ABSTRACT
The New Zealand Government’s National Policy Statement on Fresh Water Management has resulted in increased scrutiny of New Zealand grazing systems with regards to their environmental impact. With this has been greater interest in nitrogen (N) loss mitigation options, including identifying crops with high nutritive value but low protein content. New Zealand data have been collated with key data fields: publication, experiment, site and, crop details and chemical analysis. This paper discusses these data with respect to the range of nutritive values observed in these New Zealand crops and feed supplements, including the implications for reducing N leaching risk. Feeds most likely to result in less urinary nitrogen (UN) excretion, and thus less nitrate (NO3−) leaching, include fodder beet, swedes, turnips, barley and wheat grain. The high soluble sugar and starch content of these feeds will support high animal production but may increase the risk of nutritional disorders.
Introduction
Urinary nitrogen (UN) excreted by grazing livestock is one of the New Zealand pastoral industry’s more significant environmental pollutants because nitrate (NO3−) derived from UN contributes to ground and surface water contamination (Di and Cameron Citation2002). High-quality temperate forages, such as perennial ryegrass and white clover in pastures, have metabolisable energy (ME) concentrations typically greater than 11.5 MJ kg DM−1 and a crude protein (CP) content of up to 30% (Waghorn et al. Citation2007; Dalley and Gardner Citation2012; Dalley and Geddes Citation2012). This high protein diet provides an excess of nitrogen (N) relative to animal requirements, and excretion of excess N results in increased environmental pollution. Dietary N requirement varies with class of livestock and physiological status. Therefore, it is important these factors are taken into consideration when developing livestock diets.
Ruminants do not utilise the N they ingest efficiently, with only 10–40% of the N ingested being converted to animal product (Haynes and Williams Citation1993; Jarvis et al. Citation1995). This low efficiency has implications for production performance and environmental impact. Of the N used, approximately 60–90% is returned to the soil-pasture system in excreta, the majority in urine (Haynes and Williams Citation1993). The amount of N deposited in urine patches (equivalent to up to 1000 kg N ha−1 for cattle (Freney Citation2011)) is greater than the capacity of pasture plants to assimilate, therefore the excess soil N is lost by ammonia volatilisation, NO3− leaching and denitrification.
Urinary N is strongly correlated with N intake, with several studies (Castillo et al. Citation2000; Kebreab et al. Citation2001) and meta-analysis of published data (Huhtanen and Hristov Citation2009; Kebreab et al. Citation2010) reporting lower total UN excretion when the protein content of the diet is less. Kebreab et al. (Citation2001) recommended several dietary adjustments to reduce N pollution, for example, grass grown with moderate fertiliser application, maize-based energy supplements formulated to provide slowly degradable protein and N intakes of less than 400 g day−1 for cows producing average milk yields. Firkins and Reynolds (Citation2005) reported that N excretion is related not only to N intake but also to the energy content of the diet and Cohen et al. (Citation2006) attributed higher milk production and lower UN excretion to supplementation of a high protein dairy cow diet with cereal grains.
Urea is the predominant N-containing compound in urine. After deposition of urine, urea is rapidly hydrolysed to ammonia, which is then converted to NO3− by nitrification in the soil (Haynes and Williams Citation1993), from where it readily leaches when soils are draining. Urine deposited on pasture in late summer, autumn and winter, which coincides with the main period of drainage in New Zealand pastoral systems (Ledgard and Menneer Citation2005), is more readily leached from below the root zone than urine deposited in spring and early summer (Di et al. Citation1999; Snow et al. Citation2011). Faecal N is present in a more stable form and, therefore, takes longer to break down than UN (Powell et al. Citation2009). Because of its slower breakdown and lower risk of leaching, a greater faecal N:UN ratio is desirable from an environmental perspective.
Mitigation strategies for reducing the amount of N from animals lost to the environment include; reducing the stocking rate, using pasture species with greater winter activity to utilise more soil N for plant growth, and increasing the efficiency of use of N by manipulating the diet, thereby reducing the amount of N excreted (Malcolm et al. Citation2014). Feeding cattle condensed tannins (Min et al. Citation2001), low degradable protein and high starch diets, and grazing cattle on grasses high in water soluble carbohydrates are options to reduce UN excretion. These dietary changes contribute to increased synthesis of microbial protein such that less ammonia is lost from the rumen (Dove and Robards Citation1974; Misselbrook et al. Citation1998; Kebreab et al. Citation2001).
With dairy cows fed only pasture, the opportunity to manipulate the protein content of the diet is limited. One approach to improving N use efficiency is to feed high-sugar ryegrass cultivars (Clark et al. Citation2001; Miller et al. Citation2001). Miller et al. (Citation2001) reported a 30% increase in feed N used for milk production and a 28% reduction in UN excretion when cows grazed a high-sugar ryegrass cultivar, compared with a standard perennial ryegrass cultivar. An alternative approach to manipulating pasture N intake is to offer feed supplements with a lower crude protein and higher carbohydrate content (Firkins and Reynolds Citation2005); however, these feeds must be profitably integrated into the grazing system (Clarke et al. Citation2007). In 2007, 40% of the New Zealand dairy farms were estimated to use some supplementary feed in their farming system (Clarke et al. Citation2007), with more than 30 types of supplements identified (Anon. Citation2005). The objectives of this study were to collate feed quality information for crops and supplements available to grazing animals in New Zealand and assess the implications of the feed types and range in nutritive value for reducing N leaching risk.
Materials and methods
New Zealand scientific literature was screened to identify crop quality data for inclusion into an Excel dataset. The key data fields were publication details, experimental details, site details, crop details, chemical analysis, mineral analysis, protein degradability and amino acid profiles. Unpublished datasets from The New Zealand Institute for Plant & Food Research Ltd and DairyNZ Ltd were also included in the database as published crop quality data were sparse.
Ten feed categories were considered: brassicas, beets, cereal grains, cereal silages, C4 grass species, legumes, herbs, hay and straw, formulated supplements and ‘other’ (e.g. sunflower). Analysis of the dataset was undertaken, as described below, to indicate trends in nutritive value within and between feed categories.
The key quality indicators that were used to identify feeds with the potential to reduce UN excretion, while still achieving high animal production performance, were CP and soluble sugars and starch (SSS). Optimum ranges for each of these for lactating and non-lactating dairy cows were determined from the literature and used to screen the data to identify feed categories for further investigation. A CP content range of 16–20% and SSS of 25–60% were identified as appropriate for the production levels of lactating dairy cows in New Zealand (Holmes et al. Citation2002). These ranges were set to meet protein requirements and minimise the risk of digestive upsets associated with high SSS intake if these feeds formed more than 40% of the diet. Appropriate ranges for non-lactating cows were 10–16% CP and 25–50% SSS. An environmental index combining CP (<12%) and SSS (>30%) was used to identify feeds likely to minimise UN excretion.
The screening of the feeds was based solely on their nutritive characteristics. No consideration was given to how these feeds might be integrated into the farm system, that is, the proportion of the feed that could be safely fed in the diet of a dairy cow or the logistics of feeding each feed to achieve the production and environmental outcomes.
Pivot tables in Excel were used to calculate the mean and ranges of nutritive value for each feed in the dataset, to identify individual crops most likely to achieve high animal performance at the same time as having the potential to reduce UN excretion. The Excel dataset was stratified per feed type, and sort/select functions used to determine the frequency of occurrence within specified CP and SSS ranges for non-lactating cow feeding and lactation periods (). Cumulative probability analysis (Excel) was used to identify ranges of values indicating opportunity to utilise feeds for intended purposes, and identify where potential crop management intervention could assist in achieving desired feed quality outcomes.
Table 1. Percentage ranges for crude protein (CP) and soluble sugar + starch (SSS) and associated index weighting for feeds giving best performance of lactating or non-lactating dairy cows, and best environmental outcomes.
Feeds with a potential to reduce NO3− leaching were primarily those with high carbohydrate (SSS) to CP ratio. In addition to the absolute levels of carbohydrate and protein in the feeds, variables were derived for ratio of SSS to protein, additive indices (CP + SSS values) or combinations (quality + environmental weighting) providing best fit for non-lactating cows, lactating cows or best environmental solution. A total index () was the summation of indices for ranges of CP, SSS and SSS:CP ratio. Feeds with a high index total were identified as best options to satisfy feed requirements or minimise UN excretion. In calculating indices for environmental outcome, added weight was given to the protein content compared to SSS as this had a proportionately greater impact on UN excretion. A simple adjustment to the environmental index was applied by doubling the weighting for high and medium range of CP. Otherwise, a simple index value of 1, 2 or 3 defined the low to high impact which differed for cow class or environment outcome. In the case of a combined CP and SSS index (Parameter No 3, ), the feeds were first identified as fitting within ranges for CP, then selected using a weighting value of 4 for fit within a SSS range, otherwise assigned a value of 1. This was also applied in the combined environment + quality index
Results
Using published data only, 91 row entries in the spreadsheet represent 32 different forages. With the exception of fodder beet (Beta vulgaris L.), where there were 13 publications including crop quality parameters, all other crop types had 6 entries or fewer (). Including unpublished data resulted in 2770 row entries representing 71 crops or crop mixes (). Crop mixtures and pastures, while included in the dataset, are not reported. Cereal silages accounted for 43% of the entries in the dataset with chicory (Cichorium intybus L.), kale (Brassica oleracea ssp. acephala L), peas (Pisum sativum L.) and fodder beet representing the second most accounted for crops.
Table 2. Nutrient composition (%), means (ranges) for forage crops and supplements in New Zealand.
The nutritive value of the feeds in the unpublished datasets were determined by near infrared reflectance spectroscopy (NIRS). A total of 2002 samples (78%) were analysed by Feedtech (Palmerston North), 229 by ARL (Napier), 210 by Hill Laboratories (Hamilton), 82 by DairyOne (Ithaca, New York, USA) and 33 by Lincoln University (Canterbury).
Legumes and herbs had the highest average CP concentrations but for most feeds there was at least a two-fold range of CP concentration (). The exceptions were whole swedes (Brassica napus spp. napobrassica; leaf + bulb), fodder beet roots (nb. the beet itself is a thickened root, not a bulb), cereal grains, Sudan grass (Sorghum sudanense (Piper) Stapf), sulla (Hedysarum coronarium L.) and some of the legumes. For some feeds, caution was required when interpreting the data due to the small number of samples in the dataset. SSS were highest in the brassicas and beets, especially the bulbs and roots, and in the cereal grains and maize silage. Average neutral detergent fibre (NDF) and acid detergent fibre (ADF) concentrations were lowest in the cereal grains, bulbs of swedes and turnips and the roots of fodder beet (). Hay & straw and cereal silages contained the highest fibre concentrations (ADF > 25% and NDF > 43%).
Of the brassica and fodder beet crops in the dataset, 90% of the whole fodder beet (leaf + root) samples contained less than 10% CP ((C)). Forty percent of the bulbs of swedes and turnips (Brassica rapa var. rapa) contained between 10% and 16% CP. Rape (B. napus ssp. biennis) samples had c. 40% of the samples in each of the 10–15.9% and 16–22% ranges ((A)). Twenty percent of rape, 40% of the leafy turnips (B. rapa; syn. B. campestris), 40% of the leaves of bulb turnips and 60% of the leaves of swedes had more than 22% CP ((A, C)). Soluble sugar and starch levels were highest in whole fodder beet, swede bulbs and turnip bulbs with 96%, 100% and 100% of the samples, respectively, having more than 25% SSS ((B, D)). Whole fodder beets were more variable in their SSS content () than whole turnips but had a considerably greater proportion of samples with more than 25% SSS.
Figure 1. Cumulative probabilities in brassicas, cereals and maize for crude protein (A, C and E) and soluble sugars + starch (B, D and F) content. Ranges of crude protein requirements for non-lactating and lactating cows are shown in light and dark shading respectively. Dashed lines (---) for SSS indicate relatively low, medium and high levels.
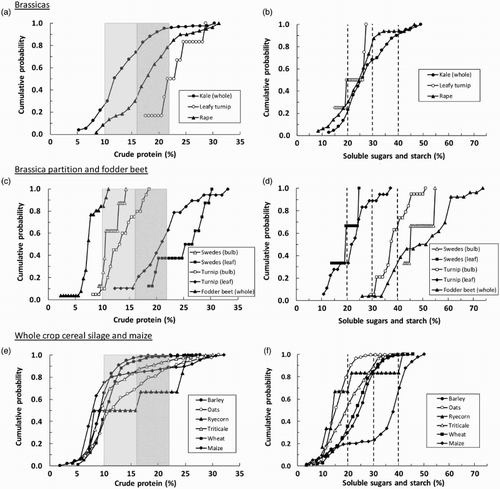
In the C4 category, maize (Zea mays L.) silage had the highest proportion of samples with less than 10% CP ((E)). Crude protein concentrations in Sorghum and Sudan grass were mostly in the 10–22% range (data not presented). Maize samples ranged widely in SSS content (), but 60% of the samples were between 35% and 50% SSS ((F)). Too few Sorghum and Sudan grass samples were represented in the dataset to report trends.
Within the cereal silage and cereal grain categories, the range in CP and SSS content varied with cereal type ((E, F)). Barley (Hordeum vulgare L.), wheat (Triticum aestivum L.) and triticale (x Triticosecale Wittm. ex A. Camus) silages had a higher proportion of samples (>90%) with a CP concentration less than 16%. In contrast, oat (Avena sativa L.) and ryecorn (Secale cereal L.) silages had 25–30% of samples greater than 16% CP. A higher proportion of wheat and barley silage samples (>34%) contained more than 25% SSS compared with triticale, oats and ryecorn (<24%). All wheat and barley grain samples were less than 16% CP and greater than 50% SSS.
Differences were found in the CP and SSS distribution of chicory and plantain (Plantago lanceolate L.) ((C, D)). Both species had fewer than 10% of samples with a CP concentration below 16%, but 70% of chicory samples had CP greater than 22%, compared with only 40% of plantain samples. No plantain samples exceeded 25% SSS but 10% of chicory samples were greater than 25% SSS. Sunflowers (Helianthus annuus L.) had a narrow range of CP concentration and no samples in the dataset exceeded 13% CP. Two thirds of sunflower samples exceeded 25% SSS.
Figure 2. Cumulative probabilities for crude protein (A, C and E) and soluble sugars + starch (B, D and F) content in legumes, herbs, sunflower and pasture. Ranges of crude protein requirements for non-lactating and lactating cows are shown in light and dark shading respectively. Dashed lines (---) for SSS indicate relatively low, medium and high levels.
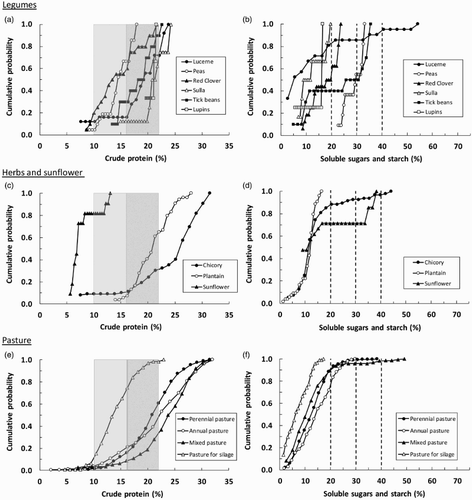
While the majority of the legumes in the dataset had CP concentrations greater than 16%, peas and red clover (Trifolium pratense L.) were exceptions with 67% and 43% of samples, respectively, containing less than 16% CP ((A)). Sulla had the highest proportion of samples exceeding 22% CP. In contrast with red clover where all samples were less than 20% SSS, all pea samples exceeded 20% SSS and 60% of the samples were greater than 25% ((B)). Lucerne (Medicago sativa L.) and tick beans (Vicia faba L.) had similar CP distribution profiles but more lucerne samples exceeded 50% SSS.
In comparison, ryegrass (Lolium perenne L.)/white clover (Trifolium repens L.) pastures were high in CP (at least 50% of samples greater than 22%), with the exception of ryegrass/white clover pasture for silage where ca. 90% of the samples in the database were between 10% and 22% CP ((E)). At least 90% of pasture samples had SSS concentrations less than 25% and the pasture for silage had no samples above 20% SSS ((F)).
Discussion
The data presented in this paper build on information reported by de Ruiter et al. (Citation2007) who summarised the nutritive value of supplements fed in New Zealand. For many of the crops the average CP content is similar between the two studies, but the range in values is wider in the current dataset. This most likely reflects the greater number of samples, from a wider range of sources, contributing to the data here.
For lactating cows, brassica (particularly rape and turnips), wheat grain and whole crop wheat silages featured strongly in the pre-defined critical ranges of 16–20% CP (Holmes et al. Citation2002) and greater than 25% SSS. While these criteria were useful for screening the dataset, they excluded high SSS feeds with low CP concentrations, for example, fodder beet, maize silage and barley grain, especially for lactating cows. Although below the CP requirements for lactating dairy cows if fed alone, these low CP but high SSS feeds are valuable supplements when offered with higher CP pasture.
For non-lactating cattle whole crop cereal silages (wheat, barley, triticale), brassicas (turnips, kale, swedes) and legumes (peas, tick beans) were most likely to contain 10–16% CP and 25–50% SSS. Expanding the range to include feeds up to 80% SSS included cereal grains (barley, wheat), maize silage and fodder beet. Diets of non-lactating cows in regions of New Zealand with slow (<5 kg DM/ha day−1) pasture growth rates in winter are already very reliant on brassica crops and fodder beet to supply nutrient requirements (Edwards et al. Citation2014) and there is widespread use of maize silage in central and northern areas of the North Island. Unexpected inclusions in this selection were peas and tick beans since legume crude protein content is generally expected to be in the moderate to high range with crude protein levels often above 20% of DM (de Ruiter et al. Citation2007).
Litherland and Lambert (Citation2007) argued that the nutritive value of feeds is important for achieving both high animal performance and profit in New Zealand grazing systems. They also hypothesised that, in the future, New Zealand livestock systems would come under increased pressure regarding environmental issues, including N contamination of water, and that controlling the nutrient content of the diet would be an important mitigating factor. The New Zealand Government’s National Policy Statement on Fresh Water Management (Ministry for the Environment Citation2011) has resulted in increased scrutiny of New Zealand grazing systems with regards to their environmental impact, and generated greater interest in N loss mitigation options. The strong correlation between N intake and UN excretion (Kebreab et al. Citation2001) and the risk of UN being leached into groundwater (Di and Cameron Citation2002) has focused research efforts on identifying crops with high-nutritive value but reduced CP concentration. Partial replacement of pasture by low-protein, high-carbohydrate supplements may be a suitable method to improve protein utilisation by grazing animals and consequently reduce UN excretion (van Vuuren et al. Citation1993; Cohen et al. Citation2006). To achieve this goal, understanding the variation in crop quality characteristics and differences between crop categories is necessary.
Development and interrogation of a New Zealand feeds dataset provided insight into crop categories and individual crops most likely to achieve high animal performance at the same time as having the potential to reduce UN excretion. It is important to note that any environmental benefit from a particular feed will be dictated by the composition of the total diet, that is, pasture plus any other feeds offered, how it is fed, in what proportions it is fed and which class of stock it is fed to. Additionally, while some feeds may have a desirable nutrient profile, it may be difficult to integrate them into the feeding system due to timing of availability, logistics of growing or feeding, and animal health considerations, for example, safely group-feeding cereal grain to grazing dairy cattle. NDF and ADF content are important indicators of the likelihood of rumen disorders when feeds high in SSS are incorporated in the diet. Stockdale et al. (Citation1997) recommended diet NDF concentrations of 25–35%, with requirements increasing as food intake increases, for effective rumen function and maintenance of milk fat content in pasture based systems in Australia.
Also important is that a large percentage of the feeds included in the dataset were analysed as fresh material. This could have implications for those feeds that are predominantly fed to livestock as silage (e.g. whole crop cereals) as the chemical composition of the feed will change when ensiled (Bruinenberg et al. Citation2002). The quality of any conserved feed is largely determined by the quality at harvest, but some aspects of conservation affect feed quality, especially wilting time, chopping length, additives and oxygen removal (Litherland and Lambert Citation2007). Successful ensiling requires a fast release of non-structural carbohydrates (Bruinenberg et al. Citation2002) and compaction to reduce oxygen levels to produce conditions suitable for anaerobic fermentation and production of organic acids which stabilise the silage.
Better environmental outcomes may be achieved when feed supplements with appropriate composition are offered to grazing livestock. Feeds most likely to result in less UN excretion, and thus less NO3− leaching, will have a CP content <12% and a SSS content >30%. These include fodder beet, potato, swedes, turnips, barley and wheat grain. The high SSS content of these diets can also support high animal production but will increase the risk of nutritional disorders, for example, acidosis, if not managed properly (Nichol Citation2007). Meeting the CP requirements of lactating cattle or growing livestock is challenging when high SSS feeds constitute a significant proportion (>40%) of the diet.
Rape, kale and turnip leaf, having consistently 10–20% CP, can be useful in managing dietary CP. In contrast, swede and turnip bulbs and fodder beet roots which have a much narrower range of CP concentration, are less useful. The leaf to bulb/root ratio is likely to have a significant impact on the nutritive value of bulb and root crops in any diet consumed, given the observed differences in CP and SSS content between these plant parts (). Crops in other feed categories, with more than 40% of the samples between 10% and 20% CP, included wheat, oat and triticale silages, plantain and the legumes (peas, red clover and lucerne).
The range in CP and SSS is an integrated response to the biotic factors contributing to the profile for specific crops and feed types. Therefore, it should be possible to manipulate crop quality by varying crop management and site-specific factors to achieve desirable quality characteristics. Insufficient crop management details were available for the majority of samples in the dataset to allow identification of the major factors contributing to differences in CP and SSS within a given crop type. Factors such as cultivar, geographic location, sowing density, stage of maturity, soil type, fertiliser history and climate can all affect the nutritive value of the plant at harvest (Litherland and Lambert Citation2007). Future reporting of data on the nutritive value of crops and supplements should include crop agronomy details. Having access to such information in the current dataset would have enabled identification of the management practices most likely to generate crops and supplements of the quality required to meet, but not exceed, animal demands.
Data from this paper could be used to test the nutritional assumptions that have been made using computer models. The proposed optimum ranges of CP and SSS in feeds to achieve high levels of production with reduced N footprint were based on nutrition principles but did not consider the practicalities of how these feeds might be integrated into the farm system. Animal models such as Molly-DairyNZ and MINDY (Gregorini et al. Citation2015a, Citation2015b) could be used to predict reductions in urinary N excretion, for a range of livestock classes, with different supplementary feeds varying in crude protein and SSS content. The outputs from this modelling could then be incorporated into farm systems models (DairyNZ Whole Farm Model; Beukes et al. Citation2008) to predict the impact of feed changes on profit, productivity and environmental performance.
Conclusions
In grazing systems, the value of feed crops and supplements is influenced by their nutritional composition and practicality of feeding, including the class of stock being fed and when the feed is available for grazing or conservation. This paper has identified supplements with the potential to achieve the goal of improved animal productivity with a reduced environmental footprint but was unable to determine the crop management practices that resulted in the range of nutritive values that was observed.
Rape, turnips, wheat grain and whole crop wheat silages featured strongly in the pre-defined critical ranges of 16–20% CP and greater than 25% SSS, providing suitable feed profiles for lactating dairy cows. Other feeds, with high SSS feeds and low CP concentrations, such as fodder beet, maize silage and barley grain, were useful dietary supplements. While they were below the CP requirements for lactation if fed alone, they are high energy and therefore valuable when offered with higher CP pasture.
For non-lactating cattle requiring less protein (10–16% range), whole crop cereal silages (wheat, barley and triticale), brassicas (turnips, kale and swedes) and legumes (peas, tick beans) were the best fit within the 15–50% SSS range. Cereal grains (barley, wheat), maize silage and fodder beet were included as ideal non-lactating feeds if the SSS content was raised to 80%, but again these are best incorporated into a mixed diet.
Feeds most likely to result in less UN excretion, and thus less NO3− leaching, will have a CP content of <12% and a SSS content of >50%. These include fodder beet, potato, swedes, turnips, barley and wheat grain. The high SSS content of these diets can also support high animal production but may increase the risk of nutritional disorders if fed as high proportion of the diet.
Acknowledgements
The Forages for Reduced Nitrate Leaching programme is a partnership between DairyNZ Ltd, AgResearch, Plant & Food Research, Lincoln University, Foundation for Arable Research and Landcare Research. We thank Louise Gibson and Elena Minnee for technical support and provision of data.
Disclosure statement
No potential conflict of interest was reported by the authors.
Additional information
Funding
References
- Anonymous. 2005. Report on forage supplements workshop. Plymouth International Hotel, New Plymouth, 13 and 13 October 2005. Cited in Clarke et al. 2007.
- Beukes P, Palliser C, Macdonald K, Lancaster J, Levy G, Thorrold B, Wastney M. 2008. Evaluation of a whole-farm model for pasture-based dairy systems. Journal of Dairy Science. 91:2353–2360. doi: 10.3168/jds.2007-0728
- Bruinenberg MH, Valk H, Korevarra H, Struik PC. 2002. Factors affecting digestibility of temperate forages from seminatural grasslands: a review. Grass and Forage Science. 57:292–301. doi: 10.1046/j.1365-2494.2002.00327.x
- Castillo AR, Kebreab E, Beever DE, France J. 2000. A review of the efficiency of nitrogen utilisation in lactating dairy cows and its relationship with environmental pollution. Journal of Animal and Feed Sciences. 9:1–32. doi: 10.22358/jafs/68025/2000
- Clark H, de Klein C, Newton P. 2001. Potential management practices and technologies to reduce nitrous oxide, methane and carbon dioxide emissions from New Zealand Agriculture. Report prepared for Ministry of Agriculture and Forestry, September. 2001.
- Clarke DA, Caradus JR, Monaghan RM, Sharp P, Thorrold BS. 2007. Issues and options for future dairy farming in New Zealand. New Zealand Journal of Agricultural Research. 50:203–221. doi: 10.1080/00288230709510291
- Cohen DC, Stockdale CR, Doyle PT. 2006. Feeding an energy supplement with white clover silage improves rumen fermentation, metabolisable protein utilisation and milk production in dairy cows. Australian Journal of Agricultural Research. 57:367–375. doi: 10.1071/AR05166
- Dalley DE, Gardner G. 2012. Pasture growth and quality on West Coast dairy farms. Proceedings of the New Zealand Grasslands Association. 74:231–236.
- Dalley DE, Geddes T. 2012. Pasture growth and quality on Southland and Otago dairy farms. Proceedings of the New Zealand Grasslands Association. 74:237–242.
- de Ruiter JM, Dalley DE, Hughes TP, Fraser TJ, Dewhurst RJ. 2007. Types of supplements: their nutritive value and use. In: Rattray PV, Brookes IM, Nicol AM, editors. Pastures and supplements for grazing animals. Hamilton: New Zealand Society of Animal Production; p. 97–115, Occasional Publication Number 14.
- Di HJ, Cameron KC. 2002. Nitrate leaching in temperate agroecosystems: sources, factors and mitigating strategies. Nutrient Cycling in Agroecosystems. 64:237–256. doi: 10.1023/A:1021471531188
- Di HJ, Cameron KC, Moore S, Smith NP. 1999. Contributions to nitrogen leaching and pasture uptake by autumn-applied dairy effluent and ammonium fertilizer labelled with 15N isotope. Plant and Soil. 210:189–198. doi: 10.1023/A:1004677902049
- Dove H, Robards GE. 1974. Effect of abomasal infusions of methionine, casein, and starch plus methionine on the wool production of merino wethers fed on lucerne or wheaten chaff. Australian Journal of Agricultural Research. 25:945–956. doi: 10.1071/AR9740945
- Edwards GR, deRuiter JM, Dalley DE, Pinxterhuis JB, Cameron KC, Bryant RH, Di HJ, Malcolm BJ, Chapman DF. 2014. Dry matter and body condition score change of dairy cows grazing fodder beet, kale and kale-oat forage systems in winter. Proceedings of the New Zealand Grasslands Association. 76:81–88.
- Firkins JL, Reynolds C. 2005. Whole-animal nitrogen balance in cattle. In: Pfeffer E, Hristov AN, editors. Nitrogen and phosphorus nutrition of cattle. Wallingford: CAB International; p. 167–186.
- Freney JR. 2011. Management practices to increase efficiency of fertilizer and animal nitrogen and minimize nitrogen loss to the atmosphere and groundwater. Technical Bulletin – Food and Fertilizer Technology Center. 186:22.
- Gregorini P, Beukes P, Pacheco D, Waghorn G, Hanigan M. 2015a. Development of an improved representation of rumen digesta outflow in a mechanistic and dynamics model of a dairy cow, molly. Ecological Modelling. 313:293–306. doi: 10.1016/j.ecolmodel.2015.06.042
- Gregorini P, Villalba JJ, Provenza FD, Beukes PC, Forbes JM. 2015b. Modelling preference and diet selection patterns by grazing ruminants: a development in a mechanistic model of a grazing dairy cow, MINDY. Animal Production Science. 55:360–375. doi: 10.1071/AN14472
- Haynes RJ, Williams PH. 1993. Nutrient cycling and soil fertility in the grazed pasture ecosystem. Advances in Agronomy. 49:119–199. doi: 10.1016/S0065-2113(08)60794-4
- Holmes CW, Brookes IM, Garrick DJ, Mackenzie DDS, Parkinson TJ, Wilson GF. 2002. Milk production from pasture: principles and practices. Palmerston North: Massey University; p. 602.
- Huhtanen P, Hristov AN. 2009. A meta-analysis of the effects of dietary protein concentration and degradability on milk protein yield and milk N efficiency in dairy cows. Journal of Dairy Science. 92:3222–3232. doi: 10.3168/jds.2008-1352
- Jarvis SC, Scholefield C, Pain B. 1995. Nitrogen cycling in grazing systems. In: Bacon PE, editor. Nitrogen fertilisation in the environmental. New York (NY): Marcel Dekker; p. 381–419.
- Kebreab E, France J, Beever DE, Castillo AR. 2001. Nitrogen pollution by dairy cows and its mitigation by dietary manipulation. Nutrient Cycling in Agroecosystems. 60:275–285. doi: 10.1023/A:1012668109662
- Kebreab E, Strathe AB, Dijkstra J, Mills JAN, Reynolds CK, Crompton LA, Yan T, France J. 2010. Energy and protein interactions and their effect on nitrogen excretion in dairy cows. In: Crovetto GM, editor. 3rd EAAP international symposium on energy and protein metabolism and nutrition. Wageningen: Wageningen Academic; p. 417–425.
- Ledgard SF, Menneer JC. 2005. Nitrate leaching in grazing systems and management strategies to reduce loses. In: Currie LD, Hanley JA, editors. Proceedings of the workshop development in fertiliser application technologies and nutrient management, Massey University, Palmerston North, New Zealand. Occasional Report No. 18. New Zealand: Massey University Palmerston North; p. 79–82.
- Litherland AJ, Lambert MG. 2007. Factors affecting the quality of pastures and supplements produced on farms. In: Rattray PV, Brookes IM, Nicol AM, editors. Pastures and supplements for grazing animals. Hamilton: New Zealand Society of Animal Production; p. 81–96, Occasional Publication Number 14.
- Malcolm BJ, Cameron KC, Edwards GR, Di HJ, Moir J. 2014. The effect of four different pasture species compositions on nitrate leaching losses under high N loading. Soil Use and Management. 30:58–68. doi: 10.1111/sum.12101
- Miller LA, Moorby JM, Davies DR, Humpreys MO, Scollan ND, MacRae JC, Theodorou MK. 2001. Increased concentration of water-soluble carbohydrate in perennial ryegrass (Lolium perenne): milk production from late lactation dairy cows. Grass and Forage Science. 56:383–394. doi: 10.1046/j.1365-2494.2001.00288.x
- Min BR, Frenandex JM, Barry TN, McNabb WC, Kemp PD. 2001. The effect of condensed tannins in Lotus corniculatus upon reproductive efficiency and wool production in ewes during autumn. Animal Feed Science and Technology. 92:185–202. doi: 10.1016/S0377-8401(01)00258-9
- Ministry for the Environment. 2011. New Zealand Government’s National Policy Statement on Fresh Water Management. https://www.mfe.govt.nz/publications/rma/nps-freshwater-management-2011/docs/nps-freshwater-mgnt-2011.pdf [accessed 2015 December 13].
- Misselbrook TH, Chadwick DR, Pain PB, Headon DM. 1998. Dietary manipulation as a means of decreasing N losses and methane emissions and improving herbage N uptake following application of pig slurry to grassland. Journal of Agricultural Science. 130:183–191. doi: 10.1017/S0021859697005194
- Nichol WW. 2007. Nutritional disorders of ruminants caused by consumption of pasture and fodder crops. In: Rattray PV, Brookes IM, Nicol AM, editors. Pastures and supplements for grazing animals. Hamilton: New Zealand Society of Animal Production; p. 133–149, Occasional Publication Number 14.
- Powell JM, Borderick GA, Grabber JA, Hymes-Fect UC. 2009. Effects of forage protein-binding polyphenols on chemistry of dairy excreta. Journal of Dairy Science. 92:1765–1769. doi: 10.3168/jds.2008-1738
- Snow VO, Shepherd MA, Cichota R, Vogeler I. 2011. Urine timing: are the 2009 Waikato results relevant to other years, soils and regions. In: Currie LD, Christensen CL, editors. Adding to the knowledge base for the nutrient manager. http://flrc.massey.ac.nz/publications.html. Occasional Report No. 24. Palmerston North: Fertilizer and Lime Research Centre, Massey University; p. 13.
- Stockdale CR, Dellow DW, Grainger C, Dalley D, Moate PJ. 1997. Supplements for dairy production in Victoria. Melbourne: Dairy Research and Development Corporation; p. 95.
- van Vuuren AM, van der Koelen CJ, Valk H, de Visser H. 1993. Effects of partial replacement of ryegrass by low protein feeds on rumen fermentation and nitrogen loss by dairy cows. Journal of Dairy Science. 76:2982–2993. doi: 10.3168/jds.S0022-0302(93)77637-7
- Waghorn GC, Burke JL, Kolver ES. 2007. Principles of feeding value. In: Rattray PV, Brookes IM, Nicol AM, editors. Pastures and supplements for grazing animals. Hamilton: New Zealand Society of Animal Production; p. 35–60, Occasional Publication Number 14.