Abstract
Research in the 1980s showed that Ranunculus acris had evolved resistance to the phenoxy herbicides MCPA and MCPB in New Zealand. Since then two ALS-inhibitor herbicides, flumetsulam and thifensulfuron-methyl, have been used but recently flumetsulam has been reported as being less effective. The mortality responses of seedling progeny of 15 R. acris populations, differing in historical exposure to phenoxy and ALS-inhibitor herbicides, were compared using a log-series of five doses of MCPA, flumetsulam and thifensulfuron-methyl. The resulting mortalities were higher than expected at lower doses, meaning LD50 values were not reliably estimated. In a second experiment, the responses of one population with no previous exposure to herbicides and one population with high exposure to flumetsulam were compared using a wider range of doses of flumetsulam (0.04 to 25 times). The LD50 values differed 5.3-fold between the resistant and susceptible biotypes. In a third experiment, four populations varying in past exposure to both herbicide groups were treated with either flumetsulam or MCPA. Resulting LD50 values were higher in populations with high previous exposure to both groups. These results confirm that resistance to both phenoxy and ALS herbicides can occur in R. acris populations through repeated use. Since these are the only two available groups for the selective control of this weed in dairy pastures in New Zealand, the options for control are reduced.
Introduction
Ranunculus acris L. subsp. acris (giant or tall buttercup) is a perennial herb that has naturalised in New Zealand and become a serious pasture weed in six of the country's 17 dairy farming regions (Tasman district, South Auckland/Waikato, Hawke's Bay, Taranaki, Wairarapa and Horowhenua) (Tuckett Citation1961; Bourdôt et al. Citation2003). Utilisation of infested pastures by dairy cattle can be reduced by up to 50% at the peak of R. acris cover in November (Bourdôt et al. Citation2003) resulting in large losses in dairy farmer revenue (Bourdôt & Saville Citation2010).
The control of R. acris in dairy pastures has been problematic for farmers since the late 1970s. The phenoxy herbicides MCPA and MCPB that were used extensively up until that time previously gave adequate control (Tuckett Citation1961) but had become virtually ineffective in some areas due to evolved resistance (Bourdôt & Hurrell Citation1988; Bourdôt et al. Citation1990). Two newer herbicides, flumetsulam and thifensulfuron-methyl, both based on a different mode of action (acetolactate synthase [ALS]-inhibitors), have been available since about 1992. These four chemicals are the only compounds registered for selective control of R. acris in dairy pastures in New Zealand. Recently, farmers have been reporting that the effectiveness of flumetsulam, the more widely used of the two ALS-inhibitor herbicides, has been declining, particularly after repeated use over several years. This is a scenario similar to that leading to the discovery of phenoxy resistance in R. acris (Bourdôt et al. Citation1990), Carduus pycnocephalus L. (slender winged thistle) and C. nutans L. (nodding thistle) (Harrington et al. Citation1988; Harrington Citation1989) in New Zealand and in many other cases of herbicide resistance both in New Zealand and overseas (Bourdôt & Suckling Citation1996).
Since ALS-inhibitor herbicides became available in the early 1980s, the number of weed biotypes resistant to them has increased rapidly. There are now more cases of resistance to ALS-inhibitors than any other mode-of-action group, and these make up about a third of all known cases of resistance worldwide (Heap Citation2014). However, there are few previous examples of weeds that have evolved resistance to ALS-inhibitors in permanent pastures, although Crepis tectorum L. (narrow-leaf hawksbeard) has developed resistance to metsulfuron-methyl in grass seed crops in Canada (Heap Citation2014). Also, Echium plantagineum (Paterson's curse) and Sonchus asper (prickly sow-thistle) have been reported as being resistant to ALS-inhibitor herbicides in pastures sown after cereal crops treated with these herbicides in South Australia and Canada, respectively (Heap Citation2014). The high frequency of cases of resistance to ALS-inhibitor herbicides is partly due to the single site of action and the large number of possible point mutations that can confer resistance to them (Tranel & Wright Citation2002). Another reason is the high usage of these products and hence high selection pressure in many pastoral and cropping systems.
Bourdôt et al. (Citation1994) found that MCPA-resistant R. acris plants also showed resistance to 2,4-D and MCPB, but that glyphosate and the ALS-inhibitors tribenuron-methyl, chlorsulfuron and thifensulfuron-methyl still gave a high level of mortality. However, in that study the MCPA-resistant plants that were treated with chlorsulfuron and thifensulfuron-methyl exhibited slower apical mortality. This suggested a difference in tolerance to ALS-inhibitor herbicides in MCPA-resistant R. acris plants even though never having been exposed to them.
These observations led us to hypothesise that the low efficacy of flumetsulam on R. acris populations in some dairy farms recently may be due to an evolving resistance to this herbicide. The objective of this study was to determine if resistance to flumetsulam has evolved in R. acris by undertaking dose-response experiments on populations with differing past exposure to herbicides. Secondary aims were to retest MCPA to determine if resistance was still evident and also to test for possible cross-resistance with thifensulfuron-methyl.
Materials and methods
Experiment 1: Screening of 15 populations for resistance to flumetsulam, thifensulfuron-methyl and MCPA
Fourteen populations of R. acris from dairy farms in Golden Bay, New Zealand that differed widely in frequency to past exposure to flumetsulam, varying from no past flumetsulam use to annual treatments since the product became available, were selected (). Each farm's history of herbicide use was ranked according to the number of times flumetsulam had been applied in the 12 years prior to seed collection.
Table 1 The 15 populations of R. acris used in Experiment 1 and their historical exposure (number of treatments) to flumetsulam, thifensulfuron-methyl and MCPA (or MCPB) over 12 years (1997–2009).
Seeds were collected in January 2010 from these farms (Tasman district, 40.858°S, 172.791°E), plus from one roadside population (P1) at Leithfield, North Canterbury, New Zealand (43.182°S, 172.700°E) that had never been treated with any herbicides (B. Yates, Environment Canterbury, pers. comm.).
On 29 March 2010, seeds from each of the 15 populations were soaked overnight in 0.02% solution of KNO3, dried on paper towels and dusted with a 50:50 w/w mix of captan (Captan 80W, 800 g kg−1, DuPont NZ) and benomyl (Benlate®, 500 g kg−1, Nufarm NZ) wettable-powder fungicides. Seeds were sown on 30 March into trays of damp vermiculite and placed into a growth cabinet (Contherm Scientific) set to 20 °C light and 10 °C dark, on a 12 h cycle. The cotyledonary seedlings were pricked out (two seedlings per pot) 21 days after sowing (DAS) into 90 mm square pots (0.5 L) filled with bark/sand potting mix supplemented with agricultural and dolomite lime and Osmocote® (Scotts Australia Pty) slow release fertiliser. Two weeks prior to spraying (129 DAS), all plants were moved from the unheated glasshouse to a nearby shade house.
The experimental design was a split plot, with the 15 R. acris populations as main plots and five rates each of flumetsulam, thifensulfuron-methyl and MCPA plus one control as subplots (). The main plots were arranged in six randomised replicate blocks. However, four of the populations yielded too few seedlings, so these populations were present in only four of the blocks, resulting in 164 seedlings (82 pots) per treatment and 2624 seedlings (1312 pots) in total.
Table 2 Dose rates of flumetsulam, thifensulfuron-methyl and MCPA applied in Experiment 1.
The herbicide dose rate treatments were a logarithmic series (multiplier of √5 = 2.236) of five doses of flumetsulam (Preside™, 800 g a.i. kg−1), the dimethyl amine (DMA) salt of MCPA (Maestro 750 g a.i. L−1) (both Dow AgroSciences [NZ]) and thifensulfuron-methyl (Harmony, Du Pont NZ, 500 g a.i. kg−1) plus an untreated control, making 16 treatments in total (). The rates chosen for the experiment were in the range 0–260 g a.i. ha−1 for flumetsulam, 0–50 g a.i. ha−1 for thifensulfuron-methyl and 0–6.25 kg a.i. ha−1 for MCPA. The recommended field rates that existed when the experiment was conducted were 52 g a.i. ha−1, 15 g a.i. ha−1 and 1.125–2.25 kg a.i. ha−1, for flumetsulam, thifensulfuron-methyl and MCPA (Maestro), respectively (Young Citation2008). A mineral oil adjuvant (Uptake™ spraying oil, Dow AgroSciences [NZ]) was added to the flumetsulam treatments at a rate of 5 mL L−1 of spray mix.
The treatments were applied 143 DAS (20 August), when the plants were growing vegetatively (BBCH-weed growth stage 14–16, using the extended scale for weeds as described by Hess et al. [Citation1997]) each having four to six fully expanded leaves and long petioles. A conveyer-belt sprayer was used to deliver a spray volume equivalent to 200 L ha−1 at 210 kPa pressure through a single 8002EVS Teejet® flat-fan hydraulic nozzle (Spraying Systems Co). The untreated control plants were sprayed with a water-only treatment. After spraying, the plants were placed back in the shade house in their randomised layout.
All plants were assessed for mortality at 102 days after treatment (DAT) (30 November) using the same criteria described by Bourdôt et al. (Citation1990). A plant was considered dead if there was no green plant material and any remaining dead material pulled away from the plant crown easily.
Experiment 2: Comparison of responses of resistant and susceptible populations to flumetsulam
Due to unexpectedly high mortality in Experiment 1 it was considered necessary to re-examine the populations that appeared the most susceptible (P1) and resistant (P15). On 23 December 2010, R. acris seeds of the two populations were prepared for germination and sown the next day, as in Experiment 1. These seeds had been stored in airtight containers at 4 °C since collection in January 2010. The seedlings were pricked out 17 DAS (one seedling per pot) into 0.5 L square pots, as in Experiment 1.
All pots were randomly allocated in a split plot design with the two populations as main plots, and 10 flumetsulam dose rate treatments as subplots. There were seven blocks in total, each consisting of three replicate pots per treatment for P15 (210 plants total) but only two replicate pots per treatment for P1 (140 plants total). The unequal replication was necessary due to fewer available seedlings for P1. The plants were grown in the same shade house as in Experiment 1.
The treatments were a logarithmic series of nine dose rates of flumetsulam, the same as in Experiment 1 () but this time with two additional dose rates above and below the median rate (2.1, 4.7, 581 and 1300 g a.i. ha−1, respectively), plus an untreated control. These rates represent 0.04 to 25 times the median rate. The spraying oil adjuvant was added to each flumetsulam treatment, as before.
The plants were sprayed 111 DAS (14 April) in the same way as in Experiment 1, when they were growing vegetatively with four to six leaves and short petioles (BBCH-weed growth stage 14–16). After spraying, the plants were placed back in the shade house in their blocks, where they remained for 2 months. In mid-June, the plants were shifted to an unheated glasshouse for the remainder of the experiment to prevent damage from frosts. Mortality was assessed 127 DAT (19 August) using the same method as in Experiment 1.
Experiment 3: Comparison of responses of four populations to flumetsulam and MCPA
On 12 September 2012, seeds from four populations (P2, P6, P13 and P15) were prepared as for Experiment 1 and sown into trays the next day. On 10 October (27 DAS) individual seedlings were planted into 0.5 L square pots as before and 94 DAS (16 December) the R. acris plants (BBCH-weed growth stage 14–16) were sprayed with one of five treatments of the DMA salt of MCPA, the same as in Experiment 1 (). For flumetsulam, the middle seven rates from Experiment 2 were used, from 4.7 to 581 g a.i. ha−1 (representing 0.09 to 11.2 times its median rate), plus an untreated control. There were eight replicate blocks (except for P2 flumetsulam treatments which had seven) arranged in a split plot design with populations as the main plots and dose rates as the subplots (52 pots per block).
Mortality was determined by the method described, on 4 February 2013 (50 DAT) for MCPA treatments, and on 14 April 2013 (119 DAT) for flumetsulam treatments. The differences in the intervals from spraying until assessment between experiments were due to varying rates of herbicide activity and plant decay related to seasonal influences.
Analyses
Data from all experiments were analysed using a generalised linear model with a probit transformation of the binomial data (Genstat v13, VSN International, Version 13). Log10 LD50 mortality values (g or kg a.i. ha−1 dose required to kill 50% of population) were calculated from the fitted curves for each population and the parameter estimates (constant and slope) of each probit curve were used to plot the curves. Back-transformed LD50 values are used in tables. For Experiment 1, the fitting of the probit curves was not appropriate for some populations because most of the values were at maximum mortality (100%). Instead of this, for each dose rate the percentage mortality was compared between populations using a two-tailed Fisher's exact test. For Experiment 3, the calculated LD50 values for flumetsulam were used in correlations with the number of past exposures to this herbicide. For MCPA, no data existed on the actual number of past exposures due to its longer history of use, instead a ranking of previous exposure was used (0 = nil, 1 = moderate, 2 = high).
Results
Experiment 1: Screening 15 populations
For clarity, the results from five populations (P1, P2, P9, P14 and P15), which represent the range of past exposures to flumetsulam and MCPA herbicides (two nil, one intermediate exposure and two high, respectively), along with the averages of all 15 populations tested, are presented ().
Table 3 Percentage mortality for five populations of giant buttercup after treatment with each of five rates of flumetsulam in Experiment 1.
Fitting of probit curves to the data was generally unsuccessful as the LD50 values calculated were mostly outside the range of rates chosen in the experiment due to unexpectedly high mortalities. Despite this, there was some evidence that the R. acris populations varied in their responses to flumetsulam (). Population P15 had the lowest mortality of all the populations for the four highest rates (23.3, 52, 116 and 260 g a.i. ha−1) and this difference was significantly different from P1, P2 and P9 (P < 0.05) at 116 g a.i. ha−1, and P1 and P2 at 23.2 g a.i. ha−1 (P < 0.1). Population P15 had the highest number of past exposures to flumetsulam (12), while P1 and P2 were never exposed ().
Thifensulfuron-methyl was the least effective of the three herbicides compared here, with the overall lowest percentage mortality across populations at each rate (). Population P15 had significantly lower mortality (P < 0.05) than P1, P2 and P14. None of the five populations had ever been previously exposed to this herbicide ().
Table 4 Percentage mortality for five populations of giant buttercup after treatment with each of five rates of thifensulfuron-methyl in Experiment 1.
As with the two ALS herbicides, the populations also varied in their responses to treatment with MCPA (). At the two highest rates, this chemical was the most effective herbicide of the three tested and had almost no plants surviving at or above the recommended rate. Population P15 had significantly lower mortality (P < 0.05) than P1 and P2 at the lowest rate, while P15, P9 and P14 had significantly lower mortality (P < 0.05) than P1 and P2 at the 0.56 kg a.i. ha−1 rate. Populations P1 and P2 have had no previous exposure to MCPA while the other three populations had moderate to high previous exposure ().
Table 5 Percentage mortality for five populations of giant buttercup after treatment with each of five rates of MCPA in Experiment 1.
Experiment 2: Comparison of responses of two contrasting populations to flumetsulam
In this experiment the probit curves were good fits to the data and the estimated LD50 values for flumetsulam were in the middle of the chosen range of rates. The log10 LD50 values for populations P15 and P1 were 2.35 (95% confidence interval [CI]: 2.20–2.51) and 1.62 (95% CI: 1.54–1.70), respectively () and the back-transformed LD50 values were 222 g a.i. ha−1 (95% CI: 160–326) and 42 g a.i. ha−1 (95% CI: 34–51), respectively. This result indicates a 5.3-fold difference in the LD50 values between these two populations.
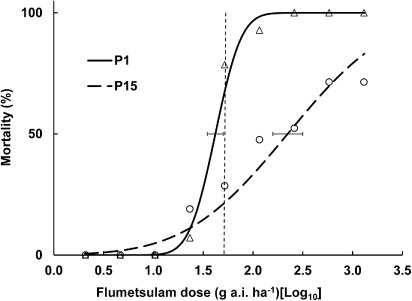
Experiment 3: Comparison of responses of four populations of R. acris to flumetsulam and MCPA
The fitted curves and treatment means for the four populations that were treated with flumetsulam are given in . The calculated LD50 value for P15 was significantly higher than those of populations P2 and P6 but not P13. Also P13 was significantly higher than P6, but not higher than P2.
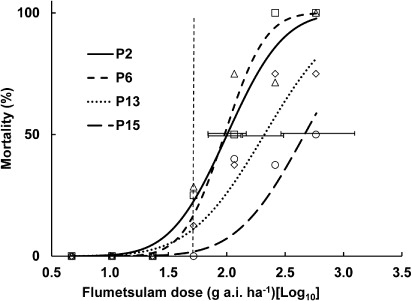
The fitted curves and treatment means for the same four populations that were treated with MCPA are given in . Population P15 had a significantly higher LD50 than populations P2, P13 and P6, and the latter two populations did not differ significantly from each other.
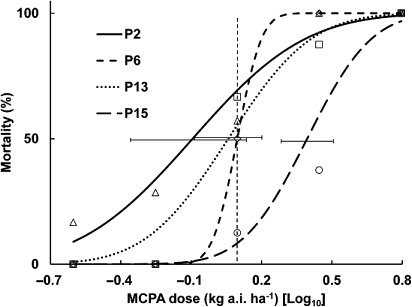
The back-transformed LD50 values for the four populations varied 4.8-fold from lowest to highest for flumetsulam (P6 cf. P15, respectively), while for MCPA the LD50 values varied by 3.1-fold (P2 cf. P15, respectively) ().
Table 6 LD50 values for four populations (P2, P6, P13 and P15) of R. acris and the corresponding number of past exposures to flumetsulam, and the ranking of past exposure to MCPA (0 = nil; 1 = moderate; 2 = high) in Experiment 3.
The log10 LD50 values for flumetsulam were correlated with the number of past exposures to that herbicide (10% significant; P = 0.074; correlation coefficient [r] = 0.93) and the log10 LD50 MCPA values were correlated with the ranking of exposure (5% significant; P = 0.020; r = 0.98) ( and , respectively).
Discussion
In Experiment 1 there were too few data points with mortality less than or equal to 50% and as a result, estimating LD50 values from the fitted probit curves was not appropriate since it could only be done for some of the populations. Instead, the averages of mortality were calculated for all the populations in order to compare them. From this there was clear evidence of significant differences in the tolerance of populations to flumetsulam, and to thifensulfuron-methyl and MCPA.
The higher than expected mortality in Experiment 1 was probably because the plants had been stressed by cooler temperatures and frost after coming out of the glasshouse in the 2 weeks prior to the spray treatments being applied and, as a consequence, may have been particularly susceptible. Another possible reason for the spring application in Experiment 1 giving higher levels of mortality than the autumn and summer applications in Experiments 2 and 3 could be the timing of application. This was previously shown by Harris & Husband (Citation1997) who found spring applications of flumetsulam to be more effective on field populations of R. acris than applications in either summer or autumn.
In order to better estimate the LD50 values, the experiment was repeated with only flumetsulam, this time with an expanded range of rates and with more replicates, using two of the populations of R. acris from Experiment 1 that differed most in their past exposure (P1 and P15). In contrast to the earlier result, the probit curves fitted well to the mortality data in Experiment 2 () and the resulting LD50 values fell in the middle of the range of rates chosen, providing better estimates than in the first experiment. Also, in Experiment 2 the mortality values at the recommended application rate for P15 and P1 were lower than in Experiment 1, averaging 54% and 92% for Experiments 2 and 1, respectively. Results from the two experiments indicated that R. acris seedling progeny from populations with high past exposure to flumetsulam had a greater tolerance of this herbicide. Experiment 2 results showed a 5.3-fold difference in the LD50 values (42 and 222 g a.i. ha−1, respectively) between R. acris progeny from a roadside population (P1) with no previous exposure, and the dairy-farm population with the highest past exposure (P15) to flumetsulam. This supports the hypothesis that genetically based resistance to flumetsulam has evolved in population P15, due to the selection pressure of repeated treatments. Experiment 3 confirmed the existence of evolved resistance to flumetsulam, as shown by its correlation with past exposure. Although population P1 was not included in this experiment, population P2 was equivalent, both having never been previously exposed to herbicides.
Population P15 was consistently the most resistant to each of the herbicides in all three experiments. This population had had the highest previous exposure to both flumetsulam and MCPA of all those tested, but it had never been treated with thifensulfuron-methyl, according to the farmer. In this study it was shown that P15 had multiple resistances to both flumetsulam and MCPA ( and ; Experiment 3) and may also be cross-resistant to thifensulfuron-methyl (; Experiment 1) because it had significantly higher survival than other populations at the highest rate, despite never being exposed to it.
In this study, less than 50% of the P15 plants were killed at 25 times the recommended rate of flumetsulam (, Experiment 2). Based on the fitted curves the resistant P15 would require about 3200 g a.i. ha−1 flumetsulam for 90% kill while the susceptible P1 would require only 80 g a.i. ha−1. Furthermore, the recommended application rate (52 g a.i. ha−1) could be expected to give only about 20% kill in the resistant population (), compared with 70% kill in the susceptible population. The predicted mortality for the susceptible population aligns with that achieved in earlier published field trials conducted with flumetsulam in New Zealand, where a single application of flumetsulam applied at 50 g a.i. ha−1 gave a 67% reduction in R. acris, averaged over nine field experiments (Harris & Husband Citation1997; Lamoureaux & Bourdôt Citation2007).
From the results of Experiments 2 and 3 it can be inferred that raising the rate of flumetsulam in a population that is showing resistance (e.g. P15) may not achieve a higher level of mortality because the proportion of survivors at these higher rates did not change. This suggests that the most resistant population was made up of a proportion of highly resistant individuals that would survive any realistic application rate and others that were susceptible. In most cases of resistance involving ALS-inhibitor herbicides, the mechanism is considered to be a target-site mutation (Tranel & Wright Citation2002) characterised by a high magnitude of resistance that is independent of herbicide dose rate, that is, resistant individuals can survive very high rates of herbicide. No attempt was made to determine the mechanisms of resistance here; however, it is evident that some individuals of P15 survived very high rates of flumetsulam, which may suggest that the mechanism for resistance in this case was also a target-site mutation. In the case of the plants resistant to the phenoxy herbicide, the resistance responses appeared more dependent on the MCPA dose rate because, unlike flumetsulam, there were no survivors at the very high rates. Therefore this rate-dependency response to MCPA may be due to herbicide inactivation through metabolic processes, which concurs with the conclusions of Bourdôt et al. (Citation1994).
This study shows that resistance can develop in populations of R. acris through repeated exposure to the only two groups of herbicides available for selective control in pastures. This suggests that the current management practice for R. acris of repeated application of currently available herbicides is unsustainable and there is no option for rotating herbicides from different groups, as is necessary for the management of herbicide resistance (Harrington Citation2005). Thifensulfuron-methyl (the only ALS-inhibitor alternative to flumetsulam) is not widely used because it is too damaging to pastures and it may have developed cross-resistance, evidently. There is no scope for increasing the rate of flumetsulam in order to get a higher level of mortality of resistant individuals, as rates would need to be increased so much that the cost of the herbicide would be uneconomic. Also, in New Zealand's clover-based pastures, the white clover (Trifolium repens) can be significantly damaged by flumetsulam when applied at above the recommended rate (Lusk et al. Citation2011). More research is needed on developing control methods with less reliance on chemicals and integrating as many non-chemical management practices as possible within affected dairy pastures; for example, rotating crops such as maize with pasture or mowing to prevent seeding. The more different tactics that are employed, the less rapidly resistant biotypes will evolve, prolonging the usefulness of the available herbicides.
The present study reports the first detection of a flumetsulam-resistant R. acris population in a dairy pasture in New Zealand. It is also one of a very few cases worldwide of a weed that has developed resistance to an ALS-inhibiting herbicide in a permanent pasture situation (Heap Citation2014). Further studies are needed to determine the wider existence of flumetsulam-resistant R. acris populations in New Zealand, where herbicide efficacy and past exposure are known. It is likely that more cases exist because many dairy farmers follow similar management practices using repeated applications of currently available herbicides. R. acris is also one of only 10 pasture weeds worldwide to have evolved resistance to either ALS-inhibitor or phenoxy herbicides (Heap Citation2014), and three of these cases have occurred in New Zealand (R. acris and two Carduus thistle species). The low incidence of pasture weed resistance is probably because of the relatively low use of herbicides in pastoral systems worldwide. By contrast, in New Zealand, herbicides are regularly used in dairy pastures because it is profitable to do so, at least in the short term, as a result of the current high economic returns from dairying.
Acknowledgements
The authors thank the farmers who provided helpful information on herbicide use and access to their farms for seed collection. Funding for the work was provided by the Ministry of Business Innovation and Employment (MBIE) in the Undermining Weeds programme (Contract No. C10X0811).
References
- Bourdôt GW, Hurrell GA 1988. Differential tolerance of MCPA among giantbuttercup (Ranunculus acris) biotypes in Takaka, Golden Bay. Proceedings of the 41st New Zealand Weed and Pest Control Conference, Auckland, New Zealand. Pp. 231–234.
- Bourdôt GW, Hurrell GA, Saville DJ 1990. Variation in MCPA-Resistance in Ranunculus acris L. subsp. acris and its correlation with historical exposure to MCPA. Weed Research 30: 449–457.
- Bourdôt GW, Hurrell GA, Saville DJ 1994. Herbicide cross-resistance in Ranunculus acris L. subsp. acris. New Zealand Journal of Agricultural Research 37: 255–262.
- Bourdôt GW, Saville DJ 2010. Giant buttercup—a threat to sustainable dairy farming in New Zealand. Christchurch, New Zealand, Australasian Dairy Science Symposium. Pp. 355–359.
- Bourdôt GW, Saville DJ, Crone D 2003. Dairy production revenue losses in New Zealand due to giant buttercup (Ranunculus acris). New Zealand Journal of Agricultural Research 46: 295–303.
- Bourdôt GW, Suckling DM eds. 1996. Pesticide resistance: prevention and management. Lincoln, New Zealand, New Zealand Plant Protection Society. 225 p.
- Harrington KC 1989. Distribution and cross-tolerance of MCPA-tolerant nodding thistle. Proceedings of the 41st New Zealand Weed and Pest Control Conference, Palmerston North, New Zealand. Pp. 39–42.
- Harrington KC 2005. General principles of herbicide resistance. In: Martin NA, Beresford RM, Harrington KC eds. Pesticide resistance: prevention and management strategies. Hastings, New Zealand, New Zealand Plant Protection Society. Pp. 143–145.
- Harrington KC, Popay AI, Robertson AG, McPherson HG 1988. Resistance of nodding thistle to MCPA in Hawkes Bay. Proceedings of the 41st New Zealand Weed and Pest Control Conference, Auckland, New Zealand. Pp. 219–222.
- Harris BM, Husband BM 1997. Flumetsulam for control of giant buttercup in pasture. Proceedings of the 50th New Zealand Plant Protection Conference, Lincoln, New Zealand. Pp. 472–476.
- Heap I 2014. International survey of herbicide resistant weeds. http://www.weedscience.org/In.asp ( accessed 5 December 2014).
- Hess M, Barralis H, Bleiholder H, Buhr L, Eggers TH, Hack H et al. 1997. Use of the extended BBCH scale-general for the descriptions of the growth stages of mono; and dicotyledonous weed species. Weed Research 37: 433–441.
- Lamoureaux SL, Bourdôt GW 2007. A review of the ecology and management of Ranunculus acris subsp. acris in pasture. Weed Research 47: 461–471.
- Lusk CS, Bourdôt GW, Harrington KC, Hurrell GA 2011. Pasture tolerance and efficacy of three herbicides used against giant buttercup (Ranunculus acris L. subsp. acris). New Zealand Plant Protection 64: 86–92.
- Tranel PJ, Wright TR 2002. Resistance of weeds to ALS-inhibiting herbicides: what have we learned? Weed Science 50: 700–712.
- Tuckett AJ 1961. Giant buttercup. Proceedings of the 14th New Zealand Weed and Pest Control Conference, New Plymouth, New Zealand. Pp. 124–126.
- Young, S 2008. New Zealand Novachem agrichemical manual. Christchurch, Agrimedia Ltd. 652 p.