Abstract
The aim of the experiment was to study diurnal variation in water-soluble carbohydrate (WSC) concentrations in leaf and stem of tall fescue and lucerne in autumn and to determine the impact of these changes on the rate and the extent of in vitro fermentation. Vegetative herbage of lucerne and tall fescue were sampled at 0900, 1300 and 1700 h, following three consecutive regrowths between April and May. Whole plants, leaf and stem were analysed for chemical composition and in vitro gas production. There was a linear increase in WSC throughout the day for all plants and plant components (P < 0.001). In both fescue and lucerne, this rise resulted in an increased rate of gas production, although this relationship was more pronounced in fescue. Use of time of day to govern grazing management decisions to potentially improve the rumen supply of WSC and nitrogen in autumn can be achieved from tall fescue or lucerne pastures.
Introduction
One of the key nutritional factors in temperate grazing systems is the enhancement of the digestive use of forages through ruminal fermentation. Nitrogen (N) compounds of temperate pastures are extensively and rapidly degraded in the rumen (Repetto et al. Citation2005; Fulkerson et al. Citation2007), releasing high amounts of NH3 to the ruminal environment (Cajarville & Aguerre et al. Citation2006; Cajarville & Pérez et al. Citation2006) that can be utilised by the microbiota. However, the quantity of readily fermentable carbohydrates is often insufficient, leading to low N utilisation and poor animal performance (Elizalde et al. Citation1996; García et al. Citation2000; Tebot et al. Citation2012). This inadequacy is even greater during autumn and early winter, as day length and luminosity are lower, and fewer water-soluble carbohydrates (WSC) are produced by the plants in comparison with late winter and spring (Delagarde et al. Citation2000).
It is well known that the concentration of WSC in plants increases during the day as a result of the balance between photosynthesis and respiration (Smith Citation1973; Ciavarella et al. Citation2000) and this can affect the performance of animals consuming these plants. Some studies have revealed that ruminants consume more Medicago sativa, commonly also called lucerne, or tall fescue (Festuca arundinacea) from hays harvested at sunset, in comparison with the same forage harvested at sunrise (Fisher et al. Citation1999, Citation2002; Burns et al. Citation2005) and this behaviour has been associated with an increase in WSC content. Other studies have associated a higher sugar concentration in the forage with grazing preferences of dairy cows (Horadagoda et al. Citation2009) or with an improvement in N usage (Huntington & Burns Citation2007) and fibre digestibility (Kokko et al. Citation2013) by the animal. The season can also influence the magnitude of the differences in the concentration of sugars throughout the day (Orr et al. Citation1997; Delagarde et al. Citation2000). However, it appears that no studies have focused on this during autumn, although this is the most challenging season regarding the WSC and N relationship and forage quality. In fact, Fulkerson et al. (Citation2007), in a seasonal nutrition study evaluating temperate legumes and grasses, observed that although the overall nutritive value was higher during the cool season (autumn and winter), WSC content was lower. During autumn, forage quality is high but forage availability is usually low (Mohammad Yusoff et al. Citation2013) and so grazing activities are restricted to a few hours a day.
Different parts of the plant differ in their nutritive value (Wilman & Rezvani Moghaddam Citation1998; Delagarde et al. Citation2000; Kaur et al. Citation2011). In grazing systems, grazing height defines which part of the plant the animals select, and therefore the final quality of the material ingested. Although it is known that leaves are higher in protein and lower in cell walls than stems, there is less information about the distribution in plants of other chemical components such as WSC, or variables related to digestive use, such as ruminal fermentation, and their variations over the day. The in vitro gas production method can be a useful tool to evaluate these variables (Rymer et al. Citation2005).
Our aim was to study variations in WSC concentrations over the course of a day, in leaves and stems, of forages commonly used for grazing in temperate zones during autumn, and the relationship between these changes and the extent and rate of fermentation.
Materials and methods
Site and experimental design
The study was conducted at the experimental station of INIA-La Estanzuela (Colonia, Uruguay, 34°20′S, 57°41′W), located entirely within the temperate zone of the Southern Hemisphere. During autumn (21 March–21 June) average high and low temperatures oscillate between 18 °C and 11.5 °C, respectively. Mean annual rainfall in this area is 1099 mm with 486 mm falling in the autumn (Meteorología Citation2007). The experimental area consisted of 30 ha: 10 ha in lucerne cv. Estanzuela Chana, 10 ha in lucerne cv. Crioula and 10 ha in tall fescue cv. Tacuabé, established as pure stands following cultivation in 2006. Soil type was a typic argiudol (USDA Citation1999) with a clay loam texture, and a 2%–4% slope. The area was grazed by cattle throughout the growing season. The herbage mass was measured before the beginning of the experiment (2 April 2007) by cutting 20 quadrats of each forage (0.1 m2) to soil level with shears.
As the main objective was to study the changes in chemical composition and in vitro gas production for leaf and stem of a grass and legume species throughout the day, a sampling scheme using three forage treatments was established. Each forage was sampled on three dates, at three times during the day on each of these dates. Each plant was divided into two plant components (i.e. leaves and stems). This sampling scheme was a hierarchical sampling with three forages (i.e. two lucerne and one tall fescue) and not a true factorial experiment.
Samples and sampling
Herbage from each forage treatment was cut on three dates: 3 April 2007, 8 May 2007 and 30 May 2007, at three different times: 0900, 1300 and 1700 h. Experimental areas were grazed by cattle immediately after each sampling. For all cuts, the plants were in a vegetative stage. Herbage samples were manually cut with shears at a height of 5 cm above the ground, from 10 random quadrats (0.1 m2) for each treatment. The herbage from each forage was treated separately throughout the experiment. For each date and time, the samples within a forage treatment were mixed and dried at 60 °C, and different plant components were manually separated into leaves (L), stems (S) or kept as whole plant (WP). Samples of L, S and WP of each forage, date and time of cut (n = 81) were ground to pass through a 1 mm screen, prior to chemical analysis and assessment using in vitro gas production.
Chemical analysis
Whole plants, leaves and stems from the different samples were analysed for organic matter (OM; 550 °C for 3 h according to AOAC Citation1990, ID 942.05), N (AOAC Citation1990, ID 942.01), acid detergent fibre (ADF; Robertson & Van Soest Citation1981) and WSC (Yemm & Willis Citation1954).
In vitro gas production
Samples of L, S and WP from each forage, date and time of cut were used as substrates to measure the in vitro gas production according to the method proposed by Theodorou et al. (Citation1994) and modified by Mauricio et al. (Citation1999). Duplicate samples (0.5 g dry matter [DM]) of each treatment were weighed into 125 mL flasks with 40 mL of a buffer (46.5 g L−1 Na2HPO4, 49 g L−1 NaHCO3, 2.35 g L−1 NaCl, 2.85 g L−1 KCl, 0.64 g L−1 MgCl and 0.2 g L−1 CaCl2), closed with a rubber stopper and hydrated at 4 °C for approximately 8 h. Flasks were then warmed to 39 °C and 10 mL of rumen liquor extracted at the time was incorporated. Rumen liquor was collected from a rumen-fistulated cow (400 kg bodyweight) fed lucerne hay (170 g crude protein [CP] kg−1 DM) ad libitum. The procedure was approved by the Bioethics Committee of Facultad de Veterinaria—UdelaR. Rumen fluid was filtered through four layers of cheesecloth and maintained in a warm flask (39 °C) under CO2 reflux. Flasks were then sealed with a rubber stopper and an aluminium crimp seal and placed in a water bath at 39 °C. All manipulations were performed under CO2 reflux. Gas production was recorded in pounds per square inch (psi) using a pressure transducer (Cole-Parmer, EW-68110-1, Illinois, USA) connected to the flask by a hypodermic needle inserted through the rubber stopper. Gas readings (psi) were taken at 2, 4, 6, 9, 12, 24, 48 and 72 h post-inoculation and corrected for blanks (two per run) using flasks with buffered rumen fluid without sample. All in vitro gas production measurements were performed in duplicate. Gas volumes (mL) were predicted using previously obtained pressure readings and a transducer connected to a three-way stopcock with a syringe to measure gas volume (mL) and a needle to insert into the flasks. Gas production was expressed as mL g−1 of DM of incubated sample. The gas volume produced for each forage sample (two runs) was fitted by non-linear regression to an exponential equation (Ørskov & McDonald Citation1979) as proposed by Chenost et al. (Citation2001) for forages:
Statistical analysis
As stated before, the sampling scheme included three forages (i.e. lucerne cv. Estanzuela Chana, lucerne cv. Crioula and tall fescue cv. Tacuabé), three sampling dates, three sampling times and two plant parts (i.e. leaves and stems).
A two-way approach following Milliken & Johnson (Citation1989) was performed to study the evolution of chemical composition and in vitro gas production for each plant component throughout the day, taking into account that the samples came from different forages and dates of cuts. First, an additive cell-means model was fitted as follows:
Linear relationships between WSC, WSC:N, in vitro gas production and time were studied for WP. Linear correlations (Pearson correlation coefficients, r) were estimated for chemical components and in vitro gas production means. In order to predict in vitro gas production from chemical components, multivariate linear regression equations were obtained using the stepwise procedure (SAS Citation1990) with a backward selection. Variables left in the model were those significant at the 0.15 level.
Table 1 Weather conditions on sampling dates and during the regrowth interval (mean values).
Table 2 Initial forage mass, dry matter content (g/kg), chemical composition (g kg DM−1) and percentage of leaves and stems (DM basis) of the pastures used in the study in each date of cut.
Results
Environmental conditions and forage characteristics
shows weather conditions on each sampling date and during the regrowth interval between samples. Heliofany (sun irradiation) was on average (3 April to 30 May) 6.5 h. Average temperatures for the whole period were 14.2 °C, oscillating between 10 °C and 19 °C with extremes of 1 °C and 31.2 °C (INIA Citation2007). The initial forage mass, the chemical composition and the percentage of L and S of each forage in each cut are shown in . Dry matter content of the whole plants during the sampling period oscillated between 150 and 220 g kg−1. Tall fescue had a high proportion of leaves for all cuts (85% on average). For both lucerne forages, CP and ADF contents increased and decreased, respectively, as the proportion of leaves increased.
Chemical composition
shows the observed variations in chemical composition according to the time of cutting for each plant component studied. A linear increase in WSC content, for both lucerne and tall fescue, occurred throughout the day (). From 0900 to 1700 h the mean WSC concentrations nearly doubled in both L and S, whereas N concentrations showed only a slight reduction in S tissue. The WSC:N ratio was more than double in the afternoon, showing a linear increase (R 2 = 0.387; P < 0.001). This trend was independent of the forage-date combination of sampling as the interaction between forage-date combination and time was non-significant (P > 0.05). Leaves had lower ADF (P < 0.05) and higher N (P < 0.001) concentrations than stems, with no differences in the concentration of sugars (WSC) (P > 0.05).
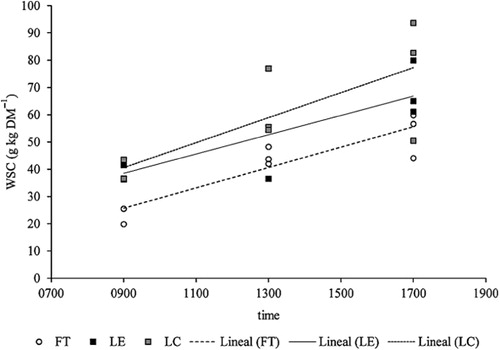
Table 3 Mean chemical composition (g kg DM−1) according to the timing of the cut (hour) for each plant component and P values and root mean squared error.
In vitro gas production
The in vitro fermentation patterns were less affected by the harvesting time than chemical composition. Although gas volumes (a + b) were higher for afternoon cuts, this was only significant in the WP tissues (). Similar results were observed for the gas production rate (c), with increases only significant for S tissue. A linear increase in c, the rate of gas production, throughout the day was observed for tall fescue (c = 0.09 × hour − 1.50; R 2 = 0.51; P = 0.03) but not for lucerne (P > 0.05). On the other hand, the rate of gas production was higher in L for lucerne (10.3% vs 9.0% h−1; P < 0.01), while L of tall fescue produced more gas (a + b, 141.6 vs 138.9 mL g−1 DM incubated; P < 0.05).
Table 4 In vitro gas production according to the timing of the cut (hour) for each plant component (PC) and P-values and root mean squared error (RMSE).
Relationships between chemical composition and in vitro gas production
The relationship between WSC and rate of gas production (c) () was low or non-significant for lucerne (lucerne cv. Estanzuela Chana (LE): r = 0.412, P = 0.033; lucerne cv. Crioula (LC): r = 0.361, P = 0.108; tall fescue cv. Tacuabé (FT): r = 0.721, P < 0.001). A high negative relationship between OM and c was observed for lucerne (r = −0.682; P < 0.001) and c could be predicted by OM, WSC and ADF (see legend ). No significant linear relationships (P > 0.05) were observed between gas volume and the chemical components measured.
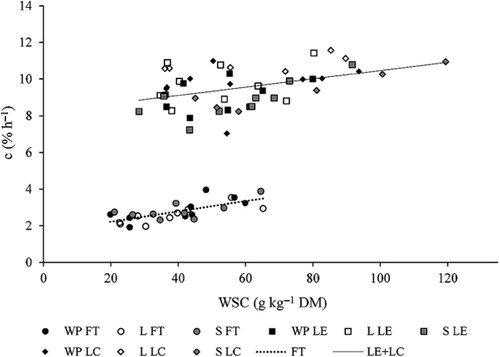
Discussion
In this study, the mean WSC content of the pastures was low (on average 48 g kg−1 DM), potentially due to the low sun exposure during the sampling period. Fulkerson et al. (Citation2007, Citation2008), working also in the Southern Hemisphere (Australia), reported similar WSC contents for C3 grasses, legumes and grain crops (triticale, oats and wheat) during autumn and winter.
Throughout the day, the most important change observed in chemical composition was the increase in WSC content, which mainly relates to photosynthesis (Smith Citation1973; Ciavarella et al. Citation2000). There was also a reduction in N content, which could be a dilution effect related to the accumulation of sugars in the plant as reported by Pelletier et al. (Citation2010). The simultaneous increase of WSC and declining N content led to an increase in the WSC:N ratios. Other authors had already described a rise in soluble sugars throughout the day. Delagarde et al. (Citation2000) reported an increase of WSC during the day (from 174 to 198 g kg−1 DM) for perennial ryegrass (spring and autumn). Ciavarella et al. (Citation2000) observed that WSC of Phalaris during spring ranged from 103 to 160 g kg−1 DM between sunrise and afternoon. Similarly, Orr et al. (Citation1997) sampling forages in summer at 0730 and 1930 h reported an increase of WSC for ryegrass from 156 to 183 g kg−1 DM and for white clover from 54 to 73 g kg−1 DM. Although our results were similar to these, two characteristics appear to be different from previous reports. One of them was the relatively low WSC content observed in both tall fescue and lucerne samples. The other was the dramatic increase of WSC content throughout the day. Leaves had 116.5% more WSC in the afternoon compared with the morning, while in stems WSC was 85.2% higher at 1700 h. The increase in WSC between morning and afternoon has been reported in other plant species, but of lower magnitude, for example, a 14% increase in perennial ryegrass (Delagarde et al. Citation2000) and 55% increase for Phalaris (Ciavarella et al. Citation2000). The increase observed in WSC from morning to afternoon has the potential to improve forage quality and could be used to influence decisions on time of harvest for silage production. Indeed, Tremblay et al. (Citation2014) observed that the higher sugar contents from lucerne afternoon cuts improved silage conservation attributes compared with cuts in the morning.
While higher N concentration and lower ADF content in leaves than in stems were expected (Jarrige et al. Citation1995; Wilman & Rezvani Moghaddam Citation1998; Kaur et al. Citation2011), similar WSC content between the two fractions was not. Delagarde et al. (Citation2000) observed higher contents of WSC—with a marked difference between morning and evening—in upper layers of a sward, with a higher proportion of leaves, due to a higher photosynthesis and gas exchange with the atmosphere in these layers. Meanwhile, Kaur et al. (Citation2011) observed that WSC content of forage rape harvested during autumn was 68% higher in stems than in leaves, supporting the hypothesis that the long-term carbohydrate reserve fraction (mainly fructans) are stored in stems (Jarrige et al. Citation1995). In our study, the concentration of WSC was similar in leaves and stems for lucerne and for tall fescue, potentially because of low photosynthetic activity due to a short day-length, leading to a low accumulation of fructans (Delagarde et al. Citation2000).
There are few studies reporting simultaneous measurements of WSC and in vitro gas volume in forages. For lucerne containing high levels of total non-structural carbohydrates (142 g kg−1 DM), Berthiaume et al. (Citation2006) reported mean gas volumes of 237 mL g−1. In the current study, the volume of gas measured in vitro was low for all the pastures and cuts analysed (mean 126 mL g−1 DM), which is consistent with the low WSC content.
One feature to highlight in the present work is that the magnitude of the rise of WSC concentration between sampling times was not reflected in the variation in the in vitro fermentation parameters. Maybe the relatively low WSC contents did not allow a more significant fermentation response. Anyway, the gas produced in vitro may not be a direct result of the chemical composition. Iantcheva et al. (Citation1999) observed lower volumes of gas than expected in high protein forages (180 g kg−1 DM) and explained this by the formation of NH4HCO3 from NH3. In the current study, NH4HCO3 formation could also have reduced the volume of gas produced.
Both lucerne cultivars (cv. Estanzuela Chana and cv. Crioula) showed a weak relationship between WSC and in vitro fermentation. Meanwhile, the increase in WSC in tall fescue cultivar used in our study led to a higher rate of fermentation. This finding could indicate different fermentation mechanisms for these forages, which deserves further study. On the other hand, the relationship between in vitro fermentation and the organic matter content of lucerne was the opposite of what was expected. These data suggest that there could have been other components (e.g. minerals, salts, secondary compounds, etc.) affecting in vitro fermentation in lucerne. Stefanon et al. (Citation1996), studying lucerne and brome grass hays harvested at different stages of maturity, observed unexpected in vitro fermentation results in immature lucerne, and suggested that it could contain substances that inhibit carbohydrate digestion. In our study, all the forages sampled were in a vegetative stage.
Information on the in vitro gas production of different plant components is scarce. Tang et al. (Citation2009) observed a higher potential gas and rate of gas production in stems than in leaf blades of maize (Zea mays) stover. In contrast, although the differences were very small, we observed a higher volume and gas production rate in leaves, indicating that this response can differ among forages and/or phenological stages. Further studies to analyse differences between plant species with regard to compounds that may inhibit fermentation are needed.
Overall, we conclude that there was an important increase of WSC content and WSC:N throughout the day for all plant components, which led to an increase in fermentation. The higher WSC content and fermentation of afternoon cuts should be considered as a tool in grazing management. When grazing activities are restricted to a few hours a day, grazing sessions should be conducted during the afternoon. Further studies are needed in order to quantify these responses with regard to plant species, plant maturity and seasons.
Acknowledgements
The authors want to thank the International Foundation for Science (Project: B/3028-1) and CSIC (Programa I+D, UdelaR) for their financial support. We also thank Sofia Stirling for the English language correction. Nicolás Errandonea had a scholarship supported by CIDEC (Facultad de Veterinaria, UdelaR).
References
- AOAC 1990. Association of official analytical chemists. Official methods of analysis. 15th edition. Arlington, VA, AOAC. 1230 p.
- Berthiaume R, Tremblay G, Castonguay Y, Bertrand A, Bélanger G, Lafrenière C et al. 2006. Length of the daylight period before cutting improves rumen fermentation of alfalfa assessed by in vitro gas production. Journal of Animal Science 84: 102.
- Burns JC, Mayland HF, Fisher DS 2005. Dry matter intake and digestion of alfalfa harvested at sunset and sunrise. Journal of Animal Science 83: 262–270.
- Cajarville C, Aguerre M, Repetto JL 2006. Rumen pH, NH3-N concentration and forage degradation kinetics of cows grazing temperate pastures and supplemented with different sources of grain. Animal Research 55: 511–520.10.1051/animres:2006036
- Cajarville C, Pérez A, Aguerre M, Britos A, Repetto JL 2006. Effect of timing of cut on ruminal environment of lambs consuming temperate pastures. Journal of Animal Science 84: 103.
- Chenost M, Aufrère J, Macheboeuf D 2001. The gas-test technique as a tool for predicting the energetic value of forage plants. Animal Research 50: 349–364.10.1051/animres:2001137
- Ciavarella TA, Simpson RJ, Dove H, Leury BJ, Sims IM 2000. Diurnal changes in the concentration of water-soluble carbohydrates in Phalaris aquatica L. pasture in spring, and the effect of short-term shading. Australian Journal of Agricultural Research 51: 749–756.10.1071/AR99150
- Delagarde R, Peyraud JL, Delaby L, Faverdin P 2000. Vertical distribution of biomass, chemical composition and pepsin––cellulase digestibility in a perennial ryegrass sward: interaction with month of year, regrowth age and time of day. Animal Feed Science and Technology 84: 49–68.10.1016/S0377-8401(00)00114-0
- Elizalde JC, Santini FJ, Pasinato AM 1996. The effect of stage of harvest on the processes of digestion in cattle fed winter oats indoors. II. Nitrogen digestion and microbial protein synthesis. Animal Feed Science and Technology 63: 245–255.
- Fisher DS, Mayland HF, Burns JC 1999. Variation in ruminants’ preference for tall fescue hays cut either at sundown or at sunup. Journal of Animal Science 77: 762–768.
- Fisher DS, Mayland HF, Burns JC 2002. Variation in ruminant preference for alfalfa hays cut at sunup and sundown. Crop Science 42: 231–237.10.2135/cropsci2002.0231
- Fulkerson WJ, Horadagoda A, Neal JS, Barchia I, Nandra KS 2008. Nutritive value of forage species grown in the warm temperate climate of Australia for dairy cows: herbs and grain crops. Livestock Science 114: 75–83.10.1016/j.livsci.2007.04.013
- Fulkerson WJ, Neal JS, Clark CF, Horadagoda A, Nandra KS, Barchia I 2007. Nutritive value of forage species grown in the warm temperate climate of Australia for dairy cows: grasses and legumes. Livestock Science 107: 253–264.10.1016/j.livsci.2006.09.029
- García SC, Santini FJ, Elizalde JC 2000. Sites of digestion and bacterial protein synthesis in dairy heifers fed fresh oats with or without corn or barley grain. Journal of Dairy Science 83: 746–775.
- Horadagoda A, Fulkerson WJ, Nandra KS, Barchia IM 2009. Grazing preferences by dairy cows for 14 forage species. Animal Production Science 49: 586–594.10.1071/EA08299
- Huntington GB, Burns JC 2007. Afternoon harvest increase readily fermentable carbohydrate concentration and voluntary intake of gamagrass and switchgrass baleage by beef steers. Journal of Animal Science 85: 276–284.10.2527/jas.2006-370
- Iantcheva N, Steingass H, Todorov N, Pavlov D 1999. A comparison of in vitro rumen fluid and enzymatic methods to predict digestibility and energy value of grass and alfalfa hay. Animal Feed Science and Technology 81: 333–344.10.1016/S0377-8401(99)00037-1
- INIA 2007. Banco Agroclimático de Estaciones INIA. Instituto Nacional de Investigación Agropecuaria. http://www.inia.org.uy/online/site/143782I1.php ( accessed 30 January 2008).
- Jarrige R, Grenet E, Demarquilly C, Beslé JM 1995. Les constituants de l’appareil végétatif des plantes fourragères. In: Jarrige R, Ruckebusch Y, Demarquilly C, Farce MH, Journet M eds. Nutrition des ruminants domestiques. Ingestion et digestion. Paris, France, INRA. Pp. 25–81.
- Kaur R, Garcia SC, Fulkerson WJ, Barchia IM 2011. Degradation kinetics of leaves, petioles and stems of forage rape (Brassica napus) as affected by maturity. Animal Feed Science and Technology 168: 165–178.10.1016/j.anifeedsci.2011.04.093
- Kokko C, Soder KJ, Brito AF, Hovey RS, Berthiaume R 2013. Effect of time of cutting and maceration on nutrient flow, microbial protein synthesis, and digestibility in dual-flow continuous culture. Journal of Animal Science 91: 1765–1774.
- Mauricio RM, Mould FL, Dhanoa MS, Owen E, Channa KS, Theodorou MK 1999. A semi-automated in vitro gas production technique for ruminant feedstuff evaluation. Animal Feed Science and Technology 79: 321–330.10.1016/S0377-8401(99)00033-4
- Meteorología 2007. El Otoño. Aspectos generales del clima durante el otoño en el Uruguay. Dirección Nacional de Meteorología. http://www.meteorologia.com.uy/OTO%D1O_2007.pdf ( accessed 30 January 2008).
- Milliken GA, Johnson DE 1989 Analysis of messy data. Volume 2. Nonreplicated experiments. New York, Chapman & Hall/CRC. 203 p.
- Mohammad Yusoff M, McKenzie BA, Moot DJ, Hill GD 2013. Dry matter accumulation and nutritive value of winter forage crops sown in Canterbury on different dates. New Zealand Journal of Agricultural Research 56: 309–321.10.1080/00288233.2013.845232
- Orr RJ, Penning PD, Harvey A, Champion RA 1997. Diurnal patterns of intake rate by sheep grazing monocultures of ryegrass and white clover. Applied Animal Behaviour Science 52: 65–77.10.1016/S0168-1591(96)01120-3
- Ørskov ER, McDonald I 1979. The estimation of protein degradability in the rumen from incubation measurements weighted according to a rate of passage. Journal of Agricultural Science (Cambridge) 92: 499–503.
- Pelletier S, Tremblay G, Bélanger G, Bertrand A, Castonguay Y, Pageau D et al. 2010. Forage nonstructural carbohydrates and nutritive value as affected by time of cutting and species. Agronomy Journal 102: 1388–1398.10.2134/agronj2010.0158
- Repetto JL, Cajarville C, D’Alessandro J, Curbelo A, Soto C, Garín D 2005. Effect of wilting and ensiling on ruminal degradability of temperate grass and legume mixtures. Animal Research 54: 73–80.10.1051/animres:2005007
- Robertson JB, Van Soest PJ 1981. The detergent system of analysis and its application to human foods. In: James WPT, Theander O eds. The analysis of dietary fibre in food. New York, Marcel Dekker. Pp. 123–158.
- Rymer C, Huntington JA, Williams BA, Givens DI 2005. In vitro cumulative gas production techniques: history, methodological considerations and challenges. Animal Feed Science and Technology 123–124: 9–30.
- SAS 1990. SAS/STAT user’s guide, version 6. 4th edition. Cary, NC, SAS Institute Inc. 1848 p.
- Smith D 1973. The non-structural carbohydrates. In: Butler GW, Dailey RW eds. Chemistry and biochemistry of herbage Vol 1. New York, Academic Press. Pp. 105–155.
- Stefanon B, Pell AN, Schofield P 1996. Effect of maturity on digestion kinetics of water-soluble and water-insoluble fractions of alfalfa and brome hay. Journal of Animal Science 74: 1104–1115.
- Tang SX, Sheng LX, Tan ZL, Tayo GO, Liao HY, Sun ZH et al. 2009. Morphological fractions and in vitro fermentation characteristics of five endosperm types maize stover harvested at two maturity stages. Journal of Animal and Feed Sciences 18: 582–598.
- Tebot I, Cajarville C, Repetto JL, Cirio A 2012. Supplementation with non-fibrous carbohydrates reduced fiber digestibility and did not improve microbial protein synthesis in sheep fed fresh forage of two nutritive values. Animal 6: 617–623.10.1017/S1751731111002011
- Theodorou MK, Williams BA, Dhanoa MS, McAllan AB, France J 1994. A simple gas production method using a pressure transducer to determine the fermentation kinetics of ruminant feeds. Animal Feed Science and Technology 48: 185–197.10.1016/0377-8401(94)90171-6
- Tremblay GF, Morin C, Bélanger G, Bertrand A, Castonguay Y, Berthiaume R et al. 2014. Silage fermentation of PM- and AM-cut alfalfa wilted in wide and narrow swaths. Crop Science 54: 439–452.10.2135/cropsci2013.07.0443
- USDA (Soil Survey Staff) 1999. Soil taxonomy. A basic system of soil classification for making and interpreting soil surveys. 2nd edition. Agriculture handbook 436. Washington, DC, United States Department of Agriculture, Natural Resources Conservation Service. 871 p.
- Wilman D, Rezvani Moghaddam P 1998. In vitro digestibility and neutral detergent fibre and lignin contents of plant parts of nine forage species. Journal of Agricultural Science 131: 51–58.10.1017/S0021859698005620
- Yemm EW, Willis AJ 1954. The estimation of carbohydrates in plant extracts by anthrone. Biochemical Journal 57: 508–514.