Abstract
Phosphorus (P) is central to the sustainability of New Zealand pastoral farming. The Winchmore long-term field experiments are the longest running grazed field trials of their type in the world. Treatments include variable inputs of both fertiliser (largely superphosphate, SSP) and irrigation. Insights from the trials include the following. After initially accumulating quickly as organic P, a steady state was reached whereby P preferentially accumulated as inorganic P in proportion to SSP applications rates. Pasture production paralleled accumulated P when SSP application stopped. Organic P decreased when lime was applied, but quickly recovered when more P was applied than necessary for plant production. P unaccounted for in a mass balance was lost in irrigation outwash. Losses related to stocking rate more than soil test P or SSP applications. The long-term field trials at Winchmore therefore represent a valuable resource to improve knowledge of agri-environmental P dynamics.
Introduction
Phosphorus (P) has been the most expensive element used in most farming operations for many decades (Zapata & Roy Citation2004). In an effort to maximise yield response to P, a number of long-term trials at Winchmore, Canterbury, New Zealand were initiated between 1949 and 1952. These examined different rates of P as annual applications (0, 188 and 376 kg ha−1) of superphosphate (SSP) or a single rate (205 kg ha−1) of reactive phosphate rock (RPR), under a constant flood irrigation regime (fertiliser trial), or a constant rate (250 kg ha−1 y−1) of SSP, but with varying rates (dryland, 10, 15 and 20% available soil moisture and three-weekly intervals) of flood irrigation (irrigation trial) to a sheep-grazed pasture. Full details of the treatments can be found in Rickard & Moss (Citation2012). As components of the fertiliser trial, the effect of lime was also assessed in sub-plots and, from 1958 to 1980, P application was stopped in two treatments to assess the effect of residual P on pasture production.
Internationally, long-term trials have revealed trends in the build-up, release and transformation of soil P that short-term trials do not. This is due to many factors, for example adaptation by plants or micro-flora, or physio-chemical processes such as sorption; precipitation; and soil physical structure such as pore space and the need for irrigation. For example, Blake et al. (Citation2000) found that at the Bad Lauchstaedt (began 1902) and Broadbalk (began 1843) long-term experiments in Germany and England, respectively, the most efficient uptake of P was in those plots with P applied as farmyard manure, whereas P uptake was better in plots with mineral P applied at Skierniewice in Poland (began 1923). The difference was ascribed to the interaction of soil physio-chemical properties (texture and organic C concentration) and P form on soil P sorption and losses. Other work in the USA has shown that 40 years of cattle feedlot manure enhanced the downward movement of P relative to an equivalent rate of P applied as triple SSP (Eghball et al. Citation1996). Sharpley et al. (Citation1993) went further to demonstrate that while 12–35 years of broiler litter application to ‘Coastal’ bermudagrass (Cynodon dactylon (L.) pers.) did decrease P sorption, examining this effect in the short-term (<5 years) may suggest that the potential for P loss (e.g. to water) may be small. However, long-term data showed that, with time, the decrease in P sorption causes the potential for P loss to increase greatly.
In contrast to the cropping trials mentioned above, the Winchmore trials are the longest running grazed pasture trial under irrigation in the world. However, while pasture production in response to P (and irrigation) was the main focus of the trials, and is covered by a companion paper in this issue (Smith et al. Citation2012), records and samples collected since their inception have also allowed researchers to investigate how production was linked to changes in soil P concentration, form and availability (e.g. Goh & Condron Citation1989). Furthermore, while unforeseen at establishment, the Winchmore trials have also enabled work to examine P in relation to two topics that have recently become of concern.
1. | The realisation that the efficiency of P use needs to improve, since P is a finite resource and is estimated by some (e.g. Vaccari Citation2009) to reach peak supply soon. | ||||
2. | A build-up of P in soil leads to loss and contamination of surface waters (McDowell et al. Citation2004). |
Phosphorus accounting
Many studies have used a mass balance approach to account for P transfers within different agricultural systems, defined here as the soil–plant–water continuum. This approach allows a researcher to account for the quantity and flux of P in different parts or pools within the system. The quantity of data, and the usually detailed recording associated with long-term trials, suit this technique. At Winchmore, mass balances of P have been conducted for the first 35 and 38 years of the 0, 188 and 376 kg SSP ha−1 y−1 treatments of the long-term fertiliser trial by Nguyen & Goh (Citation1992) and Williams & Haynes (Citation1992), respectively. Interestingly, while there were similarities between the two assessments, there were also discrepancies in the percentage of fertiliser P accounted for. Williams & Haynes (Citation1992) accounted for 63% and 65% of the fertiliser P applied in the 188 and 376 SSP treatments, while Nguyen & Goh (Citation1992) put the values at 94% and 83%, respectively (). The difference lies in the comparison of the 188 and 376 SSP plots against a wilderness (ungrazed) site in the study of Williams & Haynes (Citation1992), while Nguyen & Goh (Citation1992) used the changes that had occurred in the grazed control as a comparison (85 kg ha−1 over 35 years sourced from soil weathering via pasture development).
Table 1 Accounting for superphosphate (SSP) added to the long-term fertiliser trial via calculation of the annual (kg ha−1 y−1) and total change (kg ha−1) from 1952–1987 in the inputs, outputs and pools of P to 300 mm depth. Data calculated from Nguyen (Citation1990) and Nguyen & Goh (Citation1992).
A number of inferences can be made from a mass balance. Evident from the data in is the relatively large amount of P within the soil and plant pools (9.6–37.9 kg P ha−1 y−1), which contrasts the small quantity output in animal products (0.6–1.4 kg ha−1 y−1). One of the balance's assumptions is that 80% of P in pasture is utilised and removed by grazing sheep (Nguyen Citation1990). However, the vast majority of this P is returned to the ground in dung proportional to plant P concentrations (Rowarth et al. Citation1988). Hence, the only output accounted for occurs as wool and from the replacement of animals culled from the trial—itself a factor of stocking rate and soil fertility.
In accounting for SSP applied, more was accounted for in the 188 SSP treatment than the 376 SSP treatment (94.3% compared to 83.1% of that applied). Although more P is cycled within the soil–plant–animal system, the unaccounted (or lost) P totalled 1.0 kg P ha−1 y−1 in the 188 SSP treatment and 5.9 kg P ha−1 y−1 in the 376 SSP treatment. Both Williams & Haynes (Citation1992) and Nguyen & Goh (Citation1992) attributed part of the loss to the observation of stock camping on the borders/crutches of each irrigation bay. Using data collected from the wider Winchmore farm (i.e. not the long-term trials), Saville et al. (Citation1997) later measured, and predicted, that the border would have approximately twice the Olsen P concentration of the strip after 25 years of grazing and fertilisation at c. 200 SSP. While Nguyen & Goh (Citation1992) estimated the transfer of P during their mass balance, this did not account for all of the P lost. It was concluded that an unknown quantity of P was being lost as P in faeces washed out the end of each irrigation bay (i.e. as outwash) and P leaching. This will be covered in a later section.
While the increased loss of P from the fertiliser trial with increasing SSP application rate is one indicator of efficiency, it is also interesting to note that while annual changes in both plant P pools and soil P increase from the 0 to 188 SSP treatments, the same is not true when comparing the 188 and 376 SSP treatments (). For instance, compared to the control, while both plant shoot and soil P increases, plant root P decreases (7.9–7.6 kg P ha−1 y−1). This probably reflects fertilisation in the 376 SSP treatment to a point where P is no longer limiting pasture production. Olsen P concentration in the 376 SSP treatment (32±3 mg l−1; Fig. 1) had reached or exceeded the optimum concentration required for sheep-grazed pasture production, usually observed at between 20 and 30 mg l−1 (Morton & Roberts Citation1999). Combined with increased losses, the additional P appears to be housed in the soil P pool (increases from 11.8 to 21.4 kg P ha−1 y−1), rather than plant shoot P pool (increases from 5.8 to 8.9 kg P ha−1 y−1). However, while a mass balance may infer efficiency, it does not give any insight into the form of soil P, and whether or not this P is available to plants or can be lost via surface runoff (including outwash) or sub-surface flow (including leaching).
Phosphorus transformations within the soil
Regular assessments of plant available P have been made in both long-term trials since their onset. From 1952 (but more commonly from 1958) to 1981, this was assessed as Truog P; Olsen P was first measured in 1976 and continues seasonally in the fertiliser trial and annually in the irrigation trial to the present day. A relationship using 142 soil samples for the 5 years where Truog P and Olsen P were both measured enabled a regression to be established
Figure 1 Mean Olsen P concentration in the long-term fertiliser trial at each sampling date from 1958 to 2007. SSP=kg ha−1 of superphosphate applied each year in winter. SED is the standard error of the difference between means.
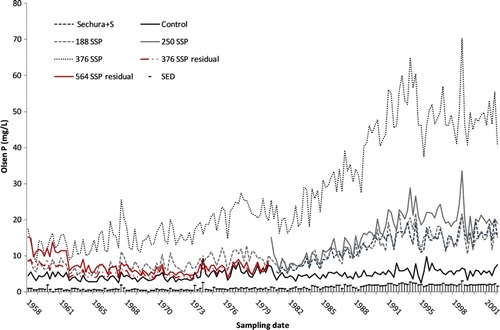
The residual treatments were included as part of a study by Condron (Citation1986) and Condron & Goh (Citation1989) examining the amounts and forms of P in the long-term fertiliser trial from 1952 to 1977. Using P fractionation, a technique that aims to extract increasingly recalcitrant pools of P from soils, they were able to show that the concentration of the readily plant available NaOH (OH−) inorganic P pool (and total inorganic P) was rapidly depleted following cessation of P to the residual treatments (). However, decreases in these pools were less from 1968 to 1977 (the end of their dataset). In those treatments that received SSP, inorganic P increased 0.7% and 8.8% annually from 1958 to 1971 in the 188 SSP and 376 SSP treatments, respectively. The greater accumulation in the 376 SSP treatment compared with the 188 SSP treatment was attributed to the application of P beyond plant requirements. This conclusion was supported by the accumulation of plant available P in and OH− extracts (). From 1972 to 1977, inorganic P increased annually by 4.6% and 7.5% in the 188 SSP and 376 SSP treatments, respectively. However,
and OH− forms either saw no change or increased little, whereas HCl-extractable P increased 2.6% and 6.7% annually in the respective treatments. This was commensurate with the application of 4 t ha−1 of lime across the trial and a shift in inorganic P from Al and Fe forms to Ca-associated forms (Sample et al. Citation1980).
Figure 2 Mean Olsen P concentration in the long-term irrigation trial at each sampling date from 1958 to 2007. SED is the standard error of the difference between means.
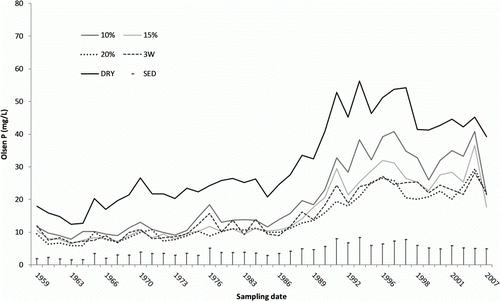
The application of lime also caused a significant decrease in the form and total quantity of organic P in the trial. Lime is well known to stimulate the mineralisation of organic matter and organic P (Halsted et al. Citation1963). Winchmore data (e.g. ) showed that the decrease was mainly in the OH−-extractable P. This questioned the hitherto held assumption that -extractable P was the more readily available than OH−-extractable P (Negassa & Leinweber Citation2009). However, the decrease could have been due to both the ample supply of P to plants via inorganic P, thereby not requiring
-extractable organic P to be mineralised, and the solubility of Ca- as opposed to Al- and Fe-inositol hexaphosphate (a major component of organic P and OH−-extractable P) (Harrison Citation1987). Additional evidence by Goh & Condron (Citation1989) showed that
-extractable P from the 188 SSP and 376 SSP treatments was plant available by a 34% decrease, compared to a 16% decrease in OH−-extractable P, following three repeated harvests of ryegrass.
Prior to the application of 4.4 t of lime in 1972, the data indicated that the accumulation of organic P was faster in the 376 SSP treatment than in the 188 SSP treatment, but both exhibited a decrease in the rate of accumulation and eventually a similar total organic P concentration. This was consistent with the pattern of recently developed pasture soils across much of New Zealand (Jackman Citation1964) and served to show that, beyond a certain total P concentration, additional P was accumulated as inorganic P. Additional data of P fractions from 1977 to 1994 (Eden Citation1995; Metherell et al. Citation1997) showed that while organic P mineralisation did occur due to the application of lime, the effect was short-lived and organic P concentrations were, in 1994, similar to those just prior to the application of lime (). These data also encompassed a change in the 376 and 564 residual treatments to the application of 250 kg SPP ha−1 to the same rate of P applied as Sechura RPR (205 kg ha−1). Increases in inorganic P in the RPR treatment were evident from an increase in undissolved RPR as HCl-extractable P. However, dissolution of RPR was sufficient to also increase plant available - and OH−-extractable P pools, which aligns with agronomic data (McBride Citation1992) to suggest that RPR is an effective maintenance P fertiliser. Data from Winchmore expanded the recommendation that RPR was suitable in environments where soil pH is less than 6.0 and rainfall less than 800 mm y−1 (Sinclair et al. Citation1994a, Citation1994b), to include irrigation as part of the annual 800 mm minimum.
In the long-term irrigation trial, mean Olsen P concentrations were greatest in the dryland treatment, followed by the treatments irrigated at 10%, 15% and 20% available soil moisture, or at three-weekly intervals (). Additional investigations into the P forms at multiple depths (0–7.5, 7.5–15 and 15–25 cm) were conducted on samples taken from the irrigation trial in 2001 by Condron et al. (Citation2006). Like Olsen P, concentrations of total and inorganic P were greater in soil taken from the dryland treatment (1301 mg total P kg−1 and 727 mg inorganic P kg−1 in the 0–7.5 cm soil layer) than irrigated treatments (1081–1249 mg total P kg−1 and 372–577 mg inorganic P kg−1 in the 0–7.5 cm soil layer). Much like the fertiliser trial, more P was accumulated as inorganic P than organic P.
Figure 3 Mean percentage of inorganic and organic P fractions and the total concentration of organic and inorganic P from 1958 to 1994. Data from Condron (Citation1986), Eden (Citation1995), Condron & Goh (Citation1989), McDowell & Condron (Citation2000) and Metherell et al. (Citation1997). In 1978, the 376 and 564 residual treatments changed to 250 SSP and 175 Sechura, respectively.
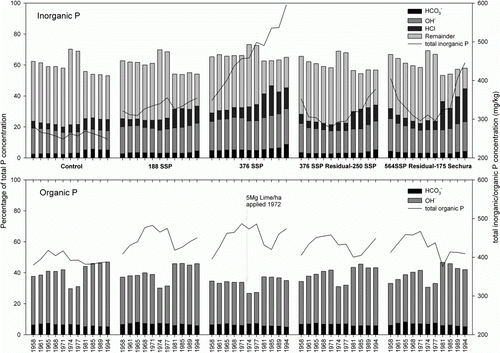
Further investigation using 31P nuclear magnetic resonance spectroscopy of NaOH-EDTA extracts of the 10%, 15% and 20% treatments indicated that while the proportion of P as orthophosphate increased, the decrease in organic P species was greater among orthophosphate monoesters (which includes IHP) than diesters (). The accumulation of monoesters is presumably due to greater pasture production and therefore monoesters, but also the strong sorption of IHP that enable it to accumulate in the soil. Since all treatments received 250 kg of SPP annually, the accumulation of P in the dryland treatment was attributed to a combination of decreased pasture growth and P loss in produce and outwash. In contrast, a combination of increased pasture production and soil biological activity was attributed to greater organic P concentration in the irrigated treatments (e.g. 672–709 mg kg−1 in the 0–7.5 cm soil layer) compared with the dryland treatment (574 mg kg−1 in the 0–7.5 cm soil layer). Additional measurements of 33P isotopic exchange kinetics indicated that the accumulation of inorganic P was proportionately greater as recalcitrant P (e.g. P exchangeable in more than 3 months, E >3m) in the dryland treatment compared with the irrigated treatments. This was attributed to physiochemical reactions such as solid-state diffusion, which decreased the availability of inorganic P over time (Frossard et al. Citation1995). Interestingly, the data also indicated that some readily available and also leachable pools (e.g. that exchangeable in less than 1 min; McDowell et al. Citation2001) had moved to at least 15 cm depth in the dryland and irrigated treatments compared to an unfertilised ‘wilderness’ control. However, the enrichment of recalcitrant pools like E >3m to depth did not occur. Clearly, the accumulation of P and P forms were central to P mobility in this trial.
Figure 4 31P nuclear magnetic resonance spectra of P forms in the NaOH-EDTA extracts of 2007 soils from the 10%, 15% and 20% treatments of the long-term irrigation trial (R.W. McDowell, unpublished data).
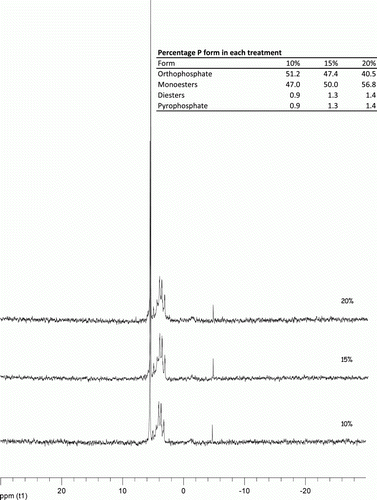
In a review of P fractions in temperate soils Negassa & Leinweber (Citation2009) concluded that applying large amounts of P in the form of manure or soluble (e.g. SSP) fertiliser increased labile P fractions, regardless of the period of application. However, they also concluded from analysis of data such as those from Winchmore that continuous application of low doses of fertiliser P (11–32 kg P ha−1y−1) increased almost all P fractions. They were able to produce a predictive equation
Phosphorus losses to water
While P fractionation has been used to isolate P forms according to plant availability, studies of Winchmore soils have calibrated the technique to also assess the potential for P loss to water. In the absence of direct field measurements, work by Hesketh & Brookes (Citation2000) and McDowell & Sharpley (Citation2001) established that a dilute 0.01 M CaCl2 extraction of topsoil (0–7.5 cm) was able to estimate the P lost in drainage water from tile drains and lysimeters, respectively. Using sequential fractionation, McDowell & Condron (Citation2000) examined the fertiliser experiment's soils and found that pools associated with Al– and Fe–P were the most closely correlated to 0.01 M CaCl2-extractable P. The selectivity of a sequential fractionation scheme towards Al–, Fe– and Ca–P pools was confirmed by the examination of four soils during the fractionation process with non-invasive solid state 31P NMR (McDowell et al. Citation2003a) (). Further work by McDowell & Condron (Citation2001) added weight to the hypothesis that sorption/desorption processes in the Winchmore soils were controlled by Al and Fe oxides. By extracting soil with dithionite-citrate to remove Al and Fe oxides, P sorption significantly decreased, whereas other treatments focusing on organic matter or removal of Ca had less effect.
Table 2 Total P concentration and 31P solid state nuclear magnetic resonance data for the Al–, Fe– and Ca–P concentration in soils from the control and 250 SSP treatments of the long-term fertiliser that had been either unextracted or extracted with NaOH and NaOH followed by HCl (designed to remove Al– and Fe–P [NaOH] and Ca–P [HCl], respectively). Data from McDowell et al. (Citation2003a).
Having established the likely mechanism behind P release from soil, additional work has examined how the potential for loss varies with soil P concentration. Like the rest of New Zealand, the soil P test used as an agronomic indicator of pasture production is Olsen P (Olsen et al. Citation1954). The Winchmore trials represent one of the few places in New Zealand where we can compare the relationship between Olsen P and pasture production or the potential for P loss. Figure shows the traditional plateau in pasture production with increasing Olsen P, but an increase in the potential for P loss. Although P loss and pasture production varies with soil type (McDowell & Condron Citation2004), work has indicated that, except for the least P-sorptive soils, the point of excessive potential P loss can be near but usually below the agronomic optimum. This reinforces the recommendation that Olsen P concentration should not exceed the agronomic optimum as this will yield little or no added economic gain, but likely represents an environmental problem via increased potential for P loss.
Figure 5 Relationship between pasture production or the potential for P loss in subsurface drainage (as estimated by 0.01 M CaCl2–P) and Olsen P in the fertiliser trial. The arrow indicates the mean Olsen P at which 95% of maximum pasture production is achieved on sedimentary soils (Morton & Roberts Citation1999) and also the concentration near which the likelihood of subsurface P losses will increase. Data from 1952 to 1986 as summarised by Rickard & McBride (Citation1987) and for the 1990 sampling by McDowell & Condron (Citation2000).
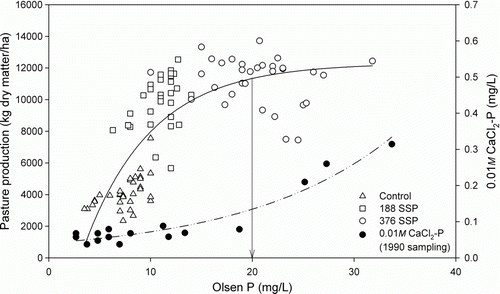
Maintaining an Olsen P at an agronomic optimum will minimise, but not eliminate, environmentally significant concentrations of P loss from soil. In order to decrease the potential for loss further, other sources of P loss need to be mitigated. The application of water-soluble fertiliser has been highlighted as a potential cause of P loss. The exact quantity of P lost will depend on two factors: 1) the water solubility of applied P, which determines how much P initially enters, and to some extent is maintained in, the soil solution, and; 2) because the enrichment of soil solution P declines with time as P is sorbed onto the surface of soil aggregates, the time between application and outwash.
Work at Winchmore was integral in determining both factors. Using soils that had not previously received P as RPR (virgin soils), Nguyen et al. (Citation1999) found that 100–200 times more dissolved reactive P (DRP) was lost in surface runoff (generated at 50 mm h−1 from repacked soils supplied with P as SPP) than similar plots that had received P as RPR. Nyugen & Quinn (Citation2001). Nguyen et al. (Citation2002) then took samples from the 175 RPR and 250 SSP treatments (with the same rate of P application, 23 kg ha−1) and repeated the experiment. Again, following a fresh fertiliser application, a similar picture to that in the virgin soils emerged. However, because soils in both sets of experiments were repacked and rained upon at a high intensity, measured losses of P, especially in particulate form were much greater (more than tenfold) than normally expected from grassland under natural conditions (Preedy et al. Citation2001). Using intact turves and a rainfall rate more representative of the area (15 mm h−1), McDowell et al. (Citation2003b) confirmed that P losses in runoff from plots with SSP applied were enriched in P, especially DRP (e.g. DRP concentration 1 day after application was c. 5.3 mg l−1 and total P=6.5 mg l−1), and declined exponentially with time. However, losses were still greater 60 days after application than lost before application occurred. In contrast, those plots with RPR applied exhibited no obvious trend after application. This and comparable data from Australia (e.g. Nash et al. Citation2004) led to the recommendation that SSP should not be applied less than 21 days in New Zealand and 7 days in Australia before a scheduled flood irrigation. Following this recommendation, annual losses of RPR- and SSP-treated soils, at the same rate of P applied, should be similar.
Although examination of rainfall simulation data can give valuable information on the potential for P losses, only quantification of outwash can give a true assessment of P loss under flood irrigation. Interestingly, outwash samples collected from RPR- and SSP-treated plots showed that the mean DRP concentration was greater in the plots with RPR applied (). This agrees with some work that suggests that RPR applied to soils may maintain a slightly greater soil solution P concentration than a soil with the same rate of SSP applied due to a slight liming effect (Chien et al. Citation1987). In 2002, the 175 RPR treatment was at a mean pH of 5.8 while the 250 SSP treatment was at a mean pH of 5.6 (unpublished data). However, modelling and field data from rain-fed (and probably sprinkler-irrigated) systems within the pH range considered optimal for pasture production indicate that the enhanced short-term potential for P loss from SSP is greater than a long-term ‘drip-fed’ supply of P via RPR and the soil solution. Due to the long time frame in which SSP-applied soils were enriched compared to those with RPR applied, it is likely that this would also be the case for flood-irrigated systems. However, this needs to be confirmed and is an obvious hypothesis to test at Winchmore.
Table 3 Mean Olsen P, dissolved reactive P and total P concentrations in outwash from the irrigation and fertiliser trials (Data from McDowell and Rowley, Citation2008). SED is the standard error of the difference between treatment means; *, ** and *** represent significance at the P<0.05, 0.01 and 0.001 level, respectively; ns means not statistically significant.
Although the Winchmore trials have shown that the application of P can increase P loss either through the enrichment of Olsen P or fertiliser P in outwash, the irrigation trial has also shed insight into the role of stocking rate. The data in (adapted from McDowell & Rowley Citation2008) show that for the fertiliser trial, the concentration of DRP and total P in outwash mirrored fertiliser P inputs and Olsen P, but at the same time stocking rate increased to utilise the extra feed grown. In contrast, the greatest losses of DRP and total P in outwash occurred in the 20% and three-weekly treatments of the irrigation trial despite the same fertiliser P and a lower Olsen P concentration than the 10% and 15% treatments. Here, like the fertiliser trial, stocking rate was matched to pasture production, but yield was much greater in the more frequently irrigated treatments. Clearly, under irrigation, stocking rate overrode the effect of soil P and fertiliser P inputs in determining P in outwash. This is probably due to a combination of more faeces (and P therein) and losses from grazed pasture plants.
Conclusions
The long-term trials at the Winchmore irrigation research station have proven to be an invaluable resource. In addition to meeting the original aims of establishing the factors important in maximising pasture production under sheep grazing in response to P applications and/or irrigation, there have been many other benefits. These have included a large soil archive, dataset and detailed records on which to model and/or study changes in soil P, especially organic P fractions, in relation to productivity. More recently, unintended consequences have arisen such as the potential environmental impact of P losses to water, which, when combined with production data, enable advice to be based on an environmental and agronomic footing. Furthermore, the Winchmore trials are ideally placed to also answer questions beginning to be asked on the limited availability of water or P or changes in climate. Without the maintenance of long-term trials such as Winchmore we lose a tool from which we are able to assess, predict and respond to these emerging or associated historical issues (e.g. cadmium applied with long-term P fertilisers).
Acknowledgements
The author gratefully acknowledges the support of the New Zealand Fertiliser Manufacturers' Research Association in preparing this manuscript.
References
- Blake , L , Mercik , S , Koerschens , M , Moskal , S , Poulton , PR , Goulding , KWT , Weigel , A and Powlson , DS . 2000 . Phosphorus content in soil, uptake by plants and balance in three long-term field experiments . Nutrient Cycling in Agroecosystems , 56 : 263 – 275 .
- Chien , SH , Hammond , LL and Leon , LA . 1987 . Long term reactions of phosphate rocks with an oxisol in Colombia . Soil Science , 144 : 257 – 265 .
- Condron , LM . 1986 . Chemical nature and plant availability of phosphorus present in soils under long-term fertilized irrigated pastures in Canterbury, New Zealand , New Zealand : PhD thesis, Lincoln College, University of Canterbury .
- Condron , LM and Goh , KH . 1989 . Effects of long-term phosphatic fertilizer applications on amounts and forms of phosphorus in soils under irrigated pasture in New Zealand . Journal of Soil Science , 40 : 383 – 395 .
- Condron , LM , Sinaj , S , McDowell , RW , Dudler-Guela , J , Scott , JT and Metherell , AK . 2006 . Influence of long-term irrigation on the distribution and availability of soil phosphorus under permanent pasture . Australian Journal of Soil Research , 44 : 127 – 133 .
- Eden , R . 1995 . Effect of fertiliser phosphorus on soil phosphorus fractions in a long-term grazed pasture trial , 1994 – 95 . Lincoln , , New Zealand : AgResearch, Summer scholarship project .
- Eghball , B , Binford , GD and Baltensperger , DD . 1996 . Phosphorus movement and adsorption in a soil receiving long-term manure and fertilizer application . Journal of Environmental Quality , 25 : 1339 – 1343 .
- Frossard , E , Brossard , M , Hedley , MJ and Metherell , A . 1995 . “ Reactions controlling the cycling of P in soil ” . In Phosphorus in the global environment , Edited by: Tiessen , H . 107 – 137 . New York , , USA : Wiley .
- Gillingham , AG , Richardson , S , Power , IL and Riley , J . 1989 . Long term effects of withholding phosphate application on North Island hill country: Whatawhata Research Centre . Proceedings of the New Zealand Grassland Association , 50 : 11 – 16 .
- Goh , KM and Condron , LM . 1989 . Plant availability of phosphorus accumulated from long-term applications of superphosphate and effluent to irrigated pasture . New Zealand Journal of Agricultural Research , 32 : 45 – 51 .
- Halsted , RL , Lapensee , JM and Ivarson , KC . 1963 . Mineralization of soil organic phosphorus with particular reference to the effect of lime . Canadian Journal of Soil Science , 43 : 97 – 106 .
- Harrison , AF . 1987 . Soil organic phosphorus: a review of world literature , Wallingford , , UK : CAB International .
- Hesketh , N and Brookes , PC . 2000 . Development of an indicator for risk of phosphorus leaching . Journal of Environmental Quality , 29 : 105 – 110 .
- Jackman , RH . 1964 . Accumulation of organic matter in some New Zealand soils under permanent pastures. I. Patterns of change in organic carbon, nitrogen, sulphur, and phosphorus . New Zealand Journal of Agricultural Research , 7 : 445 – 471 .
- Lambert , MG , Clark , DA and Mackay , AD . 1989 . Long term effects of withholding phosphate application on North Island hill country: Ballantrae . Proceedings of the New Zealand Grassland Association , 50 : 25 – 28 .
- McBride , SD . 1992 . Agronomic performance of RPR fertiliser in a grazed pasture . Proceedings of the New Zealand Grassland Association , 54 : 41 – 44 .
- McBride , SD , Nguyen , ML and Rickard , DS . 1990 . Implications of ceasing annual superphosphate topdressing applications on pasture production . Proceedings of the New Zealand Grassland Association , 52 : 177 – 180 .
- McDowell , RW and Condron , LM . 2000 . Chemical nature and potential mobility of phosphorus in fertilized grassland soils . Nutrient Cycling in Agroecosystems , 57 : 225 – 233 .
- McDowell , RW and Condron , LM . 2001 . Influence of soil constituents on soil phosphorus sorption and desorption . Communications in Soil Science and Plant Analysis , 32 : 2531 – 2547 .
- McDowell , RW and Condron , LM . 2004 . Estimating phosphorus loss from New Zealand grassland soils . New Zealand Journal of Agricultural Research , 47 : 137 – 145 .
- McDowell , RW and Rowley , D . 2008 . The fate of phosphorus under contrasting border-check irrigation regimes . Australian Journal of Soil Research , 46 : 309 – 314 .
- McDowell , RW and Sharpley , AN . 2001 . Approximating phosphorus release from soils to surface runoff and subsurface drainage . Journal of Environmental Quality , 30 : 508 – 520 .
- McDowell , RW , Sinaj , S , Sharpley , A and Frossard , E . 2001 . The use of isotopic exchange kinetics to assess phosphorus availability in overland flow and subsurface drainage waters . Soil Science , 166 : 365 – 373 .
- McDowell , RW , Condron , LM and Mahieu , N . 2003a . Analysis of phosphorus in sequentially extracted grassland soils using solid state NMR . Communications in Soil Science and Plant Analysis , 34 : 1623 – 1636 .
- McDowell , RW , Monaghan , RM and Carey , PL . 2003b . Phosphorus losses in overland flow from pastoral soils receiving long-term applications of either superphosphate or reactive phosphate rock . New Zealand Journal of Agricultural Research , 46 : 329 – 337 .
- McDowell , RW , Biggs , BJF , Sharpley , AN and Nguyen , L . 2004 . Connecting phosphorus loss from land to surface water quality . Chemistry and Ecology , 20 : 1 – 40 .
- Metherell A , Condron LM , Eden RM 1997 . Effects of fertiliser rates and forms on soil phosphorus fractions in a long term trial at Winchmore, New Zealand . 18th International Grasslands Congress, Session 10, Soil Fertility Winnipeg , Manitoba , Canada .
- Morton , JD and Roberts , AHC . 1999 . Fertiliser use on New Zealand sheep and beef farms , Auckland : New Zealand Fertiliser Manufacturer's Research Association .
- Nash , D , Hannah , M , Halliwell , D , Webb , B and Chapman , D . 2004 . A field study of phosphorus mobilisation from commercial fertilisers . Australian Journal of Soil Research , 42 : 313 – 320 .
- Negassa , W and Leinweber , P . 2009 . How does the Hedley sequential phosphorus fractionation reflect impacts of land use and management on soil phosphorus: a review . Journal of Plant Nutrition and Soil Science , 172 : 305 – 325 .
- Nguyen , ML . 1990 . Sulphur cycling in soil–plant–animal systems under grazed, irrigated pastures in Canterbury, New Zealand and its implication on pasture sulphur requirements , Canterbury , , New Zealand : Unpublished PhD thesis, Lincoln University .
- Nguyen , ML and Goh , KM . 1992 . Nutrient cycling and losses based on a mass-balance model in grazed pastures receiving long-term superphosphate applications in New Zealand . Journal of Agricultural Science , 119 : 89 – 106 .
- Nguyen L , Quinn B 2001 . Potential phosphorus losses in runoff from New Zealand pastoral soils receiving superphosphate and reactive phosphate rock applications . In: Haygarth PM et al. Proceedings of the Workshop Connecting Phosphorus Transfer from Agriculture to Impacts in Surface Waters , Plymouth University , UK 28 August – 1 September .
- Nguyen ML , Cooper AB , Thorrold BS 1999 . Phosphorus losses in surface runoff from field plots receiving soluble and slow-release phosphatic fertilisers . Hamilton, New Zealand, National Institute of Water and Atmospheric Research Ltd. NIWA client report SQF90201 .
- Nguyen L , Quinn B , Sukias J 2002 . Potential losses of phosphorus and nitrogen in runoff and drainage from pastoral soils applied with superphosphate and reactive phosphate rock . In: Currie LD Loganathan P Proceedings of Dairy Farm Management Workshop, Massey University, Palmerston North, New Zealand, 13–14 February. Massey University, Palmerston North, New Zealand, Fertilizer and Lime Research Centre. Occasional report no. 15. Pp. 137 – 153 .
- O'Connor , MB , Shannon , PW and Gillingham , AG . 1985 . The effects of withholding fertiliser on pastoral production on hill country. Proceedings of the Technical Conference of the New Zealand Fertiliser Manufacturer's . Research Association , 20 : 260 – 273 .
- Olsen SR , Cole CV , Watanabe FS , Dean LA 1954 . Estimation of available phosphorus in soils by extraction with sodium bicarbonate . Washington, DC , , USA : United States Government Print Office . United States Department of Agriculture circular no. 939 .
- Preedy , N , McTiernan , K , Mathews , R , Heathwaite , L and Haygarth , P . 2001 . Rapid incidental phosphorus transfers from grassland . Journal of Environmental Quality , 30 : 2105 – 2122 .
- Rickard DS , McBride SD 1987 . Long term application and residual effects of superphosphate and effect of reactive phosphate rock on irrigated pasture . Ashburton, New Zealand, Winchmore Irrigation Research Station, Ministry of Agriculture and Fisheries, Technical report no. 22 .
- Rickard , DS and Moss , RA . 2012 . Winchmore and the long-term trials: the early history . New Zealand Journal of Agricultural Research , 55 : 93 – 103 .
- Rowarth , JS , Gillingham , AG , Tillman , RW and Syers , JK . 1988 . Effects of season and fertiliser rate on phosphorus concentrations in pastures and sheep faeces in hill country . New Zealand Journal of Agricultural Research , 31 : 187 – 193 .
- Sample , EC , Soper , RJ and Racz , GJ . 1980 . “ Reactions of phosphate fertilizers in soils ” . In The role of phosphorus in agriculture , Edited by: Khasawneh , FE . 263 – 310 . Madison, WI , , USA : American Society of Agronomy .
- Saville , DJ , Moss , RA , Bray , AR and Hannagan , RB . 1997 . Soil nutrient distribution in pastures flood-irrigated by the border strip method . New Zealand Journal of Agricultural Research , 40 : 99 – 110 .
- Sharpley , AN , Smith , SJ and Bain , WR . 1993 . Nitrogen and phosphorus fate from long-term poultry litter applications to Oklahoma soils . Soil Science Society of America Journal , 57 : 1131 – 1137 .
- Sinclair , AG , Johnston , PD , Smith , LC , O'Connor , MB and Nguyen , L . 1994a . Agronomy, modelling and economics of reactive phosphate rocks as slow release fertiliser for grasslands . Fertilizer Research , 36 : 229 – 238 .
- Sinclair , AG , Johnstone , PD , Smith , LC , Risk , WH , Morton , JD , O'Connor , MB , Roberts , AHC , Nguyen , L and Shannon , PW . 1994b . Patterns of, and a model for, dry matter yield response in grass/clover pastures to annual applications of triple superphosphate fertiliser . New Zealand Journal of Agricultural Research , 37 : 239 – 253 .
- Smith , LC , Moss , RA , Morton , JD , Metherell , AK and Fraser , TJ . 2012 . Pasture production from a long-term fertiliser trial under irrigation . New Zealand Journal of Agricultural Research , 55 : 105 – 117 .
- Vaccari , DA . 2009 . Phosphorus: a looming crisis . Scientific American , 300 : 54 – 59 .
- Williams , PH and Haynes , RJ . 1992 . Balance sheet of phosphorus, sulphur and potassium in a long-term grazed pasture supplied with superphosphate . Fertiliser Research , 31 : 51 – 60 .
- Zapata , F and Roy , RN . 2004 . Use of phosphate rocks for sustainable agriculture , Rome , , Italy : Food and Agriculture Organization of the United Nations .