ABSTRACT
A farm systems experiment comparing five pasture-based dairy systems was conducted in south-western Australia over 4 years on an annual ryegrass:subterranean clover sward. Fertiliser N inputs (0, 0.5, 1, 1.5 and 2 kg N ha−1 day−1) and stocking rate (SR; 1.25, 1.50, 1.75, 2.0 and 2.25 milking cows ha−1) increased concurrently for each treatment (F1–F5, respectively). By design, milk yield per cow did not differ between treatments; therefore, the response was measured in terms of changes in herbage dry matter (DM) utilisation and nutritive characteristics, as well as differences in feed input and imported forage required. Total annual pasture utilisation (t DM ha−1) increased from treatment F1–F3 (5.1, 5.9 and 7.3 t DM−1 ha−1, respectively), but responses beyond treatment F3 were not significant. Mean total N response efficiency was 9, 13, 8 and 7 kg DM per kg applied N for F2–F5, respectively, while mean marginal N response efficiency was 9, 17, −2 and 3 kg DM per kg applied N for F2–F5, respectively. The most efficient use of N fertiliser was achieved on treatment F3, where annual pasture utilisation levels in excess of 7 t DM ha−1 yr−1 were consistently demonstrated under the management strategies outlined, substantially greater than the local industry average.
Introduction
Dairy production systems in Western Australia (WA) are pasture-based and mostly rain-fed, with a typical herbage growth period of seven months, and mean annual rainfall ranging from 700 to 1000 mm (McDonnell et al. Citationforthcoming). Swards mainly comprise annual ryegrass (Lolium rigidum Gaud.), Italian ryegrass (L. multiflorum Lam.) and subterranean clover (Trifolium subterraneum L.). These species are well adapted to the sandy soils and Mediterranean climate of south-western Australia and provide high-quality feed for lactating dairy cows (Callow et al. Citation2000). The seasonal pattern of rainfall in the region typically results in 85% of annual rainfall occurring during the cool, wet growing season (GS) between May and October, which is followed by a warmer, dry period between November and April. Therefore, in spring (September/October), grazed pasture on dairy farms is surplus to requirements, meaning it can be conserved for use in the non-grazing summer period (McDonnell et al. Citation2017).
The level of herbage production and utilisation has been repeatedly shown to be a key determinant of profitability in pasture-based dairy systems (Dillon Citation2006; Chapman et al. Citation2014; Tharmaraj et al. Citation2014). As milk price fluctuation has become increasingly volatile in recent decades, greater focus has been directed towards finding efficiency improvements in variable inputs such as fertiliser, and management decisions such as the optimum SR. Bolland & Guthridge (Citation2007a, Citation2007b, Citation2009) previously reported dry matter (DM) yield responses to applied N fertiliser on dairy pastures in WA, and showed linear increases in herbage DM yield in response to applied N up to 320 kg ha−1 year−1, with no maximum yield plateaus defined. Nitrogen response efficiencies of ∼12.5 kg DM per kg of applied N were also observed. However, these studies were designed as grazing plot experiments and only measured the response of grazed herbage to applied N fertiliser. This differs from commercial dairy farms where the SR is adapted to herbage production and farm management must cope with the effects of seasonal variation in herbage availability. Furthermore, a greater amount of N is excreted in faeces and urine as the N fertiliser application rate increases, whereas the amount that can be recycled decreases, thus causing an increase in N losses (Ledgard et al. Citation1999). Consequently, on commercial dairy farms, milk yield and composition responses determine the economics of N fertiliser use and the optimal N application rate.
Classical small-plot N experiments have typically examined the response to increasing rates of N fertiliser application independent of other factors such as SR (Deenen & Lantinga Citation1993; Lantinga et al. Citation1999; McKenzie et al. Citation2003a; Bolland & Guthridge Citation2007a, Citation2007b). Therefore, it was considered necessary to conduct a systems experiment in which an individual livestock herd was allocated to a self-contained and independent farmlet, at five different levels of N fertiliser. As SR is also considered to be a key variable affecting herbage utilisation, it was decided to increase SR in relation to the expected rise in herbage yield generated by the higher levels of N fertiliser application. In the 2-year farmlet experiment of Humphreys et al. (Citation2008), SR was also adjusted upwards with each increased rate of N fertiliser application. A similar design was chosen for this experiment in the knowledge that while the independent effects of N fertiliser would be confounded by the different SR, it would also more closely reflect the reality of different dairy farm systems employed in the region. A previous farmlet experiment conducted on the same site (Staines et al. Citation2007), showed that the annual forage requirement for lactating dairy cows on WA dryland farms was ∼4000 kg DM ha−1 (grazed and conserved forage). Bolland & Guthridge (Citation2007a) demonstrated that an application rate of 0.5 kg N ha−1 day−1 (equivalent to ∼100 kg N ha−1 year−1) in WA could be expected to generate an additional ryegrass herbage yield of ∼1000 kg DM ha−1 GS−1 in an experiment conducted at a nearby site. This would be sufficient to meet the annual forage requirements of 0.25 cows ha−1, based on the farmlet experiment of Staines et al. (Citation2007), and thus SR were increased by 0.25 dairy cows ha−1 for each N fertiliser increment of 0.5 kg N ha−1 day−1. This systems approach enabled responses to be measured in terms of total and consumed herbage yield, botanical composition changes, and nutritive characteristics of grazed and conserved herbage. The combination of the Mediterranean-type climate, large precipitation surplus in the GS, ryegrass/clover swards with annual species, and the sandy, duplex soil type also provided a unique aspect to this experiment that has not been replicated elsewhere.
This paper compares annual herbage yield and utilisation on each system, as well as changes in botanical, nutritive and mineral composition of herbage throughout the 4 years of the experiment. Nitrogen response efficiencies for each level of N fertiliser use are also presented and discussed.
Materials and methods
The experimental site
The experiment was carried out on the WA Department of Agriculture and Food’s Vasse Research Centre (VRC, 33°45′ S, 115°21′ E, elevation 30 m), approximately 15 km south of Busselton in south-western Australia. Meteorological data throughout the experiment are shown in , while pre-experimental soil characteristics are outlined in . The duplex soil was 1–2 m sand to sandy loam over ‘massive’ clay (10–20 m deep), known locally as Abba sand (Tille & Lantzke Citation1990) and classified as Chromosolic Redoxic Hydrosol (Isbell Citation2002) or Glossic Natrudalf (Soil Survey Staff Citation1987). As is common for many soils in the region, the topography of Abba sands is flat and the soils are often intermittently waterlogged from June to early September (Tille & Lantzke Citation1990).
Table 1. Rainfall recorded at the Vasse Research Centre (VRC) during the experimental period and long-term average climate data for the Busselton post office (∼15 km north of the VRC).
Table 2. Pre-experimental soil chemical properties and average soil test values from January 2006 to 2009 from each paddock in the five farmlets.
Experimental design
The experiment consisted of five farmlets (F1–F5), each with a different N fertiliser rate (kg N ha−1 day−1) and target SR (dairy cows ha−1; ). Paddocks were blocked on paddock history, soil test data and distance to the dairy and then allocated to farmlet treatments, such that farmlet area decreased with increasing SR. The objective was to maintain 20 autumn calving (April–May) Holstein-Friesian dairy cows in each farmlet throughout the experiment. Herds comprised 71% multiparous and 29% primiparous cows (±7%), with multiparous cows weighing 614 ± 66 kg and primiparous cows 502 ± 51 kg. Cows were milked twice daily throughout the experiment, before being dried off at a lactation length of approximately 310 days. They were then moved to a non-lactating cow herd within their respective farmlet and fed hay or silage. Three weeks before their expected calving date, non-lactating cows were moved to a single shared pre-calving herd outside of the farmlet paddocks and fed ad libitum pasture hay supplemented with concentrate. No grazed pasture was provided to transition cows at any stage. Young stock were not kept within the experimental farmlets but were reared separately at VRC until three weeks before their first calving.
Table 3. Target fertiliser N application levels, stocking rates, farmlet area and herd size for each farmlet treatment.
The experiment started with the first grazing in growing season 1 (GS1) on 14 June 2005, and covered four complete GS (GS1–GS4) to 3 December 2008, including the three intervening dry seasons (DS1–DS3; ). Grazed herbage provided most feed in the GS, while conserved pasture from the previous GS provided the majority of forage consumed during the DS. Concentrate was fed in all seasons throughout the experiment.
Table 4. Start and end dates for the four growing seasons (GS1–GS4) and the three dry seasons (DS1–DS3) during the experiment.
Soil sampling and analysis
Standard procedures for soil testing of high-rainfall pastures in Australia to a depth of 10 cm were used in January of each year (Bolland & Russell Citation2010). A total of 50–100 samples were collected per paddock, depending on the size of the paddock, which equated to an approximate rate of 75 samples per hectare. Samples were then bulked, mixed and air-dried before dispatch to an accredited commercial laboratory (CSBP Soil and Plant Analysis, Perth, WA, Australia) for analysis. Methods used in this experiment to measure soil Colwell P (and consequently P buffering index [PBI] and critical Colwell P), Colwell K, sulphur (S), pH (in calcium chloride), organic carbon and electrical conductivity (EC) are all detailed by Bolland & Russell (Citation2010).
Pasture species and varieties
The VRC had been used for intensive dairy grazing experiments since 1999 (Staines et al. Citation2007). From 2001 to 2005, paddocks used for the present experiment were reseeded with annual ryegrass (variety Progrow) each year at 15 kg ha−1, and subterranean clover (varieties Gosse and Trikkala) at 10 kg ha−1 in 30% of the overall experimental area. Paddocks were scored before the beginning of GS2, GS3 and GS4 on a scale of 1–5 based on abundance of natural seed-set. All paddocks scored at 2 or less were then reseeded. In 2006 (GS2), there was a particularly late start to the GS with poor natural seed set, and 46% of the total experimental area (all farmlet treatments) was reseeded with annual ryegrass at 25 kg ha−1. Prior to GS3, clover levels in the sward had diminished significantly, therefore, a mix of clover and annual ryegrass (40:60 ratio) was used to reseed 26% of the total experimental area at 25 kg ha−1. In early 2008, before the start of GS4, natural seed-set scoring revealed 45% of the total experimental area required reseeding, and therefore a mix of clover and annual ryegrass (40:60 ratio; 25 kg ha−1) was sown at the start of the GS.
Grazing management
Grazing management was based on the ryegrass leaf stage method of Fulkerson & Donaghy (Citation2001), where the target was to commence grazing when ryegrass had regrown three fully extended leaves per tiller. This strategy was followed while ryegrass plants were vegetative, typically from the beginning of the GS up to mid-October. Once plants reached the reproductive stage, mean rotation length ranged from 20 to 26 days, in order to minimise the decline in herbage quality (Callow et al. Citation2000). Cows were allocated a new strip to graze after each milking. Back-fencing was used in any section of paddock which had been grazed more than three days earlier, to prevent cows grazing regrowth shoots.
Measurement of herbage mass
Daily allocation of herbage to be grazed was based on ryegrass leaf stage and weekly estimation of herbage mass using a rising plate meter (RPM; Earle & McGowan Citation1979). As it was not practical to measure herbage mass before and after every grazing, RPM measurements were taken every Tuesday in all farmlet paddocks (n = 103 ± 31) across the same diagonal transect. Every Tuesday, during GS2, and every second Tuesday during GS1 and GS3, the RPM measurements were calibrated with herbage cuts within the experimental paddocks. On each occasion, 20–30 herbage samples were plated using the RPM and then cut to ground level. All calibration data were pooled together with other calibration data (3325 calibration samples of grazed herbage were collected from 2000 to 2007 at VRC before silage/hay harvest, and 300 calibration samples were collected for herbage regrowing after silage/hay was harvested in October–November) and an equation was generated. Six operators were responsible for 95% of the RPM measurements throughout the experiment. Before converting average RPM height to herbage mass DM, the RPM heights were adjusted for operator differences calculated from a multiple linear regression model fitted to the Tuesday pasture height data. The magnitude of the correction averaged 0.04 cm (SD = 0.60 cm). The derived calibration equation to calculate herbage mass from RPM height was:where D is the number of days since 1 May each year and H is the RPM height (cm). The standard error for this equation is ∼200 kg DM ha−1, at a herbage height of 5–10 cm. The root mean square error is 187 kg ha−1 at a height of 5 cm or 245 kg ha−1 at a height of 10 cm. Target compressed heights for post-grazing residuals adopted in this experiment were those typically used in the region, namely 2–3 cm at the seedling stage, 5 cm during the main GS, and 7 cm at seed-set in late spring (Staines et al. Citation2007).
Allocation of herbage
Cows were provided with enough feed to maintain a target milk production of 7000 L cow−1 per lactation in all five farmlets, using the AFRC (Citation1993) feeding standard. All cows received the same daily amount of concentrate regardless of farmlet treatment, therefore more concentrate and conserved forage was fed per hectare as SR was increased. As a consequence of the chosen experimental design, milk yield per cow did not differ (P > .05) between farmlets throughout the experiment (overall mean of 23.1 L cow−1 day−1). A greater amount of herbage was produced for grazing and conservation as both fertiliser N and SR increased from treatments F1–F5, which provided some of the extra feed required, whilst the balance was supplied by imported conserved forage. Where there was a deficit of conserved forage on a farmlet it was imported, in the first instance from other farmlets with surpluses, and then from elsewhere on the VRC.
The daily area allocated to each herd was determined by the rotation speed (days to 3 leaves). For example, when it took 30 days to grow ryegrass to 3 leaves, the cows would be given access to 1/30th of the farmlet area each day. Herbage mass adequacy was then assessed as herbage mass DM per paddock divided by the daily target grazed herbage DMI per cow (which varied throughout the year depending on metabolisable energy [ME] demand and use of feed supplements). Where the herbage plus target supplement allocation did not meet cow requirements for ME, additional conserved forage was provided. Where the herbage plus supplement allocation exceeded cow requirements for ME, conserved forage supplements were reduced and/or paddocks were removed from the grazing rotation for conservation. Paddocks were visually assessed every Friday to ensure cows had grazed to the target herbage residual height and if necessary, allocations of supplements were adjusted accordingly.
Fertiliser use
Fertiliser N was applied as urea (46% N) during GS1, GS2 and GS3 and as a blended fertiliser containing urea and ammonium sulphate during GS4. Fertiliser N application started after the first grazing in each GS and continued into late spring, until soil moisture was limiting herbage growth, generally by early to mid-November. The amount of fertiliser N applied after each grazing was calculated by multiplying the N rate (kg N ha−1 day−1) for a given farmlet treatment by the number of days since the last grazing (see Bolland & Guthridge Citation2009; Rawnsley et al. Citation2014). So if the N rate was 1.5 kg ha−1 day−1 and it was 30 days since the last grazing, then 45 kg N ha−1 was applied. The first N application rate in each GS was determined by the number of days from onset of season opening rains to the first grazing. If a paddock was locked up for conservation, a second fertiliser N application was made mid-way through the silage growth period in GS2, GS3 and GS4, if the lock-up period was long enough to warrant it. The average amount of fertiliser N actually applied in the experiment is shown in , and variation in total N applied between years are due to differences in the length of each GS.
Table 5. Fertiliser nitrogen (N) levels actually applied to each farmlet treatment during the four growing seasons (GS1–GS4; mean ± standard deviation) and average values for the four seasons.
There was no effect of farmlet treatment on the amount of P, K or S applied over the four GS, determined from annual soil tests. During GS1 and GS3, fertiliser S was applied once annually as gypsum (17% S). In GS4, S was applied as gypsum for treatment F1 and as fertiliser blends for treatments F2–F5. Four custom fertiliser blends for treatments F2–F5 were used in GS4; urea, ammonium sulphate (21% N, 24% S), potassium chloride (50% K) and potassium sulphate (42% K, 17% S) to ensure that the same amounts of K and S were applied per ha, while N increased with farmlet treatment as required. This was done so that S could be supplied more frequently in a more rapidly acting form than gypsum, as herbage tissue samples towards the end of the previous wetter seasons of 2005 and 2007 (GS1 and GS3) suggested herbage S concentrations were approaching marginal levels.
Soil analyses, before the experiment started in January 2005, indicated that lime applications were necessary to increase soil pH (mean pH in calcium chloride = 4.9). Therefore, 5 t ha−1 of lime was applied each year from GS2 to GS4 if pH was <4.5, 3.5 t ha−1 if pH was 4.5–4.9, 2 t ha−1 for pH 5.0–5.4 and 1.3 t ha−1 for pH 5.5–6.0.
Conserved forage and concentrates
Paddocks were removed from the grazing rotation and set aside for conservation when ryegrass in two or more paddocks within a farmlet exceeded the 3-leaf stage, generally from July onwards, and typically harvested from early to mid-October. Depending on the farmlet treatment, 25–50% of all paddocks were set aside for conservation as silage (round bales) in early to mid-October, by which time mean herbage mass had reached ∼5.0 (range 1.4–9.2) t DM ha−1. A small amount of hay was made when pasture surpluses occurred in November (mean herbage mass 3.4; range 1.1–5.7 t DM ha−1). Core samples were taken from 20% to 25% of silage and hay bales 5–6 weeks after baling, dried at 60°C for 72 h, ground and stored pending analyses. Typically, by early December, herbage growth and quality had declined to inadequate levels, and feeding of silage commenced at 10–14 kg DM cow−1 day−1, depending on the level of concentrate provided at that stage of the year. Residues of pasture silage were estimated visually when significant amounts were left and assigned equally to all cows in the applicable herd.
Even though there was no herbage available to be grazed during summer/autumn, herds were still rotated around their paddocks to ensure faeces, urine and feed refusals were distributed evenly across all paddocks. The provision of silage was gradually reduced once grazing started in late autumn (normally late May), until no silage was required, usually by mid to late June.
Concentrate was fed twice daily during milking in the May–November GS. The daily amount of concentrate fed per cow varied with stage of lactation and with seasonal changes in availability and quality of herbage. During the December–April period, when there was no herbage available for grazing, part of the concentrate (typically 5–6 kg cow−1 day−1) was fed as a partial mixed ration with silage provided in troughs in the paddocks. The composition of the concentrate mix varied between seasons as follows to achieve a nutritionally balanced total ration; during the GS, 87% was cereal grain, 9% lupins and 4% minerals and other additives, while during the DS, 58% was cereal grain, 38% lupins and 3.4% minerals and other additives.
Fresh and conserved herbage sampling and analysis
Herbage samples were collected from each paddock just before grazing at regular intervals during the GS. Samples were cut to a sward surface height of 5 cm with a battery powered hand-shears in strips 10 cm wide and 30 cm long (10 times per paddock immediately before grazing). For each paddock, half the sample was sorted into botanical components, while the other half was oven dried (60°C for 72 h), ground and stored pending subsequent analysis for nutritive characteristics.
For mineral concentration of herbage, 20–30 samples were hand plucked (using gloves) just before grazing at a sward surface height of ∼5 cm from each paddock in winter of each GS. The samples were oven dried at 60°C for 72 h, ground and dissolved in a nitric/perchloric acid mixture (Yuen & Pollard Citation1954). Subsequently the concentrations of elements in the digest were measured by inductively coupled plasma-atomic emission spectrometry (ICP-AES; Zarcinas et al. Citation1987).
Fresh and conserved herbage nutritive characteristics
Grazed herbage, silage and hay samples were analysed for DM content, ash and organic matter (OM) by ashing samples in a muffle furnace at 600°C for 10 h. Dry matter and OM digestibilities were measured using a pepsin-cellulase in vitro method (Aufrere & Michalet-Doreau Citation1988), from which DM digestibility, OM digestibility (OMD), digestible OM in DM (DOMD) and ME were calculated as outlined by the SCA (Citation1990). Total N in DM was measured using the Kjeldahl method as described by Isaac & Johnson (Citation1976), and crude protein (CP) was calculated as N × 6.25. Neutral detergent fibre (NDF) concentration was determined according to Van Soest et al. (Citation1991).
Calculating herbage consumption in each farmlet
Two methods were used to calculate herd herbage consumption in each GS. Firstly, grazed herbage consumption (t DM ha−1) was ‘back-calculated’ based on herd ME requirements and estimated ME intake from supplements (ME-calculated; see Fulkerson et al. Citation1986). We used the web-based calculator of Heard (Citation2012), and the inputs used are shown in supplementary Table S1.
Secondly, total grazed herbage consumption for each farmlet in each GS was estimated based on the weekly measurements of herbage biomass using the RPM (RPM-measured). The yield of conserved herbage DM (sum of all conserved forage bale weights) produced in each farmlet every year was then added to both estimates of grazed herbage consumption to give two estimates of total herbage use in each farmlet per season.
illustrates the relationship between ME calculated and RPM-measured grazed herbage consumption. Linear regression equations describing the relationship between both methods for each GS, as well as the overall grazed herbage intake across the four GS were as follows:
Figure 1. Relationship between grazed herbage consumption calculated using rising plate meters (RPM-measured herbage consumption) and grazed herbage consumption calculated from total ME requirements of each N farmlet each GS (ME-calculated herbage consumption).
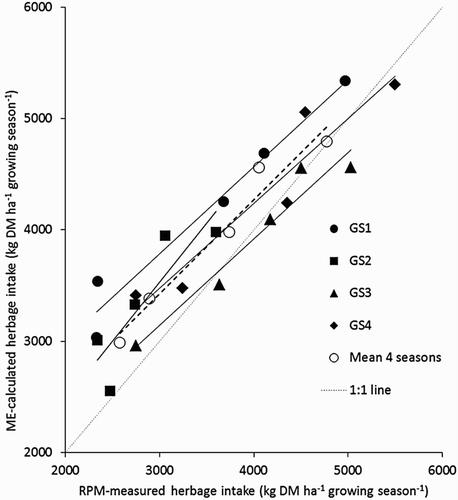
There was good agreement between the two methods, with a mean r2 of 0.96 observed across all four GS, therefore grazed herbage DM consumed per farmlet is presented as the mean of the two methods.
Analysis of data
Analysis of variance (ANOVA; GENSTAT 14th Edition, VSN International, Hemel Hempstead, UK) was used to compare treatment means. ‘Farmlet × GS’ was considered the experimental unit, where the five farmlets (4 d.f.) and four GS (3 d.f.) provided 20 observations in each analysis of variance (error term 12 d.f.). Least significant differences (P < .05) were used in multiple comparisons, if effects were significant (P < .05).
Total pasture harvest was plotted against total N fertiliser used in each season, to determine the efficiency of the pasture response to N fertiliser for each farmlet. This process was repeated monthly to determine the monthly N efficiency of the pasture response for each farmlet.
Results
Annual herbage yield response to farmlet treatment
shows the relationship between annual herbage consumption (grazed plus conserved) and the annual amount of N applied per ha. Total herbage yield responses to farmlet treatment in each year were highly significant (P < .001), with DM yields increasing from F1 to F3, but did not differ between F3 and F5. The responses were also significantly different (P < .001) in each of the four GS.
Herbage mass pre- and post-grazing
Average monthly pre-grazing herbage mass on all farmlets for the 4 years () differed significantly in each month (P < .001), increasing as the GS progressed from June to September, from (pre- and post-grazing, respectively) 1406 and 909 kg DM ha−1 in June to 2610 and 1844 kg DM ha−1 in November. The effect of farmlet treatment on mean pre-grazing herbage mass across the whole GS was highly significant (mean: 2010, 2107, 2335, 2257 and 2385 kg DM ha−1 for treatments F1–F5, respectively; P < .001; 5% LSD = 159). Likewise, mean post-grazing herbage mass per GS was significantly affected by farmlet treatment (mean: 1475, 1561, 1575, 1513 and 1504 kg DM ha−1 for treatments F1–F5, respectively; P = 0.025; 5% LSD = 66).
Nitrogen response efficiency (grazed plus conserved)
Total N response efficiency (kg herbage DM ha−1 per kg applied N ha−1) on each farmlet was calculated in each GS from cumulative herbage yield responses to total N fertiliser applied, as listed in . In all 4 years, total N response efficiency was largest for treatment F3 and decreased for F4 and F5. It was highest in GS2 (the driest year), lowest in GS3 and intermediate in GS1 and GS4. The marginal N response efficiency data (marginal kg DM ha−1 per marginal kg N ha−1 applied) were calculated from yearly marginal herbage responses between each treatment and changes in N rate and associated SR between each treatment listed in . Similar to total N response efficiency, in all 4 years, marginal N response efficiency was largest for F3 and decreased for F4 and F5. It was also highest in GS2 (2006; the driest year) lowest in GS3 and intermediate in GS1 and GS4. Monthly N response efficiencies () were calculated across all farmlets in the four GS to analyse the effect across different stages of the GS. Differences between seasons in length limited the availability of sufficient data to calculate monthly responses at the start and end of each GS, hence results are only presented from May to October for 2 years only. On average, however, mean N response efficiency increased throughout the GS, being the lowest in May (5 kg DM kg−1 applied N) and the highest in October (20 kg DM kg−1 applied N).
Table 6. Yield responses (kg DM ha−1) to increasing N fertiliser application rates on each farmlet treatment, together with corresponding N response efficiencies.
Table 7. Average monthly N response efficiencies (kg DM per kg N ha−1) obtained in each GS across all five farmlet treatments, and mean values across all four growing seasons.
Leaf emergence rate
The effect of farmlet treatment on the amount of extrapolated days required to grow 3 leafs per ryegrass tiller was 37.7, 36.8, 35.1, 34.8 and 31.8 days for F1–F5, respectively (P < 0.001; 5% LSD = 2.04).
Conserved forage
On average throughout the four GS, the total conserved area was 9.8, 7.7, 7.3, 5.3, and 4.6 ha on treatments F1–F5, respectively, which corresponded to 61, 58, 63, 53 and 52% of the total farmlet area on treatments F1–F5. Mean silage yield (t DM ha−1) from the areas taken out of the grazing rotation for conservation of forage throughout the experiment was 4.0, 4.9, 5.8, 5.6 and 5.2 for F1–F5, respectively, and the effect of farmlet treatment on mean silage yield was highly significant (P = .002; 5% LSD = 0.76). When expressed as tonnes of DM per actual number of cows on each farmlet, silage yield was 1.8, 1.8, 2.0, 1.5 and 1.2 t DM cow−1 for F1–F5, respectively, and again the effect of treatment was highly significant (P < .001; 5% LSD = 0.29).
The average date (±days) when paddocks were removed from the grazing rotation for silage in each GS was 24 July ±10 in GS1, 10 August ±22 in GS2, 19 August ±28 in GS3 and 12 August ± 17 in GS4. The average number of silage growing days was 91 ± 10 in GS1, 55 ± 15 in GS2, 71 ± 24 in GS3, and 78 ± 17 in GS4. Although the number of growing days differed significantly between years, farmlet treatment effects were not significant (data not shown).
Sward botanical composition
The effect of farmlet treatment and GS on herbage botanical composition is shown in . The mean proportion of ryegrass was lower (P < .001) and clover greater (P < .001) on F1 throughout the experiment than F2–F5, which did not differ from each other. For treatment F1, mean ryegrass content was 60%, 71%, 63% and 81%, and mean clover content was 27%, 9%, 26%, and 10%, for GS1–GS4, respectively. For treatments F2–F5, mean ryegrass content was 80%, 82%, 78% and 89%, and mean clover content was 9%, 3%, 10%, and 3%, for the four respective GS.
Table 8. Effect of farmlet treatment (F1–F5) and GS on botanical composition of herbage.
All components of sward botanical composition were significantly affected by GS (P < .001). The average sward clover content on all farmlets decreased from 12% to 4% between GS1 and GS2, then increased to 13% in GS3 (due to reseeding of clover at the start of GS3) before declining again to 4% in GS4 despite the fact a significant proportion of the farmlets were reseeded with clover again in GS4. Therefore, an increase in the mean ryegrass content of swards across all farmlets was observed between GS1 and GS4.
Herbage mineral concentration
The calcium (Ca) concentration of herbage was higher (P = .01) in F1 than F2–F5, and concentrations for F2–F5 did not differ significantly (). The boron concentration of herbage on the F1 treatment was also greater (P = .006) than treatments F2–F5; concentrations for F2–F5 did not differ. Copper (Cu) concentration of herbage samples was greater (P = .024) in F1 and F2 than F3–F5 (which did not differ). Nitrate concentration of herbage increased significantly (P > .01) between farmlet treatments; treatment F5 had a greater nitrate concentration than F1–F3, with F4 being intermediate.
Table 9. Effect of farmlet treatment on mineral concentration of hand-plucked herbage samples collected just before grazing during winter of each growing season.
Grazed herbage and silage nutritive characteristics
Herbage CP concentration increased significantly (P < .001) with increasing N fertiliser application rates (). Mean NDF concentration over the 4 years in treatment F1 was lower (P = .02) than treatments F2–F5, which did not differ. No significant effect (P > .05) of treatment on ME or mineral ash concentration of herbage was detected. The same farmlet treatment effects were also observed in silage, with the ME content of silage unaffected by treatment, CP concentration increasing (P < .001) with higher N fertiliser application rates, and silage NDF concentration lower (P = .002) in F1 than the F2–F5 treatments which did not differ.
Table 10. The effect of farmlet treatment (fertiliser N level and SR) on grazed herbage and silage nutritive characteristics over the four growing seasons.
Discussion
This experiment included a farmlet without fertiliser N to compare herbage production based on biological N2 fixation by subterranean clover with that based on different rates of fertiliser N. This is a common approach used in the previous N response experiments conducted under varying soil types and environmental conditions in Australia and New Zealand (Penno et al. Citation1996; McKenzie et al. Citation2003a; Monaghan et al. Citation2005; Bolland & Guthridge Citation2007a), but less so in perennial pastures in temperate climates of Western Europe where most N response studies were conducted on pure grass swards (Morrison et al. Citation1980; Deenen & Lantinga Citation1993; Lantinga et al. Citation1999). Where clovers are well adapted, studies on the effect of fertiliser N on herbage production should be conducted in mixed grass/clover swards. This has more practical relevance and allows the replacement of clover and biological N2 fixation by fertiliser N to be quantified. Compared to many previous studies, the maximum N fertiliser rates used in this experiment were modest, although they were considered high by WA dairy industry standards (annual average 100 kg N ha−1 for rain-fed dairy pastures).
Soil and herbage tissue testing in the present experiment showed that herbage yield was not limited by any essential nutrients other than N, with observed values greater than published minimum requirements for both ryegrass and clover production (Pinkerton et al. Citation1997; Bolland & Russell Citation2010). Electrical conductivity, an indicator of soil salinity, exceeded levels likely to be limiting ryegrass production but this effect was likely to only have an impact very early in the GS, when surface salinity levels would have increased over the DS due to the lack of rain (D. Bennett, 2017; pers. comm.). Soil pH in CaCl2 was similar on all farmlet sites, and within the range recommended by Bolland & Russell (Citation2010) to avoid herbage yield limitations on similar soil types.
Comparison of procedures used to estimate grazed herbage intake
There was good agreement between the two procedures used to estimate grazed herbage DMI, as reported in an earlier study (Fulkerson et al. Citation1986). Our data were generally of a better fit (r2 = .79−.93) than those reported by Heard et al. (Citation2011; r2 = .59), possibly because they consisted of 4 years of the same experiment, whereas the data of Heard et al. (Citation2011) were derived from four different experiments conducted at separate locations in different years. At a producer level, accurate estimation of herbage DMI is difficult, with use of tools such as the RPM to measure herbage yield by farmers in the region relatively low (McDonnell et al. Citation2017). Despite the strong relationship between the two datasets, there did appear to be a slight systematic bias towards higher estimates of herbage DMI using the ME-calculated method, particularly at lower levels of herbage intake. A similar trend was also observed by Heard et al. (Citation2011), where the ME-calculated model marginally overestimated intake at low levels and underestimated intake at higher levels. One possibility is that this occurrence was caused by negative associative effects, whereby animals fed starch-based cereal supplements in the parlour, while also consuming grazed herbage, utilise less ME than is actually available in the feeds. The mechanisms behind this effect have been well documented (Doyle et al. Citation2005; Heard et al. Citation2011), and are likely to have affected the amount of ME available to the animals in this experiment, particularly when herbage DMI was low and concentrate intake would have been greater. However, cows in all farmlet treatments were provided with the same level of concentrate and therefore these negative associative effects should have been the same across treatments. Moreover, limitations also surround the accurate measurement of herbage DMI from groups of grazing dairy cows using a RPM, including exclusion of areas with faecal matter and not allowing for pasture loss due to trampling (Williams et al. Citation2002), while this method also does not account for herbage growth during grazing (Humphreys et al. Citation2008). It is acknowledged that at the higher SR, there may have been a more pronounced effect of pasture treading on sward regrowth and utilisation (Drewry et al. Citation2008), which also could have confounded the results to some degree.
Herbage yield responses to farmlet treatment
The response to N fertiliser and associated SR was linear to treatment F3, with negligible herbage yield responses observed beyond this treatment. This equated to an average annual N application rate of 174 kg ha−1, or 1.12 kg N ha−1 day−1 for this treatment. Overall N response efficiency values were within the range found in previous studies for temperate (Eckard & Franks Citation1998; McGrath et al. Citation1998; Monaghan et al. Citation2005; Tharmaraj et al. Citation2014), subtropical (Eckard Citation1989; McKenzie Citation1996) and Mediterranean (Bolland & Guthridge Citation2007a, Citation2007b, Citation2009) environments with annual and perennial ryegrass dominant swards. In most of these studies, herbage yield responses to applied fertiliser N followed laws of diminishing returns and tended to plateau. The exception was for small-plot grazing experiments where there was an ongoing linear response to applied N (Eckard & Franks Citation1998; McKenzie et al. Citation2003a; Bolland & Guthridge Citation2007a). This has been attributed to fertiliser N rates used in these studies being too low to reach maximum yield plateaus. A further reason for this may be an underestimation of the role of N recycling by grazing animals, which is one of the primary reasons why the farmlet approach is preferred to assess the impact on the overall system of the various N treatments (Whitehead Citation1995).
More recently, Rawnsley et al. (Citation2014), in a two-year plot experiment using perennial ryegrass dominant pastures, reported a significant effect of time on N response efficiencies, with very high herbage responses to applied N observed in the first six months (March–September) of their study (>20 kg DM ha−1 per kg of applied N). However, these responses had virtually disappeared by the end of the 2-year study, with nil N treatments achieving comparable daily growth rates to plots receiving as much as 3 kg ha−1 day−1 of applied N. These authors suggested that a significant increase in the proportion of white clover in the nil N treatment as the experiment progressed, may have contributed to this effect. Despite this, their data would appear to indicate that long-term repeated applications of N fertiliser may result in reduced herbage yield responses to fertiliser N. In contrast, however, this effect was not observed during the 4 years of our experiment. Several factors can affect herbage yield responses to N fertiliser, including groundwater level (Whitehead Citation1995), botanical composition (Rawnsley et al. Citation2014; Tharmaraj et al. Citation2014), and the amount of N consumed in imported supplementary feeds (Ryan et al. Citation2012). In the present experiment, concentrate and conserved forage supplementation per cow did not differ across treatments which meant the amount of N ha−1 imported in concentrate was greater as SR increased. Additionally, there was a small surplus of silage on treatments F1–F3, and a sharp deficit in F4 and F5 meaning a large amount of silage was imported on the latter two treatments (data not shown).
Herbage yield responses to farmlet treatment varied across different GS and this is in line with previous studies (Whitehead Citation1995; Eckard & Franks Citation1998; McKenzie et al. Citation2003a; Bolland & Guthridge, Citation2007a). Yield responses to N and associated SR characterised a pattern resembling a ‘broken stick’, due to the absence of any significant yield response beyond the F3 treatment. An alternative method to present the response was to fit a polynomial regression through the five data points in each season and consequently calculate the N fertiliser rate required to achieve 95% of the maximum yield. We also analysed the data using this approach, and found that across the four GS, 95% of the maximum herbage yield was obtained at an annual N rate of 181 kg N ha−1 (equivalent to 1.19 kg N ha−1 day−1 from the first to last application of N fertiliser), which is highly comparable to the average amount of N applied on the F3 treatment (174 kg N ha−1; 1.12 kg N ha−1 day−1).
The greatest N response efficiencies were obtained in GS2 (the driest GS), compared to the wetter GS1, GS3 and GS4. This outcome reflects the unique nature of the soils in the region, where unlike New Zealand, for example, little N is lost via leaching due to the duplex soil characteristics where there is an impermeable layer beneath the initial sandy surface. Instead, N losses in the region are caused by surface runoff and denitrification, which are intensified in wetter seasons (Bolland & Russell Citation2010). Greater use of fertiliser N in wetter conditions (greater surface runoff due to intense rainfall and duplex soil) not only enhances the cost of its use but may also lead to increased losses of N to the environment beyond the farm (Whitehead Citation1995). While we acknowledge that the effects of N fertiliser in the present experiment are not independent due to the adjusted SR, it is still important to note that marginal N response efficiencies were highest for the F3 treatment in all years, reflecting the absence of herbage yield responses beyond this treatment. Therefore, the key outcome from this experiment was that under these environmental and agronomic conditions, there is little to no herbage DM yield benefit in increasing the annual average N fertiliser application rate beyond 1.1 kg ha−1 day−1. Often, lower amounts of N may be adequate under the Mediterranean climate and growing conditions that this experiment was conducted in, as evidenced by the high total and marginal N response efficiencies on the F2 and F3 farmlets in the driest season (GS2). Furthermore, while the monthly N response efficiencies presented here are limited to averages of all farmlet treatments, the data clearly shows that the response to N fertiliser is greatest in the spring months of September and October and lowest in winter. This is in agreement with previous local research by Bolland and Guthridge (Citation2007a, Citation2009), who also reported higher herbage yield responses to N fertiliser in the region in spring, due to an increase in solar radiation and temperatures, and a decrease in waterlogging. These data indicate producers in the region should strategically apply N throughout the GS, and we suggest an average application rate of ∼0.5 kg ha−1 day−1 is sufficient in the autumn/early winter period, increasing to ∼1.5 kg ha−1 day−1 in spring when growing conditions are more favourable and yield responses are greater.
Total herbage yields and grazing management
A recent study of 13 commercial farms in WA (McDonnell et al. Citation2017) with SR ranging from 1.1 to 2.8 cows ha−1, showed the mean annual dryland forage yield in the region is approximately 5 t DM ha−1. However, this figure varied from 3 to 8 t DM ha−1 between farms, indicating significant scope for improving home grown forage production exists on many farms in WA. The present experiment has demonstrated that even with no fertiliser N inputs, mean herbage production over the 4-year period was >5 t DM ha−1, and that consistent annual herbage yields exceeding 7 t DM ha−1 are possible in the region under the grazing and fertiliser management techniques employed here. Similar levels of annual herbage utilisation growth were also reported in an earlier dairy farmlet experiment at the same location (Staines et al. Citation2007).
The 3-leaf grazing principle (Fulkerson & Donaghy Citation2001) was used in the present experiment to determine the time of grazing. Differences between treatments in mean leaf stage at grazing were considered too small to be of biological relevance (range 2.7–3.1 leaves per tiller; data not shown). Interestingly, greater levels of N fertiliser did result in a significantly faster leaf emergence rate (days to 3 leaves) in this experiment. Similarly, Lee et al. (Citation2007) reported a minor positive effect of N fertiliser on leaf emergence rate in perennial ryegrass, but other results are inconclusive. Whitehead (Citation1995) stated that nutrient supply is generally thought to have minimal impact on leaf emergence rates in most grasses.
The results for mean pre- and post-grazing herbage mass on each farmlet shows that post-grazing mass increased steadily on all farmlets as the GS progressed, which agrees with Bolland and Guthridge (Citation2007a, Citation2007b) as well as the farmlet experiment of Staines et al. (Citation2007) at the same site. The higher post-grazing residuals in November and December on all farmlets are a deliberate management practice specially adopted for annual pasture species in WA, to encourage natural seed-set for the subsequent GS (Callow et al. Citation2000). Pre-grazing herbage mass also increased in all treatments from May to September, but then appeared to plateau from October to December in all farmlets apart from F4 and F5. We did not have sufficient data points to statistically analyse month × treatment interactions in pre- or post-grazing herbage mass, but the observed higher pre-grazing herbage mass from October to December in treatments F4 and F5 support the theory that N application rates in excess of 1 kg ha−1 day−1 towards the end of the GS, when environmental conditions are more favourable for ryegrass production, may result in higher pre-grazing herbage yields during this period, provided grazing management does not change. However, a further consideration is that increased ryegrass growth rates in late spring, particularly at high N fertiliser application levels, may lead to canopy closure before the 3-leaf stage, promoting stem elongation and reduced production of sugars by photosynthesis (Rawnsley et al. Citation2014). Under this scenario, delaying grazing to the 3-leaf stage may cause herbage mass to go beyond optimum pre-grazing herbage mass levels, which has previously been shown to have a negative effect on herbage utilisation (Curran et al. Citation2010).
Herbage botanical composition
The ryegrass and clover content of grazed herbage did not differ from treatments F2–F5, but clover content was lower and ryegrass content higher in F2–F5 compared to F1. The effects of treatment on sward botanical composition over the 4 years were heavily confounded by the reseeding of clover in a large number of paddocks throughout the experiment and should be interpreted with caution. Nevertheless, the combination of N fertiliser and higher SR appeared to cause a decline in clover content despite the re-seeding of clover in selected paddocks at the start of GS3 and GS4. This observed decline in sward clover content over time supports results of previous research on intensively grazed pastures in south-western Australia (Bolland & Guthridge Citation2007a) and elsewhere (Whitehead Citation1995; Monaghan et al. Citation2005). Significant changes in sward composition will have implications for fertiliser programmes. For example, we have recently shown that annual and Italian ryegrass swards have a much lower minimum K requirement (12 g kg−1 DM; McDonnell et al. Citationforthcoming) than subterranean clover (>20 g kg−1 DM; Pinkerton et al. Citation1997). In the present experiment, soil and herbage tissue testing indicated that clover production was not limited by availability of P, K or S, all of which are required in greater amounts for clover than ryegrass (Pinkerton et al. Citation1997). The increase in ryegrass content of swards over time in the present experiment may be due to a higher response of ryegrass to N fertiliser, leading to shading out of clover plants which causes reduced clover stolon numbers and lengths, and reduced stolon branching (Harris & Clark, Citation1996). However, McKenzie et al. (Citation2003c) showed that the rate of N fertiliser had no impact on the white clover content of a mixed white clover/perennial ryegrass sward, and suggested that this was due to optimal grazing management practices in N fertilised pastures where low post-grazing residuals were obtained, thus preventing ryegrass shading out clover. In the present experiment, it appears that the actual rate of N fertiliser applied had no effect on the clover content of the sward, as evidenced by the absence of a difference from treatments F2–F5. While the presence of N fertiliser caused a decline in sward clover composition compared to the nil N treatment (F1), it was not the sole factor affecting the reduction, as F1 also had a significantly reduced level of clover in GS4 compared to GS1. A similar decline in clover content independent of N fertiliser application rate was reported by McKenzie et al. (Citation2003c), who suggested the presence of grasses further along the ecological succession continuum than clover may have resulted in clover giving way to ryegrass as OM and fertility status increased. The increase in SR may also have contributed to the decline in clover content of the sward; previous studies have shown increased treading by grazing livestock on clover swards can contribute to stolon death by smothering via dung (Whitehead Citation1995), while urine patches high in soluble N generate a suppressive effect on N2 fixation and leads to the death of nodules and a reduction in the weight and length of stolons (Marriott et al. Citation1987).
Nutritive characteristics
The CP concentration of silage was greater at the highest rates of N fertiliser, which is in agreement with results of an earlier plot experiment by Jacobs et al. (Citation1998), using perennial ryegrass dominant swards. These authors reported higher CP and ME concentrations with increasing N application rates in silage conserved 35 days after lock-up, however, by 64 days after lock-up CP and ME tended to decrease with higher N applications. In the present experiment, the mean number of days to grow silage was greater than 64 in all but one season (GS2), while the ME concentration of silage was unaffected by N fertiliser and associated SR. Furthermore, silage was usually harvested in mid-October as the typical wet conditions on the experimental site in early spring meant limited silage harvest was possible before 1 October in most years. Grazed herbage ME content was unaffected by the rate of N fertiliser application in this experiment and this supports results of a previous study in this region by Bolland and Guthridge (Citation2007a) using small-plot grazing experiments. In contrast, other experiments with perennial ryegrass observed higher herbage ME contents as fertiliser N rates increased (Jacobs et al. Citation1999; Jacobs et al. Citation2002; McKenzie et al. Citation2003b). In the latter experiment, however, these authors observed no change in the ratio of clover to ryegrass, a reduction in which can be associated with a reduced ME content (Whitehead Citation1995). In the present experiment, herbage CP concentration increased as fertiliser N rate increased whereas in a previous study in WA by Bolland and Guthridge (Citation2007a), effects of N fertiliser rates on herbage CP concentrations were inconsistent as more N was applied. Unfortunately, the non-protein N concentration of herbage in the present experiment was not measured, however, the nitrate concentration of herbage from F1 to F5 progressively increased, supporting earlier results of Bolland and Guthridge (Citation2007b) in WA, Eckard (Citation1989) in South Africa and elsewhere as summarised by Whitehead (Citation1995). The highest nitrate levels found during August and September each year were less than 2000 mg kg−1 DM, well below the level harmful to grazing livestock (>4000 mg kg−1; SCA Citation1990).
The lower herbage DM concentrations observed as the rate of N fertiliser increased in the present experiment are well documented in the literature (Whitehead Citation1995; Peyraud & Astigarraga Citation1998), and are worthy of consideration due to their potential effect on the voluntary herbage intake in lactating cows fed large amounts of fresh herbage. The rate of DMI decreases when cows consume fresh forages with a DM content lower than 180 g kg−1 DM, possibly due to a physical limitation caused by excess water in the rumen (Peyraud & Astigarraga Citation1998). Neutral detergent fibre values of both fresh herbage and silage were lower in treatment F1 compared to F2–F5, which did not differ. Some studies have found NDF values significantly decreased as N application levels increased (Jacobs et al. Citation1999), while others have observed no effect of N fertiliser on NDF concentration of herbage (Jacobs et al. Citation2002). The effects of farmlet treatment on NDF concentrations of grazed herbage and silage observed in the present experiment were likely due to the higher proportion of clover, which has a lower NDF concentration than ryegrass (Whitehead Citation1995) in the F1 treatment relative to the other treatments.
Mineral concentration of herbage
Herbage tissue testing is an alternative method to soil testing for monitoring the P, K and S status of pastures in the region, due to the predominantly sandy soils causing these nutrients to leach below pasture root zones, particularly in wet years (Bolland et al. Citation2002; Bolland & Guthridge Citation2009; Bolland & Russell Citation2010). In the present experiment, mineral concentration was determined for herbage samples collected in winter when plant tissue was in the vegetative stage of growth and indicated fertiliser strategies used for P, K and S provided adequate amounts of these nutrients for ryegrass production, supporting results of other local studies (Bolland & Guthridge Citation2009; Bolland & Russell Citation2010).
The higher herbage Ca concentration observed on F1 was likely due to the greater presence of clover in the sward, which has a higher level of Ca than ryegrass (Whitehead Citation1995). Calcium concentration of herbage was unaffected by the rate of N, however, as evidenced by the lack of change from treatments F2–F5. This agrees with earlier work by McKenzie et al. (Citation2003b) on perennial ryegrass/white clover swards. A comprehensive recent study outlined the typical mineral concentration of a large range of forages commonly fed on dairy farms in Australia (Rugoho et al. Citation2017), and values for annual ryegrass were comparable to those observed here on annual ryegrass dominant swards. Overall, the mineral concentration of herbage on all farmlet treatments was adequate for high producing dairy cows, as outlined by McKenzie et al. (Citation2003b), while the variation in herbage mineral concentration from all five treatments did not appear to be of sufficient magnitude to negatively affect animal health (SCA Citation1990).
Conclusions
Maximum total and marginal N response efficiencies were achieved in a system stocked at 1.75 cows ha−1 where an average of approximately 1.1 kg N ha−1 day−1 throughout the GS was applied. Negligible herbage yield responses to N fertiliser were observed beyond this rate of N and associated SR, but imported supplementary feed increased significantly beyond this treatment, indicating the most cost-effective use of N fertiliser was on this system. The response to N fertiliser was lower in winter and greater in spring, when environmental conditions were more suited for rapid herbage growth. Therefore, we recommend producers in the region strategically apply ∼0.5 kg ha−1 day−1 earlier in the GS and ∼1.5 kg ha−1 day−1 in spring when N response efficiencies are greater, equating to an overall average of ∼1.1 kg ha−1 day−1 across the whole GS. The subterranean clover content of swards declined on all farmlets, despite reseeding of clover throughout the experiment but the decline was greater on farmlets receiving N fertiliser. The experiment also showed that under the grazing management practices employed, producers in the region should be able to obtain annual herbage utilisation rates on dryland farms of 6–8 t DM ha−1, which is substantially higher than average utilisation rates currently achieved by WA dairy farmers in similar systems.
Appendix 1: Data used in the back-calculation of herbage consumption, using procedures described by Heard (2012) and Heard et al. (2011)
Download MS Word (17.2 KB)Acknowledgements
We wish to thank the following people for their contribution to this experiment: J Lucey, W Russell, D Bennett, T Casson, A Van Burgel, J Baker, L Paszkudzka-Baizert, P Donnelly, P Jelinek, A Lindsay, K Lawson, J Milligan, L Chinnery, J Crosby, K Gardiner, D Russell, J Hahn, N Mercer, N Smith and F Treasure. We would also like to thank the project steering committee for their guidance. We are grateful for positive critical comments by H van der Meer and W Fulkerson who helped us to greatly improve the paper.
Disclosure statement
No potential conflict of interest was reported by the authors.
ORCID
R.P. McDonnell http://orcid.org/0000-0002-2461-7027
Additional information
Funding
References
- AFRC. 1993. Energy and protein requirements of ruminants. Wallingford, CT: CAB International.
- Aufrere J, Michalet-Doreau B. 1988. Comparison of methods for predicting digestibility of feeds. Animal Feed Science and Technology. 20:203–218. doi: 10.1016/0377-8401(88)90044-2
- Bolland M, Cox W, Codling B. 2002. Soil and tissue tests to predict pasture yield responses to applications of potassium fertiliser in high-rainfall areas of south-western Australia. Australian Journal of Experimental Agriculture. 42:149–164. doi: 10.1071/EA01060
- Bolland M, Guthridge I. 2007a. Responses of intensively grazed dairy pastures to applications of fertiliser nitrogen in south-western Australia. Australian Journal of Experimental Agriculture. 47:927–941. doi: 10.1071/EA06014
- Bolland M, Guthridge I. 2007b. Determining the fertiliser phosphorus requirements of intensively grazed dairy pastures in south-western Australia with or without adequate nitrogen fertiliser. Australian Journal of Experimental Agriculture. 47:801–814. doi: 10.1071/EA05184
- Bolland M, Guthridge I. 2009. Quantifying pasture dry matter responses to applications of potassium fertiliser for an intensively grazed, rain-fed dairy pasture in south-western Australia with or without adequate nitrogen fertiliser. Animal Production Science. 49:121–130. doi: 10.1071/EA08106
- Bolland M, Russell W. 2010. Changes in chemical properties of 48 intensively grazed, rain-fed dairy paddocks on sandy soils over 11 years of liming in south-western Australia. Australian Journal of Soil Research. 48:682–692. doi: 10.1071/SR09199
- Burkitt L, Moody P, Gourley C, Hannah M. 2002. A simple phosphorus buffering index for Australian soils. Australian Journal of Soil Research. 40:497–513. doi: 10.1071/SR01050
- Callow M, Michell P, Baker J, Hough G. 2000. The effect of defoliation practice in Western Australia on tiller development of annual ryegrass (Lolium rigidum) and Italian ryegrass (Lolium multiflorum) and its association with forage quality. Grass and Forage Science. 55:232–241. doi: 10.1046/j.1365-2494.2000.00220.x
- Chapman D, Beca D, Hill J, Tharmaraj J, Jacobs J, Cullen B. 2014. Increasing home-grown forage consumption and profit in non-irrigated dairy systems. 4. Economic performance. Animal Production Science. 54:256–262. doi: 10.1071/AN13186
- Curran J, Delaby L, Kennedy E, Murphy JP, Boland TM, O’Donovan M. 2010. Sward characteristics, grass dry matter intake and milk production performance are affected by pre-grazing herbage mass and pasture allowance. Livestock Science. 127:144–154. doi: 10.1016/j.livsci.2009.09.004
- Deenen P, Lantinga E. 1993. Herbage and animal production responses to fertilizer nitrogen in perennial ryegrass swards. I. Continuous grazing and cutting. NJAS Wageningen Journal of Life Sciences. 41:179–203.
- Dillon P. 2006. Achieving high dry-matter intake from pasture with grazing dairy cows. In: Fresh herbage for dairy cattle, the key to a sustainable food chain. 18th ed. Heidelberg: Springer-Verlag; p. 1–26.
- Doyle PT, Francis SA, Stockdale CR. 2005. Associative effects between feeds when concentrate supplements are fed to grazing dairy cows: a review of likely impacts on metabolisable energy supply. Australian Journal of Agricultural Research. 56:1315–1329. doi: 10.1071/AR05087
- Drewry JJ, Cameron KC, Buchan GD. 2008. Pasture yield and soil physical property responses to soil compaction from treading and grazing – a review. Australian Journal of Soil Research. 46:237–256. doi: 10.1071/SR07125
- Earle D, McGowan A. 1979. Evaluation and calibration of an automated rising plate meter for estimating dry matter yield of pasture. Australian Journal of Experimental Agriculture. 19:337–343. doi: 10.1071/EA9790337
- Eckard R. 1989. The response of Italian ryegrass to applied nitrogen in the natal midlands. Journal of the Grassland Society of Southern Africa. 6:19–22. doi: 10.1080/02566702.1989.9648154
- Eckard R, Franks D. 1998. Strategic nitrogen fertiliser use on perennial ryegrass and white clover pasture in north-western Tasmania. Australian Journal of Experimental Agriculture. 38:155–160. doi: 10.1071/EA97022
- Fulkerson W, Dobos R, Michell P. 1986. Relationship between predicted energy requirements and measured energy intake of dairy cattle at pasture. Australian Journal of Experimental Agriculture. 26:523–526. doi: 10.1071/EA9860523
- Fulkerson W, Donaghy D. 2001. Plant-soluble carbohydrate reserves and senescence-key criteria for developing an effective grazing management system for ryegrass-based pastures: a review. Australian Journal of Experimental Agriculture. 41:261–275. doi: 10.1071/EA00062
- Harris S, Clark D. 1996. Effect of high rates of nitrogen fertiliser on white clover growth, morphology, and nitrogen fixation activity in grazed dairy pasture in northern New Zealand. New Zealand Journal of Agricultural Research. 39:149–158. doi: 10.1080/00288233.1996.9513173
- Heard J. (2012). Pasture consumption and feed conversion efficiency calculator. Department of Environment and Primary Industries, Victoria, Australia. Available from: http://www.dpi.vic.gov.au/agriculture/dairy/pastures-management/calculator.
- Heard J, Doyle P, Francis S, Staines M, Wales W. 2011. Calculating dry matter consumption of dairy herds in Australia: the need to fully account for energy requirements and issues with estimating energy supply. Animal Production Science. 51:605–614. doi: 10.1071/AN10079
- Humphreys J, O’connell K, Casey I. 2008. Nitrogen flows and balances in four grassland-based systems of dairy production on a clay-loam soil in a moist temperate climate. Grass and Forage Science. 63:467–480. doi: 10.1111/j.1365-2494.2008.00660.x
- Isaac RA, Johnson WC. 1976. Determination of total nitrogen in plant tissue, using a block digestor. Journal of the Association of Official Analytical Chemists. 59:98–100.
- Isbell R. 2002. The Australian soil classification. Melbourne: CSIRO Publishing.
- Jacobs JL, McKenzie FR, Kearney G. 2002. Nitrogen fertiliser effects on nutritive characteristics of perennial ryegrass during late autumn, and mid- and late winter in western Victoria. Australian Journal of Experimental Agriculture. 42:541–548. doi: 10.1071/EA01144
- Jacobs JL, McKenzie FR, Rigby SE, Kearney G. 1998. Effect of nitrogen fertiliser application and length of lock up on dairy pasture dry matter yield and quality for silage in south-western Victoria. Australian Journal of Experimental Agriculture. 38:219–226. doi: 10.1071/EA97151
- Jacobs JL, McKenzie FR, Ryan MJ, Kearney G. 1999. Effect of rate and time of nitrogen application from autumn to midwinter on perennial ryegrass & white clover dairy pastures in western Victoria. 2. Pasture nutritive value. Australian Journal of Agricultural Research. 50:1067–1072. doi: 10.1071/AR98197
- Lantinga E, Deenen P, Van Keulen H. 1999. Herbage and animal production responses to fertilizer nitrogen in perennial ryegrass swards. II. Rotational grazing and cutting. NJAS Wageningen Journal of Life Sciences. 47:243–261.
- Ledgard SF, Penno JW, Sprosen MS. 1999. Nitrogen inputs and losses from clover/grass pastures grazed by dairy cows, as affected by nitrogen fertilizer application. The Journal of Agricultural Science. 132:215–225. doi: 10.1017/S002185969800625X
- Lee JM, Donaghy DJ, Roche JR. 2007. The effect of grazing severity and fertiliser application during winter on herbage regrowth and quality of perennial ryegrass (Lolium perenne L.). Australian Journal of Experimental Agriculture. 47:825–832. doi: 10.1071/EA06037
- Marriott CA, Smith MA, Baird M. 1987. The effect of sheep urine on clover performance in a grazed upland sward. The Journal of Agricultural Science. 109:177–185. doi: 10.1017/S0021859600081120
- McDonnell RP, Staines MVH, Bolland MDA. Forthcoming. Determining the critical plant test potassium concentration for annual and Italian ryegrass on dairy pastures in south-western Australia. Grass and Forage Science. doi:10.1111/gfs.12286.
- McDonnell RP, Staines MVH, Edmunds BE, Morris R. 2017. Feeding management, production and performance of thirteen pasture-based dairy farms in a Mediterranean environment. Animal Production Science. 57:1940–1951. doi: 10.1071/AN15768
- McGrath J, Penno J, Macdonald K, Carter W. 1998. Using nitrogen fertiliser to increase dairy farm profitability. Proceedings of the New Zealand Society of Animal Production. p. 117–120.
- McKenzie F. 1996. The influence of applied nitrogen on the vigour of Lolium perenne during the establishment year under subtropical conditions. Tropical Grasslands. 30:345–349.
- McKenzie F, Jacobs J, Kearney G. 2003a. Long-term effects of multiple applications of nitrogen fertiliser on grazed dryland perennial ryegrass/white clover dairy pastures in south-west Victoria. 2. Growth rates, dry matter consumed, and nitrogen response efficiencies. Australian Journal of Agricultural Research. 54:471–476. doi: 10.1071/AR02188
- McKenzie F, Jacobs J, Kearney G. 2003b. Long-term effects of multiple applications of nitrogen fertiliser on grazed dryland perennial ryegrass/white clover dairy pastures in south-west Victoria. 3. Botanical composition, nutritive characteristics, mineral content, and nutrient selection. Australian Journal of Agricultural Research. 54:477–485. doi: 10.1071/AR02189
- McKenzie F, Jacobs J, Riffkin P, Kearney G, McCaskill M. 2003c. Long-term effects of multiple applications of nitrogen fertiliser on grazed dryland perennial ryegrass/white clover dairy pastures in south-west Victoria. 1. Nitrogen fixation by white clover. Australian Journal of Agricultural Research. 54:461–469. doi: 10.1071/AR02187
- Monaghan R, Paton R, Smith L, Drewry J, Littlejohn R. 2005. The impacts of nitrogen fertilisation and increased stocking rate on pasture yield, soil physical condition and nutrient losses in drainage from a cattle-grazed pasture. New Zealand Journal of Agricultural Research. 48:227–240. doi: 10.1080/00288233.2005.9513652
- Morrison J, Jackson M, Sparrow P. 1980. The response of perennial ryegrass to fertilizer nitrogen in relation to climate and soil. Report of the joint ADAS/GRI grassland manuring trial-GM. 20. Technical Report, Hurley: Grassland Research Institute; p. 71.
- Penno J, Macdonald K, Bryant A. 1996. The economics of No 2 dairy systems. Proceedings of the Ruakura Farmers Conference; Hamilton, NZ. p. 11–19.
- Peyraud J, Astigarraga L. 1998. Review of the effect of nitrogen fertilization on the chemical composition, intake, digestion and nutritive value of fresh herbage: consequences on animal nutrition and N balance. Animal Feed Science and Technology. 72:235–259. doi: 10.1016/S0377-8401(97)00191-0
- Pinkerton A, Smith F, Lewis D. 1997. Pasture species. 2nd ed. In: Reuter DJ, Robinson JB, editors. Plant analysis – an interpretation manual. Collingwood, CSIRO Publishing; p. 287–346.
- Rawnsley R, Langworthy A, Pembleton K, Turner L, Corkrey R, Donaghy D. 2014. Quantifying the interactions between grazing interval, grazing intensity, and nitrogen on the yield and growth rate of dryland and irrigated perennial ryegrass. Crop and Pasture Science. 65:735–746. doi: 10.1071/CP13453
- Rugoho I, Gourley CJP, Hannah MC. 2017. Nutritive characteristics, mineral concentrations and dietary cation–anion difference of feeds used within grazing-based dairy farms in Australia. Animal Production Science. 57:858–876. doi: 10.1071/AN15761
- Ryan W, Hennessy D, Boland T, Shalloo L. 2012. The effect of grazing season length on nitrogen utilization efficiency and nitrogen balance in spring-calving dairy production systems. The Journal of Agricultural Science. 150:630–643. doi: 10.1017/S002185961200010X
- SCA. 1990. Feeding standards for Australian livestock. Ruminants. Melbourne: CSIRO Publishing.
- Soil Survey staff. 1987. ‘Keys to soil taxonomy.’ Soil management support services, Technical Monograph No. 6. Ithaca (NY): USDA/Cornell University.
- Staines M, Morris R, Bennett D, White C, Bolland M, Lucey J, Russell W, Windsor D. 2007. Vasse milk farmlets: Productivity, profitability & sustainability of intensive dryland dairying systems in the Mediterranean climate zone of Australia. Final report to Dairy Australia DAW046, December 2007.
- Tharmaraj J, Chapman D, Hill J, Jacobs J, Cullen B. 2014. Increasing home-grown forage consumption and profit in non-irrigated dairy systems. 2. forage harvested. Animal Production Science. 54:234–246. doi: 10.1071/AN12296
- Tille PJ, Lantzke NC. 1990. Busselton, Margaret River, Augusta-land capability study. Land Resources Series-Western Australian Department of Agriculture (Australia).
- Van Soest P, Robertson J, Lewis B. 1991. Methods for dietary fiber, neutral detergent fiber, and nonstarch polysaccharides in relation to animal nutrition. Journal of Dairy Science. 74:3583–3597. doi: 10.3168/jds.S0022-0302(91)78551-2
- Whitehead DC. 1995. Grassland nitrogen. Wallingford: CAB International.
- Williams Y, Wales W, Doyle P, Heard J. 2002. Dry matter intake of dairy cows grazing irrigated perennial pastures estimated by three methods. Animal Production in Australia. 24:265–268.
- Yuen S, Pollard A. 1954. Determination of nitrogen in agricultural materials by the nessler reagent. II – micro-determinations in plant tissue and in soil extracts. Journal of the Science of Food and Agriculture. 5:364–369. doi: 10.1002/jsfa.2740050803
- Zarcinas B, Cartwright B, Spouncer L. 1987. Nitric acid digestion and multi-element analysis of plant material by inductively coupled plasma spectrometry. Communications in Soil Science & Plant Analysis. 18:131–146. doi: 10.1080/00103628709367806