ABSTRACT
Cadmium (Cd) losses from acidic organic soils amended with phosphate fertiliser are potentially important for the assessment of Cd accumulation, but are currently poorly understood. This study investigated the effect of soil pH and single superphosphate (SSP) fertiliser at application rates of 0, 50, 100 and 200 kg P ha−1 on Cd leaching and plant uptake from an organic soil in a lysimeter study. Average leaching losses ranged from 0.14–0.39 g Cd ha−1. The greatest leaching losses were from soils at pH 4.5 at the highest rates of P application (100 and 200 kg P ha−1). In comparison, there was no significant difference in the amounts of Cd lost at pH 5.5 or 6.5 at any rate of P application. Losses equated to <1% of the Cd added to the soil from an application of 200 kg P ha−1. Average ryegrass Cd concentrations ranged from 0.03–0.09 mg kg−1 dry weight. Soil pH had no significant effect on concentrations for the two lowest SSP application rates, whereas at the two high SSP treatments, Cd concentrations increased between pH 4.5 and 5.5, but decreased between pH 5.5 and 6.5. Cadmium losses from both leaching and plant uptake are low in this organic soil, highlighting the important role that organic matter and pH provide in retaining Cd.
Introduction
Cadmium (Cd) is a biotoxic heavy metal and an impurity in many phosphorus (P) containing fertilisers (Gray et al. Citation1999a). In New Zealand (Rys Citation2011), as well as internationally (Six & Smolders Citation2014; Zhao et al. Citation2015), there is concern regarding the historic and ongoing accumulation of Cd in agricultural soils because of its potential toxicity to humans and other living organisms. To address these concerns in New Zealand, in 2011 a National Cadmium Working Group released a National Cadmium Management Strategy (MAF Citation2011) based on an assessment of the risk Cd poses to agricultural systems. This strategy outlines a risk-based approach for managing Cd in agricultural soils, based on a Tiered Fertiliser Management System (TFMS). This framework is intended to allow landowners to self-manage Cd accumulation in soils with time. It contains five tiers and four trigger concentrations, whereby as soil Cd concentrations increase, increasingly stringent fertiliser management practices are imposed.
One of the recommended management actions in the TFMS when soil Cd concentrations exceed tier two (>1.0 mg kg−1) is the use of a ‘Cd balance programme’ to help ensure that soil Cd concentrations do not exceed an acceptable threshold within the next 50 years. Currently, the only available Cd balance programme calibrated for use in New Zealand is CadBal, a Cd balance model developed for the Fertiliser Association of New Zealand (FANZ) by Roberts & Longhurst (Citation1996) and updated in 2005. CadBal is essentially a mass-balance model that predicts Cd accumulation or depletion in soils over time with Cd inputs from atmospheric deposition, fertiliser application and biosolids application and Cd losses such as crop offtake, leaching and soil erosion. However, a recent review of CadBal highlighted limited data on Cd leaching losses for many important soil orders in New Zealand (Cavanagh Citation2014). The data set used in CadBal is that of Gray et al. (Citation2003), which only quantifies Cd leaching losses from four (i.e. Pumice, Allophanic, Brown and Pallic) of the 15 soil orders in New Zealand and only from sites representing permanent pasture amended with inorganic fertiliser.
One soil order for which there is currently no Cd leaching losses data from New Zealand or relevant international studies is organic soils. In New Zealand, organic soils cover about 258,748 ha, of which 186,165 ha is in agricultural production (Dresser et al. Citation2012). When developed, they require drainage, significant inputs of lime to increase pH, and P fertiliser to improve fertility (O'Connor et al. Citation2001). Depending on the type of P and the rate at which it is being applied, fertiliser can also add significant amounts of Cd to soils (Gray et al. Citation1999a). While previous studies have shown that Cd added to organic soils is readily sorbed, a significant proportion of this Cd has also been shown to be able to be desorbed back into soil solution, especially at low soil pH (Gray et al. Citation1998) and therefore may be available to be leached.
Because organic soils have a high porosity and hydraulic conductivity, it is unclear whether the Cd being added in P fertiliser to acidic soils when they are being developed can be leached, and if so, how much can be lost. This is important information if we want to know how long it would take to decrease Cd enrichment in soil and to calibrate a model such as CadBal. Further, while capital amounts of lime are required to be applied during the development of acid organic soils, it is also unclear if the increase in soil pH to 5.0–5.5 as recommended for optimal pasture production in organic soils (Roberts & Morton Citation2009) is sufficient to minimise plant uptake of Cd.
The primary aim of this study was therefore to quantify the amount of Cd lost via leaching from an organic soil amended with different rates of a soluble P fertiliser at different soil pH values typical of conditions for these soils as they are developed. And secondly to assess what effect soil pH had on Cd uptake in ryegrass grown in organic soils.
Materials and methods
Soil
An Invercargill peat (Acid Mesic organic soil in the New Zealand Soil Classification) (Hewitt Citation2010) was sampled between 300–600 mm depth (to minimise the contribution of any previous fertiliser applications) from a pastoral soil that had been developed and in production for 1 year under a forage crop of swede (Brassica napobrassica sp.). A summary of soil properties is given in .
Table 1. Selected properties of the soil used in lysimeters and fertiliser total Cd concentration.
Lysimeter set-up and treatments
Soil was air-dried, crushed and passed through an 8 mm sieve before being packed into lysimeters at 5 cm intervals to a bulk density of 0.31 g cm3, equivalent to that displayed in the field (Simmonds et al. Citation2015). Lysimeters were 213 mm in diameter and 300 mm deep, with a nylon mesh (1000 µm) covering a 5 mm hole drilled in the bottom to prevent soil loss, but allowing the collection of drainage via a tube into a vented container. As lysimeters received regular irrigation, edge flow and drying effects were considered to be negligible (Cameron et al. Citation1992).
Treatments included three soil pH levels—i.e. pH 4.5, 5.5 and 6.5—and four rates of single superphosphate (SSP) fertiliser—i.e. 0, 50, 100 and 200 kg P ha−1—each replicated four times. The target pH was achieved by adding both laboratory grade Ca(OH)2 and CaCO3 (hereafter referred to as lime) to ensure a rapid pH change, using the method outlined in Lierop (Citation1983). Lime was mixed throughout the soil in the lysimeters, followed by a 6 week equilibration period with three wetting and drying cycles. Single superphosphate was sieved (<2 mm) and applied to the surface of each lysimeter. The rates of P were nil and equivalent to either a maintenance P application rate (50 kg P ha−1) or capital applications (100 and 200 kg P ha−1), increasing total soil Cd concentrations from 0.08 mg kg−1 in the nil treatment, to 0.13, 0.17 and 0.24 mg kg−1 in the three fertiliser treatments, respectively. Lysimeters were placed in a light- and temperature-controlled greenhouse and arranged in a randomised block design.
After the treatments were applied, lysimeters were watered and perennial ryegrass (Lolium perenne) was sown at a rate of 22 kg ha−1 (Young et al. Citation2010) and watered daily to retain moisture until germination without causing leaching. Three weeks after germination, 40 mm of tap water was applied to lysimeters over a 72 h period (13.3 mm day−1) using a commercial garden sprinkler system (2 mm full circle micro jet spray fittings) to trigger leaching across all lysimeters, and ryegrass was harvested to a dry matter (DM) residual of 1500 kg ha−1. Irrigation was then applied as three, automated 2 min daily rainfall cycles over the 12 month duration of the experiment, 1025 mm applied in total. Leachate was collected every 3 days when there was sufficient drainage and ryegrass harvested at monthly intervals.
Analysis
Soil and fertiliser
Soil was oven-dried and passed through a 2 mm sieve prior to chemical analysis. Soil pH was measured in a deionised water suspension with soil:solution ratio 1:10 (Hendershot et al. Citation1993). Olsen P was determined by bicarbonate extraction (Olsen et al. Citation1954). Total carbon (C) was measured by combustion (Metson et al. Citation1979). Cation exchange capacity (CEC) was determined by ammonium acetate extraction followed by analysis of cations by inductively coupled plasma–optical emission spectrometry (ICP-OES). Amorphous iron (Fe) and aluminium (Al) oxides were determined by oxalate-extraction (McKeague & Day Citation1966). Total Cd was determined by nitric acid/hydrochloric digestion (US EPA Method Citation2002) followed by analysis by inductively coupled plasma–mass spectrometry (ICP-MS).
Phosphate fertiliser was dried and passed through a 2 mm sieve prior to analysis of total Cd determined by nitric acid/hydrochloric digestion followed analysis by ICP-OES.
Leachate analysis
The volume of leachate from each drainage event was measured and subsamples were filtered through a 0.45 µm polyvinylidene fluoride (PVDF) filter membrane before Cd analysis of composite samples at the end of the experiment. Cadmium was measured by ICP-MS. The limit of detection in drainage was 0.05 µg Cd L−1. Cadmium loads (g ha−1) were determined by combining concentration data with leachate volumes and data reported on hectare basis using the surface area of the lysimeter.
Plant analysis
Plant matter was dried at 60 °C and weighed to determine the cumulative DM yield over the 12 month trial. Plant samples were digested in nitric acid/hydrogen peroxide digestion followed by Cd analysis by ICP-MS.
Statistical analysis
Statistical analyses were performed by GENSTAT for Windows v16. All data were checked for normality before being subjected to a two-way Analysis of Variance (ANOVA) to test for the effects of pH and rate of P application. Effects were considered significant if they differed at the probability level of 5% based on Fisher's unprotected least significant difference (LSD) test.
Results and discussion
Total Cd leached
The average Cd load leached from each treatment is given in . On average, the greatest amounts of Cd were lost from soil at pH 4.5 at the two highest rates of P application (100 and 200 kg P ha), i.e. 0.35 g ha−1 and 0.39 g ha−1, respectively. There was no significant difference in the amounts of Cd lost at pH 5.5 or 6.5 at any of the rates of P application.
Figure 1. Effect of soil pH and rate of P application on mean Cd leaching loads (g ha−1). Mean (n = 4) and standard error followed by the same letter are not significantly different (P < 0.05).
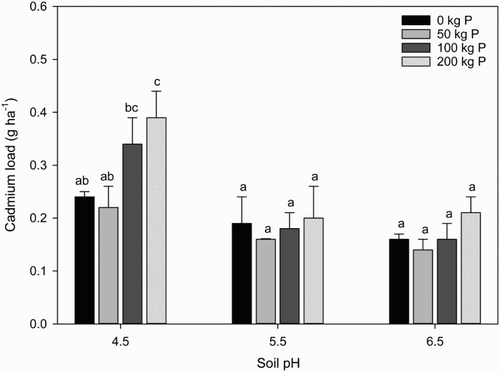
There are very few studies that have determined Cd leaching in New Zealand or worldwide and almost none looking at losses from organic soils. Gray et al. (Citation2003) measured Cd losses from four soil orders (i.e. Pumice, Allophanic, Brown and Pallic) that had received Cd from P fertiliser. Leaching losses ranged from 0.27 g ha−1 from a Pallic soil to 0.86 g ha−1 from an Allophanic soil. McLaren et al. (Citation2004) measured Cd losses 0.8 and 3.3 g ha−1 in the control treatments of a Brown and Allophanic pasture soil, respectively. While more recently, Carrick et al. (Citation2014) measured Cd losses of 0.5–0.8 g ha−1 from a stony Recent soil after the application of 95 kg P. On average, Cd losses measured from the organic soil in this study are at the lower end of the range compared with other soil orders. In part, this is a reflection of the lower soil Cd status of the soil used in this study (i.e. <0.24 mg kg−1), which is less than other reported Cd leaching studies (Gray et al. Citation2003).
In addition to being low compared with other soils, the leaching losses were low compared with Cd added in fertiliser. The total Cd concentration of the fertiliser was 282 mg Cd kg−1 P (), resulting in 0, 14, 28 or 56 g Cd being applied at the 0, 50, 100 and 200 kg P ha−1 yr−1 treatments, respectively. Using the 200 kg P and pH 4.5 treatment as an example, and ignoring any potential native Cd losses, <1% of the Cd added from fertiliser was leached from this soil. This compares with Cd losses of 5%–7% of that added in fertiliser to a Recent soil (Carrick et al. Citation2014) and 5%–15% reported for four other soil orders (Gray et al. Citation2003). It appears that this organic soil, even at low pH, has a very high affinity to retain added Cd.
The importance of organic matter and soil pH for Cd sorption has previously been documented (Gray et al. Citation1998). More Cd is sorbed to organic matter as soil pH increases (McBride et al. Citation1997). For example, Mann & Ritchie (Citation1993) showed that as pH in a peaty sand increased from 4.5 to 6.5, Cd associated with organic matter increased from 35% to 55%. Various models have been put forward to describe this sorption phenomenon. organic matter has a high negative charge density, arising mainly from the dissociation of carboxyl and phenolic groups. The net charge on soil colloids becomes increasingly negative as soil pH increases, increasing sorption (Naidu et al. Citation1994).
Ryegrass production and Cd uptake
Soil pH had a significant effect on ryegrass DM production (). Low yields were found at pH 4.5, which is well below the recommended optimal pH range of 5.0–5.5 for pasture production for organic soils in New Zealand (Roberts & Morton Citation2009). There was a small increase in production from pH 5.5 to 6.5.
Table 2. Cumulative mean ryegrass yield (g DM pot−1) for each treatment.
Average ryegrass Cd concentrations ranged from 0.03–0.09 mg kg−1 DW (). The highest concentration was found in the high P treatment at pH 5.5, which is in line with concentrations reported in other New Zealand studies, although overall Cd concentrations were low. For example, Reiser et al. (Citation2014) reported a mean Cd concentration of 0.13 mg kg−1 DW in a survey of ryegrass/clover dominated mixed pastures, while Roberts et al. (Citation1994) reported a mean Cd concentration of 0.10 mg kg−1 DW in the grass component of pasture. In a glasshouse trial investigating Cd uptake in ryegrass grown in 10 different soils, Cd concentrations ranging between 0.07 and 0.30 mg kg−1 DW have been reported (Gray et al. Citation1999b). While there are currently no New Zealand limits on Cd in forages for animal production, the concentrations are well within the EU Animal Feed Directive (European Union Citation2005) that specifies a maximum of 1 mg Cd kg−1 of animal feed derived from vegetable origin (based on a moisture content of 12%). Based on this limit, Cd concentrations in ryegrass grown on this organic soil are low.
Figure 2. Effect of soil pH and rate of P application on ryegrass Cd concentration (mg kg−1) in DM. Mean (n = 4) and standard error followed by the same letter are not significantly different (P < 0.05).
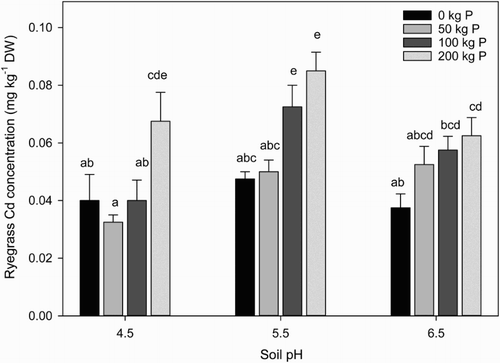
Soil pH had no significant effect on ryegrass Cd concentrations for the two lowest P treatments (0 and 50 kg ha−1). However, there was a significant increase in ryegrass Cd concentration between pH 4.5 and 5.5 in the 100 kg P ha−1 treatment, and a decrease between 5.5 and 6.5. The same decrease in Cd concentration was noted for the pH 6.5 compared with pH 5.5 treatment at 200 kg P ha−1, but no change was noted between pH 4.5 and 5.5.
The change in plant Cd concentrations from pH 5.5 to 6.5 reflects decreasing availability in the soil solution due to increasing soil sorption with pH (Loganathan et al. Citation2012). In contrast, the increase in ryegrass Cd concentration between pH 4.5 and 5.5 is likely to be due to increased nutrient availability and decreased toxicity (e.g. Al) resulting in better plant health and yield (). The exception being the high P treatment at pH 4.5, where there was a high ryegrass Cd concentration. Although because of the significantly lower yields found at pH 4.5, total Cd uptake in all P treatments was significantly lower than at pH 5.5 or 6.5 (data not shown). Wang et al. (Citation2006) similarly found significantly lower plant Cd concentrations and total Cd uptake at pH 4.7 than at pH 6.0, reported to be due to poor plant growth at pH 4.7 from Al and Mn toxicity that impaired Cd uptake.
Despite Cd losses in this study being low, as organic soils are developed for agriculture, activities such as drainage and cultivation will decrease carbon concentrations as peat is oxidised to carbon dioxide (Schipper & McLeod Citation2002). Therefore, as the organic matter concentrations decrease, it will be important to maintain low Cd concentrations in fertiliser so that the soil is not being enriched with Cd. Even if some enrichment does occur, the very high concentration of organic matter in organic soils means there is good potential to maintain Cd in the soil and not in the soil solution where it can be taken up by plant roots. Furthermore, some protection of Cd loss is also afforded by the fact that at pH 4.5 plant growth was inhibited to the extent that large capital inputs of P fertiliser (and Cd) are unlikely to occur, hence Cd loss is limited.
Conclusions
The findings of this study demonstrated that increasing amounts of Cd can be lost in drainage or taken up by plants from the application of P fertiliser. However, soil pH affects the amount of Cd lost and overall losses are low compared with the amount of Cd added in fertiliser. This is likely to be a result of the large effect that soil organic matter and, to a lesser extent, soil pH have on Cd sorption in organic soils. Therefore, by ensuring that soil pH is maintained at 5.5 or above, Cd leaching loss and plant uptake will be low from organic soils.
Disclosure statement
No potential conflict of interest was reported by the authors.
Additional information
Funding
References
- Cameron K, Smith N, McLay C, Fraser P, McPherson R, Harrison D, Harbottle P. 1992. Lysimeters without edge flow: an improved design and sampling procedure. Soil Sci Soc Am J. 56:1625–1628. doi: 10.2136/sssaj1992.03615995005600050048x
- Carrick S, Cavanagh J, Scott J, McLeod M. 2014. Understanding phosphorus, nitrogen and cadmium transfer through a young stony soil. In: Currie LD, Christensen CL, editors. Nutrient management for the farm, catchment and community. Occasional Report No. 27. Palmerston North: Fertilizer and Lime Research Centre, Massey University; [cited 2015 Jan 20]. Available from: http://flrc.massey.ac.nz/publications.html
- Cavanagh J. 2014. Review of models to predict cadmium accumulation in agricultural soils. Lincoln, New Zealand: Landcare Research. LC 1820. 20 p.
- European Union. 2005. Commission directive 2005/87/EC of 5 December 2005 amending Annex 1 to Directive 2002/32 EC of the European parliament and of the Council on undesirable substances in animal feed as regards lead, fluorine and cadmium. Off J Eur Union. L 318:19–24.
- Dresser M, Hewitt A, Willoughby J, Belliss S. 2012. Area of organic soils. MAF Technical Paper No: 2012/14. Wellington: Ministry of Agriculture and Forestry.
- Gray CW, McLaren RG, Roberts AHC. 2003. Cadmium leaching from some New Zealand pasture soils. Eur J Soil Sci. 54:159–166. doi: 10.1046/j.1365-2389.2003.00495.x
- Gray CW, McLaren RG, Roberts AHC, Condron LM. 1998. Sorption and desorption of cadmium from some New Zealand soils: effect of pH and contact time. Aust J Soil Res. 36:199–216. doi: 10.1071/S97085
- Gray CW, McLaren RG, Roberts AHC, Condron LM. 1999a. The effect of long-term phosphatic fertiliser applications on the amounts and forms of cadmium in soils under pasture in New Zealand. Nutr Cycl Agroecosys. 54:267–277. doi: 10.1023/A:1009883010490
- Gray CW, McLaren RG, Roberts AHC, Condron LM. 1999b. Cadmium phytoavailability in some New Zealand soils. Aust J Soil Res. 37:461–477. doi: 10.1071/S98070
- Hendershot WH, Lalande H, Duquette M. 1993. Soil reaction and exchangeable acidity. In: Carter MR, editor. Soil sampling and methods of analysis. Baton Raton, FL: CRC Press/Lewis Publishers; p. 141–146.
- Hewitt AE. 2010. New Zealand soil classification. 3rd ed. Lincoln: Manaaki Whenua Press, Landcare Research.
- Lierop WV 1983. Lime requirement determination of acid organic soils using buffer-pH methods. Can J Soil Sci. 63:411–423. doi: 10.4141/cjss83-043
- Loganathan P, Vigneswaran S, Kandasamy J, Naidu R. 2012. Cadmium sorption and desorption in soils: a review. Crit Rev Env Sci Tec. 42:489–533. doi: 10.1080/10643389.2010.520234
- MAF. 2011. Cadmium and New Zealand agriculture and horticulture: a strategy for long term risk management. Wellington: Ministry of Agriculture and Forestry.
- Mann SS, Ritchie GSP. 1993. The influence of pH on the forms of cadmium in four West Australian soils. Aust J Soil Res. 31:255–270. doi: 10.1071/SR9930255
- McBride M, Sauve S, Hendershot W. 1997. Solubility control of Cu, Zn, Cd and Pb in contaminated soils. Eur J Soil Sci. 48:337–346. doi: 10.1111/j.1365-2389.1997.tb00554.x
- McKeague JA, Day JH. 1966. Dithionite- and oxalate-extractable Fe and Al as aids in differentiating various classes of soils. Can J Soil Sci. 46:13–22. doi: 10.4141/cjss66-003
- McLaren RG, Clucas LM, Taylor MD, Hendry T. 2004. Leaching of macronutrients and metals from undisturbed soils treated with metal-spiked sewage sludge. 2. Leaching of metals. Aust J Soil Res. 42:459–471. doi: 10.1071/SR03168
- Metson AJ, Blakemore LC, Rhoades DA. 1979. Methods for the determination of soil organic carbon: a review, and application to New Zealand soils. New Zeal J Sci. 22:205–228.
- Naidu R, Bolan NS, Kookana RS, Tiller KG. 1994. Ionic-strength and pH effects on the sorption of cadmium and the surface charge of soils. Eur J Soil Sci. 45:419–429. doi: 10.1111/j.1365-2389.1994.tb00527.x
- O'Connor MB, Longhurst RD, Johnston TJM, Portegys FN. 2001. Fertiliser requirements for peat soils in the Waikato region. Proc New Zeal Grassland Assoc. 63:47–51.
- Olsen SR, Cole CV, Watanabe FS, Dean LA. 1954. Estimation of available phosphorus in soils by extraction with sodium bicarbonate. USDA Circular 939. Washington, DC: US Government Printing Office.
- Reiser R, Simmler M, Portmann D, Clucas L, Schulin R, Robinson B. 2014. Cadmium concentrations in New Zealand pastures: relationships to soil and climate variables. J Environ Qual. 43:917–925. doi: 10.2134/jeq2013.09.0367
- Roberts AHC, Longhurst RD. 1996. A cadmium balance model for New Zealand pastoral and horticultural systems. Report to the New Zealand Fertiliser Manufacturers Research Association. Wellington: Ministry of Agriculture and Forestry.
- Roberts AHC, Longhurst RD, Brown MW. 1994. Cadmium status of soils, plants and grazing animals in New Zealand. New Zeal J Agr Res. 37:119–129. doi: 10.1080/00288233.1994.9513048
- Roberts AHC, Morton JD. 2009. Fertiliser use on dairy farms: the principles and practice of soil fertility and fertiliser use on New Zealand dairy farms. Auckland: New Zealand Fertiliser Manufacturers’ Association.
- Rys GJ. 2011. A national cadmium management strategy for New Zealand agriculture. In: Currie LD, Christensen CL, editors. Adding to the knowledge base for the nutrient manager. Occasional Report No. 24. Palmerston North: Fertilizer and Lime Research Centre, Massey University; [cited 2016 Feb 16]. Available from: http://flrc.massey.ac.nz/workshops/11/paperlist11.htm
- Schipper LA, McLeod M. 2002. Subsidence rates and carbon loss in peat soils following conversion to pasture in the Waikato Region, New Zealand. Soil Use Manage. 18:91–93. doi: 10.1111/j.1475-2743.2002.tb00225.x
- Simmonds BM, McDowell RW, Condron LM, Jowett T. 2015. Potential phosphorus losses from organic and podzol soils: prediction and the influence of soil physico-chemical properties and management. New Zeal J Agr Res. 58:170–180. doi: 10.1080/00288233.2014.988830
- Six L, Smolders E. 2014. Future trends in soil cadmium concentration under current cadmium fluxes to European agricultural soils. Sci Total Environ. 485–486:319–328. doi: 10.1016/j.scitotenv.2014.03.109
- US EPA Method. 2002. Sample preparation procedure for spectrochemical determination of total recoverable elements. Cincinnati, OH: National Exposure Research Laboratory, Office of Water, US EPA, October 1999.
- Wang AS, Angle SJ, Chaney RL, Delorme TA, Reeves RD. 2006. Soil pH effects on uptake of Cd and Zn by Thlaspi caerulescens. Plant Soil. 281:325–337. doi: 10.1007/s11104-005-4642-9
- Young S, Townsend R, Swaminathan J, O'Callaghan M. 2010. Serratia entomophila-coated seed to improve ryegrass establishment in the presence of grass grubs. New Zeal Plant Protec. 63:229–234.
- Zhao F, Ma Y, Zhu Y, Tang Z, McGrath SP. 2015. Soil contamination in China: current status and mitigation strategies. Environ Sci Technol. 49:750–759. doi: 10.1021/es5047099