Abstract
Terrestrial Alternanthera philoxeroides is difficult to control with current tools. Shading as a control tool depends on the relative shade tolerances of the target weed and co-occurring species. This study examined A. philoxeroides’ shade tolerance with inter-specific competition from pasture species. In glasshouse conditions, shade and competition reduced A. philoxeroides growth but did not cause senescence. Grasses senesced in response to shade. In the field, competing species’ biomass reduced by over 90% in shade. A. philoxeroides biomass was unaffected by shade, contrasting with glasshouse results and resulting in increased dominance in shade. Connection to unshaded ramets in the glasshouse reduced some, but not all, of the differences between shaded and unshaded plants. Thus, resource sharing likely facilitated A. philoxeroides growth in the field, but may have been less influential than release from inter-specific competition. This research suggests shading may have limited use for controlling A. philoxeroides unless shade-tolerant inter-specific competition is provided.
Introduction
Increases in available, unused resources generally increase community invasibility (Davis et al. Citation2000). Increases in open habitats may thus facilitate invasion of exotic plant species, often adapted to high-light environments. By the late twentieth century, around 23% of New Zealand's land area was under forest cover, compared with around 85% before human colonisation (Taylor & Smith Citation1997). This has substantially increased light penetration to many herb and shrub communities. Median lighting at water level in native forest streams was 2.9%, compared with 42% for pasture streams (Davies-Colley & Quinn Citation1998). Imposing artificial shade has been found to be a useful tool in managing invasive weeds such as Tradescantia fluminensis Vell.: Commelinaceae (Standish Citation2002) and may be useful within farm ecosystems (for instance along riparian margins).
Plants exposed to low irradiance commonly exhibit a range of shade-avoidance and/or tolerance mechanisms, such as elongation of shoots, increased leaf area with low leaf mass per unit area, low chloroplast numbers and changes in chlorophyll A to chlorophyll B ratios. These changes enable plants to outgrow their neighbours and maximise light capture (Salter et al. Citation2003). Similarly, there may be a proportional increase in allocation of resources to above-ground parts of the plant, and a decrease in biomass overall (Maule et al. Citation1995; Weihe Citation1997).
Alternanthera philoxeroides (Mart.) Griseb: Amaranthaceae (alligator weed) is native to South America, and its introduced range includes northern New Zealand, Australia, and parts of Asia and Europe (Julien et al. Citation1995; Wagh et al. Citation1995; Garbari & Pedulla 2001). An amphibious, clonal herb, A. philoxeroides thrives in aquatic margins and moist terrestrial habitats. Physiological integration increases growth of apical ramets and facilitates expansion into new environments (Wang et al. Citation2009; Yu et al. Citation2009). In pasture ecosystems, A. philoxeroides steadily increases in biomass and displaces other species (Julien et al. Citation1992). Invasion in horticultural systems results in yield losses up to 45% (Shen et al. Citation2005). A. philoxeroides also impacts native ecosystems including altering invertebrate communities and patterns of decomposition (Bassett et al. Citation2010, Bassett et al. Citation2011).
A. philoxeroides shows limited translocation of herbicides (Bowmer & Eberbach, Citation1993) and current biocontrol is effective only in aquatic environments (Sainty et al. Citation1998). Cultural control methods, including manipulating shade and competition, may therefore be important in managing terrestrial A. philoxeroides.
Existing research is conflicting with respect to A. philoxeroides’ responses to shading. A. philoxeroides in the field has been reported as both shade tolerant (Longstreth et al. Citation1985; Timmins & Mackenzie Citation1995) and shade intolerant (Davis et al. Citation1983). Laboratory observations are similarly conflicting. Grown in a nutrient solution, fragment dry weight exhibited a negative linear relationship to light intensity, down to an average of 160 µmol m-2 s-1 (Longstreth & Mason, Citation1984). This model predicted dry weight would be zero at approximately 5 mol m-2 day-1, or an average 99 µmol m-2 s-1 over the 14 h photoperiod. In contrast, emergent nodes, also in a nutrient solution, produced similar root and shoot growth whether grown in light or dark (Quimby & Kay, Citation1977). Although not in a replicated experiment, Gangstad (Citation1978) reported continued growth after 1 year in complete darkness, suggesting a much higher degree of shade tolerance than that predicted by Longstreth & Mason (Citation1984). (Jiang et al. Citation2010) found potted A. philoxeroides increased the proportion of biomass allocated to above-ground tissues when shaded. However, morphological responses to shading were modified by varying nitrogen levels. (Jiang et al. Citation2010) suggested that A. philoxeroides’ success in a wide range of heterogeneous environments may be aided by its high phenotypic plasticity and an ability to use high levels of one resource to compensate for low levels of another resource.
In addition to providing somewhat conflicting descriptions of A. philoxeroides’ shade tolerance, existing research has not examined A. philoxeroides’ responses to shading in the presence of inter-specific competition or under field conditions. It is therefore not possible to predict changes in A. philoxeroides’ relative dominance within plant communities following imposition of shading. To address this issue, the authors carried out two experiments to test the hypothesis that growth of A. philoxeroides would be restricted by shading in both controlled glasshouse conditions and field conditions, but that competing pasture grass would be more restricted than A. philoxeroides. In light of results gained from the first two experiments, a third experiment was designed to test the additional hypothesis that A. philoxeroides growth would be less restricted by shading when connected to unshaded plant parts than when connected only to other shaded plant parts.
Methods
Experiment 1
A full factorial design included three shade treatments and three competition treatments. Shade treatments used shade cloth covers over individual pots (500 mm high, 350 mm diameter). The full sun treatment varied with cloud cover and time of day up to a maximum of 1550 µmol s-1 m-2, and being of the order of 250–500 µmol s-1 m-2 under cloudy conditions in the middle of the day (LI-250 light meters, LI-COR Biosciences, Lincoln, Nebraska, USA; quantum sensor measures photosynthetically active radiation). The photoperiod ranged from approximately 10 to 11 hours per day during the course of the experiment. Partial shade received 31%±2% of full sun and full shade received 7%±2% of full sun. The partial and full shade treatments were, respectively, similar to stream light levels in pasture and native or plantation forests in New Zealand (Maule et al. Citation1995; Davies-Colley & Quinn Citation1998).
As A. philoxeroides in pastures and adjoining riparian edges most commonly competes with introduced pasture grasses, a ryegrass (Lolium perenne L. (Poaceae))/ fine fescue (Festuca sp. (Poaceae)) turf was chosen for the competition treatments. The three competition treatments were no competition (bare ground), patchy competition and full competition. Patchy and full competition covered two diagonally opposite or all four quarters of the pot with turf grass respectively.
A. philoxeroides stems were collected from northwest Auckland (36°44.5′S, 174°26.7′E) and pre-rooted in water. Eight replicate pots (diameter 180 mm, height 150 mm) were filled with potting mix for each treatment. The potting mix comprised bark, pumice, peat moss and a slow-release fertiliser with N:P:K ratio of 5:5:6 plus trace elements. A single, randomly allocated A. philoxeroides node, with 0–5 mm stem growth and at least one leaf, was planted into the centre of each pot. At planting, roots were 5–25 mm long from node to furthest root tip. Pots were randomly blocked within the glasshouse. The experiment was conducted in late winter (August); temperatures ranged between a minimum of 12 °C and a maximum of 30 °C. Pots stood in 50 mm of water and were surface watered every 2 to 3 days as required, providing a damp terrestrial environment.
After 4 weeks, total shoot length was measured, this being the length of the primary stem, plus the length of all lateral stems from the point of branching to the base of the furthest node. Plants were then removed from the soil. Primary root length was measured from the basal node to the furthest root tip. Root and shoot dry mass and grass shoot dry mass were determined after oven drying for 24 h at 70 °C. Root parameters were measured only for A. philoxeroides plants grown without competition, as it was impossible to reliably disentangle A. philoxeroides and grass roots where the two were grown together.
Two-way analysis of variance (ANOVA) tested for effects of shade and inter-specific competition on shoot parameters, with plants nested in blocks for the error term. Root parameters from no-competition pots were analysed by one-way ANOVA for effect of shade, with plants nested in blocks for the error term. Tukey's post-hoc tests were used to determine pair-wise differences. Where necessary, data were log or square root transformed to overcome heteroscedasticity or non-normality.
Experiment 2
The field experiment was conducted in northwest Auckland, New Zealand (36°44.5′S, 174°26.7′E), using a random block design, with eight replicates per treatment. Treatments were no shade, part shade and full shade. The no-shade treatments were marked as permanent quadrats, 0.5 m by 0.4 m. Shade treatments used wooden frames, 0.5 m by 0.4 m internal area and 1 m tall, covered by shade cloth. Unshaded treatments were exposed to full daylight, varying with cloud cover and time of day up to a maximum of 2002 µmol s−1 m−2 (LI-250 light meters). Photoperiod decreased from approximately 14.5 h at the beginning of the experiment to 13 h at the end of the experiment. Mean light levels in shaded treatments were 44%±9% and 8%±4% of full sun in the partial shade and full shade treatments, respectively. A 100 mm gap was left uncovered at the bottom to minimise differences resulting from altered microclimate (Saitoh et al. Citation2002) and to minimise exclusion of potential herbivores. The experiment ran for 10 weeks over the summer (mid December to late February). The study site was fenced to exclude cattle, although there was an incursion at week nine. Temperature and relative humidity were measured at 30 minute intervals by Hobo H8 dataloggers (Onset Computer Corporation, Massachusetts). One datalogger from each of the unshaded and part -shade treatments was destroyed during the stock incursion. The neighbouring full-shade datalogger was also excluded from analyses, reducing the replication for temperature and humidity from four to three dataloggers per treatment.
Pre-experiment cover and biomass were measured in ten quadrats that were not subsequently used as treatment quadrats due to the extractive biomass sampling. All plants within quadrats were identified. Percentage cover of alligator weed and other species was estimated from 100 random points imposed on a digital photograph of each plot (Digital Sampling Method v1.00; Landcare Research, New Zealand). All above-ground vegetation was removed. This was sorted into A. philoxeroides and ‘other’ (being all other plant species present), and dry mass was determined after 24 h at 70 °C.
At the end of the experiment, cover and biomass were measured for all treatment plots as described above. In addition, the length of the fifth inter-node from the growing tip was measured for 10 randomly selected A. philoxeroides stems from each quadrat.
One-way ANOVA, with quadrats nested in blocks for the error term, tested for the effects of shade on cover and biomass. To avoid pseudo-replication (Hurlbert Citation1984), mean inter-node length was determined for each quadrat, then used in a one-way ANOVA, with quadrats nested in blocks for the error term. Environmental variables were analysed with one-way ANOVA. Data were log or square root transformed where needed to overcome heteroscedasticity or non-normality. Tukey's post-hoc tests were used to determine pair-wise differences.
Experiment 3
A. philoxeroides stolons were grown in troughs (450 mm long, 180 mm wide, 130 mm deep), with a node in each half of the trough connected below ground. Mean inter-node length between the two nodes was 100 mm±40 mm. Three shade treatments were imposed, with eight replicates each: fully shaded, where the entire trough (both nodes) was shaded; unshaded, where the entire trough was unshaded; and partially shaded, where one end of the trough (one node) was shaded and the other was not. Shade cloth frames were placed over half, or the entire, trough. The two shade treatments did not differ in shade intensity, with an overall mean of 12%±0.5% of full sunlight (t-test, p=0.081). The unshaded treatments received the same light environment as in experiment 1, again ranging up to a maximum 1550 µmol s-1 m-2, but with the photoperiod ranging between approximately 13.5–14.5 h per day over the course of the experiment.
Plant responses were measured at only one randomly selected pre-designated end of each trough (being the shaded end in the partially shaded treatment). Troughs were arranged in a random block design within the glasshouse, and were watered every 2–3 days as required for 6 weeks. Measurements included length of longest (primary) stem, total length of all stems combined, number of stems (i.e. degree of branching) and above-ground dry mass.
One-way ANOVAs tested for the effect of shade on shoot parameters, with individual plants nested in blocks for the error term. Tukey's post-hoc tests were used for pair-wise differences. Where necessary, data were log transformed to overcome heteroscedasticity. Analyses for all three experiments were performed in R v.2.2.1 (Ihaka & Gentleman Citation1996).
Results
Experiment 1
Primary and total stem length of A. philoxeroides were reduced by increasing shade (P<0.001 in both cases; ). There was a marginally significant interaction for total stem length, with the competition effect most apparent without shade. Without competition, light availability explained 88% of variation in A. philoxeroides total stem length (). If this relationship continued outside the range of conditions tested here, stem length would be reduced to zero at 0.24% of full sunlight. Without competition, primary root length also decreased with increasing shade (P<0.001; ), as did root dry mass (P<0.001; ). Root:shoot ratio was not significantly affected by shade (P=0.08; ).
Figure 1 Experiment 1: Relationship between A. philoxeroides total stem length and light intensity in the absence of inter-specific competition in the glasshouse.
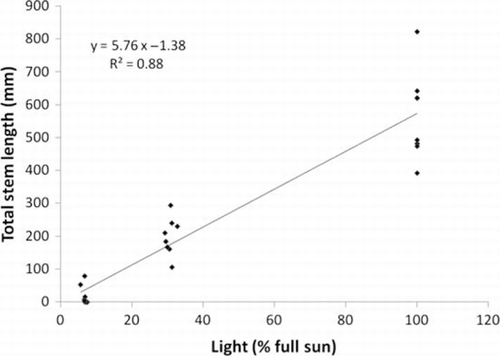
Table 1 Experiment 1: Shoot and root growth compared between shade treatments (mean±SEM). All measures are for A. philoxeroides unless otherwise stated. Within a row, *P<0.05, **P<0.01, ***P<0.001. Values with the same letter are not significant at P<0.05.
Shade and competition interacted to affect A. philoxeroides dry shoot mass (P<0.05). Increasing shade decreased shoot dry mass. No senescence of A. philoxeroides shoots was observed; rather, growth occurred more slowly in shade than in full sun. Grass dry shoot mass reduced with shade, down to 2.9% in full shade (P<0.001; ). Senescence of grass shoots continued throughout the experiment.
Experiment 2
Pre-treatment mean A. philoxeroides dry biomass was 0.09 (±0.01) kg/m2, or 20%±3% of total plant biomass. A. philoxeroides comprised 36%±4% of total plant cover pre-experiment. Grasses (Poaceae) comprised 35%±3% of cover. Remaining cover consisted of four herbaceous genera: Calystegia (Convolvulaceae); Ranunculus (Ranunculaceae); Rumex (Polygonaceae); and Trifolium (Fabaceae).
Post-treatment A. philoxeroides cover was greater under full shade than in no shade or part shade (P<0.001; ). Cover of other pasture species was substantially reduced in full shade compared with unshaded and partly shaded treatments (P<0.001; ). Grasses were particularly restricted by shading, with Ranunculus sp. the least affected of the ‘other’ plant species.
Table 2 Cover and above-ground biomass dynamics of A. philoxeroides and all ‘other’ co-occurring pasture plants under varying shade levels in the field, and environmental measures (mean±SEM). Within a row, *P<0.05, **P<0.01, ***P<0.001. Values with the same letter are not significant at P<0.05.
Mean A. philoxeroides dry mass did not differ between shade treatments post-experiment (P=0.54; ), but dry mass of other pasture species did differ, decreasing with increasing shade (P<0.001; ). Consequently, A. philoxeroides comprised a greater proportion of total biomass in the fully shaded treatment than in either unshaded or partly shaded treatments (P<0.001; ). A. philoxeroides inter-node length differed between all three shade treatments, increasing with increasing shade (P<0.001; ).
Mean temperature was higher in the shaded treatments than in the no shade treatments (P<0.01), but did not differ between part and full shade (). Neither maximum nor minimum temperature differed between shade treatments (P=0.08 and 0.15, respectively; ). Humidity was higher in shaded treatments than the unshaded treatment, but did not differ between part and full shade (P<0.001; ).
Experiment 3
Above-ground dry biomass differed between all treatments, with partially shaded plants being intermediate between those entirely with or without shade (P<0.001; ). Total stem length was greater in unshaded plants than in partially or fully shaded plants (P<0.01; ). Primary stem length did not differ between treatments (P=0.83; ). The mean number of stems per plant differed between all shade treatments, with least branching under full shade (P<0.001; ). Neither maximum nor minimum temperature varied between treatments (P=0.2 and 0.4, respectively; ).
Table 3 Experiment 3: A. philoxeroides above-ground growth (mean±SEM) under varying shade levels in glasshouse conditions. Partial shade plants received full shade, but were connected to unshaded parts of the plant. Within a row, *P<0.05, **P<0.01, ***P<0.001. Values with the same letter are not significant at P<0.05.
Discussion
As expected, shading in the glasshouse restricted A. philoxeroides growth, including length and mass of both above- and below-ground plant parts. A. philoxeroides continued to grow, albeit slowly, at light levels well below those predicted for zero growth by Longstreth & Mason (Citation1984). This supports a previous assessment of A. philoxeroides as a ‘shade–tolerant’ weed (Timmons & Mackenzie 1995). As such, light levels alone do not guarantee New Zealand forests protection from invasion by A. philoxeroides.
Data from the first experiment did not support the hypothesis that A. philoxeroides would be less heavily restricted than grass by shading. However, these data may be misleading in that A. philoxeroides showed no senescence in response to shade, whereas grass shoots showed wide-scale and continuing senescence. Had the experiment been continued for longer, the results for grass shoot mass would likely have more accurately reflected the senescence that was visually evident. Substantial senescence of grass occurred again in the field experiment, and this time was clearly evident in the experimental results. The net effect of shading in the field was to substantially increase the dominance of A. philoxeroides. Thus, there was overall support for our expectation that competing pasture species would be more heavily restricted by shading.
In the field, A. philoxeroides above-ground dry mass was the same across all shade treatments. This contrasted with the restricted A. philoxeroides growth observed under glasshouse conditions. A number of factors may have contributed to the conflicting glasshouse and field results, including resource sharing with unshaded plant parts, release from competition and changes in other environmental variables. Each of these will be discussed below.
In the glasshouse, connection to unshaded plant parts reduced (but did not entirely offset) the effect of shade on above-ground biomass and number of stems produced. However, connection to unshaded plant parts did not mitigate the effect of shade on total stem length. A. philoxeroides has previously been shown to be able to share resources to facilitate growth in suboptimal conditions (Yu et al. Citation2009). Connection with ramets in bare ground improved the growth and vegetative reproduction of A. philoxeroides exposed to inter-specific competition (Yu et al. Citation2009). There is thus some evidence from both the current study and previous research suggesting that resource sharing with unshaded plant parts could contribute to the shade tolerance displayed by A. philoxeroides in the field experiment. However, the somewhat limited benefits of resource sharing in shaded glasshouse conditions suggest that connection with unshaded plants does not entirely explain the field results.
Release from competition is also likely to have contributed to the unrestricted growth of A. philoxeroides under shade in the field. The full-shade treatment resulted in competing pasture biomass being reduced by over 90% compared to the unshaded treatment. This represents a substantial reduction in inter-specific competition. Competition with pasture grasses was shown to restrict A. philoxeroides growth in the first glasshouse experiment. Similarly, Yu et al. (Citation2009) reported reduced growth of A. philoxeroides with high-density inter-specific competition compared with low-density competition, and Bowmer et al. (Citation1989) noted slower post-herbicide re-growth in the presence of inter-specific competition. Thus, loss of competition almost certainly benefited A. philoxeroides growth in our field experiment. Although A. philoxeroides biomass did not differ between shade treatments in the field, inter-node length did differ, even between partial and full shade. Shoot elongation in response to shade enables plants to capture light at the expense of competing species (Salter et al. Citation2003). Morphological plasticity may thereby contribute to A. philoxeroides’ competitiveness under low light conditions.
Perry & Galatowitsch (Citation2004) showed that, despite similar responses to shade in monoculture, shading increased competitiveness of the invasive sedge Phalaris arundinacea L. (Poaceae) compared with the native sedge Carex hystericina Muhl. ex Willd. (Cyperaceae) when the two were grown together. Perry & Galatowitsch concluded that sowing a cover crop for shade in North American prairie wetland restorations was unlikely to improve establishment of C. hystericina in competition with P. arundinacea. Thus the effectiveness of shading for weed control depends not only on the shade tolerance of the target weed, but also on the relative tolerance and competitiveness of co-existing vegetation.
Differences in environmental conditions are a third factor potentially contributing to the unrestricted growth of A. philoxeroides under shade in the field. Mean temperature and humidity were both higher in shaded than unshaded treatments. Higher humidity is likely to have benefited A. philoxeroides given its preference for damp sites. Similarly, the warmer temperatures under shaded conditions were within the optimal growth range of 15–20 °C (Julien et al. Citation1995), whereas the unshaded treatment was slightly cooler. However, there were no differences in temperature or humidity between the two shaded treatments. If these environmental factors were substantially facilitating growth in the shaded treatments, we would have expected to see stronger growth in the part-shade treatment than the full shade treatment. This suggests that increased temperature and humidity do not explain the unrestricted growth of A. philoxeroides under shade in the field.
Artificial shading has been found to provide some control of the weed Tradescantia fluminensis (Commelinaceae) (Standish Citation2002). While shading does have the potential to reduce A. philoxeroides growth, the results from the current study suggest factors such as competitive release and resource sharing may limit its effectiveness as a control tool. In New Zealand, A. philoxeroides often grows in competition with highly light-demanding grass species. Loss of this inter-specific competition may offset some of the benefits of shading. Provision of shade-tolerant competing species may therefore be important in successful control through shading. Given that A. philoxeroides has a strong preference for damp ground, changes in soil moisture may also be critical in altering the competitive balance between A. philoxeroides and neighbouring plants exposed to shading. This could be explored through further experiments manipulating soil moisture in addition to light and competition. The apparent ability of A. philoxeroides to use resource sharing to mitigate low-light conditions may also limit the effectiveness of shading unless comprehensive coverage can be achieved.
Acknowledgements
The authors would like to acknowledge funding from the University of Auckland, Landcare Research and the Co-operative Research Center for Australian Weed Management. Comments from Peter Williams and Adrian Walcroft improved this manuscript.
References
- Bassett , IE , Beggs , JR and Paynter , Q . 2010 . Decomposition dynamics of invasive alligator weed compared with native sedges in a Northland lake . New Zealand Journal of Ecology , 34 : 324 – 331 .
- Bassett , I , Paynter , Q and Beggs , JR . 2011 . Invasive Alternanthera philoxeroides (alligator weed) associated with increased fungivore dominance in Coleoptera on decomposing leaf litter . Biological Invasions , 13 : 1377 – 1385 .
- Bowmer , KH and Eberbach , PL . 1993 . Uptake and translocation of 14C-glyphosate in Alternanthera philoxeroides (Mart.) Griseb. (alligator weed) II. Effect of plant size and photoperiod . Weed Research , 33 : 59 – 67 .
- Bowmer KH , McCorkelle G , Sale P , Eberbach P 1989 . Progress in the chemical control of alligator weed Proceedings of 5th Noxious Plant Conference, Northern Rivers College of Advanced Education . New South Wales Agriculture and Fisheries. Lismore, NSW Pp. 54 – 61 .
- Davies-Colley , RJ and Quinn , JM . 1998 . Stream lighting in five regions of North Island, New Zealand: control by channel size and riparian vegetation . New Zealand Journal of Marine and Freshwater Research , 32 : 591 – 605 .
- Davis GJ , Stanley DW , Brinson MM 1983 . Biomass dynamics of Alternanthera philoxeroides in a swamp forest stream and floodplain Proceedings of Symposium on Aquatic Macrophytes University of Nijmegen Nijimegen, , The Netherlands
- Davis , MA , Grime , JP and Thompson , K . 2000 . Fluctuating resources in plant communities: a general theory of invasibility . Journal of Ecology , 88 : 528 – 534 .
- Garbari , F and Pedulla , ML . 2001 . Alternanthera philoxeroides (Mart.) Griseb. (Amaranthaceae), a new species for the exotic flora of Italy . Webbia , 56 : 139 – 143 .
- Gangstad , EO . 1978 . Weed control methods for river basin management , Boca Raton, FL : CRC Press .
- Hurlbert , SH . 1984 . Pseudoreplication and the design of ecological field experiments . Ecological Monographs , 54 : 187 – 211 .
- Ihaka , R and Gentleman , R . 1996 . R: A language for data analysis and graphics . Journal of Computational and Graphical Statistics , 5 : 299 – 314 .
- Jiang , L-Z , Wang , D , Liu , S-N , Pan , R , Shen , F and Zhou , J . 2010 . Effect of light and nitrogen on morphological traits and biomass allocation of an invasive weed Alternanthera philoxeroides (Mart.) Griseb . Acta Hydrobiologica Sinica , 34 : 101 – 107 .
- Julien , MH , Bourne , AS and Low , VHK . 1992 . Growth of the weed Alternanthera philoxeroides (Martius) Grisebach, (alligator weed) in aquatic and terrestrial habitats in Australia . Plant Protection Quarterly , 7 : 102 – 108 .
- Julien , MH , Skarratt , B and Maywald , GF . 1995 . Potential geographical distribution of alligator weed and its biological control by Agasicles hygrophila . Journal of Aquatic Plant Management , 33 : 55 – 60 .
- Longstreth , DJ and Mason , CB . 1984 . The effect of light on growth and dry matter allocation patterns of Alternanthera philoxeroides . Botanical Gazette , 145 : 105 – 109 .
- Longstreth , DJ , Bolanos , JA and Goddard , RH . 1985 . Photosynthetic rate and mesophyll surface area in expanding leaves of Alternanthera philoxeroides grown at two light levels . American Journal of Botany , 72 : 14 – 19 .
- Maule , HG , Andrews , MA , Morton , JD , Jones , AV and Daly , GT . 1995 . Sun/shade acclimation and nitrogen nutrition of Tradescantia fluminensis, a problem weed in New Zealand native forest remnants . New Zealand Journal of Ecology , 19 : 35 – 46 .
- Perry , LG and Galatowitsch , SM . 2004 . The influence of light availability on competition between Phalaris arundinacea and a native wetland sedge . Plant Ecology , 170 : 73 – 81 .
- Quimby , PC and Kay , SH . 1977 . Hypoxic quiescence in alligator weed . Physiologia Plantarum , 40 : 163 – 168 .
- Sainty , G , McCorkelle , G and Julien , M . 1998 . Control and spread of alligator weed Alternanthera philoxeroides (Mart.) Griseb., in Australia: lessons for other regions . Wetlands Ecology and Management , 5 : 195 – 201 .
- Saitoh , T , Seiwa , K and Nishiwaki , A . 2002 . Importance of physiological integration of dwarf bamboo to persistence in forest understorey: a field experiment . Journal of Ecology , 90 : 78 – 85 .
- Salter , MG , Franklin , KA and Whitelam , GC . 2003 . Gating of the rapid shade-avoidance response by the circadian clock in plants . Nature , 426 : 680 – 683 .
- Shen , J , Shen , M , Wang , X and Lu , Y . 2005 . Effect of environmental factors on shoot emergence and vegetative growth of alligator weed (Alternanthera philoxeroides) . Weed Science , 53 : 471 – 478 .
- Standish , R J . 2002 . Experimenting with methods to control Tradescantia fluminensis, an invasive weed of native forest remnants in New Zealand . New Zealand Journal of Ecology , 26 : 161 – 170 .
- Taylor , RI and Smith , I . 1997 . The state of New Zealand's environment , Wellington : Ministry for the Environment .
- Timmins , SM and Mackenzie , IW . 1995 . Weeds in New Zealand protected natural areas database , Wellington : Department of Conservation .
- Wagh , GK , Ghate , HV and Ghate , VS . 1995 . First record of the alligator weed Alternanthera philoxeroides (Mart.) Griseb. from Pune, Maharashtra . Journal of the Bombay Natural History Society , 92 : 141 – 143 .
- Wang , N , Yu , F-H , Li , P-X , He , W-M , Liu , J , Yu , G-L , Song , Y-B and Dong , M . 2009 . Clonal integration supports the expansion from terrestrial to aquatic environments of the amphibious stoloniferous herb Alternanthera philoxeroides . Plant Biology , 11 : 483 – 489 .
- Weihe , PE . 1997 . The effects of shading on competition between purple loosestrife and broad-leaved cattail . Aquatic Botany , 59 : 127 – 138 .
- Yu , F-H , Wang , N , Alpert , P , He , W-M and Dong , M . 2009 . Physiological integration in an introduced invasive plant increases spread into experimental communities and modifies their structure . American Journal of Botany , 96 : 1983 – 1989 .