ABSTRACT
The development of economic indexing systems for ranking cultivars of forage species requires new knowledge of factors that may influence the scaling of agronomic data collected in controlled, small-plot evaluation trials to whole farm systems. In the case of perennial ryegrass indexing, one such knowledge gap is the effect of growing clover with ryegrass on the relative rankings of different ryegrass cultivars. The objective of the investigation reported in this series was to test whether the relative yield rankings of perennial ryegrass (Lolium perenne L.) cultivars representing the range in phenotypes now available to New Zealand farmers as a result of recent breeding differed when those cultivars were grown in monocultures (as per standard protocols for cultivar yield trialling systems such as the National Forage Variety Trials) versus mixtures of grass and clover. This paper presents the rationale for the investigation by reviewing developments in perennial ryegrass breeding and evaluation in the context of grass–clover relationships in grazed pasture systems. The papers that follow report results from a multi-year, multi-site investigation comparing the yield, nutritive value and other productivity-related variables of eight perennial ryegrass cultivars grown with or without white clover (monoculture versus mixture respectively) at two levels of nitrogen fertiliser. Key considerations in the design of the experiment on which the investigation was based are discussed.
Introduction
The papers in this series report results from a multi-year, multi-site investigation of the interactions between grass and clover in grazed dairy pastures. The core purpose of the study was to test whether or not the relative yield rankings of different perennial ryegrass (Lolium perenne L.) cultivars (representing the range in phenotypes now available to New Zealand farmers as a result of recent breeding) differed when those cultivars were grown in monocultures (as per standard protocols for cultivar yield trialling systems such as the National Forage Variety Trials, NFVT, Easton et al. Citation2001, and Advanced Yield Trials, AYT, Kerr et al. Citation2012) versus mixtures of grass and clover.
The context for the study was the development of the DairyNZ Forage Value Index (FVI; Chapman et al. Citation2017). The FVI combines performance values (PV) for individual cultivars with economic values (EV; Chapman et al. Citation2012) to estimate total economic merit of cultivars for defined traits relative to a genetic base of older cultivars. It uses agronomic information from the NFVT database to calculate PV. If re-ranking or scaling interactions are present for any traits when cultivars are grown with white clover (Trifolium repens L.), compared with when they are grown as monocultures, then some adjustment for this might be required in the calculation of PV for the FVI. Therefore, failure to incorporate any interaction(s) could lead to inaccuracies in the FVI. It could also undermine farmer confidence in the FVI, since farmers are generally well aware of the value of white clover (Caradus et al. Citation1996b) and consistently include white clover seed when sowing new pastures.
The FVI is a regionally based quantitative indexing system (Chapman et al. Citation2017). Currently, it includes only seasonal dry matter (DM) yield traits using five defined seasons aligned with key stages in the annual dairy production cycle. In contrast to the Pasture Profit Index (PPI) system developed in Ireland (McEvoy et al. Citation2011; O’Donovan et al. Citation2017), which presents a single ranking table for the whole country, a regional approach was deemed necessary in New Zealand to account for differences from north to south in seasonal pasture growth patterns. These have implications for key strategic management decisions such as the date of planned start of calving and result in differences between regions in the EV of additional DM yield by season which can be quite substantial (Chapman et al. Citation2012). Furthermore, analysis of historical NFVT data revealed a significant cultivar × environment interaction in DM yield caused by re-ranking between the upper North Island region compared with other regions (Chapman et al. Citation2017). This evidence dictated that a more-granular approach was needed, accommodating regional EV and PV in a framework that could be built upon progressively as data for other key productivity traits (principally nutritive value and the persistence of yield) become available. In the process of working through the FVI approach, several gaps in scientific understanding of the drivers of whole pasture performance were identified (Chapman et al. Citation2015). New research was initiated to fill these gaps, including the investigation into the effects of grass/clover interactions on DM yield and nutritive value rankings that is reported in this series.
The key question addressed in this investigation was: to what extent do perennial ryegrass cultivars re-rank, and/or how does the range between best and poorest cultivars change, when they are grown in mixed ryegrass/white clover pastures and their performance is assessed in terms of total pasture production compared with ryegrass monocultures? An important supplementary question was: if re-ranking or scaling interactions are observed, how should this be accommodated in the calculation of PV for perennial ryegrass cultivars?
To provide robust answers to these questions, the design of the investigation needed to account for several critical factors, namely:
variation in perennial ryegrass cultivars generated from plant breeding programmes over the past three decades (which, in the New Zealand context, must also take into consideration developments in the associated strain of ryegrass endophyte, Epichloë festucae var. lolii (formerly Neotyphodium lolii, Thom et al. Citation2012; Leuchtmann et al. Citation2014));
variation in the physical environment, especially in climatic factors, and the incidence of insect pest challenges; and
cultivar × environment interactions, where environment includes management factors such as nitrogen (N) and other fertiliser inputs.
Thus, an experiment was established at four sites across New Zealand as described later in this paper and in the subsequent papers of the series. While the focus of the experiment was primarily on the grass × clover interaction term of the statistical analysis (as described later in the General Methods section), the design allowed several more-general observations to be drawn regarding the factors driving productivity of pastures based on modern ryegrass cultivars under current management practices across different climatic environments. In this regard, the investigation provided an opportunity to update the knowledge drawn from earlier studies (e.g. Harris and Thomas Citation1973; Brougham et al. Citation1978; Hoglund et al. Citation1979; Widdup and Turner Citation1983) upon which current expectations of the performance of grass/clover mixtures are based (Chapman et al. Citation1996; Parsons et al. Citation2006).
The papers in this series present the results of the experiment by region (Cosgrove et al. Citation2018; Lee et al. Citation2018; Stevens et al. Citation2018; Chapman et al. Citation2018b), and collectively (Chapman et al. Citation2018a), at two levels: firstly, at the level required to answer the original research question; and secondly at a higher level from which the drivers of productivity of modern grass/clover mixtures can be teased out and compared with our understanding based on past research. Finally, the experiment also provided a venue for addressing questions regarding variation in plant macro- and micro-nutrient content and its causes, and this information is also presented in the series (Crush et al. Citation2018a, Citation2018b). It is relevant to the challenge of rejuvenating the contribution of grass/clover mixtures to New Zealand pastoral systems given the heavy emphasis on N fertiliser use that has emerged in recent years and the implications of this for the mineral nutrition of clover.
The objectives of this paper are to: (1) briefly review developments in perennial ryegrass plant breeding and evaluation over the past few decades, focussing particularly on changes in plant phenotype that may have influenced grass-clover interactions in mixed pastures; (2) briefly review the farm systems, ecophysiological and agronomic factors affecting grass-clover relationships in grazed pastures as a basis for interpretation of findings presented in the papers that follow, and (3) describe the key considerations taken into account in the design of the experiment. More-detailed information on methods used in each region can be found in the relevant papers.
Developments in perennial ryegrass breeding and evaluation
The situation in the late twentieth century
Until the early 1990s, commercial cultivars of perennial ryegrass were based exclusively on New Zealand ecotype collections from sheep-grazed pastures in Hawkes Bay or, after c. 1970, dairy pasture in Mangere, south Auckland (Stewart Citation2006). Cultivars such as Grasslands Nui, Yatsyn and Ellett, all based on the Mangere ecotype, dominated new pasture sowings on dairy pastures throughout New Zealand. These are all defined as mid-season heading cultivars and classified as heading date ‘day 0’ under the New Zealand Plant Breeding and Research Association (NZPBRA) evaluation protocols, meaning that 50% of tillers have initiated reproductive stem elongation by 22nd October in Canterbury. They are diploid, relatively densely tillered cultivars. In the temperate regions of New Zealand where the more intensive forms of pastoral agriculture (dairy, high-producing sheep and beef) are practiced, they display strong winter and spring growth, but only moderate summer growth. This seasonal growth pattern mirrors the climate of these regions which is characterised by year-round rainfall with moderate–strong winter and spring dominance, mild winters, and warm summers with relatively high evapotranspiration rates (White Citation1999).
Early farmer and research experience with these cultivars revealed substantial variation between them in persistency, which retrospectively was explained by differences in ryegrass endophyte infection levels resulting from differences in seed storage conditions. This conundrum was resolved by re-selecting within the original Mangere ecotype gene pool which inadvertently (but advantageously from the perspective of population survival) led to high infection rates of what was later identified as the ‘wild type’ ryegrass endophyte, now renamed ‘standard endophyte’ (SE; Thom et al. Citation2012). Perennial ryegrass in pastures grazed by dairy cows across New Zealand through the 1970s, 1980s and well into the 1990s would almost certainly have been of the Mangere ecotype with high levels of infection of SE, carrying the attendant issues of ryegrass toxicosis in grazing animals caused by endophyte alkaloids which became clear in the late 1980s and 1990s (Thom et al. Citation2012). This was also the period when much of the foundation grazing management research for dairy production systems was carried out in the Waikato (Bryant Citation1984; Bryant and L'Huillier Citation1986; Bryant Citation1990; Macdonald and Penno Citation1998), the results of which could have been influenced to some extent by the plant–animal interactions associated with the combination of diploid mid-season heading cultivars and SE (Fletcher and Harvey Citation1981; Gallagher et al. Citation1981; Van Heeswijck and McDonald Citation1992). Of particular relevance to this series of papers, the same plant-endophyte complex could also have influenced grass-clover interactions indirectly through animal grazing behaviour but also through the protection from insect damage afforded by SE to ryegrass. Both pathways would likely have helped sustain the competitive advantage of perennial ryegrass relative to clover. Subsequent developments in perennial ryegrass breeding and endophyte strain selections have markedly changed many of these interactions, as described below, and this should be borne in mind when interpreting and applying the findings of research from the late twentieth century to current dairy systems.
Changes since c. 1990
The major developments in plant breeding since c. 1990 have been: the inclusion of Spanish germplasm in crosses with adapted New Zealand material which, among other outcomes, has delayed heading date by up to 25 days in diploid cultivars and improved summer growth (Stewart Citation2006); the introduction of tetraploids, initially of Mangere ecotype material but subsequently incorporating European germplasm; and the identification of genetic variation in the ryegrass endophyte and introgression of ‘novel’ endophytes with different alkaloid profiles compared with SE into ryegrass cultivars. The latter development essentially mandates that perennial ryegrass cultivars must now be regarded as a combination of two genomes, the ryegrass host plant and the associated endophyte strain, rather than simply the plant genome [Note: for simplicity, the term ‘ryegrass cultivar’ is used in this series except in situations where the host plant-endophyte complex must be specified in order to interpret results]. This has many implications for ryegrass evaluation methods (including the FVI system), and farmer selection of the best germplasm for their unique farm environment. There has also been some penetration of European cultivars bred for high water soluble carbohydrate (WSC) content in leaf material into the New Zealand market.
The result is that dairy farmers in New Zealand now have more than 30 perennial ryegrass cultivars to choose from when sowing new pastures (refer to FVI 2017 lists), ranging in heading date from day 0 to day 25. The list is still dominated by diploids but the Spanish germplasm, in particular, has altered the morphology of plants by increasing leaf/tiller size without compromising tiller density (Griffiths et al. Citation2017). The smaller number of tetraploid cultivars in the FVI lists conform to the phenotype confirmed in European studies (Neuteboom et al. Citation1988; Swift et al. Citation1993), being more ‘open’ with larger leaves and higher leaf:stem ratio compared with diploid cultivars (Griffiths et al. Citation2017).
The key question for farmers is, what do these developments mean in terms of productivity gains? Lee et al. (Citation2012) reviewed published studies of genetic gain in ryegrass DM yield from New Zealand and internationally. The mean of the four studies available for New Zealand was 5% per decade (range 2.5–7.3%), which aligns with overseas data-sets (range 2.5–6% per decade; Wilkins and Humphreys Citation2003; McDonagh et al. Citation2016). More recently, Harmer et al. (Citation2016) have proposed two phases of gain in DM yield based on trial data from Australia and New Zealand: one prior to 1990 where the rate of gain was negligible (about 20 kg DM/ha per decade); and one post-1990 showing a sharp increase to 1050 kg DM/ha per decade (7.6%). Importantly, the yield gains reported by Harmer et al. (Citation2016) have mainly come outside the peak growth period of late spring, thus helping reduce the seasonality of pasture growth by increasing early spring, summer and autumn growth. Additional DM from pasture in these seasons has a higher economic value to dairy farm systems than additional DM in the late spring period (Chapman et al. Citation2012, Citation2017).
Harmer et al. (Citation2016) proposed that the acceleration in gains post-1990 reflects the increased breeding diversity described above, facilitated by the introduction of Plant Variety Rights legislation in New Zealand, and Plant Breeders Rights in Australia, in the early 1970s and subsequent commercial investment in breeding. Concurrently, public investment in breeding programmes for the mainstream species such as ryegrass and white clover was phased out as investment in public good research shifted to areas where market failure was evident. Notably, there are only two published analyses of rates of genetic gain in ryegrass for the other key productivity trait of nutritive value. In Europe, Sampoux et al. (Citation2011) reported genetic changes in lignin content, neutral detergent fibre (NDF) content, in vitro digestibility of NDF, WSC content, and in vitro dry matter digestibility (DMD) over 40 years of perennial ryegrass breeding of −1.58%, −0.71%, 1.0%, 5.0% and 0.49% per decade respectively, relative to the overall cultivar mean. There was no statistically significant trend in the crude protein (CP) content of ryegrass over that time. In contrast, McDonagh et al. (Citation2016) reported that there was no evidence of improvements in digestibility over a 34-year period (1980–2013) in Northern Ireland.
Of relevance to this series of papers, reported rates of genetic gain in DM yield in white clover in New Zealand are higher than those for perennial ryegrass: 6% per decade (Woodfield and Caradus Citation1994); and 12–15% per decade (Woodfield Citation1999). No analyses have been published subsequently, however Widdup and Barrett (Citation2011) reported that cultivars of white clover released around the year 2000 display higher stolon density within their leaf size class than their antecedents. This indicates that a shift in plant morphology has been achieved which should favour improved persistence of medium and large leaved clover phenotypes with higher yield potential.
Cultivar evaluation
While the published information on rates of gain in perennial ryegrass and white clover is encouraging, it comes entirely from small-plot yield evaluation trials. These trials are conducted under prescribed management protocols designed to minimise the likelihood of factors such as soil fertility or defoliation management limiting plant growth and therefore the expression of genetic differences (Grogan and Gilliland Citation2011). There is little direct evidence available from which to assess how well the differences observed in small-plot studies translate to the level of the whole farm system and, hence, potential impacts on farm production and profit. This is a scaling issue, within which several factors such as the standard of pasture and defoliation management, spatial and temporal variation, and plant–animal (including invertebrates) interactions are nested. The effects of ryegrass breeding on grass-clover interactions also sit in this category, since small-plot grass evaluation studies are almost always conducted using grass monocultures. Leaving aside these uncertainties, Chapman et al. (Citation2017), using the FVI, calculated that perennial ryegrass plant breeding is delivering between $120/ha and $180/ha increased profit per decade. By comparison, dairy cattle breeding is delivering an estimated $110/cow per decade increased profitability (Chapman et al. Citation2015), or >$300/ha per decade at average industry stocking rates.
There are many assumptions involved in such extrapolations, and ultimately it is the animal production response per hectare that matters in judging the rate of genetic progress. The type of experiments required to measure this are difficult and expensive to run. A notable example of such an experiment was reported by Crush et al. (Citation2006), who compared animal production from pastures based on all combinations (n = 4) of ‘old’ (bred in 1980s) and ‘new’ (released ca. 1998) perennial ryegrass and white clover cultivars in a replicated farm systems experiment over four years. Crush et al. (Citation2006) observed no significant differences in milk production and economic farm surplus between the ‘new’ and ‘old’ perennial ryegrass cultivars over four lactations. On a seasonal basis (spring, summer, autumn, winter), the perennial ryegrass content (% DM) of pastures based on ‘new’ ryegrass cultivars was significantly greater than that of pastures based on ‘old’ cultivars about 50% of the time, and pasture growth rates were higher for ‘new’ than ‘old’ about 15% of the time. However, these differences were clearly insufficient to drive differences in animal production that could be detected with the number of replicates available.
Of relevance to this series of papers, the only statistically significant animal production response reported by Crush et al. (Citation2006) was due to clover type in the third year of the experiment where higher total milksolids per hectare was observed for pastures based on the ‘new’ clover cultivars than for pastures based on the ‘old’ clover cultivars. This was a result of higher clover content, and greater nutritive value, in the ‘new’ clover pastures, especially in summer and autumn of that year. This, plus the observation that both the new ryegrass and new clover cultivars showed evidence of better persistence than the old cultivars, led Crush et al. (Citation2006) to conclude that ‘progress has been made in cultivar development’. However, they also cautioned that ‘herbage gains demonstrated in small plots are not necessarily robust indicators of performance in larger scale, self-contained farmlets’.
Implications of breeding and evaluation developments for grass-clover interactions
Several points emerge from the review above that support the argument for a re-appraisal of grass-clover dynamics in today’s grazed dairy pastures. Firstly, changes in the seasonal pattern of distribution of ryegrass yield through breeding later-heading cultivars could impact on grass-clover interactions. Increased summer growth of perennial ryegrass may increase competition from ryegrass during this period, when warmer temperatures are generally thought to favour white clover growth (optimised at c. 24°C; Mitchell Citation1956) over ryegrass growth (optimised at c. 18–21°C; Mitchell Citation1956). There is ample empirical evidence which shows clover content in mixtures peaking in summer (Brougham Citation1959; Harris and Hoglund Citation1977), supporting the idea that white clover gains some competitive ground with perennial ryegrass at this time, ground which could be lost if ryegrass growth in summer is increased through genetic selection. In addition, Collins and Rhodes (Citation1989), Davies and Fothergill (Citation1990) and Gilliland (Citation1996) all observed lower clover content in mixtures based on late heading ryegrasses than mixtures based on earlier heading varieties in Europe. While there are no data available for New Zealand conditions, there is no reason to expect a different outcome. Therefore, the relative total productivity (accounting for clover presence) of pastures based on ryegrass cultivars with different heading dates could differ from that observed when the same cultivars are grown as monocultures. The effect of this factor, plus others discussed below, will of course depend on the amount of clover present in the mixture.
Secondly, the advent of tetraploid ryegrasses in the New Zealand market could also alter competitive interactions if the inverse relationship between ryegrass tiller density and clover content proposed by Brereton et al. (Citation1985) holds across temperate regions, and the differences in tiller density recorded in Europe (Neuteboom et al. Citation1988; Swift et al. Citation1993) are also evident in New Zealand cultivars. Brereton et al. (Citation1985) observed a transition from clover dominance to clover suppression when ryegrass tiller density increased above about 5000/m2, but noted that the transition point appeared to ‘vary with morphology of the companion grass’. In a survey conducted in autumn of pastures on dairy farms in the Waikato/Bay of Plenty, Taranaki, and Canterbury regions of New Zealand, Tozer et al. (Citation2014) recorded mean tiller densities of tetraploid pastures which were almost half those in mid- and late heading diploids (1800 versus 3000 tillers/m2, respectively). While all pastures were below the 5000 tillers/m2 threshold proposed by Brereton et al. (Citation1985), autumn is when tiller density is at its lowest point in the annual cycle, and densities increase rapidly once soil moisture conditions improve. The relationship proposed by Brereton et al. (Citation1985) has not been systematically investigated, although the notion that more-densely tillered ryegrass types can suppress clover content does appear in the literature (e.g. Rhodes Citation1981) despite evidence that tetraploids do not generally support higher clover content than diploids (Elgersma and Schlepers Citation1997; Cougnon et al. Citation2012). However, the possibility cannot be ignored.
Thirdly, the unfolding of the ryegrass endophyte story (Thom et al. Citation2012) has posed further challenges to the elucidation of the drivers of ryegrass-clover dynamics in dairy pastures in New Zealand. The protection conferred to ryegrass plants against highly damaging pests such as Argentine stem weevil (ASW; Listronotus bonariensis) and black beetle (Heteronychus arator) by some endophyte strains can play a major role in controlling perennial ryegrass population survival, especially in the upper North Island of New Zealand. When a ryegrass cultivar that does not contain the appropriate controlling endophyte is sown and pest damage subsequently occurs, the rapid drop in ryegrass presence can release white clover from some of the competition pressure previously exerted. In turn, this can lead to an increase in clover content. For example, Thom et al. (Citation2014) observed that, in March 2008 (three years after sowing), pastures sown with AR37 endophyte had double the perennial ryegrass tiller density of pastures sown with AR1 endophyte (3420 versus 1680 tillers/m2 respectively) due to lower insect pest pressure in AR37-sown pastures. Conversely, white clover content was significantly higher in pasture sown with AR1 than in pastures sown with AR37 (8% versus 2% of total DM respectively). Thom et al. (Citation2013) reported that these effects were sufficient to create differences in animal production between endophyte-protected and non-protected treatments. This is an indirect effect of endophyte that can be controlled by correct selection of the endophyte strain to counter the expected pest problems. There have also been suggestions of direct effects of endophyte on clover growth in mixtures (e.g. Eerens et al. Citation1998 and references therein), but the results have been equivocal. However, again, the possibility cannot be ignored.
In the same way that endophyte can influence clover-ryegrass interactions so too do the feeding preferences for clover or ryegrass of pest insects and nematodes that are not affected by endophyte. Those interactions will also affect the population dynamics of the pests via resource availability. Clover root weevil (Sitona obsoletus), white-fringed weevil (Naupactus leucoloma) and various nematodes species, including root knot nematode (Meloidogyne spp.), clover cyst nematode (Heterodera trifolii) and lesion nematode (Pratylenchus spp.), all feed primarily on clover. Reducing the impact of nematodes (Mercer et al. Citation2008) or clover root weevil (CRW) (Gerard et al. Citation2007) leads to clover dominance while black beetle damage to ryegrass can increase clover content but also that of unsown species (Blank and Olson Citation1988). Others such as the endemic grass grub (Costelytra giveni, formerly C. zealandica) are more omnivorous but nevertheless exhibit a preference for clover over ryegrass such that populations tend to be higher where clover is present (Kain and Atkinson Citation1977). When such changes persist across generations, pest populations will respond positively or negatively to these fluctuations in their resources. Nitrogen fertiliser has less consistent effects on insects than presence of host species. An N application increased damage to ryegrass by ASW leading to reduced ryegrass content (Hunt et al. Citation1988) but had little or no effect on grass grub relative to the effect of host species (Prestidge et al. Citation1985). Differential effects of invertebrate herbivory are only part of the equation as any changes occurring as a direct result of their feeding also influence interspecific competition within the sward.
Finally, evidence that total annual perennial ryegrass DM yield is increasing by around 1 t DM/ha per decade since ca. 1990 (Harmer et al. Citation2016, and above) raises the possibility that the overall intensity of competition for resources from the grass on the clover content of mixtures is also increasing commensurately. This effect is likely to be most acute in summer as described above, but could conceivably compound if present in other seasons as well. It is well known that there is an inverse relationship between grass growth and clover content in mixed pasture (e.g. Cowling and Lockyer Citation1965). The most clear-cut example of this is the strong negative effect of increased rates of N fertiliser on clover %, as described in a later section. In this context, it is notable that N fertiliser use has increased substantially in dairy pasture systems in New Zealand over the last three decades (as described later). The obvious counter to this trend would come from commensurate rates of genetic gain in the growth and competitive ability of white clover: however, as noted above, there is no recent evidence available to judge whether or not this has been achieved.
Basis for possible re-ranking of ryegrass cultivar performance with versus without clover
The preceding section illustrates that recent developments in breeding have created phenotypic variation in perennial ryegrass that could potentially change the relative agronomic performance of different perennial ryegrass cultivars when grown in mixtures compared with monocultures. A necessary condition for differences in relative agronomic performance to be expressed is that the presence of clover alters the productive properties of mixed pastures compared with monocultures. There are three main ways in which this could occur:
via transfer of biologically fixed N from clover to grass which increases grass growth in the mixture;
via a direct yield benefit of the clover over-and-above that of the grass; and
via increased nutritive and/or feeding value of the pasture.
There is a wealth of evidence for the existence of all three pathways (see, for example, reviews by Ledgard and Steele Citation1992, and Nicol and Edwards Citation2011 for the first and third pathways, respectively). The direct yield pathway (#2) generally receives less emphasis than the other two in articles describing the benefits of white clover (and other legumes) in mixtures. This is despite the seminal work on the complementarity of the seasonal growth patterns of temperate grasses and legumes (Brougham Citation1959; Harris and Hoglund Citation1977) which allows increases in total annual herbage yield above those achievable by monocultures of either species (Harris and Hoglund Citation1977; Harris Citation2001; Ledgard et al. Citation2001).
In the context of the research question, for any of these pathways to work, the phenotypic and other factors described in preceding sections must result in different contents of white clover in the mixed sward, and those compositional differences must drive different rates of change in the functional properties. The key term in the statistical model used to analyse the data from the study (see description in the General Methods section) is the clover × cultivar interaction term. Statistical significance in this term could arise from re-ranking interactions, or from scaling interactions, which could play out in different ways as shown in and . Re-ranking could occur among ryegrass cultivars if some phenotypes ‘allow’ clover to grow more vigorously in the mixture than others, irrespective of whether the ryegrass cultivars do or do not differ in yield in monoculture (e.g. (A, B) versus (C)). Interactions are possible even if the absolute ryegrass yield does not differ between monocultures and mixtures. Also, substitution of grass yield with clover yield (e.g. (D), (E) and (F)), or facilitation of additional grass yield via the N fixed by clover, could still occur without dampening the interaction.
Figure 1. Hypothetical examples of re-ranking interactions in ryegrass yield between monocultures (‘mono’) and grass–clover mixtures (‘mixture’). Black bars = ryegrass yield; grey bars = clover yield. In examples A–C, ryegrass yields do not differ between monocultures and mixtures, while in D–F there is some substitution of ryegrass yield by clover. Re-ranking may occur when ryegrass cultivar yields differ in monoculture (examples A and B, and D and E), or when cultivar yields are similar in monoculture (C, F).
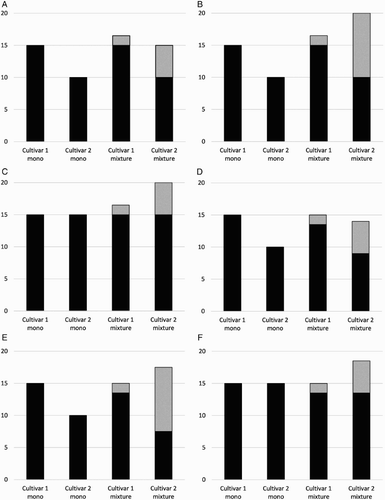
Figure 2. Hypothetical examples of scaling interactions in ryegrass yield between monocultures (‘mono’) and grass–clover mixtures (‘mixture’). Black bars = ryegrass yield; grey bars = clover yield. In example A, ryegrass yields do not differ between monocultures and mixtures, while in B there is some substitution of ryegrass yield by clover.
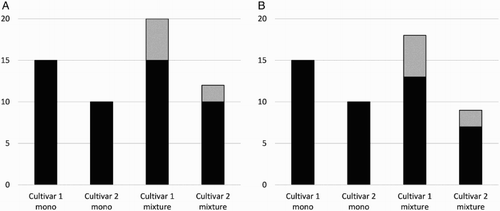
Concepts of competitive and combining ability have been developed by various authors (Harris and Thomas Citation1973; Rhodes Citation1981) to explain how breeding selection for particular phenotypes, either grass or clover, can influence the interactions and help increase total yield of swards. These concepts have been embedded in breeding objectives. For example, in the 1980s and 1990s, UK breeders selected for relatively long leaves and erect tillers in ryegrass to allow light to penetrate deeper into the sward so that clover leaves can compete more effectively for light compared with dense, prostrate leaved ryegrass phenotypes that maintain a high leaf area index at the top of the canopy and force clover to elevate lamina on longer petioles to reach the light (Rhodes Citation1971; Woledge et al. Citation1992). Essentially, the aim was to breed a ryegrass cultivar that behaved like cultivar 2 in (C): able to maintain yield potential in the mixture but allow clover to exploit growth resources better and increase total herbage yield.
While such breeding programmes did not lead directly to ryegrass cultivars with improved clover compatibility traits, white clover phenotypic selections have successfully exploited the principles of competition and combining ability via the highly heritable trait of leaf size (Woodfield and Caradus Citation1990; Caradus and Chapman Citation1996). Thus, large-leaved cultivars have been bred to achieve relatively high clover leaf area index in the upper sward layers of pastures based on erect perennial ryegrass types, such as those used in rotationally grazed, high-fertility dairy pastures (Caradus et al. Citation1996a; Woodfield et al. Citation2001). However, the same phenotype is generally not suitable for the more-dense, closely grazed swards that typically develop in sheep-grazed pastures, where small-leaved cultivars with their associated dense stolon network are more persistent (Caradus et al. Citation1996a). This important management × phenotype interaction was demonstrated by Brock (Citation1988) who compared four white clover cultivars with different leaf sizes under rotational and continuous grazing by sheep at the same stocking rate. In that experiment the ranking order of clover phenotypes was completely reversed under rotational grazing (large-leaved material ranked highest) compared with continuous grazing (small-leaved material ranked first).
Empirical evidence for re-ranking; and a hypothesis
Several studies have compared yields of different perennial ryegrass cultivars, or different grass species, in monoculture or in mixtures with white clover. Often these studies were conducted to investigate combining ability and the potential to raise total herbage yield above those possible with monocultures, rather than to assess whether re-ranking occurred. The results are equivocal: although most studies found little evidence of differences in combining ability and subsequent relative yield rankings (sometimes yield was not measured and the clover content of the mixture was used to assess compatibility), a smaller number have shown interactions. Some of these studies are described here; further information can be found in Rossi (Citation2016).
Cowling and Lockyer (Citation1965) compared three cultivars of perennial ryegrass and four other species (cocksfoot, Dactylis glomerata L.; timothy, Phleum pratense L.; meadow fescue, Festuca pratensis; and browntop, Agrostis tenuis) grown with and without clover under an infrequent cutting management and concluded that ‘The yields of grasses when sown with clover were in similar order to their yields when sown pure.’ Connolly (Citation1968) observed no significant interaction between grass and clover when the yield of all combinations of three varieties of perennial ryegrass and six varieties of white clover were compared under sheep grazing for three years. There was a trend toward mixtures based on the earlier-heading New Zealand ryegrass variety to maintain a higher clover content than S.23 and Glasnevin, but this difference was in general too small to reach statistical significance.
Chestnutt and Lowe (Citation1970) reviewed 22 studies conducted in the UK and Ireland where the clover content of mixtures based on between two and six different perennial ryegrass cultivars was reported. Compared with the mixture with the highest clover content (given a relative score of 100 by Chestnutt and Lowe, Citation1970), the relative clover content of the mixture with the lowest clover content was seldom less than 80 in each of the 22 studies. Chestnutt and Lowe (Citation1970) did note, however, that ‘ … it would appear that Aberystwyth cultivars were somewhat less compatible with clover than Irish … ’ and New Zealand varieties. They explained that the Irish variety ‘ … has a lower persistency than S23 … ’ (the standard Aberystwyth variety at the time) and could therefore be expected to be less aggressive toward white clover. Williams et al. (Citation2000) compared mixtures of all combinations of four ryegrass and two clover cultivars with ryegrass monocultures in a grazing study over four years. The ryegrasses used were: two tetraploid hybrids between perennial and Italian ryegrass; a diploid Italian ryegrass; and a tetraploid perennial ryegrass. The authors concluded that ‘Grass yields in mixture were greater than in monoculture but showed similar relative rankings.’
A key study by Camlin (Citation1981) assessing the competitive relationships between three white clover cultivars with different leaf size (from small to medium–large) and 10 perennial ryegrass cultivars (from early to late season ryegrass) receiving N fertiliser (200–240 kg N/ha/year) revealed that the medium–large leaved clover cultivar (Kersey) was more aggressive toward grass, produced a greater contribution to total herbage yield, and depressed the yield of some of the companion grass cultivars. The mean (over three years) clover content of the mixtures sown to Kersey was 20% of total DM yield, whereas the mean clover content in mixtures based on the other two clover cultivars (S100 and S184) was between 8% and 10% of total DM yield. The results also showed that the compatibility of the ryegrass cultivars with clover was inversely related to persistence. Camlin (Citation1981) concluded that
The interactions revealed in the experiment showed that both ryegrass and clover cultivars have the potential to influence each other when in association although, with minor exceptions, total annual yields were similar for all grass and clover mixtures at the moderately high level of N applied.
Another important conclusion of this work was that differences in total herbage yield tended to reflect differences in the yield of the grass component.
In contrast, Davies et al. (Citation1993), in a comparison of three perennial ryegrass cultivars (an early flowering diploid, a late flowering diploid, and a late flowering tetraploid) grown as monocultures or in mixtures with clover and grazed by sheep, highlighted ‘the large difference between varieties when assessed as grass/clover swards compared with the small differences between them as grass-only swards. … . This was attributed to differences between the grasses in their compatibility with white clover’. While there was no significant difference in yield among the cultivars when grown in monocultures, the highest-ranked cultivar (the early diploid) out-yielded the lowest cultivar (the late diploid) by 21% (1.3 t DM/ha) when grown in mixture with clover, reflecting the higher clover content in the early diploid (13% of total DM versus 5% for the respective cultivars). These results indicate a re-ranking interaction of the type shown in (C) although, in the Davies et al. (Citation1993) experiment, the ryegrass yields were lower in the mixture treatments because no N was applied (apart from 75 kg N/ha in the first of the three years) whereas the monoculture treatments received 200 kg N/ha.
Munro et al. (Citation1992) drew a similar conclusion from their comparison of diploid perennial ryegrass cultivars with different flowering sown as grass-only or grass/clover swards and grazed by sheep at a lowland site in Wales. They observed a scaling interaction whereby the annual lamb production from grass/clover swards of the very early, early, intermediate and late cultivars, respectively, was ‘127, 124, 117 and 100% of that from their corresponding grass-only treatments’. They attributed this to differences among the cultivars in compatibility with white clover, whereby mean clover contents ranged from 15.3% in swards of the very early flowering cultivar to 9.6% in swards of the late flowering cultivar.
While there is large variation among these studies in the types of ryegrasses (or grasses, in the case of studies that compared different species) and clover used, defoliation management, fertiliser inputs and other factors, most seem to indicate that measurable relative yield rank changes between monocultures and mixtures of different ryegrass cultivars are unlikely to occur. Hence, the hypothesis for the investigation reported in this series was that the relative yield rankings of perennial ryegrass cultivars do not differ when those cultivars are grown as monocultures or as mixtures with white clover and that this would apply at both low and high levels of N fertiliser application. Importantly, the hypothesis was tested in multiple environments using a structured range of recently-released cultivars of perennial ryegrass managed under intensive dairy cattle grazing to impose rates of pasture utilisation typically achieved on high-performing dairy farms.
The inclusion of multiple climatic environments was considered a key requirement because: (a) the six studies briefly reviewed above were all conducted in the UK and Ireland in environments that were quite similar to each other and different from New Zealand where dairying is distributed from the sub-tropical far north of the country to the cool-temperate south; (b) climate strongly influences pasture production variables, especially the seasonal pattern of growth and botanical composition; and (c) differences between perennial ryegrass and white clover in their response to e.g. temperature (as described earlier) could lead to interactions with climate that result in different grass–clover relationships among regions. Thus, the possibility that the hypothesis may be supported in some environments but not in others must be considered. This is pertinent because the FVI is stratified into four zones (as described later in the General Methods section) to account for the effects of regional environmental differences on farm systems management and for perennial ryegrass cultivar x region interactions in ryegrass DM yield (Chapman et al. Citation2017).
The context of previously published studies, both in the types of germplasm used and the sward management practices applied, was quite different from the context of this investigation. Developments in perennial ryegrass germplasm that are relevant to the current study have already been reviewed in previous sections. However, further context for the management changes that are relevant to the investigation is also needed, and this is now presented in the following section.
Grass-clover interactions in grazed pastures: system, ecological and agronomic processes
The traditional reliance of New Zealand’s pastoral industries on grass/clover pasture mixtures for feeding livestock has been well documented (e.g. Brock et al. Citation1988; Woodfield Citation1996). Since the early 1900s, well-adapted legume species such as white clover have provided the key to building fertility in soils with naturally low levels of N and other macronutrients, thereby raising grass growth and overall pasture productivity to support increased meat, wool and milk production. The resulting grass/clover association was viewed as a natural system that did not require inputs of N thus obviating the need for expending energy on the manufacture of artificial fertiliser.
Fracture lines in this foundation of low-cost, pasture-based production began to appear in the 1980s, with observations of strong pasture growth and animal responses to N fertiliser applied tactically in winter/early spring to overcome feed shortages (e.g. Ball et al. Citation1976; Field and Ball Citation1978; Bryant et al. Citation1981). By the early 1990s, tactical use of N fertiliser to increase cool season pasture growth was becoming commonplace as particularly dairy farmers recognised the value of this additional feed in early lactation and the possibilities for increasing stocking rate and milk production that it presented. From here, change occurred quickly taking N fertiliser from a tactical management input on dairy farms to a strategic input where it is used year-round to increase total pasture supply. In the period 1990–2003, total N fertiliser usage across the New Zealand pastoral industries grew from approximately 50,000 tonnes per year to 350,000 tonnes per year (MPI Citation2012), with most of this increase in the dairy sector. According to Harris et al. (Citation1996), N fertiliser use on New Zealand dairy farms increased eightfold from 1988 to reach an average of 90 kg N/ha per year in 1994. In the three-year period 2008/2009 to 2010/2011, weighted mean N fertiliser application on dairy farms was 150 kg N/ha per year (data from DairyBase: pers. comm), ranging from around 120 kg N/ha per year in Taranaki to 240 kg N/ha per year in Canterbury, and amounting to a total of approximately 170 m tonnes N applied annually across the industry.
Nitrogen fertiliser is a powerful tool for increasing grass growth. However the direct outcome of this is an increase in the competitive advantage of the grass and suppression of clover growth. The ecophysiological processes involved in the competitive dynamics of grass/clover mixtures have been thoroughly documented (e.g. Parsons et al. Citation1991; Woledge et al. Citation1992; Nassiri and Elgersma Citation1998), reviewed (e.g. Haynes Citation1980; Davies Citation2001), and modelled (e.g. Schwinning and Parsons Citation1996a, Citation1996b). The agronomic outcomes have been demonstrated consistently in numerous empirical studies conducted in temperate pasture systems (e.g. Ball and Field Citation1982; O’Connor Citation1982; Harris et al. Citation1996). In a study comparing ryegrass monocultures with grass/clover mixtures under dairy grazing in Ireland, Enriquez-Hidalgo et al. (Citation2015) reported a mean (over three years) reduction in white clover yield in mixtures of 8 kg DM/ha for every 1 kg N fertiliser increase over the range 0–240 kg N/ha per year. The corresponding decline in biological N fixation was estimated to be around 0.5 kg N fixed per kg N fertiliser added (N fixation efficiency averaged 44 kg N/tonne clover DM across all treatments and years, Enriquez-Hidalgo et al. Citation2015). If we assume about 50% of the N fixed becomes available for associated grasses through N cycling processes (Ledgard Citation1991), then the net gain in the supply of additional N for grass growth in the mixed pastures is about 25% less than that implied by the N fertiliser rate.
In New Zealand, Ball and Field (Citation1985) collated results from grazing trials reported by Harris et al. (Citation1973), Ball (Citation1979) and Harris and Hoglund (Citation1977), plus one unpublished trial, where perennial ryegrass and white clover yields were measured under different N application rates up 450 kg N/ha per year. This analysis revealed a reduction of 1 t DM/ha per year in clover yield for every 3 t DM/ha per year increase in grass yield. Using the commonly accepted N fertiliser response efficiency of 10 kg grass DM per kg N applied (Clark and Harris Citation1996), the relationship reported by Ball and Field (Citation1985) is equivalent to a reduction in white clover yield of around 3.3 kg DM/ha for every 1 kg N fertiliser applied. Even if a response efficiency of 15 kg DM/kg N was assumed, the estimated reduction in clover yield is 5 kg DM/ha per kg N applied, still considerably less than the 8 kg clover DM reduction reported by Enriquez-Hidalgo et al. (Citation2015). Ball and Field (Citation1985) noted that Ennik (Citation1982), in a review of trials conducted in the Netherlands, reported a reduction of 1 t DM/ha per year in clover yield for every 1.75 t DM/ha per year increase in grass yield (equivalent to around 5.7 kg DM reduction in clover yield for every 1 kg N applied, if the same assumption about N response efficiency, above, is used). They noted that the apparent greater stability of clover yield production in mixtures fertilised with N in New Zealand compared with north-west Europe ‘arises from less overlap of the period of grass responsiveness to N and the period when most legume growth occurs’.
Given the depth of scientific information on the negative relationship between N fertiliser use and clover yield in mixed pastures, the steep rise in N fertiliser use on New Zealand dairy farms over the past 2–3 decades described above provides a large part of the explanation for low clover contents in dairy pastures noted by many authors (e.g. Ball and Field Citation1982; O’Connor Citation1982; Harris et al. Citation1996). Using the relationships reported by Enriquez-Hidalgo et al. (Citation2015), the weighted mean 150 kg N/ha fertiliser rate used on New Zealand dairy farms in 2008/2009 to 2011/2012 (see above) could be associated with 1.2 t less white clover DM/ha per year, and a reduction in N fixation of 75 kg N/ha per year, compared with the pre-N fertiliser days of dairy farming (net gain of additional N for grass growth = ∼120 kg N/ha). Nonetheless, the gross benefit for pasture growth is still substantial if a ‘standard’ N response efficiency of around 10 kg DM/ha per kg N fertiliser applied (to, generally, mixed pastures) is assumed (Ledgard et al. Citation2001). Furthermore, assuming a cost of around $NZ1 per kg N applied (including application costs), and a standard response of around 1 kg milksolids (MS) per 13 kg feed DM offered (Wales and Kolver Citation2017), N fertiliser use is a much cheaper source of extra feed than imported supplements for New Zealand dairy farmers: hence its near-universal adoption as a strategic farm input over the past several decades.
One aspect of these trends that has received minimal attention is the resultant mineral nutrient composition of herbage and implications for pasture and animal production, and the environmental impacts of dairying. Clover can initially dominate swards sown on nitrogen-impoverished soils because its symbiotic nitrogen fixation capacity means it is independent of the soil N supply. However, as the supply of soil mineral N increases, either through mineralisation of soil organic N accumulated over time or directly from N fertiliser, clover loses its competitive advantage and grasses become dominant (Sears Citation1962; Schwinning and Parsons Citation1996a). Because much less clover than grass herbage is grown in this situation, the grasses represent larger sinks for the macro- and micro-nutrients essential for plant growth. For example, the grass component of Waikato dairy pastures growing 17.2 t DM ha−1 with 18% clover in the herbage contains 5–6 times more P, K and S than the clover component (Crush et al. Citation2006). Because clover competes poorly against grasses for nutrients such as P, the P fertiliser requirement of mixed swards is up to three times greater than that needed to grow the components separately (Dunlop and Hart Citation1987).
There is genetically controlled variation in the mineral composition of ryegrass (Butler et al. Citation1962) however there are no published data available on the foliar mineral composition of the ryegrass cultivars currently sown in New Zealand. This information is required to determine whether or not changes in pasture composition over recent decades have consequences for fertiliser recommendations, animal production, or the environmental impacts of nutrient losses from pasture-based livestock systems: and, if so, whether there are opportunities to exploit current genetic variation to address the resultant issues. The field experiment reported in this series of papers provided an opportunity to quantify the mineral profiles of contemporary ryegrass cultivars and assess the potential for modification of clover–grass nutrient relationships for economic and environmental benefit. The results of this analysis are reported in the two papers that complete this series (Crush et al. Citation2018a, Citation2018b).
General methods
Overall considerations and constraints
As noted in the Introduction, the design of the investigation needed to account for variation among perennial ryegrass cultivars, variation among physical environments (since cultivar × environment interactions in perennial ryegrass yield have been observed in the development of the FVI, Chapman et al. Citation2017), and the effects of management factors. The number of levels chosen for each factor was eight for perennial ryegrass cultivar, four for environment and two for management (‘low’ or ‘high’ N fertiliser). Perennial ryegrass cultivars were selected such that each of two morphological contrasts (fine leaved and dense versus large leaved and open) and two heading date contrasts (mid-season and late season) were nested in the design, each represented by two cultivars (). The contrasts were included so that, if significant re-ranking or scaling interactions between ryegrass cultivars and clover were observed, this structure may allow identification of the key phenotypic factor(s) involved. This could then allow adjustments to be implemented in the FVI (if necessary) based on functional type categories (e.g. ploidy level, or heading date) to which any individual cultivar can be assigned based on its breeding and/or phenotype, rather than requiring cultivar-specific evaluation data. In practice, it was impossible to construct rigorous contrasts because the phenotypic traits of interest were confounded by variation in other traits. Nonetheless, the results of the contrasts are included in this series if the two cultivars in each pair did not differ significantly for key variables, and if this information assists interpretation of the overall trends.
Table 1. Perennial ryegrass cultivars used to investigate interactions between ryegrass phenotype and white clover in grazed dairy pastures.
Nor was it possible to completely balance all cultivars or contrasts for endophyte strain because the cultivars chosen were not all marketed with the same endophyte. Cultivars without endophyte, or with standard endophyte, were avoided, leaving AR1, AR37 and NEA2/6 as the choices available. Further detail regarding their efficacy against the two major insect pests (via their unique alkaloid profile) Argentine stem weevil and black beetle was presented by Thom et al. (Citation2012). In brief, all are effective against the former. AR37 and NEA2/6 offer moderate–high protection against black beetle but AR1 provides little protection against this pest. Thus, there was a risk that a black beetle outbreak at the Waikato site of the experiment (black beetle is only present in damaging numbers in northern New Zealand; Bell et al. Citation2011) could have compromised the integrity of the experiment. This did not happen and ryegrass tiller density remained relatively consistent across treatments at this site (Lee et al. Citation2018), though the existence of other effects of variation among endophytes could not be ruled out.
Finally, the requirement that all treatments be grazed by dairy cattle under best practice grazing management for dairy farms (e.g. Macdonald and Penno Citation1998) forced the adoption of a split-plot design since it was impossible to individually graze all replicates of all treatment combinations. To avoid N transfer between the low and high N fertiliser treatments, and remove the (strong) risk of preferential grazing of mixture plots containing clover over monoculture plots (Rutter Citation2006; Chapman et al. Citation2007), all combinations of N treatment by pasture type treatment (monoculture or mixture) served as main plots. Each main plot was grazed individually within each block. The REML model used in Genstat (VSN International Ltd. Citation2013) for analysis of most variables included block (n = 5), main plot within block, and plot as random effects, and cultivar, N and clover treatments, and their interactions as fixed effects. Data were analysed within sites and the results are presented in the individual site papers that follow this paper, along with further details of the statistical analysis methods. Some cross-site analyses were conducted to investigate general trends across regions: the results of these analyses are reported in Chapman et al. (Citation2018a), along with further detail of the statistical methods that were used.
This design meant that ryegrass cultivars were randomised within main plots, which left open the risk of preferential grazing (or, at least, differences in grazing behaviour) among ryegrass cultivars (e.g. Smit et al. Citation2006). These effects could affect regrowth and total herbage accumulation, but they could not be controlled. They were minimised to some extent by the grazing decision rules (as described below and in more detail in the site papers that follow). The collection of post-grazing sward height information in all plots at all grazings allowed the magnitude of differences in grazing among the cultivars to be quantified to aid subsequent data interpretation.
Regions, and definition of seasons for data analysis
The FVI presents cultivar ranking information for four regions of New Zealand: upper North Island, lower North Island, upper South Island and lower South Island (Chapman et al. Citation2017). Given the core purpose of the investigation reported in this series, the experiment was conducted at one location in each of those regions. The upper North Island (UNI) site was located at Newstead near Hamilton (Waikato) to represent the warm-temperate climate of this region which is also characterised by frequent, damaging outbreaks of Argentine stem weevil and black beetle. The lower North Island (LNI) site was located at Massey University near Palmerston North (Manawatu) representing the temperate climate with generally moist summers typical for the region. The upper South Island (USI) site was located at Lincoln University (Canterbury) representing the cool-temperate climate of the region where low summer rainfall is augmented by irrigation to maintain pasture growth. The lower South Island (LSI) site was at Woodlands near Invercargill (Southland) representing the summer-moist, cool-temperate climate of the region which supports good summer pasture growth in the absence of irrigation, but allows only low pasture growth rates in winter.
Similarly, to maintain consistency with the structure of the FVI, five growth periods (hereafter referred to as ‘seasons’) were used to analyse the major variables of pasture growth, botanical composition and herbage nutritive value. In the FVI, winter, early spring and late spring are each defined as two-month periods to capture the transition from winter to early spring (where there is a strong management focus on feed supply, dictated by the typical start of calving date for different parts of the country), followed by the period when pasture growth typically matches then exceeds feed demand in late spring (when the management focus shifts to maintaining pasture quality). Many important tactical management decisions such as N fertiliser and supplementary feed use, silage conservation and grazing management fall within these periods of the annual management cycle (Holmes et al., Citation2002). Because climate and, therefore, monthly pasture growth rates differ across the country, a strict calendar-based allocation of months to seasons does not capture these management dynamics. Therefore, in the FVI, and in this series of papers, the seasons in UNI and LNI were defined as: winter – May and June; early spring – July and August; late spring – September and October; summer – November, December and January; and autumn – February, March and April. In USI and LSI the seasons were defined as: winter – June and July; early spring – August and September; late spring – October and November; summer – December, January and February; and autumn – March, April and May.
Grazing management
Plots were rotationally grazed by dairy cows, with all main plots grazed at the same time. The timing of the first grazing after sowing was determined by the ‘pull’ test, which involves grasping ryegrass seedlings firmly between the thumb and forefinger and tugging in a single quick movement to mimic a cow biting (Hampton et al. Citation1999). If the seedling was uprooted from the ground, the pasture was not considered to be ready for grazing. If the leaves broke off and the roots remained in the ground, the pasture was considered ready for grazing. Subsequently, grazing was targeted to take place when the average herbage mass across the eight cultivars in a main plot reached 2500–3300 kg DM/ha. Pastures were generally grazed at the lower end of this range in winter and early spring, and in summer when pasture growth rates were lower (except for irrigated pastures at the Canterbury site in summer), and nearer the upper end of the range in late spring and autumn when growth rates were higher (except when soil conditions were dry in autumn). This rule meant that main plots receiving the low or high nitrogen treatments could be grazed at different times according to differences in growth rates; indeed, in most years, one or two more grazing events were implemented in high N main plots compared with low N plots over the annual cycle. The target post-grazing residual was 4–5 cm uncompressed sward height as measured with a rising plate meter (approximately 1500–1750 kg DM/ha using the standard winter calibration equation, where kg DM/ha = compressed sward height × 140 + 500, and sward height is in units of 0.5 cm, DairyNZ, Citation2008). At each grazing a discrete group of cows were allocated to each main plot. The number of cows in each group was determined by pasture availability above the desired residual plus expected cow intake so that the target residual could be achieved in one grazing (either between morning and afternoon milking, or afternoon and morning milking).
Nitrogen fertiliser
The amounts of N applied in the low and high N fertiliser treatments were chosen to reflect variation within and between regions in N use on farms. Between-region variation reflects differences in length of the pasture growing season, the balance between feed supply and demand, and the likelihood of economic responses in pasture growth to additional N applied. Data on N fertiliser use obtained from DairyBase for the 2010/2011 milk production season showed that mean N application rates were 130–140 kg N/ha higher in USI than the other three regions which were all reasonably close to each other in the mean and range of N rates (). Hence, the target rate of N chosen for the Low N treatment was the same across LNI, USI and LSI (50 kg N/ha per year), as was the target rate chosen for the High N rate in these regions (225 kg N/ha per year), whereas higher rates were targeted in USI ().
Table 2. Nitrogen fertiliser rates (kg N/ha per year) used on farms in the 2010/2011 production season, and rates targeted in the Low N and High N treatments of the experiment, for New Zealand dairying regions.
Invertebrate sampling
Population densities of insect and nematode pests were sampled annually from each trial during February or early March between 2013 and 2016 for the Waikato, Manawatu and Canterbury sites and between 2014 and 2017 for the Southland site. Macroinvertebrate populations were determined by taking 10 soil cores, 10 cm diameter × 15 cm deep, from within each plot of each treatment. The top 1–3 cm of each core, comprising herbage and top of the roots, was cut off and processed in a Berlese heat extraction system, in which the insects are driven out by heat and collected into glycol. The samples were stored until the contents could be identified under a microscope, with all insect pest species counted and their numbers recorded.
The remainder of the cores were searched by hand in the field, with the soil returned to the core hole. Larvae of CRW, grass grub, black beetle, white fringed weevil, wireworm (Conoderus excul) and porina (Wiseana spp.), plus other lepidopteran larvae and slugs, were counted. Root aphid (Aploneura lentisci) and pasture mealybug (Balanococcus poae) were scored for presence but counts of colonies or individuals could not be assessed in the field
Nematodes were sampled from beneath Base AR37, Bealey NEA2/6, One50 AR37 and Abermagic AR1 ryegrass which had been sown with clover and fertilised with the low rate of nitrogen to give a total of 20 plots per site. Samples, consisting of ten 2.5 cm diameter × 10 cm deep cores, were taken in April 2013–2015 and in February 2016 from within each plot. The cores from each plot were bulked then crumbled and mixed by hand. To determine soil moisture, a subsample of approximately 100 g from each plot was oven dried for 48 h at 80°C then reweighed. Another 100 g (wet weight) subsample of soil was placed in a modified Whitehead tray and nematodes were extracted using the method of Mercer et al. (Citation2008). Briefly, each sample was placed on a two-ply paper tissue, supported by two layers of nylon gauze within a shallow tray to which 500 ml of tap water was added. The tray was left for 72 h, after which the liquid was poured into a 1 L plastic beaker, left to settle for 4 hours, then reduced to 100 ml by aspiration. The 100 ml samples were transferred to 100 ml plastic beakers and allowed to settle for 4 hours before reduction to a final volume of 20 ml. The total number of nematodes (which includes plant parasitic, bacterial and fungal feeding species) and plant parasitic nematodes were counted using a Doncaster dish under a stereo-microscope at 40–80× magnification with plant parasitic nematodes identified to genera. The ITS, 18S or 28S rDNA genes were sequenced from a number of root-knot (Meloidogyne), cyst (Heterodera) or lesion (Pratylenchus) nematodes respectively at each site to determine the species present by matching sequences with those in Genbank.
Conclusions
The advent of performance indexing systems for perennial ryegrass cultivars in New Zealand and other countries raises several questions about the scalability of information on relative cultivar performance derived from small-plot evaluation trials to the level of the whole farm system. The potential for re-ranking or scaling interactions occurring in grass–clover mixtures (compared with monocultures) is one of the questions that must be addressed to ensure farmers can have confidence in the information contained in forage indices. A re-examination of grass–clover relations in pastures grazed by dairy cattle in New Zealand is therefore timely because the consequences of developments in perennial ryegrass breeding and dairy farm management practices over the past 30 years have not been systematically investigated. These developments have potentially large implications for the ability of dairy farmers to maintain profitability while also complying with looming environmental regulations, especially regulations that limit the nitrate leaching emissions permissible from dairy farm systems.
The core objective of the investigation reported in the subsequent papers of this series was to address the ryegrass cultivar re-ranking question noted above. However, it also touches on the other issues. Hence, our secondary objective is to add new insights to the stock of scientific knowledge available for managing grass–clover relationships to achieve best possible productivity and environmental outcomes in the dairy industry specifically, and all pastoral agriculture sectors generally.
Disclosure statement
No potential conflict of interest was reported by the authors.
ORCID
David F. Chapman http://orcid.org/0000-0002-6997-2380
Julia M. Lee http://orcid.org/0000-0002-2442-2086
Additional information
Funding
References
- Armstrong CS. 1977. ‘Grasslands Nui’ perennial ryegrass. New Zealand Journal of Experimental Agriculture. 5:381–384. doi: 10.1080/03015521.1977.10426000
- Ball PR. 1979. Nitrogen relationships in grazed and cut grass-clover systems [PhD thesis]. Massey University.
- Ball PR, Field TRO. 1982. Responses to nitrogen as affected by pasture characteristics, season and grazing management. In: Lynch PB editor. Nitrogen fertilisers in New Zealand agriculture. Auckland: Ray Richards; p. 45–64.
- Ball PR, Field TRO. 1985. Productivity and economics of legume-based pastures and grass swards receiving fertiliser nitrogen in New Zealand. In: Barnes RF, Ball PR, Brougham RW, Marten GC, Minson DJ, editors. Forage legumes for energy-efficient animal production. Melbourne: CSIRO; p. 47–55.
- Ball PR, Inglis JAH, Mauger JH. 1976. Tactical application of fertilizer nitrogen to offset a seasonal feed shortage on a heavily stocked sheep farm in southern Hawke’s Bay. Proceedings of the New Zealand Grassland Association. 37:166–181.
- Bell NL, Townsend RJ, Popay AJ, Mercer CF, Jackson TA. 2011. Black beetle: lessons from the past and options for the future. In: Mercer CF, editor. Pasture persistence symposium. Grassland Research and Practice Series No. 15. Hamilton: New Zealand Grassland Association; p. 119–124.
- Blank RH, Olson MH. 1988. Effect of black beetle, in association with nitrogen and summer spelling, on pasture production in sandy soils. New Zealand Journal of Agricultural Research. 31:445-453.
- Brereton AJ, Carton OT, O'Keefe WF. 1985. The effect of grass tiller density on the performance of white clover. Proceedings of the 15th International Grassland Congress:756–757.
- Brock JL. 1988. Evaluation of New Zealand bred white clover cultivars under rotational grazing and set stocking with sheep. Proceedings of the New Zealand Grassland Association. 49:203–206.
- Brock JL, Caradus JR, Hay MJM. 1988. Fifty years of white clover research in New Zealand. Proceedings of the New Zealand Grassland Association. 50:25–39.
- Brougham RW. 1959. The effects of season and weather on the growth rate of a ryegrass clover pasture. New Zealand Journal of Agricultural Research. 2:283–296. doi: 10.1080/00288233.1959.10420316
- Brougham RW, Ball PR, Williams WM. 1978. The ecology and management of white-clover based pastures. In: Wilson JR, editor. Plant relations in pastures. East Melbourne: CSIRO; p. 309–324.
- Bryant AM. 1984. Feed and management strategies at Ruakura. Proceedings of the Ruakura Farmers’ Conference. 36:20–24.
- Bryant AM. 1990. Optimum stocking and feed management practices. Proceedings of the Ruakura Farmers’ Conference. 42:55–59.
- Bryant AM, L'Huillier PJ. 1986. Better use of pastures. Proceedings of the Ruakura Farmers’ Conference. 38:43–51.
- Bryant AM, Macdonald KA, Clayton DG. 1981. Effects of nitrogen fertiliser on production of milk solids from grazed pasture. Proceedings of the New Zealand Grassland Association. 43:58–63.
- Butler GW, Barclay PC, Glenday AC. 1962. Genetic and environmental differences in the mineral composition of ryegrass herbage. Plant and Soil. 16:214–228. doi: 10.1007/BF01377217
- Camlin MS. 1981. Competitive effects between ten cultivars of perennial ryegrass and three cultivars of white clover grown in association. Grass and Forage Science. 36:169–178. doi: 10.1111/j.1365-2494.1981.tb01553.x
- Caradus JR, Chapman DF. 1996. Selection for and heritability of stolon characteristics of two cultivars of white clover. Crop Science. 36:900–904. doi: 10.2135/cropsci1996.0011183X0036000400014x
- Caradus JR, Hay RJM, Woodfield DR. 1996a. The positioning of white clover cultivars in New Zealand. In: Woodfield DR editor. White clover: New Zealand’s competitive edge. Grassland Research and Practice Series No. 6. Palmerston North: New Zealand Grassland Association; p. 45–49.
- Caradus JR, Woodfield DR, Stewart AV. 1996b. Overview and vision for white clover. In: Woodfield DR editor. White clover: New Zealand's competitive edge. Grassland Research and Practice Series No. 6. Palmerston North: New Zealand Grassland Association; p. 1–6.
- Chapman DF, Bryant JR, McMillan WH, Khaembah EA. 2012. Economic values for evaluating pasture plant traits. Proceedings of the New Zealand Grassland Association. 74:209–215.
- Chapman DF, Bryant JR, Olayemi ME, Edwards GR, Thorrold BS, McMillan WH, Kerr GA, Judson G, Cookson T, Moorhead A, Norris M. 2017. An economically based evaluation index for perennial and short-term ryegrasses in New Zealand dairy systems. Grass and Forage Science. 72:1–21. doi: 10.1111/gfs.12213
- Chapman DF, Crush JR, Lee JM, Cosgrobe GP, Stevens DR, Rossi L, Popay AJ, Edwards GR, King WM. 2018a. Implications of grass-clover interactions in dairy pastures for forage value indexing systems. 6. Cross-site analysis and general discussion. New Zealand Journal of Agricultural Research (this series).
- Chapman DF, Edwards GR, Stewart AV, McEvoy M, O’Donovan M, Waghorn GC. 2015. Valuing forages for genetic selection: what traits should we focus on? Animal Production Science. 55:869–882. doi: 10.1071/AN14838
- Chapman DF, Parsons AJ, Cosgrove GP, Barker DJ, Marotti DM, Venning KJ, Rutter SM, Hill J, Thompson AN. 2007. Impacts of spatial patterns in pasture on animal grazing behaviour, intake, and performance. Crop Science. 47:399–415. doi: 10.2135/cropsci2006.01.0036
- Chapman DF, Parsons AJ, Schwinning S. 1996. Management of clover in grazed pastures: expectations, limitations and opportunities. In: Woodfield DR editor. White clover: New Zealand's Competitive Edge; Grassland Research and Practice Series No. 6. Palmerston North: New Zealand Grassland Association; p. 55–64.
- Chapman DF, Rossi L, Lee JM, Edwards GR. 2018b. Implications of grass-clover interactions in dairy pastures for forage value indexing systems. 4. Canterbury. New Zealand Journal of Agricultural Research (this series).
- Chestnutt DMB, Lowe J. 1970. White clover/grass relationships: agronomy of white clover/grass swards: a review. Occasional Symposium 6 of the British Grassland Society:191–213.
- Clark DA, Harris SL. 1996. White clover or nitrogen fertiliser for dairying? In: Woodfield DR editor. White Clover: New Zealand’s Competitive Edge. Grassland Research and Practice Series No. 6. Palmerston North: New Zealand Grassland Association; p. 107–114.
- Collins RP, Rhodes I. 1989. Yield of white clover populations in mixture with contrasting perennial ryegrasses. Grass and Forage Science. 44:111–115. doi: 10.1111/j.1365-2494.1989.tb01918.x
- Connolly V. 1968. A comparison of six white clover and three perennial ryegrass varieties under a system of grazing management. Irish Journal of Agricultural Research. 7:227–242.
- Cosgrove GP, Popay AJ, Taylor PS, Wilson DJ, Aalders LT, Bell NL. 2018. Implications of grass-clover interactions in dairy pastures for forage value indexing systems. 3. Manawatu. New Zealand Journal of Agricultural Research (this series).
- Cougnon M, Baert J, Waes C, Reheul D. 2012. Effect of grass species and ploidy on clover content in grass-clover mixtures. In: Goliński P, Warda M, Stypiński P, editors. Grassland - a European resource? Proceedings of 24th General Meeting of the European Grassland Federation, Lubin, Poland. Poznan, Poland: Polskie Towarzystwo akarskie (Polish Grassland Society); p. 100–102.
- Cowling DW, Lockyer DR. 1965. A comparison of the reaction of different grass species to fertilizer nitrogen and to growth in association with white clover. 1. Yield of dry matter. Grass and Forage Science. 20:197–204. doi: 10.1111/j.1365-2494.1965.tb00423.x
- Crush JR, Lee JM, Cosgrove GP, Rossi L, Chapman DF, Stevens DR. 2018a. Foliar macronutrient concentrations of eight perennial ryegrass (Lolium perenne L.) cultivars grown in four regions of New Zealand. New Zealand Journal of Agricultural Research (this series).
- Crush JR, Lee JM, Cosgrove GP, Rossi L, Chapman DF, Stevens DR. 2018b. Foliar micronutrient concentrations of eight perennial ryegrass (Lolium perenne L.) cultivars grown in four regions of New Zealand. New Zealand Journal of Agricultural Research (this series).
- Crush JR, Woodward SL, Eerens JPJ, Macdonald KA. 2006. Growth and milksolids production in pastures of older and more recent ryegrass and white clover cultivars under dairy grazing. New Zealand Journal of Agricultural Research. 49:119–135. doi: 10.1080/00288233.2006.9513702
- DairyNZ. 2008. DairyNZ farmfact 1–15 using the rising plate meter. Hamilton: DairyNZ.
- Davies A. 2001. Competition between grasses and legumes in established pastures. In Tow PG, Lazenby A editors. Competition and succession in pastures. Wallingford, CAB International; p. 63–83.
- Davies DA, Fothergill M. 1990. Productivity and persistence of white clover grown with three perennial ryegrass varieties and continuously stocked with sheep. In: Soil-grassland-animal relationships. Proceedings of 13th General Meeting of the European Grassland Federation, Banska Bystrica, Czechoslovakia: Grassland Research Institute. Volume 2; p. 157–162.
- Davies DA, Fothergill M, Morgan CT. 1993. Assessment of contrasting perennial ryegrasses, with and without white clover, under continuous sheep stocking in the uplands. 5. Herbage production, quality and intake in years 4–6. Grass and Forage Science. 48:213–222. doi: 10.1111/j.1365-2494.1993.tb01854.x
- Dunlop J, Hart AL 1987. Mineral nutrition. In: Baker MJ, Williams WM editors. White clover. Wallingford: CAB International; p. 153–183.
- Easton HS, Baird DB, Cameron NE, Kerr GA, Norriss M, Stewart AV. 2001. Perennial ryegrass cultivars: herbage yield in multi-site plot trials. Proceedings of the New Zealand Grassland Association. 63:183–188.
- Eerens JPJ, Lucas RJ, Easton HS, White JGH. 1998. Influence of the ryegrass endophyte (Neotyphodium lolii) in a cool-moist environment III. Interaction with white clover. New Zealand Journal of Agricultural Research. 41:201–207. doi: 10.1080/00288233.1998.9513303
- Elgersma A, Schlepers H. 1997. Performance of white clover/perennial ryegrass mixtures under cutting. Grass and Forage Science. 52:134–146. doi: 10.1111/j.1365-2494.1997.tb02344.x
- Ennik GC. 1982. The contribution of white clover to the herbage yield of mixed swards. In: The efficiency of N-utilization by grass and legume and grass/clover swards. Proceedings of an EEC workshop, Braunschweig, 8–9 June 1982. p. 23–27.
- Enriquez-Hidalgo D, Gilliland T, Hennessy D. 2015. Herbage and nitrogen yields, fixation and transfer by white clover to companion grasses in grazed swards under different rates of nitrogen fertilization. Grass and Forage Science. 71:559–574. doi: 10.1111/gfs.12201
- Field TRO, Ball PR. 1978. Tactical use of nitrogen fertiliser. Proceedings of the Agronomy Society of New Zealand. 8:129–133.
- Fletcher LR, Harvey IC. 1981. An association of a Lolium endophyte with ryegrass staggers. New Zealand Veterinary Journal. 29:185–186.
- Gallagher RT, White EP, Mortimer PH. 1981. Ryegrass staggers: isolation of potent neurotoxins lolitrem A and lolitrem B from staggers producing pastures. New Zealand Veterinary Journal. 29:189–190. doi: 10.1080/00480169.1981.34843
- Gerard PJ, Hackell DL, Bell NL. 2007. Impact of clover root weevil Sitona lepidus (Coleoptera: Curculionidae) larvae on herbage yield and species composition in a ryegrass-white clover sward. New Zealand Journal of Agricultural Research. 50:381–392. doi: 10.1080/00288230709510306
- Gilliland TJ. 1996. Assessment of perennial ryegrass variety compatibility with white clover under grazing. Plant Varieties and Seeds. 9:65–75.
- Griffiths WM, Matthew C, Lee JM, Chapman DF. 2017. Is there a tiller morphology ideotype for yield differences in perennial ryegrass (Lolium perenne L.)? Grass and Forage Science. Early view online. doi:10.1111/gfs.12268.
- Grogan D, Gilliland TJ. 2011. A review of ryegrass variety evaluation in Ireland. Irish Journal of Agricultural and Food Research. 50:65–81.
- Hampton JG, Kemp PD, White JGH. 1999. Pasture establishment. In: White J, Hodgson J. editors. New Zealand pasture and crop science. Auckland: Oxford University Press; p. 101–115.
- Harmer M, Stewart A, Woodfield D. 2016. Genetic gain in perennial ryegrass forage yield in Australia and New Zealand. Journal of New Zealand Grasslands. 78:133–138.
- Harris AJ, Brown KR, Turner JD, Johnson JM, Ryan DL, Hickey MJ. 1973. Some factors affecting pasture growth in Southland. New Zealand Journal of Experimental Agriculture. 1:139–163. doi: 10.1080/03015521.1973.10427634
- Harris SL, Thom ER, Clark DA. 1996. Effect of high rates of nitrogen fertiliser on perennial ryegrass growth and morphology in grazed dairy pasture in northern New Zealand. New Zealand Journal of Agricultural Research. 39:159–169. doi: 10.1080/00288233.1996.9513174
- Harris W. 2001. Formulation of pasture seed mixtures with reference to competition and succession in pastures. In: Tow PG, Lazenby A editors. Competition and succession in pastures. Wallingford: CAB International; p. 149–174.
- Harris W, Hoglund JH. 1977. Influence of seasonal growth periodicity and N-fixation on competitive combining abilities of grasses and legumes. In: Proceedings of the 13th International Grassland Congress. Sectional Papers, Sections 1–2, 138–149.
- Harris W, Thomas VJ. 1973. Competition among pasture plants III. Effects of frequency and height of cutting on competition between white clover and two ryegrass cultivars. New Zealand Journal of Agricultural Research. 16:49–58. doi: 10.1080/00288233.1973.10421159
- Haynes RJ. 1980. Competitive aspects of the grass-legume association. Advances in Agronomy. 33:227–261. doi: 10.1016/S0065-2113(08)60168-6
- Hoglund JH, Crush JR, Brock JL, Ball R, Carran RA. 1979. Nitrogen fixation in pasture. XII. General discussion. New Zealand Journal of Experimental Agriculture. 7:45–51. doi: 10.1080/03015521.1979.10426162
- Holmes CW, Brookes IM, Garrick DJ, MacKenzie DDS, Parkinson TJ, Wilson GF. 2002. Milk production from pasture. Principles and Practice. Massey University, Palmerston North. ISBN: 0-473-08308-6.
- Hunt WF, Dymock JJ, Gaynor DL. 1988. Spring and autumn nitrogen effects on susceptibility of low-endophyte ‘Grasslands Nui’ ryegrass to damage by Argentine stem weevil larvae. New Zealand Journal of Agricultural Research. 31:389–393. doi: 10.1080/00288233.1988.10423432
- Kain WM, Atkinson DS. 1977. Development of resistant pasture and methods of pasture management for grass grub Costelytra zealandica control. New Zealand Journal of Agricultural Research. 20:507–518. doi: 10.1080/00288233.1977.10427367
- Kerr GA, Chapman DF, Thom ER, Matthew C, Van der Linden A, Baird DB, Johnston E, Corkran JR. 2012. Evaluating perennial ryegrass cultivars: improving testing. Proceedings of the New Zealand Grassland Association. 74:127–135.
- Ledgard SF. 1991. Transfer of fixed nitrogen from white clover to associated grasses in swards grazed by dairy cows, estimated using 15N methods. Plant and Soil. 131:215–223. doi: 10.1007/BF00009451
- Ledgard SF, Sprosen MS, Penno JW, Rajendram GS. 2001. Nitrogen fixation by white clover in pastures grazed by dairy cows: temporal variation and effects of nitrogen fertilization. Plant and Soil. 229:177–187. doi: 10.1023/A:1004833804002
- Ledgard SF, Steele KW. 1992. Biological nitrogen fixation in mixed legume/grass pastures. Plant and Soil. 141:137–153. doi: 10.1007/BF00011314
- Lee JM, Chapman DF, Wims CM, Griffiths WM. 2018. Implications of grass-clover interactions in dairy pastures for forage value indexing systems. 2. Waikato. New Zealand Journal of Agricultural Research (this series).
- Lee JM, Matthew C, Thom ER, Chapman DF. 2012. Perennial ryegrass breeding in New Zealand: a dairy industry perspective. Crop and Pasture Science. 63:107–127. doi: 10.1071/CP11282
- Leuchtmann A, Bacon CW, Schardl CL, White JF, Tadych M. 2014. Nomenclatural realignment of Neotyphodium species with genus Epichloë. Mycologia. 106:202–215. doi: 10.3852/13-251
- Macdonald KA, Penno JW. 1998. Management decision rules to optimise milksolids production on dairy farms. Proceedings of the New Zealand Society of Animal Production. 58:132–135.
- McDonagh J, O’Donovan M, McEvoy M, Gilliland TJ. 2016. Genetic gain in perennial ryegrass (Lolium perenne) varieties 1973 to 2013. Euphytica. 212:187–199. doi: 10.1007/s10681-016-1754-7
- McEvoy M, O’Donovan M, Shalloo L. 2011. Development and application of an economic ranking index for perennial ryegrass cultivars. Journal of Dairy Science. 94:1627–1639. doi: 10.3168/jds.2010-3322
- Mercer CF, Bell NL, Yeates GW. 2008. Plant-parasitic nematodes on pasture in New Zealand. Australasian Plant Pathology. 37:279–288. doi: 10.1071/AP08025
- Mitchell KJ. 1956. Growth of pasture species under controlled environment. 1. Growth at various levels of constant temperature. New Zealand Journal of Science and Technology. A38:203–216.
- MPI. 2012. Pastoral input trends in New Zealand: a snapshot. Wellington: Ministry for Primary Industries. ISBN: 978-0-478-33864-0.
- Munro JMM, Davies DA, Evans WB, Scurlock RV 1992. Animal production evaluation of herbage varieties. 1. Comparison of Aurora with Frances, Talbot and Melle perennial ryegrasses when grown alone and with clover. Grass and Forage Science. 47:259–273. doi: 10.1111/j.1365-2494.1992.tb02270.x
- Nassiri M, Elgersma A. 1998. Competition in perennial ryegrass-white clover mixtures under cutting. 2. Leaf characteristics, light interception and dry-matter production during regrowth. Grass and Forage Science. 53:367–379. doi: 10.1046/j.1365-2494.1998.00152.x
- Neuteboom JH, Lantinga EA, Wind K. 1988. Tillering characteristics of diploid and tetraploid perennial ryegrass. In: Proceedings of the 12th General Meeting of the European Grassland Federation; 1988 July 4–7; Dublin. p. 498–503.
- Nicol AM, Edwards GR. 2011. Why is clover better than ryegrass? Proceedings of the New Zealand Society of Animal Production. 71:71–78.
- O’Connor MB. 1982. Nitrogen fertilisers for production of out-of-season grass. In: Lynch PB editor. Nitrogen fertilisers in New Zealand agriculture. Auckland: Ray Richards; p. 65–74.
- O’Donovan M, McHugh N, McEvoy M, Grogan D, Shalloo, L. 2017. Combining seasonal yield, silage dry matter yield, quality and persistency in an economic index to assist perennial ryegrass variety selection. Journal of Agricultural Science. 155:556–568.
- Parsons AJ, Edwards GR, Chapman DF, Carran RA. 2006. How far have we come: 75 years ‘in clover’? Proceedings of the New Zealand Grassland Association. 68:7–13.
- Parsons AJ, Harvey A, Woledge J. 1991. Plant-animal interactions in a continuously grazed mixture. I. Differences in the physiology of leaf expansion and the fate of leaves of grass and clover. Journal of Applied Ecology. 28:619–634. doi: 10.2307/2404572
- Prestidge RA, Zijpp Svd, Badan D. 1985. Effects of plant species and fertilisers on grass grub larvae, Costelytra zealandica. New Zealand Journal of Agricultural Research. 28:409–417. doi: 10.1080/00288233.1985.10430446
- Rhodes I. 1971. The relationship between productivity and some components of canopy structure in ryegrass (Lolium spp.). II. Yield, canopy structure and light interception. Journal of Agricultural Science. Cambridge 77:283–292. doi: 10.1017/S0021859600024436
- Rhodes I. 1981. The physiological basis of variation in the yield of grass/clover mixtures. In: Wright CE editor. Plant physiology and herbage production. British Grassland Society Occasional Symposium. 13:149–161.
- Rossi L. 2016. Interactions between pasture species and management and their implications for evaluating perennial ryegrass (Lolium perenne L.) cultivars in dairy systems. PhD Thesis, Lincoln University, New Zealand.
- Rutter SM. 2006. Diet preference for grass and legumes in free-ranging domestic sheep and cattle: current theory and future application. Applied Animal Behaviour Science. 97:17–35. doi: 10.1016/j.applanim.2005.11.016
- Sampoux JP, Baudouin P, Bayle B, Béguier V, Bourdon P, Chosson JF, Deneufbourg F, Galbrun C, Ghesquière M, Noël D, et al. 2011. Breeding perennial grasses for forage usage: an experimental assessment of trait changes in diploid perennial ryegrass (Lolium perenne L.) cultivars released in the last four decades. Field Crops Research. 123:117–129. doi: 10.1016/j.fcr.2011.05.007
- Schwinning S, Parsons AJ. 1996a. Analysis of the coexistence mechanisms for grasses and legumes in grazing systems. Journal of Ecology. 84:799–813. doi: 10.2307/2960553
- Schwinning S, Parsons AJ. 1996b. A spatially explicit population model of stoloniferous N-fixing legumes in mixed pasture with grass. Journal of Ecology. 84:815–826. doi: 10.2307/2960554
- Sears PD. 1962. Exploitation of high production pastures in New Zealand. Proceedings of the New Zealand Ecological Society. 9:57–63.
- Smit HJ, Tamminga S, Elgersma A. 2006. Dairy cattle grazing preference among six cultivars of perennial ryegrass. Agronomy Journal. 98:1213–1220. doi: 10.2134/agronj2005.0264
- Stevens DR, Bryson BJ, Ferguson CM, Wilson DJ, Bell NL, Aalders LT, Popay AJ. 2018. Implications of grass-clover interactions in dairy pastures for forage value indexing systems. 5. Southland. New Zealand Journal of Agricultural Research (this series).
- Stewart AV. 2006. Genetic origins of New Zealand perennial ryegrass (Lolium perenne) cultivars. In: Mercer CF editor. Advances in pasture breeding. Grassland Research and Practice Series No. 12. Palmerston North: New Zealand Grassland Association; p. 55–61.
- Swift G, Vipond JE, McClelland TH, Cleland AT, Milne JA, Hunter EA 1993. A comparison of diploid and tetraploid perennial ryegrass and tetraploid ryegrass white clover swards under continuous sheep stocking at controlled sward heights. 1. Sward characteristics. Grass and Forage Science. 48:279–289. doi: 10.1111/j.1365-2494.1993.tb01861.x
- Thom ER, Popay AJ, Hume DE, Fletcher LR. 2012. Evaluating the performance of endophytes in farm systems to improve farmer outcomes – a review. Crop and Pasture Science. 63:927–943. doi: 10.1071/CP12152
- Thom ER, Popay AJ, Waugh CD, Minneé EMK. 2014. Impact of novel endophytes in perennial ryegrass on herbage production and insect pests from pastures under dairy cow grazing in northern New Zealand. Grass and Forage Science. 69:191–204. doi: 10.1111/gfs.12040
- Thom ER, Waugh CD, Minnee EMK, Waghorn GC. 2013. Effects of novel and wild-type endophytes in perennial ryegrass on cow health and production. New Zealand Veterinary Journal. 61:87–97. doi: 10.1080/00480169.2012.715379
- Tozer KN, Chapman DF, Bell NL, Crush JR, King WM, Rennie GM, Wilson DJ, Mapp NR, Rossi L, Aalders LT, et al. 2014. Botanical survey of perennial ryegrass-based dairy pastures in three regions of New Zealand: implications for ryegrass persistence. New Zealand Journal of Agricultural Research. 57:14–29. doi: 10.1080/00288233.2013.863785
- Van Heeswijck R, McDonald G. 1992. Acremonium endophytes in perennial ryegrass and other pasture grasses in Australia and New Zealand. Australian Journal of Agricultural Research. 43:1683–1709. doi: 10.1071/AR9921683
- VSN International. 2013. Genstat for windows. 16th ed. Hemel Hempstead: VSN International. https://www.vsni.co.uk/downloads/genstat/16th-edition-upgrade/.
- Wales WJ, Kolver ES. 2017. Challenges of feeding dairy cows in Australia and New Zealand. Animal Production Science. 57:1366–1383. doi: 10.1071/AN16828
- White, JGH. 1999. The farmlands of New Zealand. In: White J, Hodgson J, editors. New Zealand pasture and crop science. Auckland: Oxford University Press; p. 1–10.
- Widdup KH, Barrett BA. 2011. Achieving persistence and productivity in white clover. In: Pasture Persistence Symposium. Grassland Research and Practice Series No. 15. p. 173–180.
- Widdup KH, Turner JD. 1983. Performance of 4 white clover populations in monoculture and with ryegrass under grazing. New Zealand Journal of Experimental Agriculture. 11:27–31. doi: 10.1080/03015521.1983.10427722
- Wilkins PW, Humphreys MO. 2003. Progress in breeding perennial forage grasses for temperate agriculture. Journal of Agricultural Science. 140:129–150. doi: 10.1017/S0021859603003058
- Williams TA, Abberton MT, Evans DR, Thornley W, Rhodes I. 2000. Contribution of white clover varieties in high-productivity systems under grazing and cutting. Journal of Agronomy and Crop Science. 185:121–128. doi: 10.1046/j.1439-037x.2000.00424.x
- Woledge J, Davidson K, Dennis WD. 1992. Growth and photosynthesis of tall and short cultivars of white clover with tall and short grasses. Grass and Forage Science. 47:230–238. doi: 10.1111/j.1365-2494.1992.tb02267.x
- Woodfield DR, editor. 1996. White clover: New Zealand’s competitive edge. Grassland Research and Practice Series No. 6. Palmerston North: New Zealand Grassland Association.
- Woodfield DR. 1999. Genetic improvements in New Zealand forage cultivars. Proceedings of the New Zealand Grassland Association. 61:3–8.
- Woodfield DR, Caradus JR. 1990. Estimates of heritability for, and relationships between, root and shoot characteristics of white clover. II. Regression of progeny on mid-parents. Euphytica. 46:211–215. doi: 10.1007/BF00027220
- Woodfield DR, Caradus JR. 1994. Genetic improvement in white clover representing six decades of plant breeding. Crop Science. 34:1205–1213. doi: 10.2135/cropsci1994.0011183X003400050011x
- Woodfield DR, Clifford PTP, Cousins GR, Ford JL, Baird IJ, Miller JE, Woodward SL, Caradus JR. 2001. Grasslands Kopu II and Crusader: new generation white clovers. Proceedings of the New Zealand Grassland Association. 63:103–108.