Abstract
Subtle changes in soil pore size and shape as a consequence of changes in land use and management practices can have significant impacts on the movement of air, water, plant roots and soil biota. This paper describes a resin impregnation technique adapted for use in New Zealand pasture soils to quantify these soil pore attributes. Image analysis of the resin impregnated soil enables detailed assessment of soil pore shape and macro pore size distribution (>50 µm).
Introduction
Soil pores are an integral component of the soil matrix, influencing soil processes such as gas exchange and water retention, as well as impacting the habitat of plant roots and soil biota (Greenwood & McKenzie Citation2001). Soil compaction, a result of physical loading on the soil by grazing livestock for example, can decrease pore size and function and limit plant growth (Greenwood & McKenzie Citation2001; Sparling et al. Citation2004; Houlbrooke et al. Citation2011). Measures of bulk density and macroporosity give an indication of changes in soil porosity, but may not provide information on more subtle changes in pore size and shape that can be gained through resin impregnation (Cattle & Southorn Citation2010). There are also non-destructive computed axial tomography (CAT) scanning techniques emerging that provide high-quality data to assess soil pore size and function (Capowiez et al. Citation1998). However, this method is expensive and unavailable in New Zealand.
The impregnation of soil with resin and subsequent image analysis enables the quantification of changes in soil pore size and shape. This technique has been used overseas (Moran et al. Citation1989; McBratney & Moran Citation1990), successfully identifying subtle changes in pore size distribution under altered animal grazing regimes (McKenzie Citation2001; Cattle & Southorn Citation2010). Altered soil physical conditions as a result of agricultural practices have also been linked to changes in soil biological communities (Elliott et al. Citation1980).This paper describes a resin impregnation technique adapted for use under New Zealand pastoral conditions, to identify changes in soil macro pore size and shape.
Methods
Quantification of soil pore shape and size distribution using the modified resin impregnated technique was developed and assessed on a range of soil types and land management practices ().
Table 1 Comparison of bulk density measurements to those collected by image analysis (macro pores, pore size and pore shape). Pore shape values closer to 1 represent circular pores, and values closer to 0 represent linear pores.
Intact soil cores were collected to a depth of 20 cm in a 15 cm-diameter PVC pipe, leaving 5 cm of headspace to apply resin. To ensure minimal soil disturbance, a lever press apparatus was used to collect cores (A). When topography prevented use of this apparatus, a handheld fencepost rammer was used (B). Samples were collected when soil moisture was below and near field capacity for each soil type to ensure penetration and curing of the resin. Before coring, herbage was trimmed to within 2 cm of the soil surface.
Figure 1 From the field to the image. A, Collecting a soil core using a lever apparatus. B, Collecting a soil core using a hand-held fence post rammer. C, Core before the application of resin. D, Applying the resin. E, Cutting the core with the drop-saw. F, Inside the dark box with core on an adjustable stand with camera and lights mounted on top. G, Raw image of pores taken by camera (colours inverted). H, The same image as converted to a 3-bit image for analysis in Solicon©.
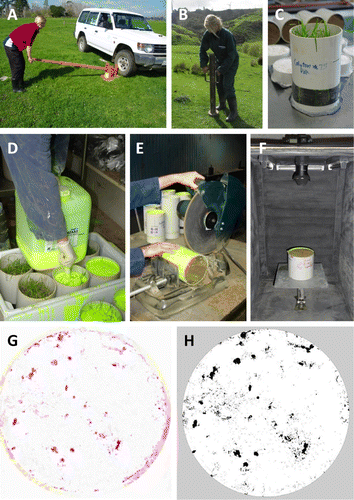
In the laboratory, loose material at the soil surface was removed by suction (using a low-power vacuum cleaner) to unblock pore openings that may have been obstructed. The cores were then placed upside down on a workbench and the soil at the bottom of the cores trimmed flush with the PVC tube using a large knife. Again, any loose material was removed by suction. Cores were then left in a well-ventilated room at ambient temperature (c. 24 °C) for 24 h.
A capped, 5 cm length of 15 cm-diameter PVC pipe, filled with vermiculite, was bonded to the base of each core using silicon sealant (Selleys 100% silicon). Foam rubber tape and a 5 cm section of car tyre inner tube were fitted around the outside of the bond to ensure the resilience of the seal (C). Cores were then left at ambient temperature for 48 h to allow the silicon sealant to set. Cores were moved to a chiller (4 °C) and a low viscosity, slow-curing epoxy resin mixture () containing ultraviolet-fluorescent dye plus an opacifying agent was poured on to the surface of each core to a depth of 5 cm. Resin was applied as required until infiltration into the soil ceased (D). The cores were then left at 4 °C for 24 h to ensure optimum permeation of the resin. At this stage, the resin still had a very low viscosity and the cores were moved to ambient temperature where they were left standing for a minimum of 48 h for the resin to set. The low viscosity of the resin (similar to water) ensured adequate penetration into the air-filled pores.
Table 2 Epoxy resin mixture, not miscible with water, used for infiltration into soil cores. The previously chilled components were thoroughly mixed at room temperature by vigorously shaking in a closed container. The resin mixture was applied to soil cores immediately after mixing.
Once the resin had set, cores were cut with a drop-saw (E) at the desired depths below the soil surface (2.5, 5, 8 and 12 cm). Before photographing, the surface of the sections were lightly washed with tap water and dried with a paper towel to remove any smearing effects. Each section was placed on a small, height-adjustable platform in a dark-box with a camera mounted on top (F). The height of the platform was adjusted to ensure that the distance between the photographed soil surface and the camera lens was kept constant. The soil sections were illuminated under white light (8 W Fluro tube mounted inside the box) to check if the lens focus required manual adjustment. With the white light turned off, the soil sections were then illuminated under ultraviolet light (using two 8 W Fluro Blacklight tubes, also mounted inside the box), and a time duration photograph taken using a Pentax digital camera (K20D, DSLR with SIGMA 50 mm DG-Macro lens). The initial photographs (G) were captured in Adobe DNG RAW image format and later converted to a three-colour binary image using Adobe Photoshop CS2®. Each pixel represented a 48 µm diameter patch on the original core; consequently, analysed pores were considered to be greater than >50 µm. Black pixels represent soil pores >50 µm (macro pores) and white pixels represent soil particles and pores <50 µm; grey pixels at the outer part of the image were not analysed (H). The outer 5 mm of the core image was removed to reduce any edge effects.
The images were analysed using Solicon© analysis software (The University of Sydney, Cotton Research and Development Corporation). Images were described in terms of macro pores (%), pore area (mm2/cm2) and pore shape. Pore area was estimated using limbs of a 16-sided polygon, where pores that could accommodate a particular 16-sided polygon were estimated using the area produced by the shortest limb. This analysis enabled data collection on the desired pore sizes. Our interest, and the focus of discussion, is on the relationship between soil pore size and biology. For example, we analysed the images with the knowledge that typical earthworm burrows are 2.5 to 5 mm diameter (Lee Citation1959; Springett Citation1983).
Results and discussion
Modifications to resin impregnation techniques used overseas were required for New Zealand soils. The first challenge was to produce a slow-curing resin mixture with a viscosity close to water. This mixture was produced in collaboration with Adhesive Technologies NZ Ltd. The second challenge was to overcome the issue of the resin not curing when soil moisture levels were high (>40% v/v). This issue was addressed by Moran et al. (Citation1989) by altering the resin mixture applied to a range of Australian soils. The resin mixture produced for New Zealand conditions had a low viscosity to ensure infiltration into the smallest pores, as well as being able to set when there was some moisture present. To ensure curing of the resin mixture, cores were collected when soil moisture was below and near field capacity and left in a well-ventilated room for 24 h before resin application, allowing for some moisture loss while preventing shrinkage and cracking (Murphy Citation1982).
The method of resin impregnation is more expensive and labour intensive than some traditional methods of measuring soil structure, but offers the advantage of more detailed assessment of soil pore size and shape. While our approach may have prevented the impregnation of some small air-filled pores, it is a useful method for assessing macro pore size and shape. If the interest extends to pores <50 µm, the application of vacuum is recommended to ensure all air-filled pores are impregnated with resin. While McKenzie (Citation2001) observed Solicon parameters to have a moderate correlation with direct measures of soil strength and aeration, further calibration is required between the different methods of assessing soil porosity. Further, traditional methods used to assess soil physical properties (bulk density, shear strength, unsaturated hydraulic conductivity) were not influenced by altered grazing regimes (Cattle & Southorn Citation2010) or increasing nitrogen fertilizer application (Douglas et al. Citation1992), while treatment differences were observed using image analysis of resin impregnated soils.
Across all our sites examined for soil pore size and shape, there was little correlation with bulk density, however, there was a positive correlation between earthworm abundance and pores that were 4–5 mm in diameter (r = 0.359). There was a positive correlation between total carbon content and proportion of pores <2 mm diameter (r = 0.502 for pores <1 mm diameter and 0.303 for pores <2 mm diameter).
The difference in soil structure is clearly evident when comparing soil under a permanent fence line, where animals could not tread, to a grazed paddock (Colyton Pallic soil, , ). Here, bulk density increases (0.73 compared with 1.10 Mg/m3) with a simultaneous decrease in pores of all sizes. The changes in soil pore size influence the function of the soil, including the soil biological community. Oribatid mite abundance (body widths <0.35 mm diameter) decreased from 22,400 ind./m2 under the fence to 1900 ind./m2 in the paddock. Earthworms that create burrows (pores) 2.5–5 mm diameter (Lee Citation1959; Springett Citation1983) were less abundant under the fence than in the paddock (358 ind./m2 vs. 662 ind./m2), where the decline in pores <2 mm in diameter was most apparent (Schon Citation2012).
Figure 2 Macro pores (v/v) as observed using resin embedding techniques in soil from: A, a permanent fence-line; B, a dairy-grazed paddock; and C, A mown and irrigated trial area on a Pallic soil (Schon Citation2012). Macro pores (black) represent 11%, 3% and 27% of the section, respectively. White represents soil particles and pores <50 µm.
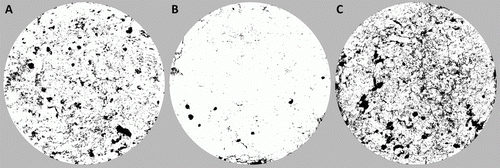
Resin impregnation is a useful method for improving our understanding of soil pore size and shape, and the implications of these changes on the soil system.
Acknowledgements
We thank S. Cattle for guidance with the use of Solicon© software. The project was funded by the FRST contract CO2XO405 and an AGMARDT Doctoral Scholarship.
Additional information
Notes on contributors
NL Schon
Current address: AgResearch Lincoln, Private Bag 4749, Christchurch 8140, New ZealandReferences
- Capowiez , Y , Pierret , A , Daniel , O , Monestiez , P and Kretzschmar , A . 1998 . 3D skeleton reconstructions of natural earthworm burrow systems using CAT scan images of soil cores . Biology and Fertility of Soils , 27 : 51 – 59 .
- Cattle , SR and Southorn , NJ . 2010 . Macroporosity of pasture topsoils after three years of set-stocked and rotational grazing by sheep . Australian Journal of Soil Research , 48 : 43 – 57 .
- Douglas , JT , Koppi , AJ and Moran , CJ . 1992 . Alteration of the structural attributes of a compact clay loam soil by growth of a perennial grass crop . Plant and Soil , 139 : 195 – 202 .
- Elliott , ET , Anderson , RV , Coleman , DC and Cole , CV . 1980 . Habitable pore space and microbial trophic interactions . Oikos , 35 : 327 – 335 .
- Greenwood , KL and McKenzie , BM . 2001 . Grazing effects on soil physical properties and the consequences for pastures: a review . Australian Journal of Experimental Agriculture , 41 : 1231 – 1250 .
- Houlbrooke , DJ , Paton , RJ , Littlejohn , RP and Morton , JD . 2011 . Land-use intensification in New Zealand: effects on soil properties and pasture production . Journal of Agricultural Science , 149 : 337 – 349 .
- Lee KE 1959 . The earthworm fauna of New Zealand . Wellington , New Zealand Department of Scientific and Industrial Research Bulletin 130 . 486 .
- McBratney , AB and Moran , CJ . 1990 . A rapid method of analysis for macropore structure: 2 Stereological model, statistical analysis, and interpretation . Soil Science Society of America Journal , 54 : 509 – 515 .
- McKenzie , DC . 2001 . Rapid assessment of soil compaction damage II. Relationships between the SOILpak score, strength and aeration measurements, clod shrinkage parameters, and image analysis data on a Vertisol . Australian Journal of Soil Research , 39 : 127 – 141 .
- Moran , CJ , McBratney , AB and Koppi , AJ . 1989 . A rapid method for analysis of soil macropore structure .1. Specimen preparation and digital binary image production . Soil Science Society of America Journal , 53 : 921 – 928 .
- Murphy , CP . 1982 . A comparative study of three methods of water removal prior to resin impregnation of two soils . Journal of Soil Science , 33 : 719 – 735 .
- Schon , NL . 2012 . Vulnerability of soil invertebrate communities to stock treading in three grassland soils . Applied Soil Ecology , 53 : 98 – 107 .
- Schon , NL , Mackay , AD , Gray , RA and Minor , MA . 2011a . Influence of phosphorus inputs and sheep treading pressures on soil macrofauna and mesofauna in hill pastures on an Andosol and a Luvisol . New Zealand Journal of Agricultural Research , 54 : 83 – 96 .
- Schon , NL , Mackay , AD and Minor , MA . 2011b . Soil fauna in sheep-grazed hill pastures under organic and conventional livestock management and in an adjacent ungrazed pasture . Pedobiologia , 54 : 161 – 168 .
- Schon , NL , Mackay , AD and Minor , MA . 2011c . Effects of dairy cow treading pressures and food resources on invertebrates in two contrasting and co-occurring soils . Soil Research , 49 : 703 – 714 .
- Schon , NL , Mackay , AD , Minor , MA , Yeates , GW and Hedley , MJ . 2008 . Soil fauna in grazed New Zealand hill country pastures at two management intensities . Applied Soil Ecology , 40 : 218 – 228 .
- Schon , NL , Mackay , AD , Yeates , GW and Minor , M . 2010 . Separating the effects of defoliation and dairy cow treading pressure on the abundance and diversity of soil invertebrates in pastures . Applied Soil Ecology , 46 : 209 – 221 .
- Sparling , GP , Schipper , LA , Bettjeman , W and Hill , R . 2004 . Soil quality monitoring in New Zealand: practical lessons from a 6-year trial . Agriculture, Ecosystems & Environment , 104 : 523 – 534 .
- Springett , JA . 1983 . Effect of five species of earthworm on some soil properties . Journal of Applied Ecology , 20 : 865 – 872 .