ABSTRACT
The efficacy of a direct-fired, tractor-mounted steam weeder was compared with that of a flame weeder. At a tractor speed of 3 km h−1, steam killed 100% of 10-day-old mustard plants, sown as a surrogate weed, and flame killed 92%. Plant survival increased with increasing tractor speed, but was always lower for the steam weeder. Mean survival over all speeds was 40% for steam and 74% for flame. By contrast, for 15 agricultural weed species, at a tractor speed of 3 km h−1 weed survival for both weeders was similar. At 3 km h−1 both methods killed Stellaria media and Veronica agrestis at all growth stages assessed, but neither killed Lolium perenne. Plants of all the other species were killed when the treatments were applied soon after emergence (cotyledon stage), but as the plants increased in size they became more resistant to heat, either because the terminal or axillary meristems were protected by soil, or because meristems were protected by thickened stems and leaves as the plants aged.
Introduction
Globally, agriculture is facing numerous challenges in terms of providing multiple and often conflicting ecosystem services (Wratten et al. Citation2013). Effective weed management is critical for provisioning services but it can negatively impact other services such as supporting and regulating services, particularly where herbicides are used (Sandhu et al. Citation2015). Weeds can be a major problem in conventional and organic crop production systems. In the former the extensive and repeated use of herbicides has led to herbicide-resistant weeds (Owen and Zelaya Citation2005) and concerns about contamination of drinking water and food. These problems have led some countries to develop policies mandating reductions of herbicide use (Knezevic et al. Citation2014). In organic systems, the problem of controlling weeds without herbicides is challenging (Kruidhof et al. Citation2008) and therefore alternative weed control methods need further evaluation (Ulloa et al. Citation2010a).
Heat has long been used as a method to control weeds, pests and diseases (Merfield et al. Citation2009). The burning of crop stubbles after-harvest can be an efficient control measure for shed weed seeds and for some stubble-borne pests and diseases (Hardison Citation1976), but in many countries environmental concerns over smoke pollution have stopped this practice (Estrellan and Iino Citation2010). Flame weeding is one alternative to chemical weed control (Datta and Knezevic Citation2013). It controls weeds primarily by rupturing plant cell membranes that leads to subsequent tissue desiccation (Lague et al. Citation2001). However, this method is a very energy inefficient process (Kerpauskas et al. Citation2006), cannot be used on windy days and can be a fire risk (Merfield et al. Citation2009).
Steam is superior to flame as a means of heat transfer to plants because of the considerably larger latent heat of condensation/vaporisation of water compared with the specific heat of dry air (Merfield et al. Citation2009). Sirvgdas et al. (Citation2002) noted that a further advantage of steam was that its lower temperature and high moisture content results in less heat loss via evaporation and/or transpiration compared with open flames. However, producing sufficient quantities of steam on tractor-mounted equipment is problematic because the standard design of pressurised steam boilers makes them ill-suited for agricultural use due to the inherent hazard of super-heated water which requires multiple safety requirements (Malek Citation2004). Merfield et al. (Citation2009) described a ‘direct-fired’ steam weeder which is able to meet the dual requirements of (i) providing steam at or above 100°C (depending on the amount of water injected) in sufficient quantity or with an energy content equivalent to existing flame weeders, and (ii) being sufficiently robust, mechanically simple and with sufficiently few safety hazards such that it would be suitable for agricultural use.
In this paper we report on a comparison of the abilities of the steam weeder (Merfield et al. Citation2009) and the Lincoln University flame weeder to control common agricultural weeds.
Materials and methods
Steam and flame weeders
The steam weeder (Merfield et al. Citation2009) ( and ) was powered by an Ecoflam burner (Ecoflam Bruciatori S.p.A., Italy) with an output of 266 kW and fuel consumption of 20.8 kg diesel h−1. The burner is mounted in the centre of one end of a cylindrical combustion chamber made of 3 mm thick mild steel plate with a diameter of 500 mm, length of 700 mm and lined with 50 mm ceramic fibre insulation. The end of the combustion cylinder opposite the burner opens into an un-insulated cylindrical water injection duct that tapers from 500 to 300 mm internal diameter. At the point of transition between the combustion chamber and the water injection duct are three, brass, size 10, TX small capacity hollow cone nozzles spaced 200 mm apart, positioned symmetrically around the long axis of the combustion chamber/water injection duct and spraying away from the combustion chamber. A pump supplies water at 10 bar to the nozzles which have a combined flow rate of 3.5 L min−1. The narrow end of the water injection duct joins at 90° to the centre of a second (steam distribution) duct with a triangular cross section with all sides being 320 mm and a length of 1700 mm. One corner of the steam distribution duct points downwards and has been cut off to make a slot 15 mm wide along the full length of the duct. The burner, combustion chamber, water injection duct and steam distribution duct are mounted on top of a hood into which the steam is introduced at the front, via the slot in the steam distribution duct. The hood is 2.4 m long, 1.750 m wide and the internal height can be adjusted from 100 to 250 mm to accommodate different bed heights. In these experiments the height was 100 mm. It has flaps front and the back to minimise wind ingress and a chimney 100 mm across by 1700 mm wide and 500 mm high on the top of the hood at the rear. The hood assembly is slung under a tool frame on which are mounted water and diesel tanks and an electrical generator to supply 240v AC power to the burner.
Figure 1. Prototype direct-fired steam weeder (Merfield et al. Citation2009). Parts: (1) Ecoflam burner, (2) combustion chamber, (3) water injection duct, (4) water pump, (5) steam distribution duct, (6) hood, (7) front and rear skids, (8) chimney, (9) tool frame, (10) water tank, (11) diesel tank. The electrical generator is not visible as it is on the far side of the water tank.
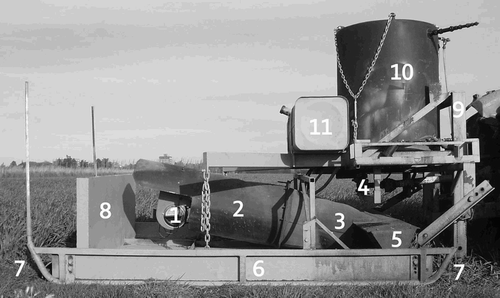
Figure 2. Schematic diagram of the direct-fired steam weeder boiler and steam application system (Merfield et al. Citation2009). Top, plan view of direct-fired steam boiler; bottom, side view of steam distribution duct and hood, aligned with the plan view. Parts: numbering is consistent with , (1) Ecoflam burner, (2) combustion chamber with ceramic wool insulation, (3) water injection duct, (5) steam distribution duct, (6) hood, (8) chimney, (12) flame from burner, (13) water nozzle and water spray, (14) slot in bottom of steam distribution duct, (15) flaps, (16) soil surface and weed seedlings. Arrows indicate direction of hot gas and steam mixture. Steam is created by the hot gasses produced by the burner (1 and 12) vaporising the fine spray of water from the water nozzle (13), which are then carried by the flow of gas from the burner to the steam distribution duct (5, both diagrams), through the slot in the bottom of the duct (14) to the underneath of the hood (6) where it heats the weeds, before exiting via the chimney (8). Flaps (15) at the front and rear are to reduce the amount of wind blowing under the hood and increase steam retention.
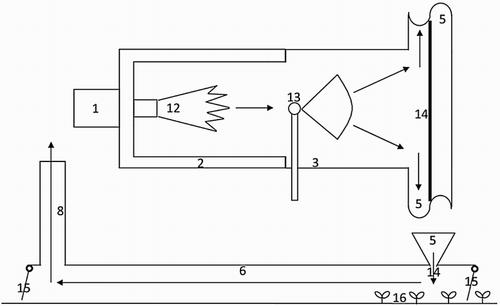
The Lincoln University flame weeder (de Rooy Citation1992, ) was based on a Hoaf KBL 1.5 (Hoaf Infrared Technology, Netherlands) but the burners were redesigned to achieve a 23% increase in efficiency (de Rooy Citation1992). The burners are positioned along the leading edge of a hood 200 mm high, 1400 mm wide and 1200 mm long and lined with 50 mm ceramic fibre insulation. The front of the hood was open while the back of the hood had baffles to reduce wind ingress. LPG was supplied at 2 bar to the burners, which are angled at 45° from the horizontal and point backwards. The flame base is 160 mm from the ground and the flame extends to the soil surface. The flame weeder used 17.5 kg LPG h−1, which has an energy content of 243 kW.
Figure 3. The Lincoln University flame weeder (de Rooy Citation1992).
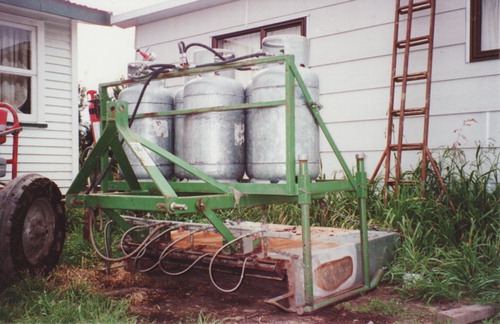
Dose-response of mustard (Experiment 1)
For this experiment mustard (Brassica juncea (L.)) was used as a quick growing surrogate weed. Seeds were sown in 1500 mm wide beds with a Stanhay Mk II belt seeder in four single rows 300 mm apart with an intra-row spacing of 2.5 mm and sowing depth of 20 mm. Each plot consisted of a 15 m length of bed with a 20 m inter-plot area for adjusting speed and positioning the weeders. The trial was a two-factorial randomised complete block design with four replicates and energy ‘dose’ (kW ha−1) and weeder type as factors. Energy dose was altered by varying the weeder’s forward speed to provide 3, 4, 5, 6 and 7 km h−1. These speeds resulted in dose rates of 507, 380, 304, 253 and 217 kW ha−1 for the steam weeder and 579, 434, 347, 289 and 248 kW ha−1 for the flame weeder. Four untreated plots served as control replicates. Treatments were applied between 06:10 and 10:20 h 10 days after sowing when the plants were between the cotyledon and two leaf stage. The trial was conducted on a stone-free Templeton silt loam soil situated at Leeston, Canterbury, New Zealand (43°48′09.21″ S 172°18′09.61″ E). The air temperature on the morning of treatment rose from 4°C to 9°C and there was no wind. Twenty-nine hours after treatment the number of surviving mustard plants was counted in five randomly selected 500 mm lengths of sown row in each plot. Data from these five sub-samples were combined to create a single count for each plot and these values were then divided by the mean of the counts for the four control plots to provide a proportion of survival. Analysis was by ANOVA on untransformed data as this was normally distributed. The results are presented as the number of plants surviving as a proportion of the control.
Weed species comparison (Experiment 2)
Ten seeds of Amaranthus retroflexus L., Chenopodium album L., Capsella bursa-pastoris L., Daucus carota L., Fumaria officinalis L., Lolium perenne L., Polygonum aviculare L., Rumex crispus L., Spergula arvensis L., Stellaria media Vill., Solanum sarrachoides Sendtner, Trifolium repens L. and Veronica agrestis L. were each sown into polyethylene bags (70 × 70 × 120 mm) containing a commercial potting mix. The bags were placed outside at the nursery and hand watered as required. After emergence, plants were thinned to five per bag. For each plant species a total of 50 sets of 5 plants/bag were established to allow for treatment at each of 5 growth stages. These were cotyledons and 2, 4, 6 and 8 true leaves (10 bags/species/growth stage; 5 for each weeder type).
In a bare field at Lincoln University, 150 mm lengths of plastic pipe, into which the bags fitted tightly, were buried in the soil, level with the soil surface, allowing the bags of weeds to be easily inserted and removed from the soil. Each of 5 blocks consisted of 10 pipes, arranged in 2 groups of 5, placed in a line across the middle of 2 tractor beds. The trial design was therefore a randomised complete block split-plot with weeder type and plant species as the two factors and growth stage as the sub-plot.
At each growth stage, one randomly selected bag of each species was placed into its pipe and the weeders were driven over the plants at 3 km h−1 to apply the treatment. The latter were applied before 10:00 h and at a wind speed of <5 km h−1. After applying the treatments the bags were returned outdoors to the nursery and kept watered. Plant survival was assessed three weeks after treatment by counting the number of live plants per bag. Data were analysed by ANOVA with a comparison of means by least significant difference. For species which had 0% (S. media and V. agrestis) or 100% (L. perenne) survival at any growth stage for both weeders, the ANOVA was completed with these data excluded.
Results
Dose-response of mustard (Experiment 1)
There was a significant difference between weeder types (P < .001) with a mean mustard survival rate of 40% for steam and 74% for the flame weeder (). Survival of mustard plants increased with increasing tractor speed (P < .001) and there was a significant interaction (P < .001) between weeder type and speed (), because while plant survival increased with increasing tractor speed, survival was always lower for the steam weeder.
Table 1. The effect of steam and flame weeding at five tractor speeds on the survival of mustard plants between cotyledon and two true leaf stage (expressed as a proportion of the control) (Experiment 1).
Weed species comparison (Experiment 2)
L. perenne survived both flaming and steaming at all growth stages while S. media and V. agrestis were killed at all growth stages. For the other 10 species there was a significant difference in survival among growth stages (P < .001), with all plants killed at the cotyledon stage (or equivalent stage for non-cotyledonous species) and most at the second growth stage (). As plants grew, they became more resistant to both flame and steam, although this differed among species. For example, no plants survived at the third growth stage for P. aviculare and T. repens, but survival at the same growth stage for the other species ranged from 12% to 60% (). For most species there were no significant differences between flame and steam weeding, the exceptions being for A. retroflexus, S. arvensis and S. sarrachoides at the fourth growth stage and D. carota at the third growth stage where steam weeding killed more plants than flame weeding, and for T. repens at the fourth growth stage where the reverse occurred ().
Table 2. Effect of flame and steam weeding at 3 km h−1, at different growth stages on plant survival (Experiment 2).
Discussion
Dose-response of mustard (Experiment 1)
The lower mustard survival achieved by the steam weeder, despite its having a lower heat output, supports the hypothesis that steam is an effective alternative to flame for weed control. However, it is not just the thermal dose that determines effectiveness, as the machines’ design, especially of the hood, can significantly alter effectiveness (de Rooy Citation1992). The Lincoln flame weeder had an internal hood height of 200 mm and length of 1200 mm while the steam weeder hood height was 100 mm and was 2500 mm long (Merfield et al. Citation2009). de Rooy (Citation1992) showed that hood height and length had a clear impact on weeder performance. In addition, the Lincoln flame weeder hood was open at the front, while the steam weeder had baffles front and back. Palmer (Citation1996) showed that under-hood temperature decreased as forward speed increased for the Lincoln flame weeder. The steam weeder hood design, being twice as long, half the height and with both front and rear baffles, is considered likely to have also contributed to its improved performance. The results of this comparison should not therefore be over interpreted as demonstrating a definitive performance difference between flame and steam for weed control. Ideally, a future comparison should be made where the two weeders have identical hood designs and heat outputs.
For both weeders there was a rapidly decreasing weed kill with increasing speed/reducing energy dose/unit area. This is as expected, and is in agreement with previous reports (Ascard Citation1994, Citation1997; Peruzzi and Raffaelli Citation1999; Juroszek et al. Citation2002).
Weed species comparison (Experiment 2)
Although there were significant differences between the two weeders in Experiment 1, Experiment 2 mostly showed that the weeders had a similar performance. One explanation for this discrepancy is that the performance differences between the weeders in the dose-response experiment were not significant at 3 km h−1 (which is the speed used in the weed survival experiment) while the largest differences in comparative performance were at 4 and 5 km h−1. The 3 km h−1 performance may have been similar because at that speed, both weeders applied more heat than was required for weed mortality.
Different survival patterns among weed and crop species as plant size increases have been reported by a number of other researchers (Ascard Citation1995; Vanhala and Rahkonen Citation1996; Sartorato et al. Citation2006; Wszelaki et al. Citation2007; Domingues et al. Citation2008; Ulloa et al. Citation2010a; Datta & Knezevic Citation2013; Knezevic et al. Citation2014). The differing plant responses can be viewed as of three types: (1) highly susceptible at all growth stages, e.g. S. media; (2) initially highly susceptible but survival rapidly increases with increasing plant size, e.g. R. crispus and (3) highly resistant at all growth stages, e.g. L. perenne. The cause of this difference was first hypothesised by Ascard (Citation1995) who suggested that upright weeds are more susceptible to flame weeders than prostrate ones and that thin leaves and unprotected growing points have higher susceptibilities. Researchers have expanded this to hypothesise that the key to weed and crop plant mortality and/or longer term biomass reduction is the ability of the heat to kill aerial meristems (Ascard Citation1998; Cisneros and Zandstra Citation2008; Sivesind et al. Citation2009; Ulloa et al. Citation2010a, Citation2010b; Knezevic et al. Citation2014). If the heat destroys only the plant’s leaves, then replacement leaves can be quickly produced from the meristems at relatively low ‘cost’ to the plant. However, if the heat kills meristems, these can only be replaced by new growth from unharmed meristems lower on the plant, which is more costly for the plant. If all the aerial meristems are destroyed then, unless the plant can regenerate foliage from the roots by dedifferentiation, the whole plant will be killed. In addition, the cambium layer round the stem is also a meristem and the phloem cells, unlike xylem, are living and need to be alive to transport water and nutrients. If the cambium or phloem cells are destroyed in the hypocotyl region of dicotyledonous plants they will also be killed if they are unable to regenerate from the roots. This can be considered akin to ring barking or steam-girdling (Parrott et al. Citation2007).
Different plant sizes and morphologies mean that plants vary in how exposed the meristems are to heat and the number that need to be killed to achieve complete plant death. For dicotyledonous plants at the cotyledon stage there is only one aerial meristem which is positioned in the cotyledon leaf axils. It is highly exposed due to the small size of the plant and is typically clear of the soil surface. As the plant grows the number of meristems increases, and in many plants they become subsumed within the leaf axil. This, coupled with the leaves becoming larger, offers greater protection to the meristems. In monocotyledonous plants, the growing point is typically at the base of the plant in the centre of the leaf sheaths, which is often below soil level, even for plants at the cotyledon stage, so the meristem is very well protected from heat.
Of the weeds tested within this experiment, only one, a monocotyledon, the grass, L. perenne had 100% survival at all growth stages, with one exception for steam at two true leaves with 84% survival. The meristem of L. perenne is at the base of the plant within the soil so was well protected from the heat. It is therefore considered a type 3 plant, using the above categories.
At the other extreme, S. media and V. agrestis had zero survival at all growth stages, except V. agrestis with 4% at four true leaves and 8% at eight true leaves for flame, and are considered type 1 plants. Both are sprawling, with narrow stems and small leaves, which are considered to offer little protection to either the meristems in the leaf axils or the cambium and phloem. Even at the largest plant sizes tested in this experiment, flames and steam were able to penetrate the entire plant canopy including the hypocotyl region as evidenced by all green tissue rapidly dying after treatment. To some extent this contradicts Ascard (Citation1995) who suggested ‘that upright weeds are more susceptible than prostrate ones’. However, at early growth stages they had a more upright growth habit and they have narrow stems and small leaves which is in agreement with Ascard’s view that ‘thin leaves and unprotected growing points increase susceptibility’. P. aviculare was also completely susceptible up to eight true leaves when it had 20% and 28% survival. While the mature plant forms a flat mat of horizontal stems with a thick central stem which is considered to make it highly resistant to thermal treatment, the young plants had an upright morphology along with narrow stems and comparatively small leaves which meant their meristems and cambium were susceptible to both steam and flame.
In-between these two extremes, A. retroflexus, C. album and S. sarrachoides reflected ‘typical’ type 2 plants in that they were completely heat susceptible at the cotyledon and two true leaves stages, but then quickly became highly resistant through the four and six/eight leaf stages. All three species show similar morphological changes over these growth stages with the stem quickly increasing in thickness, and the leaves enlarging. When treated at the largest growth stage the leaf blades were destroyed but the bottom of the petiole and stem including the meristem in the axil was unharmed. Such plants then grew new leaves without difficulty. At intermediate stages the top part of the stem including the apical meristem was destroyed, but lower meristems survived and allowed the plant to regrow.
In comparison, F. officinalis, S. arvensis, C. bursa-pastoris and D. carota became resistant and survived thermal treatment because the young plant formed a rosette or the leaves all emerged as a dense cluster without a stem, such that the meristems were protected by the leaf bases. R. crispus became completely tolerant to heat treatment as it grew because it not only had a rosette morphology, but the stem also contracted, pulling the apical meristem underground. T. repens was similar to F. officinalis, S. arvensis, C. bursa-pastoris and D. carota in that the leaves all emerged from a greatly shortened stem, but at the largest size, the plants had formed a canopy of the leaves at the end of long petioles about 8 cm above the ground. These leaves remained turgid for a few tens of seconds after flaming, so they may have protected the stems, and the meristems within them, from the flames and steam by acting as a barrier.
These results are considered therefore to support the hypothesis that to kill both weeds and crop plants, the thermal treatment has to kill the aerial meristems not just defoliate them.
In conclusion, these experiments show that (i) steam is a viable, and potentially more effective, alternative to open flame for thermal weeding; (ii) for any given weed there is a clear relationship between the dose/weeder speed and weed survival, with survival rapidly increasing with decreasing dose/increasing speed and (iii) a plant’s response to thermal treatment is determined by how well its morphology protects its aerial meristems.
For some weeds at small growth stages flame and steam can provide an alternative to the use of herbicides for weed control. Plant morphology and how that does or does not protect the aerial meristems is considered the key to a plant’s susceptibility or resistance to foliar thermal treatment. The large variation in thermal susceptibility among plant species further allows for selective thermal weeding, such as the control of dicotyledonous weeds in monocotyledonous crops such as onion (Allium cepa) (Dastgheib et al. Citation2010). Current energy use by thermal weeders is clearly considerable and efficiency need to be improved. Non-fossil fuels are also required. For example, flame and steam weeders can be fuelled by methane (‘bio-gas’) produced by on-farm bio-digesters, and steam weeders can be fuelled by renewable diesel, bio-diesel and unrefined vegetable oil (Merfield et al. Citation2009). Such improved thermal weeders can therefore provide another option in the global challenges agriculture faces.
Acknowledgements
The authors thank: Tim and Rose Chamberlain, Harts Creek Farm for use of their farm and equipment; the Biological Husbandry Unit (BHU) Organics Trust for the inaugural ‘Lincoln University BHU Organics Trust Doctoral Scholarship’ and use of land and equipment; Ivan Barnett of the BHU for technical assistance; Alistair Morrow of Morrow Engineering Ltd., and Geoff, Alan and Dave of Geoff Hampton Engineering Ltd. for building and advice on the steam weeder; Paul Mesman of Wool Research Organisation of New Zealand (WRONZ) for his work on the initial steam boiler design parameters; Dr Alison Lister for statistical support and the staff of the Lincoln University nursery for looking after the weeds.
Disclosure statement
No potential conflict of interest was reported by the authors.
Additional information
Funding
References
- Ascard J. 1994. Dose-response models for flame weeding in relation to plant size and density. Weed Research. 34:377–385. doi: 10.1111/j.1365-3180.1994.tb02007.x
- Ascard J. 1995. Effects of flame weeding on weed species at different developmental stages. Weed Research. 35:397–411. doi: 10.1111/j.1365-3180.1995.tb01636.x
- Ascard J. 1997. Flame weeding: effects of fuel pressure and tandem burners. Weed Research. 37:77–86. doi: 10.1046/j.1365-3180.1996.d01-5.x
- Ascard J. 1998. Comparison of flaming and infrared radiation techniques for thermal weed control. Weed Research. 38:69–76. doi: 10.1046/j.1365-3180.1998.00073.x
- Cisneros JJ, Zandstra BH. 2008. Flame weeding effects on several weed species. Weed Technology. 22(2):290–295. doi: 10.1614/WT-07-113.1
- Dastgheib F, Merfield CN, Chamberlain TP. 2010. Post-emergence thermal weeding in onions (Allium cepa). Agronomy New Zealand. 40:177–186.
- Datta A, Knezevic SJ. 2013. Flaming as an alternative weed control method for conventional and organic agronomic crop productions systems: a review. Advances in Agronomy. 118:399–428. doi: 10.1016/B978-0-12-405942-9.00006-2
- de Rooy SC. 1992. Improved efficiencies in flame weeding [Unpublished thesis]. Canterbury: Lincoln University. 87 p.
- Domingues AC, Ulloa SM, Datta A, Knezevic SZ. 2008. Weed response to broadcast flaming. RURALS: Review of Undergraduate Research in Agricultural and Life Sciences. 3(1): Article 2.
- Estrellan CR, Iino F. 2010. Toxic emissions from open burning. Chemosphere. 80(3):193–207. doi: 10.1016/j.chemosphere.2010.03.057
- Hardison JR. 1976. Fire and flame for plant disease control. Annual Review of Phytopathology. 14:355–379. doi: 10.1146/annurev.py.14.090176.002035
- Juroszek P, Berg M, Lukashyk P, Köpke U. 2002. Thermal control of Vicia hirsuta and Vicia tetrasperma in winter cereals. In: Bàrberi P, Cloutier DC, editors. 5th EWRS workshop on physical and cultural weed control, Pisa, Italy. p. 244–252.
- Kerpauskas P, Sirvgdas AP, Lazauskas P, Vasinauskiene R, Tamosiunas A. 2006. Possibilities of weed control by water steam. Agronomy Research. 4(Special Issue):221–225.
- Knezevic SZ, Stepanovic S, Datta A. 2014. Growth stage affects response of selected weed species to flaming. Weed Technology. 28:233–242. doi: 10.1614/WT-D-13-00054.1
- Kruidhof HM, Bastiaans L, Kropff MJ. 2008. Ecological weed management by cover cropping: effects on weed growth in autumn and weed establishment in spring. Weed Research. 48(6):492–502. doi: 10.1111/j.1365-3180.2008.00665.x
- Lague C, Gill J, Peloquin G. 2001. Thermal control in plant protection. In: Vincent C, Panneton B, Fleurat-Lessard F, editors. Physical control methods in plant protection. Berlin: Springer-Verlag; p. 35–46.
- Malek MA. 2004. Power boiler design, inspection and repair: ASME code simplified. New York (NY): McGraw-Hill Professional.
- Merfield CN, Hampton JG, Wratten SD. 2009. A direct-fired steam weeder. Weed Research. 49(6):553–556. doi: 10.1111/j.1365-3180.2009.00733.x
- Owen MDK, Zelaya IA. 2005. Herbicide-resistant crops and weed resistance to herbicides. Pest Management Science. 61(3):301–311. doi: 10.1002/ps.1015
- Palmer DM. 1996. Lincoln University flame weeder improvements: an unpublished report for ENNR 425 Natural Resources Engineering Project. 30 p.
- Parrott DL, McInnerney K, Feller U, Fischer AM. 2007. Steam-girdling of barley (Hordeum vulgare) leaves leads to carbohydrate accumulation and accelerated leaf senescence, facilitating transcriptomic analysis of senescence-associated genes. New Phytology. 176(1):56–69. doi: 10.1111/j.1469-8137.2007.02158.x
- Peruzzi A, Raffaelli M. 1999. Experimental tests of selective flame weeding for different spring summer crops in central Italy. Proceedings of Trends in Agricultural Engineering, Prague, Czech Republic. p. 254–260.
- Sandhu H, Wratten S, Costanza R, Pretty J, Porter JR, Reganold J. 2015. Significance and value of non-traded ecosystem services on farmland. PeerJ. 3:e762. doi: 10.7717/peerj.762
- Sartorato I, Zanin G, Baldoin C, Zanche C. 2006. Observations on the potential of microwaves for weed control. Weed Research. 46(1):1–9. doi: 10.1111/j.1365-3180.2006.00484.x
- Sirvgdas A, Lazauskas P, Vasinauskiene R, Kerpauskas P. 2002. Thermal weed control by water steam. 5th EWRS workshop on physical and cultural weed control. p. 253–262.
- Sivesind EC, Leblanc ML, Cloutier DC, Seguin P, Stewart KA. 2009. Weed response to flame weeding at different developmental stages. Weed Technology. 23(3):438–443. doi: 10.1614/WT-08-155.1
- Ulloa S, Datta A, Knezevic SZ. 2010a. Tolerance of selected weed species to broadcast flaming at different growth stages. Crop Protection. 29:1381–1388. doi: 10.1016/j.cropro.2010.07.017
- Ulloa SM, Datta A, Knezevic SZ. 2010b. Growth stage impacts tolerance of winter wheat (Triticum aestivum L.) to broadcast flaming. Crop Protection. 29(10):1130–1135. doi: 10.1016/j.cropro.2010.04.009
- Vanhala P, Rahkonen J. 1996. Response of weed populations to flaming. Second International Weed Control Congress. p. 1115–1120.
- Wratten SD, Sandhu H, Cullen R, Costanza R, editors. 2013. Ecosystem services in agricultural and urban landscapes. Chichester: Wiley-Blackwell.
- Wszelaki AL, Doohan DJ, Alexandrou A. 2007. Weed control and crop quality in cabbage (Brassica oleracea (capitata group)) and tomato (Lycopersicon lycopersicum) using a propane flamer. Crop Protection. 26(2):134–144. doi: 10.1016/j.cropro.2006.04.012