at experiment 1. The most logical explanation for this is that, although both sites had similar levels of soil pH, the exchangeable aluminium levels of the surface soil were much higher at experiment 1 than at experiment 2. Aluminium toxicity is known to inhibit root growth in sensitive species such as lucerne (Scott et al. Citation2008) and, when subjected to additional stress such as moisture deficit, it is plausible that high exchangeable aluminium may cause increased levels of mortality in ageing lucerne stands as was observed in this study. This adds support for the requirement of increased levels of aluminium tolerance in lucerne to maximise its persistence in these environments.
Conclusion
No species in the current study was consistently shown to dry the soil profile to the same extent as lucerne. However, chicory showed some similarities to lucerne in terms of the pattern of soil drying at the experiment 1 site where chicory persistence was greater.
Summer-active cultivars of cocksfoot and tall fescue were found not to have superior soil drying capacities compared with the summer-dormant types at the two lower rainfall sites. This study has highlighted the importance of perennial plant persistence in creating a dry soil buffer, particularly in low-rainfall environments.
Acknowledgements
Part of this study was used by RC Hayes in a Masters thesis submitted to Charles Sturt University. Financial support for the postgraduate study was provided by Australian Wool Innovation and NSW Department of Primary Industries. This study was run in conjunction with a national project that was supported in NSW by NSW Department of Primary Industries, the Grains Research and Development Corporation and the CRC for Plant-based Management of Dryland Salinity. Additional financial support was gratefully received from the Upper Lachlan Catchment Management Authority for activities conducted at the Cootamundra site. The authors gratefully acknowledge the contributions of Eric McKenzie and family of ‘Grasmere’ Cootamundra, for providing the land on which experiment 1 was conducted and of Mr Craig Lihou who provided much technical assistance for this research.
References
- Angus , JF , Gault , RR , Peoples , MB , Stapper , M and van Herwaarden , AF . 2001 . Soil water extraction by dryland crops, annual pastures, and lucerne in south-eastern Australia . Australian Journal of Agricultural Research , 52 ( 2 ) : 183 – 192 .
- Asseng , S , Fillery , IRP , Dunin , FX , Keating , BA and Meinke , H . 2001 . Potential deep drainage under wheat crops in a Mediterranean climate. I. Temporal and spatial variability . Australian Journal of Agricultural Research , 52 ( 1 ) : 45 – 56 .
- Bell , LW , Ryan , MH , Moore , GA and Ewing , MA . 2006 . Comparative water use by Dorycnium hirsutum-, lucerne-, and annual-based pastures in the Western Australian wheatbelt . Australian Journal of Agricultural Research , 57 ( 8 ) : 857 – 865 .
- Bennett , OL and Doss , BD . 1963 . Effects of soil moisture regime on yield and evapotranspiration from cool-season perennial forage species . Agronomy Journal , 55 : 275 – 278 .
- Black , AL , Brown , PL , Halvorson , AD and Siddoway , FH . 1981 . Dryland cropping strategies for efficient water-use to control saline seeps in the Northern Great Plains, U.S.A . Agricultural Water Management , 4 ( 1–3 ) : 295 – 311 .
- Blaesing D 2003 . Controlling nitrogen lossescan we learn from the European experience? Solutions for a better environment: proceedings of the 11th Australian Agronomy Conference , Geelong, Victoria, 2–6 February 2003. http://www.regional.org.au/au/asa/2003/c/8/blaesing.htm#Top0fPage
- BOM 2009 . Australian Bureau of Meteorology . http://www.bom.gov.au/jsp/ncc/cdio/weatherData/av?p_ncc0bsCode = 139&p_display_type = dataFile&p_stn_num = 73142 (accessed 2 October 2009).
- Brown , HE , Moot , DJ and Pollock , KM . 2005 . Herbage production, persistence, nutritive characteristics and water use of perennial forages grown over 6 years on a Wakanui silt loam . New Zealand Journal of Agricultural Research , 48 : 423 – 439 .
- Christian , KR . 1977 . Effects of the environment on the growth of alfalfa . Advances in Agronomy , 29 : 183 – 227 .
- Cocks , PS . 2001 . Ecology of herbaceous perennial legumes: a review of characteristics that may provide management options for the control of salinity and waterlogging in dryland cropping systems . Australian Journal of Agricultural Research , 52 ( 2 ) : 137 – 151 .
- Connor , DJ . 2004 . Designing cropping systems for efficient use of limited water in southern Australia . European Journal of Agronomy , 21 ( 4 ) : 419 – 431 .
- Cransberg , L and McFarlane , DJ . 1994 . Can perennial pastures provide the basis for a sustainable farming system in southern Australia? . New Zealand Journal of Agricultural Research , 37 : 287 – 294 .
- Dear , BS and Ewing , MA . 2008 . The search for new pasture plants to achieve more sustainable production systems in southern Australia . Australian Journal of Experimental Agriculture , 48 : 387 – 396 .
- Dear , BS , Moore , GA and Hughes , SJ . 2003 . Adaptation and potential contribution of temperate perennial legumes to the southern Australian wheatbelt: a review . Australian Journal of Experimental Agriculture , 43 ( 1 ) : 1 – 18 .
- Dear , BS , Reed , KFM and Craig , AD . 2008 . Outcomes of the search for new perennial and salt tolerant pasture plants for southern Australia . Australian Journal of Experimental Agriculture , 48 : 578 – 588 .
- Dunin , F and Passioura , J . 2006 . Prologue: Amending agricultural water use to maintain production while affording environmental protection through control of outflow . Australian Journal of Agricultural Research , 57 ( 3 ) : 251 – 255 .
- Fillery , IRP and Poulter , RE . 2006 . Use of long-season annual legumes and herbaceous perennials in pastures to manage deep drainage in acidic sandy soils in Western Australia . Australian Journal of Agricultural Research , 57 ( 3 ) : 297 – 308 .
- Gilmour , AR , Cullis , BR , Welham , SJ and Thompson , R . 2006 . ASReml user guide, release 2 , London : VSN International Ltd .
- Hayes , RC , Dear , BS , Li , GD , Virgona , JM , Conyers , MK , Hackney , BF and Tidd , J . 2010 . Perennial pastures for recharge control in temperate drought prone environments. Part 1: productivity, persistence and herbage quality of key species . New Zealand Journal of Agricultural Research , 53 ( 4 ) : 283 – 302 .
- Heng , LK , White , RE , Helyar , KR , Fisher , R and Chen , D . 2001 . Seasonal differences in the soil water balance under perennial and annual pastures on an acid Sodosol in southeastern Australia . European Journal of Soil Science , 52 ( 2 ) : 227 – 236 .
- Hirth , JR , Haines , PJ , Ridley , AM and Wilson , KF . 2001 . Lucerne in crop rotations on the Riverine Plains. 2. Biomass and grain yields, water use efficiency, soil nitrogen, and profitability . Australian Journal of Agricultural Research , 52 ( 2 ) : 279 – 293 .
- Hoffmann JD , Eberbach PL , Virgona JA , Katupitiya A 2003 . Conservative water use by lucerne . Proceedings of the 11th Australian Agronomy conference on solutions for a new environment. Geelong, Victoria, 2–6 February 2003. http://www.regional.org.au/au/asa/2003/c/10/hoffmann.htm#Top0fPage
- Isbell , RF . 1996 . The Australian soil classification , Melbourne : CSIRO .
- Latta , RA , Blacklow , LJ and Cocks , PS . 2001 . Comparative soil water, pasture production, and crop yields in phase farming systems with lucerne and annual pasture in Western Australia . Australian Journal of Agricultural Research , 52 ( 2 ) : 295 – 303 .
- Li , GD , Lodge , GM , Moore , GA , Craig , AD , Dear , BS , Boschma , SP , Albertson , TO , Miller , SM , Harden , S , Hayes , RC and others . 2008 . Evaluation of perennial pasture legumes and herbs to identify species with high herbage production and persistence in mixed farming zones in southern Australia . Australian Journal of Experimental Agriculture , 48 : 449 – 466 .
- Lolicato , SJ . 2000 . Soil water dynamics and growth of perennial pasture species for dryland salinity control . Australian Journal of Experimental Agriculture , 40 ( 1 ) : 37 – 45 .
- Malinowski , DP , Zuo , H , Kramp , BA , Muir , JP and Pinchak , WE . 2005 . Obligatory summer-dormant cool-season perennial grasses for semiarid environments of the Southern Great Plains . Agronomy Journal , 97 : 147 – 154 .
- Norton , MR , Lelièvre , F and Volaire , F . 2006a . Summer dormancy in Dactylis glomerata L.: the influence of season of sowing and a simulated mid-summer storm on two contrasting cultivars . Australian Journal of Agricultural Research , 57 ( 5 ) : 565 – 575 .
- Norton , MR , Volaire , F and Lelièvre , F . 2006b . Summer dormancy in Festuca arundinacea Schreb.; the influence of season of sowing and a simulated mid-summer storm on two contrasting cultivars . Australian Journal of Agricultural Research , 57 ( 12 ) : 1267 – 1277 .
- Reed , KFM , Nie , ZN , Miller , S , Hackney , BF , Boschma , SP , Mitchell , ML , Albertson , TO , Moore , GA , Clark , SG , Craig , AD and others . 2008 . Field evaluation of perennial grasses and herbs in southern Australia. 1. Establishment and herbage production . Australian Journal of Experimental Agriculture , 48 : 409 – 423 .
- Ridley , AM , White , RE , Simpson , RJ and Callinan , L . 1997 . Water use and drainage under phalaris, cocksfoot, and annual ryegrass pastures . Australian Journal of Agricultural Research , 48 ( 7 ) : 1011 – 1024 .
- Ridley , AM , Christy , B , Dunin , FX , Haines , PJ , Wilson , KF and Ellington , A . 2001a . Lucerne in crop rotations on the Riverine Plains. 1. The soil water balance . Australian Journal of Agricultural Research , 52 ( 2 ) : 263 – 277 .
- Ridley , AM , White , RE , Helyar , KR , Morrison , GR , Heng , LK and Fisher , R . 2001b . Nitrate leaching loss under annual and perennial pastures with and without lime on a duplex (texture contrast) soil in humid southeastern Australia . European Journal of Soil Science , 52 ( 2 ) : 237 – 252 .
- Sandral , GA , Dear , BS , Virgona , JM , Swan , AD and Orchard , BA . 2006 . Changes in soil water content under annual- and perennial-based pasture systems in the wheatbelt of southern New South Wales . Australian Journal of Agricultural Research , 57 ( 3 ) : 321 – 333 .
- Scott , BJ , Ridley , AM and Conyers , MK . 2000 . Management of soil acidity in long-term pastures of south-eastern Australia: a review . Australian Journal of Experimental Agriculture , 40 ( 8 ) : 1173 – 1198 .
- Scott , BJ , Ewing , MA , Williams , R , Humphries , A and Coombes , NE . 2008 . Tolerance of aluminium toxicity in annual Medicago species and lucerne . Australian Journal of Experimental Agriculture , 48 : 499 – 511 .
- Singh , DK , Bird , PR and Saul , GR . 2003 . Maximising the use of soil water by herbaceous species in the high rainfall zone of southern Australia: a review . Australian Journal of Agricultural Research , 54 ( 7 ) : 677 – 691 .
- Ward , PR . 2006 . Predicting the impact of perennial phases on average leakage from farming systems in south-western Australia . Australian Journal of Agricultural Research , 57 ( 3 ) : 269 – 280 .
- Ward , PR , Micin , SF and Dunin , FX . 2006 . Using soil, climate, and agronomy to predict soil water use by lucerne compared with soil water use by annual crops or pastures . Australian Journal of Agricultural Research , 57 ( 3 ) : 347 – 354 .