ABSTRACT
Time of urine application on mineral N (Nmin) leaching from a pumice soil was tested. Artificial urine (600 kg N ha−1) was applied in early June or late July to small plots after removing a kale/swede crop or to ryegrass/white clover. Mineral N leached in the first winter (measured by porous cups, 600 mm deep) was similar under pasture or ex-forage crop: 185 vs. 60 kg N ha−1 for June or July urine, with 170–300 kg N ha−1 remaining in the soil (0–600 mm) at the end of drainage. Additionally, 54–118 kg N ha−1 leached in the second winter. In total, later application decreased Nmin leaching by 35–40%. Application of the process inhibitor dicyandiamide increased soil Nmin levels in the post forage crop site for the early urine application but had no effect in the late-applied urine treatment. There was no significant effect on N leaching.
Introduction
Brassica crops such as kale (Brassica oleracea) and swede (Brassica napobrassica) grown as winter forage crops can contain large amounts of nitrogen (N) at maturity. For example, Edwards et al. (Citation2014) report pre-grazing kale yields in Canterbury of 11.9–15.5 t DM ha−1, with a protein content of 12.5%, equivalent to 240–300 kg N ha−1. Lucci et al. (Citation2013) reported a yield of kale/swede mix in Central North Island of 10 t DM ha−1 containing 220 kg N ha−1. Grazing in situ in winter means that a large proportion of this N will be returned as excreta on to bare soil at a time when rainfall generally exceeds evaporation and drainage occurs. Consequently, the N leaching risk from grazed winter forage crops can be large, making a disproportionately large contribution to annual N leaching despite representing a relatively small area of the farming system (Monaghan et al. Citation2007). Shepherd et al. (Citation2012) measured leaching of 130–170 kg N−1 from grazing a kale/swede crop. Malcolm et al. (Citation2016) using lysimeters showed that 21–32% of urinary N applied mid-late June could be leached over winter from bare soil in Canterbury.
Generally, lysimeter and grazing experiments studying N leaching tend to mimic a single grazing event, whereas in practice a farm will graze a winter forage block from approximately the start of June through to the end of July. This may have implications for N leaching losses, given that there will be less rainfall to displace urinary N from a late-grazed crop compared with crop grazed at the start of the winter. With later grazing, not all urinary N might be leached by the end of drainage, providing an opportunity for the succeeding crop/pasture to utilise this remaining mineral N (Nmin) before drainage in the following winter. This has been demonstrated with ‘catch crops’ (Carey et al. Citation2016) but may also apply to the following renewed pasture or another forage crop established in spring. The overall farm N loss will therefore be an average of losses from the different grazing times.
Shepherd et al. (Citation2012) argued that focusing measurements at the start of winter simulates a worst-case situation. We therefore aimed to test the hypothesis that urine deposited in late winter would leach less N, overall, than urine deposited at the start of winter because of the opportunity of a following crop to retain some of the soil Nmin before further leaching of any residual N in a second winter. A pasture site was used for comparison with the winter forage crop. At the time of the experiment, the nitrification inhibitor dicyandiamide (DCD) was still also available. This had previously been shown to decrease N leaching from grazed forage crops by 20–54% (Smith et al. Citation2008; Shepherd et al. Citation2012; Malcolm et al. Citation2015), although others have reported no significant effect on N leaching (Smith et al. Citation2012; Monaghan et al. Citation2013). We hypothesised that we were more likely to see a DCD effect with early than late grazing because of potentially less N leaching (and consequently less opportunity to express a DCD response).
Preliminary results from the experiment were previously reported by Carlson et al. (Citation2013). Here we extend these results to include second-year effects from the study where residual N leaching was measured (and so fully evaluating our original hypothesis). The aim of this short communication is to document the full set of findings from this 2-year study.
Materials and methods
Experiment site and treatment applications
The experiment was located on a dairy farm near Mangakino in the upper Waikato River catchment. The soil type was a freely draining Taupo sandy loam (Immature Orthic Pumice: Hewitt Citation2010). Adjacent paddocks of a mixed kale/swede winter brassica crop and ryegrass/white clover pasture were identified in April 2012 and fenced off to prevent stock access. The brassica forage crop had been drilled in the previous November following cultivation of a poorly performing pasture.
Thirty-two plots (1.5 × 1.5 m) were marked out in each paddock. Four porous ceramic cups (Webster et al. Citation1993) were installed in each plot to a depth of 600 mm at a 45° angle from the edge of the plot so that there was no soil disturbance in the plot. Simulated grazing treatments were applied ‘early’ (12 June) or ‘late’ (27 July) by manually removing the forage brassica crop, or by mowing the pasture plots and removing herbage, followed by an application of synthetic cow urine at an equivalent rate of 600 kg N ha−1. Half of the treated plots received DCD. The DCD applied was a granular zeolite coated DCD formulation (DCn™; 24% DCD) which was applied at a rate of 50 kg ha−1, or the equivalent of 12 kg active ingredient ha−1 immediately after ‘grazing’. The early-graze treatment received a second application six weeks after the first. The late-graze treatment received only one application.
To replicate farm practice, chicory was sown on to the bare forage crops in September (c. 3 kg/ha). Both the pasture plots and chicory were regularly trimmed to about 50 mm height and the herbage removed from the plots, but no further urine applied as the intention was to monitor N leaching from urine applications in the second winter after application.
Measurements
Soils were sampled for Nmin, the sum of ammonium-N (NH4-N) and nitrate-N (NO3-N) to 600 mm before each urine application (sampling dates 29 May and 27 July). Eight soil cores were bulked from around the outside of each block to avoid damage to the plots. At the end of the first winter drainage season soils from the plots themselves were sampled (four cores per plot, bulked). Soils were analysed for Nmin by sieving (4 mm) and then extracting with 2 M KCl, shaken for 2 hours and filtered (Mulvaney, Citation1996). The filtered soil extracts were analysed for NO3-N and NH4-N using the same method as for the leachates. Laboratory values (mg N kg−1 dry soil) were converted to kg N ha−1 using measured soil bulk density values for the site (0.71–0.8 t soil m−3).
Sampling of porous cups started on 22 June 2012 and continued until the end of the spring drainage period in October 2012. Sampling then resumed at the onset of drainage in May 2013. Soil solution was collected from the porous cups by applying a suction of ca. 60 kPa for four hours before removing all the collected soil solution. The target was to sample after every 50 mm drainage. In total, there were nine collections taken before drainage ceased in spring 2012 and a further five collections in winter 2013. The collected leachate samples were frozen for storage before being thawed for colorimetric analysis of NO3-N (Henriksen and Selmer-Olsen Citation1970) and NH4-N (Searle Citation1984) using a Skalar SAN++ segmented flow analyser (Skalar Analytical B.V., Breda, Netherlands).
Mineral N leaching fluxes were estimated from modelled drainage and soil solution Nmin concentrations using the method described by Lord and Shepherd (Citation1993). A soil water drainage model (Woodward et al. Citation2001) was used to estimate winter drainage. The model version used assumes a pasture cover when estimating actual evapotranspiration (AET) from potential evapotranspiration (PET). To estimate the effects of bare soil post grazing of the forage crop on AET and drainage, a conversion factor of 0.8 PET was used since this gave a good fit to measured soil moisture status on pasture with low crop cover reported by Woodward et al. (Citation2001). Climate inputs for the Woodward model were from NIWA’s Virtual Climate Station network (Tait et al. Citation2006) as shown in .
Table 1. Monthly rainfall and average monthly air temperature, compared with the 30-year (1980–2010) long-term average (LTA).
Statistical analysis
Experiments were designed as a 2 (early vs. late graze) x 2 (± DCD) factorial with eight replicates of each treatment in both paddocks, in a randomised block design. Data were analysed using the Genstat statistical package (release 18.1). Data were log transformed before analysis of variance where they deviated from a normal distribution.
Results
Weather and estimated drainage
The lead-in period into the experiment (January–May 2012) was slightly wetter (533 vs. 477 mm) and cooler (14.4°C vs. 15.1°C) than average (). May to October 2012 (the main drainage period) was also wetter and cooler than average (820 vs. 778 mm, 9.2°C vs. 9.6°C, respectively). The Woodward model estimated there was 405 mm drainage from pasture between application of the first treatment in early June and end of drainage in late October. The adjusted drainage to account for bare soil post grazing of the forage crop was 450 mm (early grazing) or 439 mm (late grazing). The amount of drainage after the late graze was estimated to be 222 mm (pasture) and 255 mm (forage crop), i.e. about 185 mm less drainage than from the early grazing.
The late spring and summer 2012/2013 period was much drier than average (338 vs. 581 mm, November–April) (), with no drainage over this period. The model estimated that drainage restarted in mid-May 2013, with 536 mm up to our last porous cup sampling at the end of August 2013. Over the duration of the experiment, estimated total drainage was 942, 986 and 975 mm for the pasture, early-grazed and late-grazed forage crop treatments, respectively.
Mineral N concentrations in soil solution
Mineral N concentrations at each porous cup sampling date were variable within a plot and between plots of the same treatment, as denoted by the error bars in and . Mineral N in the soil solution sampled at 600 mm depth was mainly in the form of NO3-N at both sites. Ammonium-N concentrations peaked at 5 mg N L−1 compared with 80 mg N L−1 for nitrate-N. There was an indication of more ammonium-N moving to depth at the pasture site, peaking at 16 mg N L−1 compared with 100 mg N L−1 for nitrate-N.
Figure 1. Soil solution ammonium-N (NH4-N) and nitrate-N (NO3-N) concentrations during winters 2012 and 2013, after simulation of forage crop grazing in winter 2012. The break in plotted lines denotes the spring/summer of 2012/2013. Treatments denote June or July applied urine, with or without dicyandiamide (DCD) application. Error bars represent the standard error of the mean.
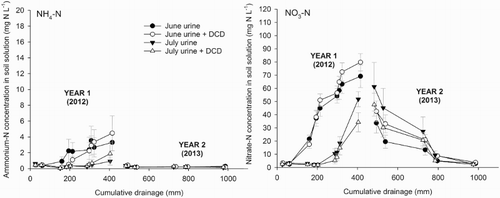
Figure 2. Soil solution ammonium-N (NH4-N) and nitrate-N (NO3-N) concentrations during winters 2012 and 2013 after simulation of pasture grazing in winter 2012. The break in plotted lines denotes the spring/summer of 2012/2013. Treatments denote June or July applied urine, with or without dicyandiamide (DCD) application. Error bars represent the standard error of the mean.
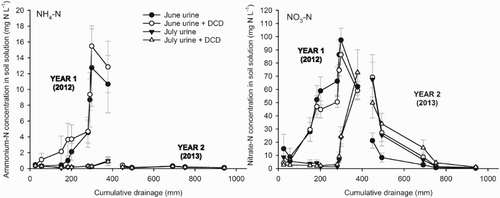
Ex-forage crop site
At the ex-forage crop site, concentrations of NH4-N peaked at 3–4 mg N L−1 at the end of drainage in the first year, compared with 70–80 mg NO3-N L−1 from the early urine application (). In the late-grazed treatment, NO3-N concentrations had not reached the same levels as for the early urine application by the end of drainage in 2012. There was no consistent effect of DCD on N concentrations.
At the start of drainage in 2013, NH4-N concentrations were less than 1 mg N L−1 in soil solution, indicating that any remaining NH4-N had been nitrified during summer. Concentrations of NO3-N in the late urine treatment peaked at c. 50–60 mg N L−1 and returned to background levels by the end of measurements in August 2013.
Pasture site
Mineral N concentrations followed similar trends in the pasture plots (). Ammonium concentrations from the early application peaked at 13–16 mg N L−1, but were highly variable between replicates but only reached 1 mg N L−1 for the late application; all NH4-N concentrations returned to background levels by winter 2013. Peak NO3-N concentrations were 90–100 mg N L−1 for early-applied urine and 70–80 mg N L−1 for late-applied urine; they returned to background levels by the end of the experiment.
Soil mineral N
Soil Nmin levels to 600 mm depth just prior to urine application under the forage crop were 18 ± 2.5 kg N ha−1 (early June treatment) and 20 ± 1.6 kg N ha−1 (late July treatment). Values for pasture were similar: 22 ± 3.6 kg N ha−1 (early June treatment) and 20 ± 2.2 kg N ha−1 (late July treatment).
The effect of urine timing on residual soil Nmin at the end of the first winter, and its position in the soil profile, is demonstrated in . Approximately 300 kg N ha−1 remained after the late urine application to the forage crop compared with c. 170 kg N ha−1 from the early application (P < .001). Over half of the Nmin was in the 0–300 mm layer for the late application compared with about one third for the early application. Although still apparent at the pasture site with more Nmin remaining from the late application (P < .05, ), the effects were not as large as for the forage crop site. This may have been because of the added effect of pasture N uptake removing some of the soil Nmin. At both sites, soil Nmin was still present as NH4-N (71 kg N ha−1, no significant treatment effect), suggesting that not all of the synthetic urine had been nitrified by the end of winter.
Table 2. Measured soil mineral N content (kg N ha−1) at the end of drainage, 2012.
Soil Nmin at the end of drainage in 2012 responded differently to DCD application at the two sites (). After the forage crop, there was a significant interaction of urine deposition time and DCD on soil Nmin (P < .05). This was seen at all individual depths and in the total (0–600 mm). DCD increased total soil Nmin contents (209 vs. 131 kg N ha−1) after the early urine application; for the late application Nmin levels were similar (308 vs. 295 kg N ha−1). In contrast, soil Nmin measured at the pasture site showed no consistent response to DCD.
Estimated N leaching losses
Estimates of Nmin leaching fluxes for year 1, residual effects in year 2 and a total of both years are presented in . Time of urine application was the only factor that had a significant effect on Nmin leaching. Leaching fluxes from year 1 were remarkably similar for both sites despite one site being bare soil and the other pasture: c. 185 vs. 60 kg N ha−1 for early and late urine applications, respectively. There was leaching in the second winter of some of the soil Nmin remaining at the end of the first drainage season. At the pasture site there was More Nmin leached from the later application in year 2 (82 vs. 54 kg N ha−1, P < .05). There appeared to be a similar trend at the crop site (118 vs. 84 kg N ha−1) but it was not statistically significant. In total, an amount of N equivalent to 40–45% of that applied was leached from the early urine application (). There was 35–40% less N leaching from late-applied urine compared with the early application. However, DCD effects on amounts of N leached did not show any significant effects after the first winter, compared the soil Nmin measurements.
Table 3. Estimated leaching of mineral N (kg N ha−1).
Discussion
The experiment clearly showed that later-applied urine N is at less risk of leaching due to less subsequent rainfall and leaching in the first winter. Shepherd et al. (Citation2012) measured a large residual pool of soil Nmin in the spring after grazing a brassica crop in early June and argued that it would be necessary to capture as much of this mineral N, otherwise it would leach in the following winter. The current experiment confirms that neither pasture nor a planted chicory crop was able to extract all of the residual Nmin and there was a substantial amount of N leaching in the second winter.
The results stress the need to capture as much of the residual Nmin as possible. However, these results have to be set partly in the context of the following summer, being much drier than average (although we did not measure yields). Also, the chicory was not sown until 10 October. Establishing a catch crop earlier, thereby maximising the period of adequate soil moisture for growth, may be of benefit (Carey et al. Citation2016). Although we did not measure yield and N recovery, we investigated first-year effects by calculating a partial N balance at the end of drainage (). The sum of estimated Nmin leaching and remaining soil Nmin was the same for the early- and late-grazed forage crop (c. 350 kg N ha−1). This was similar to the N balance for early urine applied to pasture (330 kg N ha−1) but the N balance for late urine on pasture was markedly less (260 kg N ha−1), suggesting removal of N in spring growth.
Table 4. A partial nitrogen (N) balance at the end of drainage 2012 based on measured N leached and soil mineral N.
The amounts of N leached in the first winter were similar from pasture and crop/bare soil. Pasture growth rate in the Central Plateau region is slow in winter (Baars et al. Citation1975) and, because of this small sink for N, our results suggest that a single urine patch deposited in early June to either bare soil or pasture is at equal risk of leaching. The main difference between the two systems, however, is that a single grazing of pasture in June may return less than 30 kg N ha−1 based on a typical pasture mass consumed of 1 t DM ha−1 and 3% N, compared with more than 200 kg N ha−1 consumed by a mob eating a high-yielding standing crop.
One of our hypotheses was that there would be a significant effect of urine deposition time on the effectiveness of DCD on mineral N leaching from early urine deposition but not from late deposition. McDowell and Houlbrooke (Citation2009) and Malcolm et al. (Citation2015) measured reductions in N leaching of 40–54% in lysimeter studies that simulated urine deposition post grazing of a forage crop in May–June. Soil Nmin measurements showed a urine timing effect on N leaching risk after the forage crop (), but this was not detected in the soil solution sampling (). One possibility is that the variability in measured soil solution concentrations between samplers was such that the power of the experiment was insufficient to demonstrate a difference in DCD effectiveness, compared with a lysimeter methodology. The evidence from soil Nmin measurements suggest that interventions using process inhibitors to reduce N leaching are most effectively targeted at early winter grazings.
Acknowledgements
We thank John Waller and Maryann Pirie for statistical advice, and Wairarapa Moana Incorporation for hosting the experiment sites.
Disclosure statement
No potential conflict of interest was reported by the authors.
Additional information
Funding
References
- Baars JA, Radcliffe JE, Brunswick L. 1975. Seasonal distribution of pasture production in New Zealand. 6. Wairakei, pasture and lucerne production. New Zeal J Exp Agr. 3:253–258. doi: 10.1080/03015521.1975.10425812
- Carey PL, Cameron KC, Di HJ, Edwards GR, Chapman DF, Aitkenhead M. 2016. Sowing a winter catch crop can reduce nitrate leaching losses from winter-applied urine under simulated forage grazing: a lysimeter study. Soil Use Manage. 32:329–337. doi: 10.1111/sum.12276
- Carlson B, Lucci G, Shepherd M. 2013. Simulated early and late winter grazing: effects on nitrogen leaching on pumice soils. Accurate and efficient use of nutrients on farms. Currie LD, Christensen CL, editors. Palmerston North: Fertilizer and Lime Research Centre, Massey University. Occasional Report No. 26.
- Edwards G, de Ruiter J, Dalley D, Pinxterhuis J, Cameron K, Bryant R DIH, Malcolm B, Chapman D. 2014. Dry matter intake and body condition score change of dairy cows grazing fodder beet, kale and kale-oat forage systems in winter. Proc. New Zeal Grass Assoc. 76:81–88.
- Henriksen A, Selmer-Olsen AR. 1970. Automatic methods for determining nitrate and nitrite in water and soil extracts. Analyst. 95:514–518. doi: 10.1039/an9709500514
- Hewitt AE. 2010. New Zealand soil classification. Landcare research science series. Lincoln (NZ): Manaaki Whenua Press, Landcare Research.
- Lord EI, Shepherd MA. 1993. Developments in the use of porous ceramic cups for measuring nitrate leaching. J Soil Sci. 44:435–449. doi: 10.1111/j.1365-2389.1993.tb00466.x
- Lucci G, Shepherd M, Carlson B, Lane M, Berry I. 2013. Establishment of winter forage crops on pumice soils–experimental results and farmers’ perspectives. Accurate and efficient use of nutrients on farms. Currie LD, Christensen CL, editors. Palmerston North: Fertilizer and Lime Research Centre, Massey University. Occasional Report No. 26.
- Malcolm B, Cameron K, Edwards G, Di H. 2015. Nitrogen leaching losses from lysimeters containing winter kale: the effects of urinary N rate and DCD application. New Zeal J Agr Res. 58:13–25. doi: 10.1080/00288233.2014.961644
- Malcolm B, Cameron K, Edwards G, Di H, de Ruiter J, Dalley D. 2016. Nitrate leaching losses from lysimeters simulating winter grazing of fodder beet by dairy cows. New Zeal J Agr Res. 59:194–203. doi: 10.1080/00288233.2016.1150307
- McDowell RW, Houlbrooke DJ. 2009. The effect of DCD on nitrate leaching losses from a winter forage crop receiving applications of sheep or cattle urine. Proc. New Zeal Grass Assoc. 71:117–120.
- Monaghan R, Wilcock R, Smith L, Tikkisetty B, Thorrold B, Costall D. 2007. Linkages between land management activities and water quality in an intensively farmed catchment in southern New Zealand. Agr Ecosyst Environ. 118:211–222. doi: 10.1016/j.agee.2006.05.016
- Monaghan RM, Smith LC, de Klein CAM. 2013. The effectiveness of the nitrification inhibitor dicyandiamide (DCD) in reducing nitrate leaching and nitrous oxide emissions from a grazed winter forage crop in southern New Zealand. Agr Ecosyst Environ. 175:29–38. doi: 10.1016/j.agee.2013.04.019
- Mulvaney RL. 1996. Nitrogen – inorganic forms. In: Sparks DL, editor. Methods of soil analysis: part 3. SSSA book series 5. Madison, WI: SSSA; p. 1123–1184.
- Searle PL. 1984. The Berthelot or indophenol reaction and its use in the analytical chemistry of nitrogen: a review. Analyst. 109:549–568. doi: 10.1039/an9840900549
- Shepherd MA, Stafford A, Smeaton D. 2012. The use of a nitrification inhibitor DCn™ to reduce nitrate leaching under a winter-grazed forage crop in the central plateau. Proc. New Zeal Grass Assoc. 74:103–108.
- Smith LC, De Klein CAM, Monaghan RM, Catto WD. 2008. The effectiveness of dicyandiamide in reducing nitrous oxide emissions from a cattle-grazed, winter forage crop in southland, New Zealand. An Prod Sci. 48:160–164.
- Smith LC, Orchiston T, Monaghan RM. 2012. The effectiveness of the nitrification inhibitor dicyandiamide (DCD) for mitigating nitrogen leaching losses from a winter grazed forage crop on a free draining soil in Northern Southland. Proc New Zeal Grass Assoc. 74:39–44.
- Tait A, Henderson R, Turner R, Zheng X. 2006. Thin plate smoothing spline interpolation of daily rainfall for New Zealand using a climatological rainfall surface. Int J Climatol. 26:2097–2115. doi: 10.1002/joc.1350
- Webster CP, Shepherd MA, Goulding KWT, Lord EI. 1993. Comparisons of methods for measuring the leaching of mineral nitrogen from arable land. J Soil Sci. 44:49–62. doi: 10.1111/j.1365-2389.1993.tb00433.x
- Woodward S, Barker DJ, Zyskowski R. 2001. A practical model for predicting soil water deficit in New Zealand pastures. New Zeal J Agr Res. 44:91–109. doi: 10.1080/00288233.2001.9513464