ABSTRACT
A grazed trial established in 1980 evaluated the effects of superphosphate fertiliser application on pasture growth on Easy (10°–20° slope) and Steep (30°–40° slope) land in the Waikato, North Island, New Zealand. Continuous and rotational grazing showed no effects on pasture responses to fertiliser. Mean annual pasture dry matter (DM) production ranged from 11,275 to 14,155 kg DM ha−1 on Easy, and 6413 to 7480 kg DM ha−1 on Steep slopes through the range of 10–100 kg ha−1 yr−1 of phosphate (P) fertiliser rates. Initial fertiliser effects on Olsen P tests were in the 0–3 cm depth only, and in the 7–15 cm soil depth only in the 100 kg ha−1 yr−1 treatment by year 4. Near maximum (97%) annual production, derived from relative yield analysis, was obtained at 0–7 cm depth soil tests of 30 on Easy slopes and at 12 on Steep slopes. Results from this trial are directly relevant to the variable fertiliser rate aerial application technology currently being developed.
Introduction
During the 1975–1985 period in particular, New Zealand North Island hill country was evaluated with respect to potential production achievable from sheep and beef farming (Brougham Citation1976; Hight Citation1979; Scott Citation1983). The estimates of up to 100% increase in production were based both on the example of the best hill country farmers, as well as the researched effects of fertiliser, subdivision and associated improved pasture utilisation, and legume introduction on production (Suckling Citation1959, Citation1975; O’Connor et al. Citation1973). The importance of applying appropriate grazing management to allow full expression of species potential was also emphasised (Bircham Citation1976; Smith & Dawson Citation1976; Lambert et al. Citation1983).
The bulk of the above research information was obtained from hill country that receives generally reliable summer rainfall, i.e. King Country and north-east Manawatu. No similar information on management and fertiliser effects was available for seasonally dry hill country especially on steep slopes. More recently, studies of the effects of phosphorus (P) and nitrogen (N) fertilisers on dry hill country have shown in situations of low pasture legume content that the pasture responded more to N than P fertiliser (Gillingham et al. Citation1998), especially in early spring and especially on steep slopes (Luscombe Citation1981; Ledgard et al. Citation1983; Gillingham et al. Citation2008).
Measurements of the effects of slope and aspect (Gillingham Citation1973; Gillingham & During Citation1973; Gillingham et al. Citation1980a)—and, later, grazing management (Sheath & Boom Citation1985)—on seasonal pasture production had been made in the northern North Island hill country which is seasonally dry. There had been, however, no evaluation of the effect of contrasting grazing systems on hill pasture response to increasing fertiliser rates. In response to this in particular, but also to the generally recognised need for more detailed assessment of pasture responses to fertiliser application in hill country (NRAC Citation1980), a large-scale trial was established at the Ministry of Agriculture and Fisheries Whatawhata Hill Country Research Station in autumn 1980. This trial was designed to provide practical recommendations for phosphate fertiliser application as well as a foundation for later detailed phosphate cycling studies (Rowarth & Gillingham Citation1990).
Methods
Trial site
The trial site was at Whatawhata Hill Country Research Station, latitude 37°48′S, altitude 200 m, 22 km west of Hamilton in the North Island of New Zealand. The total area was 21.4 ha with a general west-north-west aspect and contained slopes ranging from flat to very steep (i.e. 40°+). The soil was predominantly a northern yellow-brown earth with some recent volcanic ash on most gentle slopes (Bruce Citation1976), i.e. allophanic brown soils, now classified as a brown soil in New Zealand (Hewitt Citation2010) or distrochrepts in the USDA classification (Soil Survey Staff Citation1999). The land was only partly developed in 1968 when purchased, received 500 kg superphosphate ha−1 in 1974 followed by 375 kg superphosphate ha−1 yr−1 from 1975–1979.
The trial area was divided into 25 paddocks such that each contained some slopes ranging from flat to greater than 30°. Because of the variable topography this resulted in paddocks of varying size (range 0.243–1.223 ha).
A summary of the Whatawhata Station long-term climate records, as well as those for the trial period, are shown in .
Table 1. Long-term mean (LTM) and annual climate records from the Whatawhata Hill Country Research Station meteorological site approximately 1 km from the trial site.
Trial management
Fertiliser treatment application
Superphosphate fertiliser (total phosphorus (TP) range of 9.0%–9.8%) was applied annually for 4 years in March or April by helicopter at treatment rates of either 10, 20, 30, 50 or 100 kg total P ha−1 to each of four randomly located replicate paddocks, i.e. to a total of 20 paddocks. Each of the remaining five paddocks was also fertilised at one of the five different treatment rates and used as pre-conditioning areas for stock being transferred on to the trial, as indicated below. In year 3 (1982–1983), P was applied as potassic superphosphate (TP 7.4%; K 8.2%).
From year 5, the trial examined the residual effects of the previous P rate treatments. These results have been reported previously (Gillingham et al. Citation1990).
Grazing management
Contrasting grazing management treatments were included in order to evaluate if there were any effects on the pasture response to increasing rates of P fertiliser. Two paddocks at each fertiliser rate were grazed on a continual basis (CG) and the remaining two rotationally grazed (RG) by Romney-cross ewes or wethers. The CG paddocks had sheep numbers adjusted as necessary during the year to maintain a standing pasture height of about 2–3 cm. Rotational grazing was by five mobs of about 200 ewes or wethers placed in pre-conditioning paddocks for 2 days to minimise the effects of differing pasture P content on associated dung P return during subsequent grazing of the trial, before being moved on to the respective phosphate rate treatment. Grazing of the first replicate usually took 2–3 days before each mob was moved on to graze the second replicate, and then subsequently removed from the trial area. Post-grazing pasture height was about 2–3 cm.
During early spring each year (i.e. September–October), the total trial was continuously grazed. This change was for two reasons. First, most hill country farms are continuously grazed at this time after lambing. Second, because of the relatively high pasture growth rates at this time of year, insufficient stock was available for adequate pasture control by rotational grazing.
In each paddock, five fixed transects were subjectively located within each of Easy (10°–20° slope) and Steep (30°–40° slope) strata to represent the range of topography present. Each ‘transect’ consisted of five grouped, pegged locations, each peg indicating a surrounding measurement area of about 1–2 m radius as defined by slope. These 25 measurement sites per strata per paddock were used for all subsequent pasture and soil measurements.
Pasture production measurement
In the RG paddocks from May 1980 to May 1983, pasture production was measured by a double sampling ‘difference’ technique incorporating a visual estimate of standing dry matter (DM) just after (post) and just before (pre) each grazing. Visual ratings of the estimated standing pasture mass within 0.18 m2 quadrats were made on all 25 measurement sites per strata per paddock. These were calibrated against a total of 10 similar quadrats per strata, rated and then the pasture was cut to ground level using a shearing hand-piece, washed before drying at 80 °C overnight and weighed. The regression relationship between visual and measured pasture dry matter was used to derive mean standing DM levels (kg ha−1) per strata in each paddock both before and after grazing.
In CG paddocks, the visual rating technique was used to derive the mean level of standing dry matter per transect and a separate regression for the CG pastures derived with measured samples. A small (0.2 m2) exclusion cage was then placed on such a representative site and the regrowth within the cage subsequently rated at the next measurement date. There were, therefore, only five assessments of pasture regrowth per strata in each of the CG pastures, compared with 25 in the RG areas.
From May 1983 to May 1984, all pasture growth measurements were made by a trim technique (Lynch Citation1966). This both standardised the measurement technique for rotationally and continuously grazed paddocks and reduced the workload in rotationally grazed paddocks. After each grazing in the rotation treatments, and at the same time in the CG paddocks, the mean standing pasture DM level per transect was visually assessed as previously. A site representing the mean value was selected, trimmed to about 2 cm height, or just below the grazing level, and a 0.2 m2 exclusion cage placed on the site. At the next measurement date, the regrowth pasture was harvested at the same cutting height and the cage repositioned.
The pasture regrowth interval varied from about 4 to 6 weeks (i.e. nine harvests per year; eight in 1983–1984 year when only a single winter harvest was made). Pasture growth results are expressed in seasonal (winter: June–August; spring: September–November; summer: December–February; autumn: March–May) and annual totals. The reported trial period is from June 1980 to May 1984. Grazing treatments commenced in April 1980.
Pasture species composition
In September each year, when all paddocks were under CG, pasture on fixed line transects was visually assessed. Paddocks contained between nine and 20 transects each, depending on their total area. At 0.5 m intervals along each 40 m transect, pasture was observed through a 5 mm diameter ring held at 5 cm above the ground surface and the species present recorded. The number of positive sightings of each species was expressed as a percentage of the total number of recording sites. Results do not therefore indicate % species composition but rather the relative presence of each species. This is referred to as % frequency of occurrence technique (G Sheath, AgResearch, pers. comm. 1980).
Pasture P content
All pre-grazed pasture samples in RG paddocks, and regrowth pasture in cages in CG paddocks were analysed for P%.
Soil analysis
At each measurement site per strata per paddock, soil was sampled at 0–3 cm, 3–7 cm and 7–15 cm depths in February–March each year. This totalled 600 samples per year. Soil was subjected to QuickTest (pH, P, calcium [Ca], potassium [K] and magnesium [Mg]) annually, water soluble P (1981 and 1983) and anion storage capacity % (ASC%) and Olsen P analysis (in February 1981) and soil moisture content at intervals during the 1983–1984 year.
The above Quick Test range in the 1980s did not include soil sulphate analysis. Therefore, archived samples from 1983 were bulked to provide a maximum of four replicates of each P rate × strata × soil depth and analysed in October 2015.
Pasture production–soil P relationships
Results from the 4 year trial were used to derive pasture production–soil P relationships for winter, spring, summer and autumn yield, as well as annual production, with soil P measured at 0–3 cm depth and a derived value for 0–7 cm depth measured at the end of each production year.
Results
Climate summary
The annual rainfall for the 1980–1981 and 1981–1982 trial years (from May to the following April) was similar to that of the long-term mean (LTM) of 1593 mm (). In the subsequent 2 years, the trial year rainfall was lower, especially in 1982–1983, at only 1203 mm. Associated monthly maximum and minimum rainfalls in 1982–1983 were the lowest of any year, and sunshine hours were the highest. The coolest 12 month period was 1983–1984 when the mean air temperature was only 12.5 °C. Sunshine hours, however, were similar to that for the LTM.
Effect of grazing treatment
There were no significant effects of grazing management on the response of pasture to increasing rates of P fertiliser on either Easy or Steep slopes. Some differences in pasture production were measured between RG and CG grazed paddocks but these could have been partly an artefact of the differences in the pasture production measurement techniques used. These results will not be discussed further here. However, there were some interesting production dynamics in switching from RG to CG to RG again in spring each year.
From early to mid-spring (September–October) each year, the RG pastures were continuously grazed. So during this period the total trial was under CG. At the end of October, the ‘rotational’ pastures were again spelled from grazing and subsequently returned to an RG system. For the period under CG, the previously RG areas recorded slower pasture growth rates than those permanently under CG. Following a return to RG, pasture growth rates exceeded those in the CG area for the first regrowth period, but had slower subsequent regrowth during the summer–autumn period. The details of these results are not reported.
Pasture growth responses to superphosphate
In the absence of any grazing system × fertiliser rate interaction, all pasture responses presented are derived as the mean from RG and CG treatments. Over the 4 year study period, average pasture growth rate was nearly twice as high on Easy slopes as on Steep slopes at all times of the year.
In year 1 (1980–1981), there was no measured pasture growth response to P fertiliser on either Easy (mean 14,228 kg DM ha−1 yr−1) or Steep (mean 7172 kg DM ha−1 yr−1) slopes for either seasonal or annual total production levels ().
Table 2. Pasture dry matter production (kg DM ha−1) from Easy and Steep slopes during winter, spring, summer and autumn and for the total year at increasing rates of fertiliser P application. The annual means for the 1981–1984 period are also shown with the relative response (%) compared with that achieved from 100 kg P ha−1 applied to Easy and Steep slopes.
In year 2 (1981–1982), there was a margina1 response (P < 0.01) in winter and a response at the 5% level of probability in summer, largely through lower production from the 10 kg P ha−1 yr−1 treatment. These effects resulted in significantly lower annual production from the 10 kg P ha−1 treatment than from the treatments receiving 50 and 100 kg P ha−1 yr−1 on Easy, and 30–100 kg P ha−1 yr−1 on Steep slopes.
In year 3 (1982–1983), pasture responses to fertiliser were measured in all seasons (P < 0.05) and for the total year (P < 0.01). Total pasture production on Easy slopes and especially on Steep slopes was the lowest for any trial year, probably affected by the relatively low rainfall and high sunshine hours in that year ().
In year 4 (1983–1984), the seasonal response pattern was similar in winter and spring but not significant over the summer–autumn period. There was a significant (P < 0.05) effect of fertiliser on total annual dry matter production.
Mean annual pasture dry matter production over the responsive period (1981–1984) ranged from 11,275–14,155 kg DM ha−1 yr−1 on Easy and 6413–7480 kg DM ha−1 yr−1 on Steep slopes through the range of P fertiliser application rates ().
Seasonal pasture responses to superphosphate
The calculated proportional pasture growth pattern in each season was very similar on both Easy and Steep slopes over the range of P ferti1iser rates, i.e. the derived averages of mean total annual dry matter production over the 4 year trial period () were: winter—Easy 14.8%, Steep 16.1%; spring—Easy 46.3%, Steep 47.8%; summer—Easy 21.4%, Steep 20.4%; autumn—Easy 17.5%, Steep 15.7%.
The actual mean dry matter response to P fertiliser was greater in spring and summer than in other seasons, due predominantly to the much faster overall growth rates in those seasons, e.g. the mean response in dry matter production over the 1981–1984 period between pastures on Easy slopes receiving 10 kg P ha−1 yr−1 and those receiving 100 kg P ha−1 yr−1 was 957 kg ha−1 in spring, 847 kg ha−1 in summer, 566 kg ha−1 in autumn and 510 kg ha−1 in winter (), totalling 2880 kg DM ha−1 yr−1. The associated mean annual response on Steep slopes was 1067 kg DM ha−1 yr−1.
Pasture P content
Throughout the 1981–1982 year (year 2 of the trial), pasture P% on Easy slopes consistently increased with an increase in fertiliser P rate applied to both Easy and Steep slopes () and was generally higher in pasture from Easy compared with Steep slopes. Pasture P% was highest in early spring and lowest in autumn. In the fourth year of the trial (1983–1984), pasture P% showed a similar pattern of variation although P% concentrations in spring were higher in the pasture receiving 50 and 100 kg P ha−1 yr−1 than in spring of year 2 (1981–1982). In summer and autumn, the pasture P% had declined to levels similar to those in 1981–1982.
Table 3. Pasture P% measured in regrowth pasture throughout year 2 (1981–1982) and year 4 (1983–1984) at each rate of P fertiliser application (kg ha−1) to Easy and Steep slopes.
Pasture species composition
The pasture species component most sensitive to P fertiliser application was moss, which declined significantly in the 1982–1984 period on both Easy and Steep slopes with increasing rates of P application (). In 1983 and 1984, subterranean clover declined on Easy slopes only, with an increase in P fertiliser rate, whereas ryegrass and white clover increased on Easy slopes by 1984 with an increase in P fertiliser rate. Application of P fertiliser to steep slopes had no effect on these species during the trial period.
Table 4. Pasture species components (% frequency of occurrence) responsive to P fertiliser application. Measured in September 1982, 1983 and 1984.
Soil Olsen P tests
Prior to the start of the trial, the soil Olsen P status of the 0–3 cm depth samples (1980) showed some minor differences between Easy and Steep slopes but not at greater depths (). From the start of the trial in 1980 to the end of year 4 (February 1984), Olsen soil P levels declined in the 0–3 cm soil depth within the 10, 20 and 30 kg P ha−1 yr−1 fertiliser rate treatments. Olsen P tests at greater depths were little affected. Olsen P tests in the 50 kg P ha−1 treatment showed little change over this period at all soil depths. By contrast, the treatment applying 100 kg P ha−1 annually induced significant increases in Olsen P test by February 1984 at 0–3 and 3–7 cm depths, but not in the 7–15 cm depth.
Table 5. Soil Olsen P test (February) on Easy and Steep slopes from 1980 (pre-trial) to 1983–1984 (year 4) and anion storage capacity % measured on 3–7 cm depth soil samples in 1981 only.
The effects of P fertiliser were generally, but not always, to generate slightly higher Olsen P tests in soil on Easy slopes than on Steep slopes. Where these effects occurred they were significant but usually in the order of only 1–2 soil test units.
Water soluble soil P tests
Water soluble soil P (WSP) measured in 1981, the first year of the trial, and in 1983, was compared with Olsen P tests at all three sample depths in those years () in order to evaluate the usefulness of WSP as a measure of plant-available soil P. There was a highly significant relationship at the 0–3 cm depth (R2 = 94%, P < 0.001), but non-significant relationships at the 3–7 cm and 7–15 cm depths. A large part of this effect was because the 0–3 cm depth reflected the wide range in fertiliser P application rates whereas there was a much more narrow range in both Olsen P and WSP at 3–7 cm and 7–15 cm depths. There was no major effect of year (1981 cf. 1983) or slope on the relationships.
Soil anion storage capacity tests
Because of the possibility that the soil on Easy slopes may have contained some, or more, volcanic ash than soil on Steep slopes, soil ASC% tests were conducted on samples collected in February 1981 (3–7 cm depth only). Results showed that the ASC% tests on soil from Easy slopes were not significantly higher than on soil from Steep slopes (), i.e. 64% and 58.1%, respectively. Similarly, there were no significant differences between fertiliser treatments in soil ASC% tests and therefore no likely differential effects on pasture responses ().
Relationships between Olsen soil P tests and pasture production
Quantitative pasture production
An exponential (asymptotic) response curve was fitted between the 0–3 cm depth soil Olsen P levels in February each year on each strata and the associated total pasture growth from the previous winter until the following autumn. The Olsen P values from the different depths were used as the explanatory variable and the residual sums of squares from the non-linear model are given in Appendix 1. A derived Olsen P value for the 0–7 cm soil depth, to relate to the standard 7.5 cm soil depth used in advisory sampling, was found to be equally useful as a predictor of yield responses as was the 0–3 cm soil depth value (Appendix 1). Consequently, subsequent relationships between Olsen P tests and pasture yield were derived for the 0–7 cm depth only. In all seasons and for annual growth there was a highly significant difference between the Easy and Steep slopes (P < 0.001), a highly significant difference due to Olsen P, and significant difference (P < 0.05) in the curvature slope with Olsen P between Easy and Steep slopes. The fitted models for the mean of each season and for total annual pasture growth are given in . The shape of the annual curve for Steep slopes was especially influenced by the curves derived for summer and autumn when drier conditions resulted in no further pasture responses above Olsen P tests of about 8.
Figure 2. Mean seasonal and annual pasture yield responses (kg ha–1) from 1981–1984 on Easy and Steep slopes to increasing soil Olsen P status at a derived 0–7 cm depth.
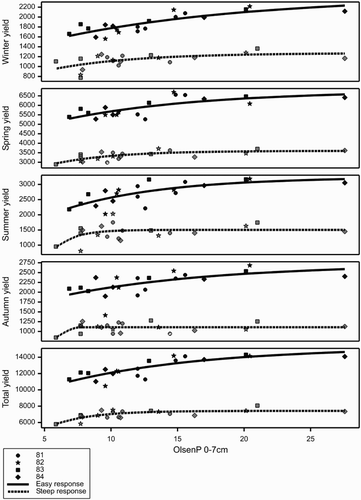
On Easy slopes, maximum annual pasture production derived was about 14,100 kg DM ha−1 yr−1. When this was related to Olsen P tests in the 0–3 cm depth, this production was obtained at an Olsen P soil test of about 43. Similarly, in relating production on Easy slopes to the derived Olsen P test at 0–7 cm soil depth, then the associated soil test was about 20.
The same analysis applied to Steep slopes showed a maximum annual yield of near 7500 kg DM ha−1 yr−1 obtained at a minimum Olsen P test to 0–3 cm depth of about 20. For 0–7 cm depth soil tests on Steep slopes, the associated minimum Olsen P test was about 12 for maximum annual production.
Relative annual yields in this trial on both Easy and Steep slopes were analysed to derive an asymptote for each response curve and to determine if these were statistically different.
Soil sulphate tests
The fertiliser treatments applied at 10, 20, 30, 50 and 100 kg P ha−1 as superphosphate also applied about 13, 27, 40, 67 and 133 kg S ha−1 yr−1, respectively. Soil sulphate tests after 3 years of treatment application were relatively similar at each depth over the range of S fertiliser application rates of 13–40 kg S ha−1 yr−1 and increased significantly with the higher rates of S application (). Sulphate tests were similar in the 0–3 cm depth soil on both Easy and Steep slopes but significantly lower in soil from Steep slopes at 3–7 and 7–15 cm depths. In most situations, soil sulphate levels declined with an increase in soil depth.
Table 6. Soil sulphate tests on archived samples from February 1983 (Year 3).
Soil pH, Ca, K and Mg tests
Soil pH and potassium levels were significantly higher on Easy than on Steep slopes throughout the trial. In contrast, soil calcium was generally similar on both Easy and Steep slopes, but more variable in 1984. Soil magnesium tests were similar at the 0–3 cm depth on both Easy and Steep slopes, but higher at greater depths on Steep slopes ().
Table 7. Soil pH, calcium, potassium and magnesium tests at 0–3, 3–7 and 7–15 cm depths from 1980 (pre-trial) to 1984 (mean of all fertiliser P rates).
Soil moisture
At all times of year, the soil moisture content (% by weight) was higher on Easy than on Steep slopes but was not affected by rate of fertiliser P application (). Soil moisture content was highest in October 1983, which was the month with highest rainfall () and lowest in January, the month with maximum sunshine hours, and one of the months with minimum rain days (). At this time, moisture content was lower in the 0–7 cm depth topsoil than in the 7–15 cm depth soil.
Table 8. Soil moisture content (% by weight in 0–7 cm depth soil) at each fertiliser P rate on both Easy and Steep slopes in Year 4 (1983–1984), both without, and with, adjustment for soil ASC% (measured on 3–7 cm depth soil samples only).
The slightly higher soil ASC% levels in soil on Easy slopes (average 64%) than on Steep slopes (average 58%) was assumed to be due to a higher volcanic ash content, which may be associated with a slightly lower soil bulk density, than in soil on Steep slopes. A similar volume of soil water would therefore result in higher soil moisture content by weight in soils of lower bulk density. Therefore, any possible differences in soil bulk density between soil on Easy and Steep slopes were adjusted by using the ASC% tests as a covariate (). This adjustment did not alter the statistical significance of the original relationships.
Discussion and conclusions
Pasture production on Easy slopes was greater and responded to higher rates of fertiliser P application than pasture on Steep slopes. Across the range of P application rates, the annual pasture production from Steep slopes ranged from only 55%–58% of that from Easy slopes.
The average ASC values for soil on Easy and Steep slopes were 64% and 58.1%, respectively. These are higher than the range of 20%–50% quoted for sedimentary soils (Edmeades et al. Citation2006) but lower than the associated range quoted for volcanic soils of 80%–100%. The brown soils on this trial site therefore fit midway between the above soil types in respect of their ASC properties.
An unexplained feature of the results is that the P application rate of 30 kg ha−1 did not generate higher Olsen P levels than the 20 kg P ha−1 application rate at any time during this trial (). Some possible reasons for this have been examined but fail to explain this anomaly. The pasture responses therefore should be related to soil P test rather than P application rate.
In order to understand the differences in the pattern of pasture responses on Easy and Steep slopes over the period of this trial it is important to recognise the changes in Olsen soil P that occurred. Over the P application rates from 10–30 kg P ha−1 yr−1, the Olsen P test in the 0–3 cm soil depth generally declined from the initial average levels of near 20 resulting from the pre-trial application of about 35 kg P ha−1 yr−1 over the previous 4 years ().
At the 50 kg P ha−1 rate, the 0–3 cm soil P tests were relatively stable through the trial period. At the 100 kg P ha−1 application rate, the Olsen soil P test increased in the 0–3 cm soil depth. At the 3–7 cm and 7–15 cm depths there was relatively little change in the Olsen P test within each P application rate and within each strata over the 4 year trial. Within each year and within each P application rate, the Olsen P test at any one soil depth was generally slightly lower on Steep than on Easy slopes. The exception to this was in the 50 and 100 kg P ha−1 treatments where from year 3 onwards the soil P test at 0–3 cm depth on Steep slopes was higher than on Easy slopes.
Three main factors therefore appear to contribute to the pattern of pasture responses measured. First, most of the effects of fertiliser application occurred in the 0–3 cm soil depth. Although this is a very shallow depth, earlier studies (Jackman & Mouat Citation1972; Gillingham Citation1978; Gillingham et al. Citation1980c) showed that more than two-thirds of relative root activity on slopes occurred within this depth. The soil P tests results in clearly show that this is the zone that reflected the greatest effects of fertiliser application, as would occur on farms with regularly topdressed pasture. It may be postulated that the concentration of soil P in the 0–3 cm soil depth is likely to accentuate the concentration of pasture root activity in that zone. The earlier studies showed that about 90% of relative root activity was in the 0–7 cm soil depth.
The second factor is that the pasture response pattern measured in this trial was due both to a decline in soil P in the 0–3 cm soil depth over the 10–30 kg P ha−1 range of application rates, together with an increase in soil P in the 0–3 cm soil depth at the 100 kg P ha−1 application rate. This showed that after the first year the pasture response was sensitive to both a decline, as well as an increase, in soil Olsen P test.
The third factor is the effect of P application rate on 0–3 cm depth Olsen soil P test on Easy and adjacent Steep slopes. At Olsen soil P tests up to about 10, the pasture on both Easy and Steep slopes responded in growth (). However, within the P application range of 10–30 kg P ha−1, Olsen P test at 0–3 cm depth was consistently lower on Steep than Easy slopes (). The net transfer loss of ingested P by grazing stock is known to be greater from Steep than from Easy slopes, i.e. 70% cf. 29%–49% of pasture P uptake, respectively (Gillingham et al. Citation1980b), and so would result in a relatively greater depletion of soil P from Steep slopes at any one fertiliser P application rate. At 100 kg P ha−1 yr−1, the soil P status in 0–3 cm depth soil was higher on Steep than on Easy slopes (). This could be at least partly attributed to the absence of a pasture response, and associated P uptake on Steep slopes, with 0–3 cm soil P tests greater than 15, and an associated accumulation of unutilised fertiliser P. In comparison, pasture on Easy slopes continued to respond and therefore utilise fertiliser P at 0–3 cm soil Olsen P levels of more than 40. This would have resulted in a relatively lower soil P test. It should be noted again that almost all of the above effects were limited to the 0–3 cm depth soil. Only at the 100 kg P ha−1 yr−1 application rate did the soil P test at 3–7 cm depth show an increase after 4 years. A further factor that must influence the Olsen P test at any one rate of fertiliser application is the effect of land slope in reducing the effective rate of P application. Steep slopes were on average about 20° steeper than Easy slopes used in this trial. This equates to about an extra 6% in land surface area and therefore 6% lower fertiliser application rate to Steep slopes.
In any on-farm situation, the effects of fertiliser application will depend on the relative importance of the above factors in contributing to the changes in soil P on Easy and Steep slopes and therefore the resulting pattern of pasture responses.
The expression of pasture production responses to increasing rates of P fertiliser as relative yields (RY) is the basis for model development to integrate results from a wide range of trials in contrasting conditions and on differing soil types (Metherell et al. Citation1995; Edmeades et al. Citation2006). The expression of RY also allows the derivation of the response curve asymptote which may not be obtained by the highest rate of P fertiliser used. In this trial, the derivation of separate RY curves for total annual yield showed that the asymptote on the Easy slopes was at 104% of the yield obtained by application of 100 kg P ha−1 yr−1, i.e. at 15,241 kg DM ha−1 yr−1 with an estimated Olsen P test of 38 (R2 = 0.711). On Steep slopes the asymptote was obtained with application of 100 kg P ha−1 yr−1 at an estimated total yield of 7425 kg DM ha−1 yr−1 with an estimated Olsen P test of 20 (R2 = 0.700). A non-linear regression accumulated analysis of variance showed that there was a highly significant effect of soil Olsen P level (P < 0.001) and a highly significant difference in the yield from Easy and Steep slopes (P < 0.001). However, there was no significant effect of land slope on Olsen P level (P = 0.291) and a reasonable, but not significant, difference in total yield relative response curvature between Easy and Steep slopes (P = 0.110).The relative yield response curvature was significantly different in autumn yields only. It could be concluded that a higher level of replication may have resulted in a significant difference for total annual yield being also detected.
A subsequent analysis using a common response curve model produced an RY curve that was dominated by, and similar to, the shape of the Easy slope relative response curve. The response curve for Steep slopes was therefore parallel to that of the Easy slopes response curve and produced similar parameters to those for the Easy response curve. It was concluded that this analysis did not properly represent the other differences between Easy and Steep slopes, and that the contrasting responses on Easy and Steep slopes were due to differences in other factors that determined the ability of the plant to acquire soil P, and should be further examined, as suggested by Edmeades et al. (Citation2006). It is clear from these results that where soils have similar type properties and P status, but have large differences in pasture productivity due for example to differences in soil moisture status, that such parameters should be included in the criteria to quantify optimum soil P conditions by use of RY curves.
Within the limits of the above analyses, the ‘critical’ Olsen P levels required to provide 97% of maximum yield (Edmeades et al. Citation2006) on Easy and Steep slopes were derived as 30 and 12, respectively. Water soluble soil P, a measure of ‘immediately plant-available’ P (Ryden & Syers Citation1977), showed a strong relationship with Olsen soil P at the 0–3 cm soil depth, mainly because of the wide range of values measured. This relationship was consistent for soil from both Easy and Steep slopes (). At greater soil depths, the range of values was much more limited and the associated relationships less clear, although it appears that at the same Olsen P test that the proportion of WSP was higher in soil from the 0–3 cm depth than at greater depths.
The seasonality of the pasture responses in the period from year 2 to year 4 varied from year to year. In year 2, responses were limited to winter and summer, all seasons contributed in year 3, and winter and spring seasons only in year 4 (). No explanation is given for this variation except that year 3 was the driest and sunniest ().Winter was the only season when pasture responses consistently occurred.
The P concentration in pasture increased with an increase in fertiliser P application rate at all times of the year in 1981–1982 and 1983–1984 with the exception of the harvest in autumn (April) 1984 (). On most occasions, the P content was slightly higher in pasture from Easy than from Steep slopes. In periods of active pasture growth (winter to late spring), the P concentrations in pasture on Easy slopes were in the optimum range (0.35%–0.40% of dry matter) (Morton & Roberts Citation1999) with fertiliser P application rates of 20 kg P ha−1 or greater. However, from late spring (November) 1981 and from November 1983 to March 1984, pasture P concentrations declined, especially on Steep slopes. This could be assumed to be due to relatively dry soil moisture conditions on Steep compared with Easy slopes at these times which would have reduced P uptake by pasture on Steep slopes. This was confirmed by soil moisture measurements taken in October 1983 and in January and April 1984 ().
Soil sulphate tests were on samples that had been archived for 32 years and so are unique in this respect. It is assumed that storage had no effect on soil sulphate test. Average sample volume-weights increased with depth, averaging 0.75 g mL−1 for 0–3 cm depth samples, 0.83 g mL−1 for 3–7 cm depth samples and 0.87 g mL−1 for 7–15 cm depth samples (data not shown). All sulphate tests were relatively high in these soils (ASC% average of 64% for Easy slopes and 58% for Steep slopes; ) compared with the values of 10–12 suggested by Morton & Roberts (Citation1999) as sufficient to sustain near maximum pasture production on sedimentary and ash derived soils. The sulphate soil test levels measured suggest that they pose no limitation to the expression of pasture responses to treatment P application levels in this trial.
Pasture species composition on Steep slopes was virtually unaffected by increased fertiliser P application (). The only exception was the moss content of pasture, measured in September, which declined significantly each year. It is of interest that although pasture growth on Steep slopes did not respond to high rates of P application, that the moss content continued to decline at these fertiliser rates. The same effect occurred on Easy slopes where moss content comprised a lower proportion of the pasture than on Steep slopes. In addition, both ryegrass (Lolium perenne) and white clover (Trifolium repens) content increased with an increase in fertiliser P rate and this was associated with a decrease in browntop (Agrostis tenuis) and subterranean clover (Trifolium subterraneum) content. The increase in white clover, and associated N fixation, and ryegrass content in the pasture on Easy slopes as a result of increased fertiliser P application, could be expected to contribute to the continued pasture dry matter responses at higher P rates on this strata.
The significantly greater steepness of slope in the Easy response curve in the average Olsen P test range of 10–15, compared with that of the Steep slope over the same soil P range (), can be attributed to pasture on the Easy areas having a greater ability to utilise available soil P to produce extra growth. The higher pasture production from easy than steeper slopes has been well documented (Gillingham & During Citation1973; Gillingham et al. Citation1980a; Lambert et al. Citation1983; Gillingham et al. Citation2007, Citation2008) and is assumed to be due largely to the higher levels of soil moisture available to pasture on Easy slopes. Periodic measurements during the 1983–1984 year confirmed that soil moisture (% by weight) was higher at all times in soil on Easy slopes, even after correcting for possible lower bulk densities associated with marginally higher ash content (and ASC%) of soil from Easy slopes. This result aligned with the soil moisture model later developed by Gillingham & Bircham (Citation1985) to predict soil moisture changes with change in slope of the surface of hill soils. The associated higher levels of soil nitrogen on Easy than Steep slopes (Ledgard et al. Citation1987) also contribute to higher pasture production from Easy slopes. In the present trial, higher levels of N uptake by pasture occurred under the higher rates of fertiliser P application (data not presented) indicating a greater N availability at levels of increasing soil P status, possibly due to greater associated legume growth as measured by Mackay et al. (Citation1990) on dry Wairarapa hill country.
The greater total responsiveness of pasture on Easy compared with Steep slopes in this trial suggests that variable rate fertiliser application technology, as is currently being developed (Morton et al. Citation2016), is appropriate on this hill country where separate target Olsen soil P tests (0–7 cm depth) of 30 on Easy slopes and 12 on Steep slopes, with associated differences in fertiliser application rates, would be appropriate.
Acknowledgements
Sincere thanks are due to S Richardson, I Power, J Riley and F McKay for technical assistance; H Hockey for the original statistical analysis and D Baird for subsequent statistical and graphical advice and analysis of data; G Sheath for useful discussions in trial planning and his technical support for pasture species recording; I Power for archiving of soil samples and progress reports; M Gray for some digitising of reports and data preparation; and AgResearch for access to archived soil samples.
Disclosure statement
No potential conflict of interest was reported by the authors.
Additional information
Funding
References
- Bircham JS. 1976. Grazing management for the improvement of browntop pastures in hill country: a programme. Proc New Zeal Grassl Assoc Conf. 38:87–93.
- Brougham RW. 1976. Some aspects of pasture development and management in New Zealand. Proc New Zeal Grassl Assoc Conf. 38:38–46.
- Bruce JG. 1976. Soil map of part of Raglan County, South Auckland, New Zealand. New Zealand Soil Bureau Map 100.
- Edmeades DC, Metherell AK, Waller JE, Roberts AHC, Morton JD. 2006. Defining the relationships between pasture production and soil P and the development of a dynamic P model for New Zealand pastures: a review of recent developments. New Zeal J Agr Res. 49:207–222. doi: 10.1080/00288233.2006.9513711
- Gillingham AG. 1973. Influence of physical factors on pasture growth on hill country. Proc New Zeal Grassl Assoc Conf. 35:77–85.
- Gillingham AG. 1978. Phosphorus cycling in grazed steep hill country unpublished [PhD thesis]. Palmerston North: Massey University.
- Gillingham AG, Bircham JS. 1985. Soil moisture monitoring and modelling on steep hill country. In: Campbell IB, editor. Proceedings of a seminar on soil dynamics and land use. Papers Presented at a Joint Seminar of the New Zealand Society of Soil Science and New Zealand Soil Conservators Association; 1985 May 20–24; Blenheim, New Zealand. Blenheim: Blenheim Printing; p. 218–229.
- Gillingham AG, During C. 1973. Pasture production and transfer of fertility within a long-established hill pasture. New Zeal J Exp Agr. 1:227–232. doi: 10.1080/03015521.1973.10427647
- Gillingham AG, Gray MH, Smith D. 1998. Pasture responses to phosphorus and nitrogen fertilisers on dry hill country. Proc New Zeal Grassl Assoc Conf. 60:135–140.
- Gillingham AG, Morton JD, Gray MH. 2007. Pasture responses to phosphorus and nitrogen fertilisers on East Coast hill country: total production from easy slopes. New Zeal J Agr Res. 50:307–320. doi: 10.1080/00288230709510299
- Gillingham AG, Morton JD, Gray MH, Roberts AHC. 2008. Pasture responses to phosphorus and nitrogen fertilisers on east coast hill country: 3. Production from steep slopes New Zealand. J Agr Res. 51:425–437. doi: 10.1080/00288230809510472
- Gillingham AG, Richardson S, Power IL, Riley J. 1990. Long term effects of withholding phosphate application on North Island hill country: Whatawhata Research Centre. Proc New Zeal Grassl Assoc Conf. 51:11–16.
- Gillingham AG, Syers JK, Gregg PEH. 1980a. Phosphorus uptake and return in grazed, steep hill pastures. 1. Pasture production and dung and litter accumulation. New Zeal J Agr Res. 23:313–321. doi: 10.1080/00288233.1980.10425362
- Gillingham AG, Syers JK, Gregg PEH. 1980b. Phosphorus uptake and return in grazed, steep hill pastures. 2. Above ground components of the phosphorus cycle. New Zeal J Agr Res. 23:323–330. doi: 10.1080/00288233.1980.10425363
- Gillingham AG, Tillman RW, Gregg PEH, Syers JK. 1980c. Uptake zones for phosphorus in spring by pasture on different strata within a hill paddock. New Zeal J Agr Res. 23:67–74. doi: 10.1080/00288233.1980.10417846
- Hewitt A. 2010. New Zealand soil classification. 3rd ed. Landcare Research Science Series 1. Lincoln: Manaaki Whenua Press.
- Hight GK. 1979. Hill country: a major agricultural resource and its capacity for increased production. P New Zeal Soc Anim Prod. 39:1–13.
- Jackman RH, Mouat MCH. 1972. Competition between grass and clover for phosphate. II. Effect of root activity, efficiency of response to phosphate and soil moisture. New Zeal J Agr Res. 15:667–675. doi: 10.1080/00288233.1972.10421623
- Lambert MG, Clark DA, Costall DA, Fletcher RM. 1983. Influence of fertiliser and grazing management on North Island moist hill country. 1. Herbage accumulation. New Zeal J Agr Res. 26:95–108. doi: 10.1080/00288233.1983.10420958
- Ledgard SF, Brier GJ, Gillingham AG, Sheath GW. 1983. Influence of some soil and pasture components on the growth of hill country pastures. II. Response to nitrogen fertiliser. New Zeal J Exp Agr. 11:251–256. doi: 10.1080/03015521.1983.10427764
- Ledgard SF, Brier GJ, Littler RA. 1987. Legume production and nitrogen fixation in hill pasture communities. New Zeal J Agr Res. 30:413–421. doi: 10.1080/00288233.1987.10417951
- Luscombe PC. 1981. Fertiliser nitrogen responses on two hill pastures of different phosphate fertiliser history. New Zeal J Exp Agr. 9:57–61. doi: 10.1080/03015521.1981.10427803
- Lynch PB. 1966. Conduct of field experiments. New Zealand Department of Agriculture Bulletin 399.
- Mackay AD, Tillman RW, Parker WJ, Barker DJ. 1990. Effect of superphosphate, lime, and cocksfoot on summer dry hill country pasture production. Proc New Zeal Grassl Assoc Conf. 51:131–134.
- Metherell AK, McCall DG, Woodward SJR. 1995. Outlook™: a phosphorus fertiliser decision support model for grazed pastures. In: Currie LD, Loganathan P, editors. Fertiliser requirements of grazed pastures and field crops. Proceedings of a workshop. Occasional Report No. 8. Palmerston North: Massey University; p. 24–39.
- Morton JD, Roberts AHC. 1999. Fertiliser use on New Zealand sheep and beef farms: the principles and practice of soil fertility and fertiliser use on New Zealand sheep and beef farms. Auckland: New Zealand Fertiliser Manufacturers’ Research Association.
- Morton JD, Stafford AD, Gillingham AG, Old A, Knowles O. 2016. The development of variable rate application of fertiliser from a fixed wing topdressing aircraft. Proceedings of the New Zealand Grassland Association Conference Hill Country Symposium, 12–13 April 2016. p. 163–167.
- [NRAC] National Research Advisory Council. 1980. Report of the National Research Advisory Council Primary Production Committee Working Party on Phosphate.
- O’Connor MB, Tonkin PJ, Ludecke TE. 1973. Fertiliser and stocking rates on two King Country soils. Proc New Zeal Grassl Assoc Conf. 35:63–76.
- Rowarth JS, Gillingham AG. 1990. Effects of withholding fertiliser on pasture production and phosphate cycling in hill country. Proc New Zeal Grassl Assoc Conf. 51:17–20.
- Ryden JC, Syers JK. 1977. Origin of the labile phosphorus pool in soils. Soil Sci. 123:353–361. doi: 10.1097/00010694-197706000-00003
- Scott M. 1983. North Island stock numbers could be doubled. Agr Econ. 3:9–11.
- Sheath GW, Boom RC. 1985. Effects of November–April grazing pressure on hill country pastures 1. Pasture structure and net accumulation rates. New Zeal J Exp Agr. 13:317–327. doi: 10.1080/03015521.1985.10426100
- Smith ME, Dawson A. 1976. Hill country grazing management. Proc New Zeal Grassl Assoc Conf. 38:47–55.
- Soil Survey Staff. 1999. Soil taxonomy. 2nd ed. USDA Natural Resources Conservation Service Agricultural Handbook 436. Washington, DC: US Government Printing Office.
- Suckling FET. 1959. Pasture management trials on unploughable hill country at Te Awa. 2. Results for 1951–57. New Zeal J Agr Res. 2:488–543. doi: 10.1080/00288233.1959.10418033
- Suckling FET. 1975. Pasture management trials on unploughable hill country at Te Awa.3. Results for 1959–69. New Zeal J Exp Agr. 3:351–436. doi: 10.1080/03015521.1975.10425834
Appendix 1
Explanation of statistics related to derivation of Olsen soil P: pasture production relationships
An exponential (asymptotic) response was fitted between the soil Olsen P levels in February each year and pasture growth from the previous winter until the following autumn. The Olsen P values from the different depths were used as the explanatory variable and the residual sums of squares from the non-linear model are given in Table A1.
Table A1.1. Residual sums of squares from fitting Olsen P from different depths to seasonal yields.
The Olsen P at 0–3 cm is the best predictor of yield response, but it is close to the predictive value for the 0–7 cm depth. The 0–7 cm was used in a subsequent analysis.
The overall differences between years were removed from the graphs. Separate rates of curvature were derived for Easy and Steep slopes. In all cases there was a highly significant difference between the Easy and Steep slopes, a highly significant difference due to Olsen P, and significant difference in the slope with Olsen P between Easy and Steep.
Refitting these models with a common non-linear parameter gave the following parameter estimates.