ABSTRACT
Phosphorus loss from pastures can impair surface water quality. Losses can be partly minimised by maintaining a low soil Olsen P concentration, but there is still potential to reduce these further by minimising P lost from plant shoot material. Furthermore, although ryegrass can produce well at low Olsen P, many legumes cannot. We tested and categorised the potential for P losses (as water extractable P) from shoot material for 10 species (ryegrass, legumes and herbs) and the supporting Pallic soil over a range of Olsen P concentrations. White clover was categorised as having low potential for P loss from shoot material while exhibiting high yield relative to other species. This was especially the case at an Olsen P concentration of 15 mg kg−1—targeted as the minimum Olsen P required for good ryegrass yield, but requiring little P inputs, and likely to lose little P to surface water. However, other legumes may be more suitable under different soil or climatic conditions.
KEYWORDS:
Introduction
Phosphorus (P) is a limiting nutrient for surface water eutrophication (Smith et al. Citation2006). In grazed grassland, P can be lost from a range of sources: the soil, as dissolved P or as particulate P in eroded soil particles (McDowell et al. Citation2003a); from fertiliser or dung prior to P sorption to topsoil (McDowell et al. Citation2003b); and from plant shoots (McDowell et al. Citation2007). Under the equivalent hydrological and topographic conditions, the magnitude of loss is proportional to the amount of P applied, but varies between sources. For instance, the potential for P losses from fertiliser can be initially high, but decreases exponentially with time as the large pool of water soluble P is sorbed to topsoil (McDowell Citation2006). Runoff soon after application can result in fertiliser accounting for 70%–80% of annual P losses (Hart et al. Citation2004). If no runoff occurs, annual losses will be a reflection of topsoil P concentration (McDowell et al. Citation2003a).
Decreasing P losses from fertiliser can be as simple as applying fertiliser when runoff is unlikely for at least 21 days (Nash et al. Citation2003). A strategy to decrease P losses from the soil is to employ tillage redistributing and lowering enriched topsoil P throughout the plough layer (Sharpley Citation2003). McDowell et al. (Citation2014) tilled a near stream area, fertilised it with 10 kg P ha−1 in summer and sowed the area in a monoculture of ryegrass that would produce well at low soil Olsen P concentrations. This management combination decreased dissolved P losses by 40% at a catchment scale. However, to maintain pasture quality and minimise the use of costly fertiliser-N, it would be advantageous to include a P-efficient legume.
Past studies of P efficiency in legumes measured efficiency as the ability to acquire P from the soil and transform it into biomass (e.g. Pang et al. Citation2010; Maxwell et al. Citation2013). However, the P loss component of P efficiency requires us to also measure how much P is available for loss from shoots relative to soil. McDowell et al. (Citation2011) showed that the potential for loss from shoot material and soil is best measured as water extractable P (WEP). Therefore, the aim of this paper was to inform the concept of a P-efficient legume by assessing which legume would maintain a low WEP in shoot material and in the soil at different Olsen P concentrations, but more specifically at a low Olsen P of 15 mg kg−1 designed to maintain ryegrass yield (Gillingham et al. Citation2008) and minimise the potential for P loss from soil.
Materials and methods
The soil was a Waitahuna silt loam (New Zealand Classification: Mottled Fragic Pallic soil; USDA Taxonomy, Typic Hapludalf) taken from a 15-year-old grazed dairy farm in South Otago, New Zealand, 240 m above sea level and with a mean annual rainfall of 736 mm. Soil was sampled from a depth of 10–15 cm to ensure a low initial Olsen P concentration. The soil was air-dried, passed through a 2 mm sieve and 2 kg placed into pots (bulk density 0.75 g cm−3). To each pot was added CaSO4.2H2O and KCl at a rate of 0.5 g kg−1 soil, together with lime (2 Mg laboratory grade CaCO3 ha−1) to shift the pH from 5.6 to 5.8, and single superphosphate (0, 0.5, 1.0, 1.5, 2.0 or 2.5 g P kg−1 soil) calculated via Roberts & Morton (Citation1999) to alter initial soil Olsen P to approximately 10 (zero superphosphate addition), 20, 30, 40, 50 or 60 mg kg−1 for each pot. The soil from each pot was well mixed, watered with 1 L of tap water and allowed to dry for 15 days, before being watered (1 L) and allowed to dry (15 days) twice more to equilibrate.
Ten species (perennial ryegrass, Lolium perenne cv. Trojan; white clover, Trifolium repens cv. Huia; red clover, T. pratense cv. Hamua; lucerne, Medicago sativa cv. Wairau; crimson clover, T. incarnatum cv. Blaza; strawberry clover, T. fragiferum cv. Palestine; haresfoot clover, T. arvense cv. Increase; sheep's burnet, Sanguisorba minor; chicory, Cichorium intybus cv. Puna II; and plantain, Plantago lanceolata cv. Tonic) were sown at agronomically recommended rates (22 [Young et al. Citation2010], 8 [McDowell et al. Citation2011], 15, 15 [Moot et al. Citation2012], 15 [Smith et al. Citation2012], 10, 6 [Lonati et al. Citation2009], 10 [Wills et al. Citation1987], 5 [Moloney & Milne Citation1993] and 4 kg ha−1, respectively). Pots were laid out in a glasshouse (mean temperate 15 °C, range 12–27 °C during the experiment) in a randomised design of four replicates of 10 species, plus one fallow treatment, by six P treatments (264 pots). Two weeks post germination, 30 kg N ha−1 as NH4NO3 was applied to all pots in order to overcome any plant N deficiencies during seedling establishment. Pasture was watered every 2 days with deionised water, and every fortnight with a solution containing the equivalent of 500 g ha−1 of boron, cobalt, molybdenum and zinc, to maintain soils at a gravimetric water content of 40% v v−1. Pasture was harvested by cutting to 2 cm of the soil surface, and drying plant material at 70 °C and weighing dry matter (DM) yield. Harvests were conducted 8, 12, 17, 21, 26 and 30 weeks after sowing. Following the final harvest, soil from each pot was sampled (0–7.5 cm), air-dried and passed through a 2 mm sieve.
Shoot material was bulked across all harvests to make one sample per pot. The bulk sample was ground and analysed for WEP (McDowell et al. Citation2011). Three soil samples were taken per pot (0–7.5 cm depth), bulked and analysed for Olsen P (Olsen et al. Citation1954) and WEP (McDowell & Condron Citation2004).
Comparisons of mean concentrations were made via an analysis of variance of log-transformed data.
Results and discussion
Mean concentrations of soil WEP for haresfoot clover, lucerne, plantain and sheep's burnet were greater (24%–37%) across all P additions compared with the fallow soil (). All other species were similar to the fallow soil. Mean concentrations of soil Olsen P in ryegrass, sheep's burnet and white clover were greater (25%–31%) than in the fallow soil (). Differences from the fallow soil represent each species’ effect on potential P loss (WEP) and plant available P (Olsen P) when sown as a monoculture, i.e. avoiding any confounding effects of companion species when sown in a mixed sward.
Table 1. Mean soil water-extractable P (WEP) and Olsen P for each treatment.
In addition to considering variation in WEP across a range of soil Olsen P concentrations, we must consider the target Olsen P concentration of 15 mg kg−1. Due to a low anion storage capacity (ASC), the WEP of the Pallic at the target Olsen P is greater than other soil orders with a greater mean ASC (McDowell & Condron Citation2004), but probably targeted as low as possible without compromising yield. Fitting a power function (y = axb) to WEP concentrations in soil material yielded significant fits (P < 0.05) against soil Olsen P concentration for all species (A). Furthermore, both slopes (b = curvature index) and intercepts (initial state) were different among species (P = 0.009 and P < 0.001, respectively). At the Olsen P target, species differences in WEP soil concentrations from line fits were similar to those derived from means across the range of Olsen P concentrations (). Specifically, soil WEP decreased in the order of haresfoot clover > lucerne > plantain > crimson clover > chicory = sheep's burnet = red clover > strawberry clover > white clover > ryegrass. For simplicity, the first four species in this ranking were classified as ‘high’ loss, the next three species as ‘medium’ loss and the last three species as ‘low’ loss ().
Figure 1. Mean concentration of water extractable P (WEP) in soil (A) sampled at the end of the trial, and in shoot material (B) bulked across all harvests of different plant species grown across a range of soil Olsen P concentrations. Lines represent the fit (P < 0.05) of a power function (y = axb) to each species.
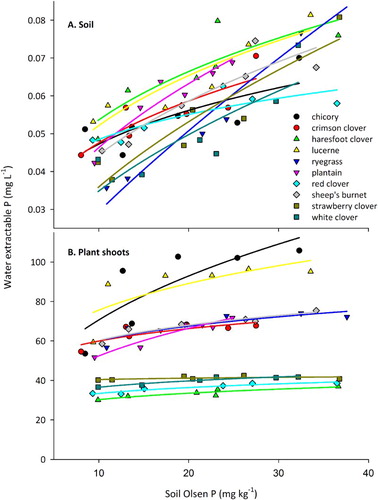
Table 2. Categorical relative weighting among species at an Olsen P of 15 mg kg−1 for potential for P loss from the soil and shoot material, and yield.
A power function was also fitted to shoot WEP and Olsen P concentrations yielding significant fits for all species (P < 0.01), and differences in slopes (P = 0.0.07) and intercepts (P < 0.001) between species. Shoot WEP could be grouped, at the target Olsen P concentration, into those of high (chicory, lucerne), medium (crimson clover, ryegrass, plantain and sheep's burnet) and low (haresfoot clover, red clover, strawberry clover and white clover) mean WEP concentration and P loss from shoots (B; ).
Those species that enrich soil WEP without sequestering it into biomass will have a greater likelihood of P loss than those that do not enrich soil WEP or maintain high WEP in shoot material. Dry matter yield at the target Olsen P concentration (15 mg kg−1) tended to be more variable than WEP concentrations at the target Olsen P (coefficient of variation of 30%, compared with 12% and 20% for WEP soil and shoots, respectively). Past research indicated that among potential sources, a soil P loss (estimated here as soil WEP) usually dominates annual P loss compared with losses from plant shoots, fertiliser or dung (McDowell et al. Citation2007). Among species with a medium to high soil WEP, those with a medium or high shoot WEP would therefore be unlikely choices for a low P loss system, unless yield (and hence P use efficiency; Pang et al. Citation2010) was much greater. This discounted chicory, crimson clover, lucerne, plantain and sheep's burnet. Of the remainder, white clover exhibited low WEP in soil and shoot material. Under the same soil conditions, yield was as good as any other species except red clover (). White clover's tolerance of cool conditions means it may be the best legume for our low-P system. However, the characteristics of other species may make them more suited in some areas, albeit with caveats. For instance: haresfoot clover is suited to semi-arid conditions, but as an annual is hard to re-establish and is likely restricted to upland areas (Boswell et al. Citation2006); red clover tends to be drought tolerant, but may not persist as long as other clovers (Ryan-Salter & Black Citation2012); and strawberry clover is a good choice for wet areas or soils with salinity issues (Moore et al. Citation2006). An additional caveat is that each species was grown as a monoculture to allow for full expression of its yield potential. Although there is no evidence to suggest WEP concentrations of the legumes would differ in a mixed grass-legume pasture, we cannot discount any effects caused by growth responses due to shading (from ryegrass) and grazing.
Conclusions
In measuring the potential for P loss via soil and plant shoot material, white clover was categorised as having low potential for P loss while exhibiting high yield relative to other species at a target Olsen P concentration of 15 mg kg−1. This target was commensurate with the establishment of an efficient dairy pasture able to produce good yields of high-quality forage (in monocultures of a selected legume and ryegrass) with low P inputs and little P losses to surface water. However, while the glasshouse trial showed white clover as the best fit for such a system, other legumes may be more suitable under different soil or climatic conditions or when used in a mixed sward.
Acknowledgements
P. Olyott, A. Rutherford and W. Worth assisted with the pot trial and N. Cox with the statistical analyses.
Disclosure statement
No potential conflict of interest was reported by the authors.
Additional information
Funding
References
- Boswell CC, Lucas RJ, Lonati M, Fletcher A, Moot DJ. 2006. The ecology of four annual clovers adventive in New Zealand grasslands. In: AGICOM: legumes for dryland pastures. New Zealand Grassland Association (Inc.) Symposium, 18 and 19 November 2003, Lincoln University. Grassland Research and Practice Series No. 11. Dunedin: New Zealand Grassland Association (Inc.): p. 175–184.
- Gillingham A, Morton J, Gray M. 2008. Pasture responses to phosphorus and nitrogen fertilisers on east coast hill country: 2. Clover and grass production from easy slopes. New Zeal J Agr Res. 51:85–97. doi: 10.1080/00288230809510438
- Hart MR, Quin BF, Nguyen ML. 2004. Phosphorus runoff from agricultural land and direct fertilizer effects: a review. J Environ Qual. 33:1954–1972. doi: 10.2134/jeq2004.1954
- Lonati M, Moot DJ, Aceto P, Cavallero A, Lucas RJ. 2009. Thermal time requirements for germination, emergence and seedling development of adventive legume and grass species. New Zeal J Agr Res. 52:17–29. doi: 10.1080/00288230909510485
- Maxwell TMR, Moir JL, Edwards GR. 2013. Phosphorus response and efficiency of four adventive annual clovers grown in a New Zealand high country soil under glasshouse conditions. New Zeal J Agr Res. 56:203–214. doi: 10.1080/00288233.2013.809775
- McDowell RW. 2006. Contaminant losses in overland flow from cattle, deer and sheep dung. Water Air Soil Pol. 174:211–222. doi: 10.1007/s11270-006-9098-x
- McDowell RW, Condron LM. 2004. Estimating phosphorus loss from New Zealand grassland soils. New Zeal J Agr Res. 47:137–145. doi: 10.1080/00288233.2004.9513581
- McDowell RW, Monaghan RM, Morton J. 2003a. Soil phosphorus concentrations to minimize potential P loss to surface waters in Southland. New Zeal J Agr Res. 46:239–253. doi: 10.1080/00288233.2003.9513550
- McDowell RW, Monaghan RM, Carey PL. 2003b. Phosphorus losses in overland flow from pastoral soils receiving long-term applications of either superphosphate or reactive phosphate rock. New Zeal J Agr Res. 46:329–337. doi: 10.1080/00288233.2003.9513561
- McDowell RW, Nash DM, Robertson F. 2007. Sources of phosphorus lost from a grazed pasture soil receiving simulated rainfall. J Environ Qual. 36:1281–1288. doi: 10.2134/jeq2006.0347
- McDowell RW, Sharpley AN, Crush JR, Simmons T. 2011. Phosphorus in pasture plants: potential implications for phosphorus loss in surface runoff. Plant Soil. 345:23–35. doi: 10.1007/s11104-010-0687-5
- McDowell RW, Cosgrove GP, Orchiston T, Chrystal J. 2014. A cost-effective management practice to decrease phosphorus loss from dairy farms. J Environ Qual. 43:2044–2052. doi: 10.2134/jeq2014.05.0214
- Moloney SC, Milne GD. 1993. Establishment and management of Grasslands Puna chicory used as a specialist high quality forage herb. Proc New Zeal Gr Assoc. 55:113–118.
- Moore G, Sanford P, Wiley T. 2006. Perennial pastures for Western Australia. Bulletin 4690. Perth, WA: Department of Agriculture and Food Western Australia.
- Moot DJ, Pollock KM, Lewis B. 2012. Plant population, yield and water use of Lucerne sown in autumn at four sowing rates. Proc New Zeal Gr Assoc. 74:97–102.
- Nash D, Hannah M, Clemow L, Webb B, Chapman D. 2003. A laboratory study of phosphorus mobilisation from commercial fertilisers. Aust J Soil Res. 41:1201–1212. doi: 10.1071/SR03024
- Olsen SR, Cole CV, Watanabe FS, Dean LA. 1954. Estimation of available phosphorus in soils by extraction with sodium bicarbonate. United States Department of Agriculture Circular No. 939. Washington, DC: United States Government Print Office.
- Pang J, Tibbett M, Denton MD, Lambers H, Siddique KMH, Bolland MDA, Revell CK, Ryan MH 2010. Variation in seedling growth of 11 perennial legumes in response to phosphorus supply. Plant Soil. 328:133–143. doi: 10.1007/s11104-009-0088-9
- Roberts AHC, Morton JD. 1999. Fertiliser use of New Zealand dairy farms. Auckland: New Zealand Fertiliser Manufacturers’ Association.
- Ryan-Salter TP, Black AD. 2012. Yield of Italian ryegrass with red clover and balansa clover. Proc New Zeal Gr Assoc. 74:201–208.
- Sharpley AN. 2003. Soil mixing to decrease surface stratification of phosphorus in manured soils. J Environ Qual. 32:1375–1384. doi: 10.2134/jeq2003.1375
- Smith VH, Joye SB, Howarth RW. 2006. Eutrophication of freshwater and marine ecosystems. Limnol Oceanogr. 51:351–355. doi: 10.4319/lo.2006.51.1_part_2.0351
- Smith SA, Popp M, Phillip D. 2012. Seedling survival and establishment costs: crimson and white clover in Bermudagrass pastures. Agron J. 106:1517–1522. doi: 10.2134/agronj2012.0117
- Wills BJ, Sheppard JS, Begg JSC. 1987. Evaluation of alternative dryland pasture plants and browse shrubs for soil conservation in drought-prince Otago grasslands. Proc New Zeal Gr Assoc. 48:115–118.
- Young SD, Townsend RJ, Swaminathan J, O'Callaghan M. 2010. Serratia entomophila-coated seed to improve ryegrass establishment in the presence of grass grubs. New Zeal Plant Protect. 63:229–234.