Abstract
Classical weed biocontrol has long been criticised, worldwide, for a lack of post-release long-term monitoring. This paper reports on a rare opportunity to follow up, three agents and 35 years later, a biocontrol programme against nodding thistle (Carduus nutans) in New Zealand. In New Zealand, the establishment of all three agents has been reported previously, but impacts have only been assessed for the receptacle weevil (Rhinocyllus conicus). This paper reports on the abundance of the nodding thistle crown weevil (Trichosirocalus horridus), and on the abundance, phenology and impact of the seed predators, R. conicus and Urophora solstitialis, in Canterbury, based on field surveys and, for the seed predators, also from a cultivated population. Trichosirocalus horridus was abundant at all field sites and on rosettes of all sizes. Seed destruction per larva by R. conicus (34 seeds per larva) was similar to previous estimates, but U. solstitialis achieved rates (21 seeds per larva) three times higher than estimates elsewhere. Population-level seed predation by R. conicus (14 and 4% in the cultivated and field populations, respectively), by U. solstitialis (9 and 7%) and cumulatively by both (23 and 11%) is insufficient to cause nodding thistle populations in New Zealand to decline. The results show that the introduction of multiple biocontrol agents does not guarantee increased pressure on a target weed host population.
Introduction
Classical biological control is an important tool in the battle against weeds in New Zealand, and many resources are invested in host testing, introduction, mass rearing and release of agents. Analysis of an agent's success or failure is essential for improving future practices, as well as for the justification of the practice altogether. Indeed, much has been said regarding the importance of long-term monitoring in enhancing the scientific foundations of the practice (e.g. Blossey Citation1999), and biological control programmes have been criticised for lack of post-release long-term monitoring (see references within McFadyen Citation1998). However, long-term monitoring is expensive and rarely done, and rarely does monitoring of a biocontrol programme go beyond verifying agent establishment (McClay Citation1992). The study reported here presented a rare opportunity, as part of a larger study, the general aim of which was to study multi-species interactions in nodding thistle biocontrol and to assess a weed biocontrol programme against nodding (musk) thistle, Carduus nutans L. (Asteraceae), 35 years after its initiation. To that end, the study involved measurement of the abundance of three and the impact of two agents introduced against the weed between 16 and 35 years prior to this work.
A common paradigm in biological control of weeds is that more than one agent is required to produce successful control. Two conflicting hypotheses lead to this paradigm. Firstly, that multiple sources of stress (which could be generated, for example, by multiple biocontrol agents) are usually necessary to sufficiently affect the weed (e.g. Harris Citation1981). Secondly, that only one agent is required for successful control but, not knowing in advance which is the ‘silver bullet’ agent, multiple introductions increase the chances of it actually being introduced (the ‘lottery ticket’ model (Myers Citation1985)). Evidence exists in support of both hypotheses, and the fact remains that introduction of multiple agents improves programme success rate (Denoth et al. Citation2002). Between 1972 and 1992, a suite of three agents, including two seed predators and one crown-root feeder, was introduced to New Zealand against C. nutans. The later-introduced seed predator, Urophora solstitialis L. (Diptera: Tephritidae), was brought in to increase pressure at the time of year not covered by the earlier introduction of Rhinocyllus conicus (Froehlich) (Coleoptera: Curculionidae). However, it was suspected that the phenology of the two seed predators is such that they co-occur at a critical time for U. solstitialis, which could limit the fly's ability to build up high populations.
Establishment in New Zealand of all three biocontrol agents is well documented (Jessep Citation1975, Citation1989b; Harman et al. Citation1996; Hayes Citation2007). However, impact on the target weed has been studied only for R. conicus (Popay et al. Citation1984; Kelly & McCallum Citation1990, 1995; Kelly et al. Citation1990; Kelly & Wood Citation1991; Shea & Kelly Citation1998).
The aim of this work was to address this gap in knowledge on C. nutans biocontrol agents in New Zealand. Specifically, to:
1. | measure the abundance of larval stages of the crown-root feeder, Trichosirocalus horridus (Panzer) (Coleoptera: Curculionidae) at variable rosette sizes in Canterbury | ||||
2. | describe the phenology of R. conicus and U. solstitialis | ||||
3. | measure the impact of R. conicus and U. solstitialis on seed production by C. nutans. |
Methods
Study organisms
Carduus nutans is a monocarpic herbaceous facultative-biennial (in the terminology of Kelly Citation1985), and a serious pasture weed in many parts of New Zealand (Jessep Citation1989a). It reproduces only by seeds (Popay & Medd Citation1990), and is highly fecund (McCarty Citation1982; Jessep Citation1990; Popay & Medd Citation1990). The first biocontrol agent, introduced to New Zealand in 1972, was the receptacle weevil, Rhinocyllus conicus (Froehlich) (Coleoptera: Curculionidae) from Canada (Agriculture Canada, Regina Station), ex Upper Rhine Valley, France (Jessep Citation1989a). The weevil larvae develop by feeding on the receptacle tissue of C. nutans (and other thistles), which prevents seed development (Zwölfer & Harris Citation1984). Rhinocyllus conicus is normally univoltine and can exhibit a partial second generation if first-generation larvae complete their development when day length is 16 h or longer (Harris Citation1984). Rhinocyllus conicus was reported to reduce early-season viable seed production of C. nutans by 78% in Nebraska (McCarty & Lamp Citation1982); however, this figure is probably an overestimate (Kelly & McCallum Citation1995). In Virginia (USA), seed loss to R. conicus was estimated at about 35% (Surles & Kok Citation1978). Successful control of C. nutans by R. conicus was reported in Virginia (Kok & Surles Citation1975), Canada (Harris Citation1984) and Oklahoma (Roduner et al. Citation2003). In Australia, R. conicus is estimated to reduce C. nutans seed production by 7–36% (Woodburn Citation1996b, Citation1997; Woodburn & Briese Citation1996), whereas in New Zealand it is estimated to reduce annual seed production in C. nutans by 36% on average (Kelly & McCallum 1990). In its native range in Europe, R. conicus is estimated to reduce C. nutans seed production by 35% (Sheppard et al. Citation1994).
The crown-root feeding weevil Trichosirocalus horridus (Panzer) (Coleoptera: Curculionidae) was the second biocontrol agent introduced to New Zealand against C. nutans, in 1982, from Canada (Agriculture Canada, Regina Station), ex Germany (Jessep Citation1989a). The species has been revised (Alonso-Zarazaga & Sánchez-Ruiz Citation2002) and the identity of the New Zealand fauna is left unresolved (Groenteman et al. Citation2008). The species is treated here as T. horridus. Trichosirocalus horridus larvae consume meristem tissue in the crown-root zone of the rosettes of C. nutans. At high density, larval feeding can kill a plant; surviving plants suffer reduced growth and become spineless and hence more palatable to livestock (Woodburn Citation1997). Trichosirocalus horridus is univoltine, but has an extended oviposition period in New Zealand (Jessep Citation1989a). In Australia, T. horridus is estimated to reduce C. nutans population-level seed production by 72% (Woodburn Citation1997). In Oklahoma, C. nutans densities decreased faster at sites with T. horridus (and R. conicus) than in sites with no T. horridus (only with R. conicus (Roduner et al. 2003)).
The third C. nutans biocontrol agent, the gall-forming fly Urophora solstitialis L. (Diptera: Tephritidae), was introduced in 1992 from a French strain that was first established in Australia (Hayes Citation2007). Larvae induce the formation of woody galls in the receptacle of C. nutans capitula, which not only prevent seed development within the attacked capitulum but also function as strong sinks for assimilates (Harris Citation1989). Urophora solstitialis is bivoltine; in New Zealand, its first generation coincides with R. conicus and its second generation is active later, after R. conicus has disappeared, and when the majority of C. nutans capitula are produced. In Australia, a 45% reduction in seed set was achieved in the first year after U. solstitialis introduction (Woodburn Citation1996a; Woodburn & Cullen Citation1996) and, in later years, 70% reduction of C. nutans seed rain was attributed largely to U. solstitialis (Woodburn & Briese Citation1996). In its native range in Europe, where a large number of natural enemies of C. nutans as well as U. solstitialis are present, it was estimated to account for only 15% of seed reduction (Sheppard et al. 1994).
Experiment 1: T. horridus abundance in C. nutans rosettes—a field survey
The pattern of T. horridus attack on C. nutans was surveyed in North Canterbury and two areas of Mid-Canterbury (Rakaia Gorge and Ashburton). Three sites in each of the three areas (nine sites in total) were haphazardly selected, in which C. nutans rosettes were sampled twice, in winter 2005 and winter 2006. Sampling during winter represents peak level of T. horridus larval activity and avoids the resulting peak rosette mortality attributed to T. horridus that occurs in late spring. Site locations are detailed in .
Table 1 Details of the sites used for T. horridus abundance survey (experiment 1).
At each site, five points were randomly selected at which the six nearest C. nutans rosettes within a radius of 3 m were dug up, their longest leaf length (cm) was measured and the root crown taken to the laboratory. If there were insufficient rosettes, more random points were sampled until there were at least 30 per site. Previous work in New Zealand established a strong relationship between longest leaf length and rosette total area in C. nutans (Kelly & Popay Citation1985). Work in Virginia found that larger rosettes were significantly more prone to attack by T. horridus (Sieburth & Kok Citation1982). Therefore, to avoid a zero-inflated dataset, extra samples of large rosettes (longest leaf ≥ 20 cm) were taken where the original 30 plants were in the smaller range.
In the laboratory, T. horridus larvae were counted from a subsample of at least 20 rosettes per site (except in three cases where fewer than 20 rosettes were processed). Although first-instar larvae do not cause much damage, they were counted because they are an indicator of initial oviposition. Leaf lengths and crown-root diameters were divided into categories. The distributions of attack (number of larvae of all instars pooled per rosette) per leaf-size category are presented. Analysis by leaf-size category would be useful in future non-destructive estimates of field infestations. Crown area was estimated from root-crown diameter and the number of larvae per unit area of crown was regressed against rosette size (expressed as longest leaf length).
Experiment 2: seed predator phenology and impact on C. nutans cultivated population
Carduus nutans plants were grown in the University of Canterbury (Christchurch) glasshouses from seeds collected in summer 2005 around Canterbury. In late autumn 2005, 120 plants were transplanted as small rosettes to an uncultivated grassy area that was regularly mown, at the Landcare Research Lincoln campus (43°38.4′S, 172°28.5′E), as part of a larger experiment (Groenteman Citation2007). The site was naturally colonised by R. conicus and U. solstitialis (i.e. no controlled releases of biocontrol agents were made). The plants were checked for ripening capitula frequently (every 2–10 days, depending on ripening rate). Ripe capitula were harvested and their height, position on the plant (primary (=terminal), secondary (=lateral on a primary branch) or tertiary (=lateral on a secondary branch)) and external diameter were recorded. The number of capitula was counted on each plant at each harvesting event. Capitula were stored individually in paper bags at ambient temperature to allow time for seed predator development, after which their receptacle diameter was measured and any signs of attack by R. conicus and U. solstitialis recorded. Rhinocyllus conicus attack signs included density of egg covers, numbers of larvae, pupae and adults, and the presence of typical feeding damage. Egg covers are made out of masticated plant tissue by ovipositing females; their presence was recorded as: 0 = none; 1 = low (<5 egg covers); 2 = medium (up to one third of the capitulum covered); 3 = high (more than one third of the capitulum covered). Urophora solstitialis attack signs includes numbers of larvae, pupae adults, and excess empty galls. Viable seeds were counted for most capitula damaged by the biocontrol agents. The first capitulum ripened on 19 December 2005 and collections continued until 16 May 2006. Of the 90 plants that bolted successfully, about two thirds were harvested in full during this time; the remainder of the plants, of which not all capitula had ripened by 16 May, were destroyed.
A formula established by Popay et al. (1984) for C. nutans in New Zealand was used to estimate seed output per capitulum (in the absence of seed predators) from receptacle area:
The number of seeds destroyed per plant was estimated for the plants for which at least 80% of capitula were harvested and processed (58 out of 90 plants that produced any capitula). For each of these plants, an estimate of seed output in the absence of seed predation was produced: actual seed counts were used for unattacked capitula and Equation (Equation1) was used to estimate seed output of attacked capitula. These were summed to produce an estimate of potential seed output for each plant in the absence of seed predation (K). Another seed output estimate, in the presence of seed predation (U) was then calculated for each plant using Equation Equation1 for unattacked capitula, and, for damaged capitula, using either actual seed counts or seed loss estimates calculated from the number of insects (extracted from the individual capitulum) multiplied by the per-larva predation estimates (calculated above). Seed production per plant accounting for predation was estimated for each seed predator separately (i.e. excluding capitula which hosted both insects) and for both seed predators together (i.e. including capitula with both insects present). Proportional seed loss per plant (T) for the ith seed predator and for their combined effect was therefore:
Experiment 3: field population
During the same time as the cultivated population was being monitored at Lincoln, a naturally occurring population at the Mid-Canterbury Hamptons Rd site (see T. horridus survey section above) was monitored for seed predator attack. Beginning on 20 December 2005 (1 day after the first ripe capitulum was collected from the cultivated population), the site was visited approximately once a fortnight. The number of flowering plants and the number of flowering capitula (i.e. those with at least one floret open) were recorded, and all ripe capitula were collected from a strip plot of 34×1 m. In the laboratory, these capitula were measured (external diameter and receptacle diameter) and the presence, number and instars of larval R. conicus and U. solstitialis recorded. Sampling ceased in mid-autumn (8 May 2006) when very few plants were still flowering and no seed predators were found in two consecutive samples.
Seed loss in the field population was estimated for the entire season in the following manner. The proportion (F) of seed destroyed per capitulum was calculated for the ith seed predator and for their combined effect:
Results
T. horridus abundance in C. nutans rosettes
T. horridus attack increased with increased rosette size in both 2005 and 2006, both absolutely, with more larvae per rosette in larger rosettes (), and proportionally, with significantly more larvae per crown unit area in larger rosettes (F 1,170=15.63, P<0.001; ). While rosette size explained only 8% of the variation (regression analysis), the increase in mean larval density was substantial: from 0.05/mm2 of root-crown in small rosettes (5 cm leaf) to six times that density in large rosettes (30 cm leaf; ). In all regions and years, a high proportion of rosettes (range 59–86%) was infested with larvae ().
Figure 1 Mean number (± SEM) of Trichosirocalus horridus larvae (all instars) per Carduus nutans crown (A) at four leaf-length categories in 2005 and (B) at three categories in 2006, in three regions of Canterbury (Culverden, Rakaia Gorge, Ashburton), in experiment 1. Missing error bar in Rakaia Gorge region in 2005 in the size category >30 is due to the presence of only one rosette in this category.
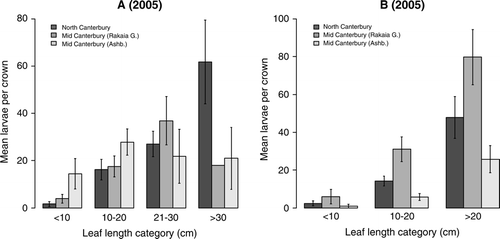
Figure 2 Number of Trichosirocalus horridus larvae per unit area (mm2) of Carduus nutans crown at growing rosette size as indicated by longest leaf length (cm). N larvae=0.041 + leaf length (cm)×0.009, F 1,170=15.63, P<0.001, adjusted r 2=0.079.
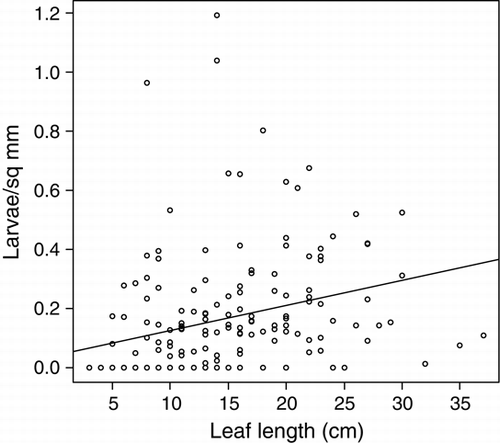
Table 2 Mean percentage (± SEM) of Carduus nutans rosettes infested with Trichosirocalus horridus larvae in three regions of Canterbury (Culverden, Rakaia Gorge, Ashburton) over two consecutive winters in experiment 1.
Seed predator phenology and impact on C. nutans
Plants in the cultivated population (experiment 2) produced 47.5±6.3 capitula and 6873±1097 seeds per plant. The proportion of capitula attacked by the seed predators revealed a similar pattern in the cultivated (experiment 2) and field (experiment 3) populations (). The results portray a single generation of R. conicus attacking early in the season, a low peak of first-generation U. solstitialis 6–8 weeks later, which overlaps with part of R. conicus activity, and a second generation of U. solstitialis about 3 months into the season.
Figure 3 Proportion of Carduus nutans capitula attacked by Rhinocyllus conicus (dark) and Urophora solstitialis (light) in: (A) the cultivated population at Landcare Research Lincoln (experiment 2) between 19 December 2005 and 16 May 2006 (all ripe capitula were collected); (B) the field population in Mid-Canterbury (experiment 3) between 20 December 2005 and 8 May 2006 (all ripe capitula were collected from a strip plot of 34×1 m approximately once a fortnight). The number above each bar represents the number of capitula collected on each occasion.
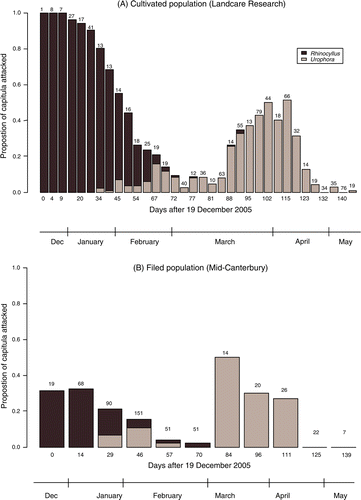
The vast majority of primary capitula in experiment 2 suffered attack by one of the seed predators (). Also, anecdotally, it appears that when both seed predators are present simultaneously (in late January), R. conicus utilises more primary capitula than U. solstitialis. Nonetheless, despite the fewer capitula attacked by U. solstitialis in the first days of its appearance, it maintains a high proportion of its attack on primary capitula ().
Figure 4 Proportion of Carduus nutans capitula attacked by Rhinocyllus conicus (dark) and Urophora solstitialis (light) on each collection date, out of those capitula that ripened on that date in the cultivated population (experiment 2), partitioned by position on the plant. The number above each bar represents the number of capitula collected at each combination of position and date.
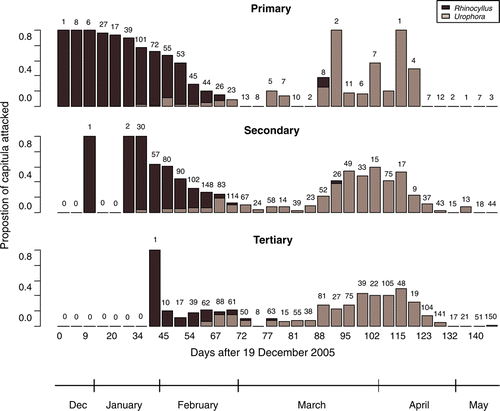
Seed destruction per larva in experiment 2 was estimated at 34.3±2.2 for R. conicus and 21.3±1 for U. solstitialis. Partitioning of seed destruction by larval density shows very high destruction per larva at low densities (1–4 larvae per capitulum) compared with densities of five or more in both seed predators (). Seed loss per plant to U. solstitialisreached similar estimates in both the cultivated (T×100 = 8.8%±1.2) and field (L×100 = 7.2%) populations, while seed loss to R. conicus appeared higher in the cultivated population (T×100 = 14.3%±1.7) compared with the field population (L×100 = 4.2%), in accordance with the overall lower attack associated with R. conicus in the field population (). Combined seed losses to the two seed predators were T×100 = 22.8%±1.9 and L×100 = 11.3% in the cultivated and field populations, respectively.
Discussion
Trichosirocalus horridus abundance
Populations of facultative-biennial weeds represent multiple cohorts of germination with great size variability, and their biocontrol can greatly benefit from root-feeding agents that distribute attack over various sizes (Briese et al. Citation2002). Trichosirocalus horridus larvae in this survey (experiment 1) were found in rosettes of all size categories (). In many cases, however, especially at early stages of infestation, signs of attack could not be detected without destruction of the crown (R. Groenteman, personal observation); this may explain why a study in Virginia, which used only non-destructive methods, failed to detect attack in small rosettes (Sieburth & Kok Citation1982). In this survey, more T. horridus larvae were found in larger rosettes in Canterbury (). This trend was clear in both 2005 and 2006, and across all three regions, and persisted even when data were corrected for unit area, such that plants with larger rosette area had higher T. horridus larval densities (). The significance of this result for biological control is further illustrated by Briese et al. (2002) who showed that increased densities of T. briesei resulted in a similar rate of increase of attack on both small and large rosettes of Onopordum spp. Thus, they suggest that, provided agent populations are high enough, all plants could be attacked at a damaging level. The mechanism proposed for higher attack on larger plants was not necessarily greater attraction to larger plants, but rather a higher frequency of encounters with larger plants and a tendency of females to remain longer on larger plants and thus deposit more eggs in that time. Gerber et al. (Citation2007) add (in a similar context) that larger plants may simply exhibit higher numbers of suitable oviposition sites. Greater attack on larger plants has also been documented in other root feeders (Prins et al. Citation1992; Pomerinke et al. Citation1995; Gerber et al. Citation2007).
Seed predator abundance and impact on C. nutans
The co-occurrence of the two seed predators within individual capitula was intriguingly low, especially considering the overlap in time between R. conicus and first-generation U. solstitialis (): out of 967 attacked capitula, only 18 showed detectable signs of attack by both seed predators and only 16 (1.7%) were actually populated by larvae of both in the cultivated population (experiment 2). In the field population (experiment 3) only one out of 93 attacked capitula was attacked by both agents. Urophora solstitialis was more abundant in its second generation than the first (), and it is suspected that more capitula in fact suffer attack by both seed predators earlier in the season; however, no evidence is left so that the results underestimate this attack. This could be the result of either or both the following processes. Firstly, both agents oviposit in the same (preferred) capitula, but U. solstitialis larvae are preyed on by R. conicus before they manage to harden a gall (Harris Citation1989), with no traces left of their presence. Alternatively, Woodburn & Sheppard (Citation1995) claim U. solstitialis actively avoid ovipositing in capitula containing R. conicus.
Regardless of the mechanism involved, it appears that potentially fewer U. solstitialis emerge from the generation that coincides with R. conicus than could be expected in its absence. This means that C. nutans capitula developing later in the season (i.e. the vast majority in New Zealand in general and in Canterbury in particular) are potentially exposed to fewer U. solstitialis than would be expected in the absence of R. conicus. Although a genuine low occurrence of U. solstitialis early in the season has yet to be ruled out as the factor limiting first-generation numbers, there is good indication from an Australian study that high densities of R. conicus early in the season reduce U. solstitialis numbers in the first generation (Woodburn Citation1996b). Interestingly, McNeill & Fletcher (Citation2005) recorded 17% co-occurrence of R. conicus and U. solstitialis in a sample of 105 mainly primary C. nutans capitula from Canterbury; however, their sampling ceased in early summer (mid-December) whereas this study continued into autumn (mid-May).
Seed destruction per larva varied significantly between insect densities of 1–4 and ≥5 individuals per capitulum (). This pattern may be the result of truncation; negative values of seed destruction were not allowed and were replaced with zeroes. This artificially increases the mean, especially at the lower insect densities, in which the vast majority of negative values arose. It is also possible that the pattern is real, i.e. that at low densities seed destruction per larva is indeed higher than at high densities. This could rise from intraspecific competition for limited resources between larvae at high densities. However, the larval densities used to estimate seed predation were recorded after any intraspecific competitive interactions. The sample considered here accounts for the entire season, all capitula positions and a range of insect densities per capitulum; therefore, the grand mean estimates of seed destruction per larva should be reliable. The estimate of 34.3 seeds lost per R. conicus larva is comparable with previous estimates from New Zealand (29.6 (Popay et al. 1984), 27.7 (Kelly et al. 1990)), Australia (29 (Woodburn Citation1996b)) and Europe (26 (Sheppard et al. 1994)). Seed loss per U. solstitialis larva was estimated at 6.9 in Australia (Woodburn Citation1996a), similar to the 7.0 estimated in Europe (Sheppard et al. 1994)—both much lower than the 21.3 estimated in this study.
In light of the high calculated seed predation per U. solstitialis larva, the total seed reductions of 9% (T) in the cultivated population (experiment 2) and 7% (L) in the field population (experiment 3) indicate a surprisingly low density of this agent. Seed reduction by U. solstitialis at an Australian site with R. conicus was estimated at only 11% (Woodburn Citation1997), much lower than the estimates for its effect when acting on its own (>45% (Woodburn & Briese Citation1996)), which may provide more evidence for interference between these two agents. The 14 and 4% seed reductions by R. conicus in the cultivated and field populations respectively are similar to the previous field estimate for Canterbury of 3% (Kelly et al. 1990). Note, however, that earlier assessments (Kelly & Wood Citation1991) of the impact of R. conicus alone found higher losses in the North Island than in Canterbury, where the current study was also based. For New Zealand, Shea et al. (Citation2005, Citation2010) predicted that U. solstitialis would have a greater effect as the only agent present.
The total seed reduction of 23% (T) in the cultivated population (experiment 2) and only 11% (L) in the field population (experiment 3) is far short of the 69% estimated required to bring C. nutans populations in New Zealand to decline (Shea & Kelly Citation1998), highlighting the debate surrounding the use of seed predators as biocontrol agents (e.g. van Klinken & Flack Citation2008; Garren & Strauss Citation2009).
Concluding remarks
Thirty-five years into the biocontrol programme and three agents later, C. nutans is still a major weed in parts of New Zealand. The main finding of the study presented here is that the overall level of seed destruction in C. nutans hardly changed, at least in Canterbury, with the introduction of the potentially highly effective agent, U. solstitialis. This is perhaps because early larvae of U. solstitialis may be negatively affected by predation from R. conicus larvae (Harris Citation1989; Groenteman et al. Citation2007). This result reveals that the impact of multiple biocontrol agents cannot necessarily be expected to increase pressure on the target weed host population and increases the importance of considering which species to introduce and in which order.
Acknowledgements
We thank Caroline Thomson, Kat Bushman, Sarah Luxton, Morgan Coleman, Helen Parish, Hagar Shaviv, Emri Dolev, Nicolette LeCren, Jennie Iles and Lindsay Smith for field and laboratory assistance and Dave Saville for statistical advice. The manuscript was improved by comments from two anonymous referees. This project was funded by the New Zealand Foundation for Research, Science and Technology under the Outsmarting Weeds programme, contract no. C10X0318.
References
- Alonso-Zarazaga , MA and Sánchez-Ruiz , M . 2002 . Revision of the Trichosirocalus horridus (Panzer) species complex, with description of two new species infesting thistles (Coleoptera: Curculionidae, Ceutorhynchinae) . Australian Journal of Entomology , 41 : 199 – 208 .
- Blossey , B . 1999 . Before, during and after: the need for long-term monitoring in invasive plant species management . Biological Invasions , 1 : 301 – 311 .
- Briese , DT , Thomann , T and Vitou , J . 2002 . Impact of the rosette crown weevil Trichosirocalus briesei on the growth and reproduction of Onopordum thistles . Journal of Applied Ecology , 39 : 688 – 698 .
- Denoth , M , Frid , L and Myers , JH . 2002 . Multiple agents in biological control: improving the odds? . Biological Control , 24 : 20 – 30 .
- Garren , JM and Strauss , SY . 2009 . Population-level compensation by an invasive thistle thwarts biological control from seed predators . Ecological Applications , 19 : 709 – 721 .
- Gerber , E , Hinz , HL and Blossey , B . 2007 . Impact of the belowground herbivore and potential biological control agent, Ceutorhynchus scrobicollis, on Alliaria petiolata performance . Biological Control , 42 : 355 – 364 .
- Groenteman R Multi-species interactions in weed biocontrol: a Carduus nutans case study . Unpublished PhD thesis , University of Canterbury Christchurch 2007 .
- Groenteman , R , Kelly , D , Fowler , SV and Bourdôt , GW . 2007 . Interactions between nodding thistle seed predators . New Zealand Plant Protection , 60 : 152 – 157 .
- Groenteman R , Kelly D , Fowler SV , Bourdôt GW 2008 . Which species of the thistle biocontrol agent Trichosirocalus are present in New Zealand? In : Julien MH et al. Proceedings of XII international symposium on biological control of weeds . CAB International , Wallingford, , UK 145 149
- Harman , HM , Syrett , P , Hill , RL and Jessep , CT . 1996 . Arthropod introductions for biological control of weeds in New Zealand, 1929–1995 . New Zealand Entomologist , 19 : 71 – 80 .
- Harris , P . 1981 . Stress as a strategy in the biological control of weeds . Beltsville Symposia in Agricultural Research , 5 : 333 – 340 .
- Harris , P . 1984 . “ Carduus nutans L., nodding thistle and C. acanthoides L., plumeless thistle (Compositae) ” . In Biological control programs against insects and weeds in Canada 1969–1980 , Edited by: Kelleher , JS and Hume , MA . 115 – 126 . Farnham, , UK : Commonwealth Agricultural Bureaux .
- Harris , P . 1989 . “ Feeding strategy, coexistance and impact of insects in spotted knapweed capitula ” . In Proceedings of the VII international symposium on biological control of weeds , Edited by: Delfosse , ES . 39 – 47 . Melbourne : CSIRO .
- Hayes L 2007 . Nodding thistle gall fly Urophora solstitialis . http://www.landcareresearch.co.nz/research/biocons/weeds/book/documents/Nodding_Thistle_Gall_Fly.pdf (accessed 12 November 2007) .
- Jessep , CT . 1975 . Introduction of a weevil for the biological control of nodding thistle . Proceedings of New Zealand Weed and Pest Control Conference , 28 : 205 – 206 .
- Jessep , CT . 1989a . “ Carduus nutans L., nodding thistle (Asteraceae) ” . In A review of biological control of invertebrate pests and weeds in New Zealand 1874 to 1987 , Edited by: Cameron , PJ , Hill , RL , Bain , J and Thomas , WP . 339 – 342 . Wallingford, , UK : CAB International .
- Jessep , CT . 1989b . Introduction of the crown weevil (Trichosirocalus horridus) as an additional biocontrol agent against nodding thistle . Proceedings of New Zealand Weed and Pest Control Conference , 42 : 52 – 54 .
- Jessep , CT . 1990 . Aspects of the biology of nodding thistle (Carduus nutans L.) in Canterbury, New-Zealand . New Zealand Journal of Agricultural Research , 33 : 173 – 183 .
- Kelly , D . 1985 . On strict and facultative biennials . Oecologia , 67 : 292 – 294 .
- Kelly , D and McCallum , K . 1990 . “ Demography, seed biology and biological control of Carduus nutans in New Zealand ” . In The biological control of invasive plants: proceedings of a conference of the British Ecological Society , Edited by: Palmer , JP . 72 – 79 . Cardiff : British Ecological Society .
- Kelly , D and McCallum , K . 1995 . “ Evaluating the impact of Rhinocyllus conicus on Carduus nutans in New Zealand ” . In Proceedings of the VIII international symposium on biological control of weeds , Edited by: Delfosse , ES and Scott , RR . 205 – 212 . Melbourne : CSIRO .
- Kelly , D and Popay , AI . 1985 . Pasture production lost to unsprayed thistles at two sites . Proceedings of the New Zealand Weed and Pest Control Conference , 38 : 115 – 118 .
- Kelly , D and Wood , R . 1991 . Why nodding thistle receptacle weevil destroys so little nodding thistle seed in Canterbury . Proceedings of New Zealand Weed and Pest Control Conference , e44 : 280 – 283 .
- Kelly , D , McCallum , K , Schmidt , CJ and Scanlan , PM . 1990 . Seed predation in nodding and slender winged thistles by nodding thistle receptacle weevil . Proceedings of New Zealand Weed and Pest Control Conference , 43 : 212 – 215 .
- Kok , LT and Surles , WW . 1975 . Successful biocontrol of musk thistle Campanulatae: Compositae by an introduced weevil, Rhinocyllus conicus Coleoptera: Curculionidae . Environmental Entomology , 4 : 1025 – 1027 .
- McCarty , MK . 1982 . Musk thistle (Carduus thoermeri) seed production . Weed Science , 30 : 441 – 445 .
- McCarty , MK and Lamp , WO . 1982 . Effect of a weevil, Rhinocyllus conicus, on musk thistle (Carduus thoermeri) seed production . Weed Science , 30 : 136 – 140 .
- McClay , AS . 1992 . “ Beyond ‘before-and-after’: experimental design and evaluation in classical weed biocontrol ” . In Proceedings of the VIII international symposium on biological control of weeds , Edited by: Delfosse , ES and Scott , RR . 213 – 219 . Walling ford, , UK : CAB International .
- McFadyen , REC . 1998 . Biological control of weeds . Annual Review of Entomology , 43 : 369 – 393 .
- McNeill , MR and Fletcher , CJ . 2005 . Interspecific competition between Rhinocyllus conicus and Urophora solstitialis L. on nodding thistle in Canterbury? . New Zealand Plant Protection , 58 : 140 – 147 .
- Myers , JH . 1985 . “ How many insect species are necessary for successful biocontrol of weeds? ” . In Proceedings of the VI international symposium on biological control of weeds , Edited by: Delfosse , ES . 77 – 82 . Ottawa, Canada, Agriculture Canada .
- Pomerinke , MA , Thompson , DC and Clason , DL . 1995 . Bionomics of Cleonidius trivittatus (Coleoptera: Curculionidae): native biological control of purple locoweed (Rosales: Fabaceae) . Environmental Entomology , 24 : 1696 – 1702 .
- Popay , AI and Medd , RW . 1990 . The biology of Australian weeds 21. Carduus nutans L. ssp. nutans . Plant Protection Quarterly , 5 : 3 – 13 .
- Popay , AI , Lyttle , LA , Edmonds , DK and Phung , HT . 1984 . Incidence of the nodding thistle receptacle weevil on nodding and slender winged thistles . Proceedings of New Zealand Weed and Pest Control Conference , 37 : 28 – 32 .
- Prins , AH , Nell , HW and Klinkhamer , PGL . 1992 . Size-dependent root herbivory on Cynoglossum officinale . Oikos , 65 : 409 – 413 .
- Roduner , MA , Cuperus , G , Mulder , P , Stritzke , J and Payton , M . 2003 . Successful biological control of the musk thistle in Oklahoma using the musk thistle head weevil and the rosette weevil . American Entomologist , 49 : 112 – 120 .
- Shea , K and Kelly , D . 1998 . Estimating biocontrol agent impact with matrix models: Carduus nutans in New Zealand . Ecological Applications , 8 : 824 – 832 .
- Shea , K , Kelly , D , Sheppard , AW and Woodburn , TL . 2005 . Context-dependent biological control of an invasive thistle . Ecology , 86 : 3174 – 3181 .
- Shea , K , Jongejans , E , Skarpaas , O , Kelly , D and Sheppard , AW . 2010 . Optimal management strategies to control local population growth or population spread may not be the same . Ecological Applications , 20 : 1148 – 1161 .
- Sheppard , AW , Cullen , JM and Aeschlimann , JP . 1994 . Predispersal seed predation on Carduus nutans (Asteraceae) in southern Europe . Acta Oecologica , 15 : 529 – 541 .
- Sieburth , PJ and Kok , LT . 1982 . Ovipositional preference of Trichosirocalus horridus (Coleoptera: Curculionidae) . Canadian Entomologist , 114 : 1201 – 1202 .
- Surles , WW and Kok , LT . 1978 . Carduus thistle seed destruction by Rhinocyllus conicus . Weed Science , 26 : 264 – 269 .
- van Klinken , RD and Flack , LK . 2008 . What limits predation rates by the specialist seed-feeder Penthobruchus germaini on an invasive shrub? . Journal of Applied Ecology , 45 : 1600 – 1611 .
- Woodburn , TL . 1996a . “ Reduction of seed set in nodding thistle (Carduus nutans) by the seed-fly, Urophora solstitialis, in Australia ” . In Fruit fly pests: a world assessment of their biology and management , Edited by: McPheron , BA and Steck , GJ . 165 – 169 . Delray Beach, Florida : St Lucie Press .
- Woodburn , TL . 1996b . “ Interspecific competition between Rhinocyllus conicus and Urophora solstitialis, two biocontrol agents released in Australia against Carduus nutans ” . In Proceedings of the IX international symposium on biological control of weeds , Edited by: Moran , VC and Hoffmann , JH . 409 – 415 . Rondebosch, , South Africa : University of Cape Town .
- Woodburn , TL . 1997 . Establishment in Australia of Trichosirocalus horridus a biological control agent for Carduus nutans, and preliminary assessment of its impact on plant growth and reproductive potential . Biocontrol Science and Technology , 7 : 645 – 656 .
- Woodburn , TL and Briese , DT . 1996 . The contribution of biological control to the management of thistles . Plant Protection Quarterly. , 11 : 250 – 253 .
- Woodburn , TL and Cullen , JM . 1996 . “ Impact of Rhinocyllus conicus and Urophora solstitialis on seed set in Carduus nutans in Australia ” . In Proceedings of international compositae conference , Edited by: Hind , NJ . 307 – 319 . Kew, , UK : Royal Botanic Gardens .
- Woodburn , TL and Sheppard , AW . 1995 . “ Urophora solstitialis, a potential biological control agent for Carduus nutans in Australia ” . In Proceedings of the VIII symposium on biological control of weeds , Edited by: Delfosse , ES and Scott , RR . 415 Melbourne : CSIRO .
- Zwölfer , H and Harris , P . 1984 . Biology and host specificity of Rhinocyllus conicus (Froel) (Col: Curculionidae), a successful agent for biocontrol of the thistle, Carduus nutans L . Zeitschrift fur Angewandte Entomologie (Journal of Applied Entomology) , 97 : 36 – 62 .