Abstract
In New Zealand vineyards, evolution of glyphosate resistance in perennial ryegrass (Lolium perenne) has been reported recently, and restricted translocation of glyphosate has been found in these resistant plants. Past research with other plant species has found that restricted glyphosate translocation is temperature dependent. Glasshouse dose-response experiments were conducted with resistant perennial ryegrass at different temperatures to investigate if this population becomes susceptible to glyphosate under cooler conditions. Glyphosate-susceptible plants (population SP) grown in either warm (average of 20 °C) or cool (average of 9 °C) temperatures following treatment with several rates of glyphosate responded similarly to the herbicide regardless of temperature. In contrast, plants of population J (glyphosate resistant) were significantly more resistant than population SP to glyphosate when treated under warm conditions, but at the cooler temperature, population J was as susceptible as population SP. Thus the mechanism of resistance in population J appeared to be suppressed under cool conditions, suggesting that application of glyphosate during winter might improve control of glyphosate-resistant perennial ryegrass infestations.
Introduction
Glyphosate is the world's most important herbicide due to its broad-spectrum activity and usefulness in seed-bed preparation, so evolution of resistance to it has posed a threat to modern agriculture (Powles Citation2008). Glyphosate has been one of most commonly used herbicides for weed management in orchards and vineyards in New Zealand (Dastgheib & Frampton Citation2000). Recently, the first cases of glyphosate resistance to develop in New Zealand were reported for perennial ryegrass (Lolium perenne L.) from several vineyards where glyphosate had been applied intensively for more than 10 years (Ghanizadeh et al. Citation2013, Citation2015a). Both target site (enzyme modification at Pro-106 [Baerson et al. Citation2002] and gene amplification [Gaines et al. Citation2010]) and non-target site (restricted herbicide translocation [Lorraine-Colwill et al. Citation2002]) mechanisms have been shown to be associated with glyphosate resistance in weeds. The restricted herbicide translocation mechanism has been found to be associated with resistance to glyphosate in two perennial ryegrass populations from New Zealand, although one of the populations had both the enzyme modification at Pro-106 and the restricted herbicide translocation (Ghanizadeh et al. Citation2015b).
The restricted glyphosate translocation could involve more sequestration of glyphosate in vacuoles within biotypes resistant to this herbicide compared with susceptible ones (Ge et al. Citation2010). Studies suggest that a unidirectional active transporter in tonoplasts probably assists vacuolar sequestration of glyphosate in resistant weeds with the restricted herbicide translocation (Sammons & Gaines Citation2014). However, glyphosate vacuole sequestration has been found to be temperature dependent in Conyza canadensis and the restricted herbicide translocation mechanism of resistance does not operate efficiently at sub-optimal (cold) temperatures (Ge et al. Citation2011). Similarly, it has been shown that glyphosate resistance in Sorghum halepense and Lolium rigidum caused by the restricted herbicide translocation mechanism of resistance is also suppressed at cooler temperatures (Vila-Aiub et al. Citation2013). Only weed species with the vacuolar sequestration mechanism of resistance appear to become sensitive to glyphosate at cool temperatures and those weeds with only a target site mutation at Pro-106 remain resistant to glyphosate when treated at sub-optimal temperatures (Sammons & Gaines Citation2014).
Although Ghanizadeh et al. (Citation2015b) have shown that glyphosate translocation is restricted in resistant perennial ryegrass from New Zealand vineyards, sequestration within vacuoles has not yet been investigated. If glyphosate resistance is reduced under cool conditions, this would provide some indirect evidence for vacuolar sequestration being involved. It would also provide a method for controlling these resistant plants within vineyards. The objective of the work described in this paper was to determine whether glyphosate-resistant perennial ryegrass with the restricted herbicide translocation becomes less resistant when treated under cooler conditions.
Materials and methods
Dose-response experiments
Conventional dose-response studies were conducted using a glyphosate-resistant population of perennial ryegrass with the restricted herbicide translocation obtained from a Marlborough vineyard (population J) (Ghanizadeh et al. Citation2015b), to determine the magnitude of resistance compared with a susceptible population under a variety of conditions. Plants of a susceptible population of perennial ryegrass (cv. Trojan) (population SP) were obtained from grazed pastures at Massey University in Manawatu as they were unlikely to have been exposed to glyphosate in the past, and had been shown to be susceptible by Ghanizadeh et al. (Citation2013). Plants were grown using the technique described by Ghanizadeh et al. (Citation2015a) which involved establishing plantlets each with two to three tillers in pots filled with potting mix. The plants were then left to develop in a heated glasshouse at Massey University and irrigated daily. When plants from each population had an average of seven to eight tillers, they were allocated to one of three groups. One group remained in the heated glasshouse, which was covered in shade-cloth to give comparable light intensities to the other two environments described below. The average light intensity measured over several days between 1100 h and 1400 h was 150 ± 10 μmol/m2/s in the heated glasshouse. The second group was shifted on 17 July 2014 to a growth chamber kept at a constant cool temperature of 8 °C with a 10 h photoperiod and 100 ± 2 μmol/m2/s light intensity. The third group was shifted on the same day to an unheated shadehouse to simulate winter growing conditions, with a light intensity of 120 ± 5 μmol/m2/s.
The relocated plants were left in their new environments for 1 week before spraying with glyphosate to allow their physiology to adapt to the reduced temperatures. Plants of all three groups were then sprayed with different rates of glyphosate (Roundup Pro 360, an isopropylamine salt) using a laboratory track sprayer calibrated to deliver 249 L/ha of spray solution at 200 kPa on 24 July 2014 (Experiment 1). Both populations received rates of 0, 80, 160, 320, 640 and 1280 g ae/ha. All herbicide treatments contained 0.1% organosilicone surfactant (Pulse Penetrant). After glyphosate treatment, plants were immediately put back into their allocated environments. The average daily temperature in the 2 weeks following application (with the daily maximum and minimum temperatures in brackets) was 20.1 °C (25.6 °C to 17.6 °C) for the heated glasshouse, 9.1 °C (16.2 °C to 4.7 °C) for the unheated shadehouse, and 8.6 °C (10.9 °C to 6.7 °C) for the cool growth room. The foliage of all plants was removed from pots at ground level 10 weeks after treatment and the dry weight of plant material was determined. Measuring the effect of different rates of herbicides on biomass production of treated plants relative to untreated plants is a common and accepted technique for evaluating herbicide resistance and estimating the level of resistance (Knezevic et al. Citation2007; Burgos et al. Citation2013). Thus, the effect of each herbicide treatment was calculated as a percentage of the dry weight of untreated plants for that population and temperature regime.
This experiment was then repeated except that only the heated glasshouse and the cool growth chamber were used as this experiment started on 29 October 2014 (Experiment 2). The daily average temperatures in the 2 weeks following application (with the daily maximum and minimum temperatures in brackets) was 20.3 °C (26.9 °C to 14.8 °C) for the heated glasshouse and 9.7 °C (11.8 °C to 7.4 °C) for the cool growth room.
Statistical analysis
Both experiments were conducted using a randomised complete block design with eight replicates of each rate. The basic assumptions for ANOVA and regression models were checked using methods described by Onofri et al. (Citation2010). A three-parameter logistic model was fitted to the data from each experiment using the following equation:where Y was plant biomass as a percentage of untreated control, d was the upper limit, x was herbicide rate, GR50 was the rate of herbicide corresponding to 50% reduction in plant biomass and b was the slope around the GR50. The data were fitted to this model using the statistical software R (Version 2.15.2) with its dose-response curve (drc) package (Knezevic et al. Citation2007). A three-way ANOVA was conducted using IBM SPSS v.20 to compare the effects of temperature, resistance status and glyphosate rates on biomass production of treated populations. The means were separated using a Fisher's (LSD) test at 5% probability. A one-way ANOVA was performed to compare parameters estimated from the dose-response tests between the two perennial ryegrass populations at different temperatures using Graphpad Prism v.5 and means were separated using a Tukey's test at 5% probability (Ademola & Eloff Citation2011).
Results
and illustrate the effect of glyphosate application on the glyphosate-resistant population (J) and glyphosate-susceptible population (SP) at different temperatures in Experiments 1 and 2. An analysis of variance of the regression model and the values of coefficient of determination (R2) revealed that the three parameter model provided a good fit of the data for both populations regardless of temperature conditions (data not shown). In both experiments, the untreated plants of both populations remained healthy until the end of the experiment for all growing conditions, thus plant health was not adversely affected by the lower temperatures.
Figure 1 Fitted dose-response curves (on log10 dose scale) for two populations of perennial ryegrass in Experiment 1 (SP [glyphosate susceptible] from Manawatu pasture and J [glyphosate resistant] from Marlborough vineyards) for the effect of glyphosate on shoot dry weight in: A, a heated glasshouse (20 °C); B, a cool growth room (below 10 °C); and C, a shadehouse in winter (daily minimum temperatures of 5 °C).
![Figure 1 Fitted dose-response curves (on log10 dose scale) for two populations of perennial ryegrass in Experiment 1 (SP [glyphosate susceptible] from Manawatu pasture and J [glyphosate resistant] from Marlborough vineyards) for the effect of glyphosate on shoot dry weight in: A, a heated glasshouse (20 °C); B, a cool growth room (below 10 °C); and C, a shadehouse in winter (daily minimum temperatures of 5 °C).](/cms/asset/14c20031-ce70-4716-bbf6-405bcb265f72/tnza_a_1076490_f0001_b.gif)
Figure 2 Fitted dose-response curves (on log10 dose scale) for two populations of perennial ryegrass in Experiment 2 (SP [glyphosate susceptible] from Manawatu pasture and J [glyphosate resistant] from Marlborough vineyards) for the effect of glyphosate on shoot dry weight in: A, a heated glasshouse (20 °C); and B, a cool growth room (below 10 °C).
![Figure 2 Fitted dose-response curves (on log10 dose scale) for two populations of perennial ryegrass in Experiment 2 (SP [glyphosate susceptible] from Manawatu pasture and J [glyphosate resistant] from Marlborough vineyards) for the effect of glyphosate on shoot dry weight in: A, a heated glasshouse (20 °C); and B, a cool growth room (below 10 °C).](/cms/asset/8d7d7c82-b7f6-45f8-8c36-9fc15ed5d6a7/tnza_a_1076490_f0002_b.gif)
In both experiments, significant differences in the response to glyphosate were recorded for population J at different temperatures. When the plants of glyphosate-resistant population J were kept in the heated glasshouse (c. 20 °C), only the glyphosate rate of 1280 g ae/ha provided adequate control. In contrast, a rate of just 320 g ae/ha was sufficient to kill all plants from population J when kept in the cool growth room (below 10 °C) (A,C). However, the plants of the susceptible population SP responded similarly to glyphosate when kept in the glasshouse or in the cool growth room, with a glyphosate rate of 320 g ae/ha providing adequate control of these plants under both temperature regimes (B,D).
Figure 3 Effect after 10 weeks of different rates of glyphosate in Experiment 1 on resistant perennial ryegrass population J (panels A and C) and susceptible population SP (B and D) kept in either a heated glasshouse (20 °C) (A and B) or a cool growth room (below 10 °C) (C and D).
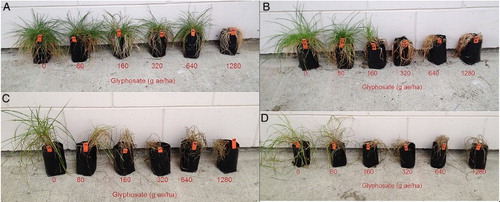
In Experiment 1, when plants were grown in the heated glasshouse (20 °C), the glyphosate rate that caused 50% reduction in growth (GR50) for population J was 749 g ae/ha, whereas the GR50 for this population dropped significantly to 154 g ae/ha when plants were kept in the cool growth room (below 10 °C) and to 84 g ae/ha in the unheated shadehouse (with daily minimum temperatures averaging 4.7 °C) ().
Table 1 Parameters estimated from the logistic regression analysis of glyphosate dose-response experiments of two perennial ryegrass populations, treated at three different temperature conditions, at 10 weeks after application of glyphosate in Experiments 1 and 2. The fitted dose-response curves are presented in and for Experiments 1 and 2, respectively.
In contrast, there were no significant differences in GR50 values for population SP for all three temperature regimes, with GR50 values of 147, 173 and 64 g ae/ha when plants were kept in the heated glasshouse, cool growth room and unheated shadehouse, respectively (). The results were very similar in Experiment 2 with GR50 values for population J of 940 and 170 g ae/ha when plants were kept in the heated glasshouse (20 °C) and the cool growth room (below 10 °C) respectively, while the GR50 values for population SP were 203 and 204 in the warm and cool conditions, respectively ().
The results of a three-way analysis of variance for Experiments 1 and 2 () also showed that temperature had a significant impact on the glyphosate resistance of population J (P < 0.0001). A comparison of the means of dry weight attained by the plants of population J and SP at each rate of glyphosate under different conditions in Experiments 1 and 2 is shown in . For each rate of glyphosate tested, plant biomass of treated plants of population J in the cooler conditions was significantly different from those treated under warm conditions (A,B). However, almost no significant differences in plant biomass were observed between treated plants of population SP at every rate of glyphosate regardless of growing conditions (C,D).
Figure 4 Biomass production of plants (as a percentage of untreated) from the resistant population J (A and B) and susceptible population SP (C and D) treated with different rates of glyphosate at different temperatures in Experiment 1 (population J [A], population SP [C]) and Experiment 2 (population J [B] and population SP [D]). Mean values within each glyphosate rate with the same letters are not different at 5% probability.
![Figure 4 Biomass production of plants (as a percentage of untreated) from the resistant population J (A and B) and susceptible population SP (C and D) treated with different rates of glyphosate at different temperatures in Experiment 1 (population J [A], population SP [C]) and Experiment 2 (population J [B] and population SP [D]). Mean values within each glyphosate rate with the same letters are not different at 5% probability.](/cms/asset/397f9213-5e53-4a83-ab92-0a6e28ae56d5/tnza_a_1076490_f0004_b.gif)
Table 2 A three-way ANOVA of the effect of glyphosate resistance, glyphosate rates and temperatures on dry weight of two populations of perennial ryegrass (population SP [Manawatu pasture] and population J [Marlborough]) in Experiments 1 and 2.
When the level of resistance to glyphosate was evaluated based on R/S (resistant/susceptible) GR50 ratios at different temperatures, in Experiment 1, population J was 5.1 times more resistant to glyphosate when plants were kept in the heated glasshouse, while the level of resistance to glyphosate for population J decreased significantly in the cool growth room and the unheated shadehouse where it was 0.9 and 1.3 times, respectively. In Experiment 2, similar results were obtained for population J which was 4.6 times more resistant than population SP in the warm conditions, while in the cool conditions population J was once again just as susceptible as population SP to glyphosate.
Discussion
These two experiments showed that glyphosate resistance in population J, which is known to involve the restricted herbicide translocation, was suppressed by sub-optimal growing temperatures (below 10 °C). Similar results have been reported for glyphosate-resistant C. canadensis (Ge et al. Citation2011), L. rigidum and S. halepense (Vila-Aiub et al. Citation2012). Glyphosate performance is temperature-dependent and glyphosate efficacy increases at warm temperatures compared with cool temperatures (Tanpipat et al. Citation1997; Adkins et al. Citation1998). This is consistent with our observations as treated plants of both populations did not show any symptoms (e.g. chlorosis) for almost 4 weeks after glyphosate treatment at cooler temperatures, whereas plants kept in warm conditions had symptoms appear 2 weeks after glyphosate application. Despite lower temperatures resulting in plants being affected more slowly by glyphosate, in population SP the dose-response curves were not significantly different between cool and warm conditions for either experiment. However, low temperatures had a much larger effect on the response to glyphosate in population J, making the plants so susceptible to glyphosate that they became as sensitive as population SP.
Although there were some differences in the light intensity between the different environments used in this study, these slight differences are unlikely to have caused the different responses to glyphosate recorded for population J in the heated glasshouse compared with the cooler environments. Although a reduction in light intensity may reduce glyphosate translocation slightly (Coupland Citation1983), the consistent responses to glyphosate recorded for population SP at the various temperature regimes suggest that the light intensity did not have a significant impact on glyphosate efficacy. Reducing the light intensity by 50% did not change the response to glyphosate in Avena fatua or Urochloa panicoides (Adkins et al. Citation1998). Glyphosate sequestration was not light-dependent in resistant plants studied elsewhere as identical amounts of glyphosate vacuolar sequestration have been measured under dark and light conditions (Ge et al. Citation2010). Therefore, the substantial differences recorded for population J under the different growing conditions were unlikely to have been caused by the small difference in the light intensity.
Suppression of glyphosate resistance in perennial ryegrass under cool conditions suggests that vacuolar sequestration may be responsible for restricted glyphosate resistance observed in this population (Ge et al. Citation2011). The molecular, physiological and biochemical mechanisms that cause sub-optimal temperatures to reduce the vacuolar sequestration have not been completely elucidated. Ge et al. (Citation2011) found that resistant plants of C. canadensis were still able to sequester glyphosate into vacuoles under cool conditions, but the amount of sequestration was significantly lower than in warm conditions. Interestingly, it was shown that plants with the vacuolar sequestration mechanism of resistance, treated with glyphosate at cool temperatures, were able to sequester the glyphosate rapidly into vacuoles once they were shifted to warmer conditions (Ge et al. Citation2011). Glyphosate sequestration into vacuoles is hypothesised to be mediated by an active transporter (Sammons & Gaines Citation2014). If so, then sub-optimal temperatures may cause thermal deactivation of this transporter but this deactivation is reversible by exposing plants to optimal temperatures (Yoshida & Matsura-Endo Citation1991). Further studies are needed to investigate the role of transporters in the vacuolar sequestration of glyphosate and also the effect of temperature on the putative transporter's catalytic activity.
The suppression of the mechanism of resistance to glyphosate in population J under cool temperatures has obvious practical implications. Application of glyphosate during winter while it is cold should provide greatly improved control of glyphosate-resistant perennial ryegrass infestations within vineyards. Field trials are now required to test this hypothesis. However, another perennial ryegrass population resistant to glyphosate that has been studied from Marlborough (population O) has been found to have two mechanisms of resistance (Ghanizadeh et al. Citation2015b). In addition to the restricted glyphosate translocation (non-target site mechanism of resistance), the plants from this population also have a modified gene (target site mechanism of resistance) that affects the structure of the enzyme that is normally inhibited by glyphosate. Although the target site mechanism of resistance is temperature insensitive (Sammons & Gaines Citation2014), it generally only makes plants two to three times more resistant to glyphosate (Baerson et al. Citation2002). Thus if most of the resistance in population O is because of restricted translocation, spraying in winter may be successful for these plants too if a higher rate is used.
Conclusion
Under cool conditions typical of winter in New Zealand, a population of perennial ryegrass that has evolved resistance to glyphosate in Marlborough vineyards becomes sensitive to the herbicide. This suggests that the restricted glyphosate translocation known to exist in this population could involve the vacuolar sequestration because similar reductions in resistance under cool conditions have been reported previously where the vacuolar sequestration causes glyphosate resistance. Thus control of glyphosate-resistant perennial ryegrass may be possible by spraying glyphosate during cool times of the year.
Acknowledgements
The authors wish to thank the staff of the Plant Growth Unit of Massey University for assistance with growing the plants. They are also grateful for the financial assistance provided by the Massey University Doctoral Scholarship, Seed Tech Services Scholarship, Dan Watkins Scholarship in Weed Science, George Mason Sustainable Land Use Scholarship, Hurley Fraser Postgraduate Scholarship, the Ministry for Primary Industries through the Sustainable Farming Fund and the Foundation of Arable Research.
Disclosure statement
No potential conflict of interest was reported by the authors.
References
- Ademola IO, Eloff JN 2011. Anthelmintic efficacy of cashew (Anarcadium occidentale L.) on in vitro susceptibility of the ova and larvae of Haemonchus contortus. African Journal of Biotechnology 10: 9700–9705.
- Adkins SW, Tanpipat S, Swarbrick JT, Boersma M 1998. Influence of environmental factors on glyphosate efficacy when applied to Avena fatua or Urochloa panicoides. Weed Research 38: 129–138. doi: 10.1046/j.1365-3180.1998.00083.x
- Baerson SR, Rodriguez DJ, Tran M, Feng YM, Biest NA, Dill GM 2002. Glyphosate-resistant goosegrass. Identification of a mutation in the target enzyme 5-enolpyruvylshikimate-3-phosphate synthase. Plant Physiology 129: 1265–1275. doi: 10.1104/pp.001560
- Burgos NR, Tranel PJ, Streibig JC, Davis VM, Shaner D, Norsworthy JK, et al. 2013. Review: confirmation of resistance to herbicides and evaluation of resistance levels. Weed Science 61: 4–20. doi: 10.1614/WS-D-12-00032.1
- Coupland D 1983. Influence of light, temperature and humidity on the translocation and activity of glyphosate in Elymus repens (Agropyron repens). Weed Research 23: 347–355. doi: 10.1111/j.1365-3180.1983.tb00558.x
- Dastgheib F, Frampton C 2000. Weed management practices in apple orchards and vineyards in the South Island of New Zealand. New Zealand Journal of Crop and Horticultural Science 28: 53–58. doi: 10.1080/01140671.2000.9514122
- Gaines TA, Zhang WL, Wang D, Bukun B, Chisholm ST, Shaner DL, et al. 2010. Gene amplification confers glyphosate resistance in Amaranthus palmeri. Proceedings of the National Academy of Sciences 107: 1029–1034. doi: 10.1073/pnas.0906649107
- Ge X, d'Avignon DA, Ackerman JJH, Duncan B, Spaur MB, Sammons RD 2011. Glyphosate-resistant horseweed made sensitive to glyphosate: low-temperature suppression of glyphosate vacuolar sequestration revealed by 31P NMR. Pest Management Science 67: 1215–1221. doi: 10.1002/ps.2169
- Ge X, d'Avignon DA, Ackerman JJH, Sammons RD 2010. Rapid vacuolar sequestration: the horseweed glyphosate resistance mechanism. Pest Management Science 66: 345–348.
- Ghanizadeh H, Harrington KC, James TK, Woolley DJ 2013. Confirmation of glyphosate resistance in two species of ryegrass from New Zealand vineyards. New Zealand Plant Protection 66: 89–93.
- Ghanizadeh H, Harrington KC, James TK, Woolley DJ 2015a. Quick tests for detecting glyphosate-resistant Italian and perennial ryegrass. New Zealand Journal of Agricultural Research 58: 108–120. doi: 10.1080/00288233.2014.981344
- Ghanizadeh H, Harrington KC, James TK, Woolley DJ, Ellison NW 2015b. Mechanisms of glyphosate resistance in two perennial ryegrass (Lolium perenne) populations. Pest Management Science (in press).
- Knezevic SZ, Streibig JC, Ritz C 2007. Utilizing R software package for dose-response studies: the concept and data analysis. Weed Technology 21: 840–848. doi: 10.1614/WT-06-161.1
- Lorraine-Colwill DF, Powles SB, Hawkes TR, Hollinshead PH, Warner SAJ, Preston C 2002. Investigations into the mechanism of glyphosate resistance in Lolium rigidum. Pesticide Biochemistry and Physiology 74: 62–72. doi: 10.1016/S0048-3575(03)00007-5
- Onofri A, Carbonell EA, Piepho HP, Mortimer AM, Cousens RD 2010. Current statistical issues in weed research. Weed Research 50: 5–24. doi: 10.1111/j.1365-3180.2009.00758.x
- Powles SB 2008. Evolved glyphosate-resistant weeds around the world: lessons to be learnt. Pest Management Science 64: 360–365. doi: 10.1002/ps.1525
- Sammons RD, Gaines TA 2014. Glyphosate resistance: state of knowledge. Pest Management Science 70: 1367–1377. doi: 10.1002/ps.3743
- Tanpipat S, Adkins SW, Swarbrick JT, Boersma M 1997. Influence of selected environmental factors on glyphosate efficacy when applied to awnless barnyard grass (Echinochloa colona (L.) Link). Australian Journal of Agricultural Research 48: 695–702. doi: 10.1071/A96141
- Vila-Aiub MM, Balbi MC, Distéfano AJ, Fernández L, Hopp E, Yu Q, et al. 2012. Glyphosate resistance in perennial Sorghum halepense (Johnsongrass), endowed by reduced glyphosate translocation and leaf uptake. Pest Management Science 68: 430–436. doi: 10.1002/ps.2286
- Vila-Aiub MM, Gundel PE, Yu Q, Powles SB 2013. Glyphosate resistance in Sorghum halepense and Lolium rigidum is reduced at suboptimal growing temperatures. Pest Management Science 69: 228–232. doi: 10.1002/ps.3464
- Yoshida S, Matsura-Endo C 1991. Comparison of temperature dependency of tonoplast proton translocation between plants sensitive and insensitive to chilling. Plant Physiology 95: 504–508. doi: 10.1104/pp.95.2.504