ABSTRACT
This paper describes how the core treatments in the national experimental design (all combinations of with/without clover and high/low nitrogen (N)) were implemented at an irrigated Canterbury site and monitored for three years. There was a consistent N × clover interaction, caused mainly by substantially lower yields in the Low N without clover treatment compared with all other treatments. At the phenotypic contrast level, the main effect of perennial ryegrass heading date on white clover content was significant in four of the nine seasonal data sets available: on these occasions, mixtures based on mid-season heading cultivars had higher clover content than later season-heading cultivars. However, this difference was not sufficient to cause any clover × cultivar interactions in herbage accumulation. Hence, the hypothesis that relative yield rankings of ryegrass cultivars do not differ when ryegrass is grown in monoculture or in mixture with white clover is supported.
Introduction
The rationale behind the experiment reported here is explained in Chapman et al. (Citation2018). The hypothesis is that the yield rankings of perennial ryegrass cultivars do not differ when grown in monoculture (as occurs in routine cultivar evaluation trials, e.g. Easton et al. Citation2001; Kerr et al. Citation2012) compared with in mixture with white clover (as per common practice on New Zealand dairy farms). The context for the experiment is the development of the DairyNZ Forage Value Index (FVI; Chapman et al. Citation2017), in particular the need to bridge from the small-plot, highly controlled scale of evaluation trials and the farm systems scale where cultivars are used in practice and for which the FVI has been developed as a decision support tool. There are several ways in which cultivar performance measured in small plots may not accurately represent what happens in farm systems. One of these is the possibility of re-ranking or scaling interactions caused by the presence of clover. This is the focus of this series of papers.
Chapman et al. (Citation2018) noted that the hypothesis must be tested in several dairy environments, since the environment (particularly climate) has a dominating effect on grass and clover growth, and on their interactions in grazed pastures (Brougham et al. Citation1978). To a certain extent climate also governs the major pasture pest species present at the regional level which can influence ryegrass-clover interactions within the pasture. A logical structure for dealing with the possibility of environmental effects is to mirror the four regions used in the FVI (Chapman et al. Citation2017). The experiment reported here was conducted in the upper South Island. Dairying in the eastern part of the region (where 1180 of the total of the approximately 1800 dairy farms in the upper South Island are located, DairyNZ Citation2016) is almost exclusively carried out with irrigation to supplement low summer rainfall and compensate for high evapotranspiration rates, and thus ensure a 9–10-month pasture growing season to match the lactation cycle.
The availability of irrigation sets this site apart from the others reported in this series. The other experiments were all conducted in rainfed regions and were, therefore, not insulated to the same degree from the effects of climate variability, especially dry conditions in summer when clover can exploit an advantage over ryegrass because of its higher optimum temperature for growth (Mitchell Citation1956). Irrigation can mitigate the damaging effects of invertebrate pests such as grass grub through compensatory growth (Tozer et al. Citation2017) but can also enhance survival of some pest species (East and Willoughby Citation1980; Hunt and Gaynor Citation1982; Eerens and Hardwick Citation2003). As was the case for all sites, in addition to testing the hypothesis, this experiment also generated data that helps define the factors driving grass-clover interactions and the implications of these interactions for pasture productivity. Compared with regions such as Waikato and Manawatu, there is relatively little published information on species, management, and their interactions under dairy grazing in irrigated Canterbury pastures because the South Island dairy industry has developed only relatively recently and does not have the same history of research that other regions have drawn on for their development. This paper, therefore, discusses the implications of the results for the overall productivity of dairy pastures, as well as for the hypothesis.
Materials and methods
Site details and establishment
The experimental site was located at the Lincoln University Research Dairy Farm in Canterbury (−43.638, 172.462; 12 m a.s.l.) on a Wakanui silt loam/Wakanui silt loam over a mottled sandy loam phase (Mottled Immature Pallic; Hewitt Citation1998). The 30-year average annual rainfall measured at the Broadfield climatological station <2 km from the site is 600 mm. This was supplemented with irrigation from spring to autumn each year as is standard for dairy farms in this region. Following sowing in April 2012, 18 mm of water was applied using a travelling irrigator to assist seedling establishment. Following this, annual irrigation water applied was 287, 194 and 400 mm/year for the 2012/2013, 2013/2014 and 2014/2015 years. The total monthly rainfall, irrigation water applied and average temperatures for the duration of the experiment are provided in .
Figure 1. Average monthly maximum temperatures (A) and minimum temperatures (B), and total rainfall (▪) plus irrigation water applied (▪) (c) from March 2012 to May 2015. The grey bars represent data during the experiment while the black lines represent the 30-year means.
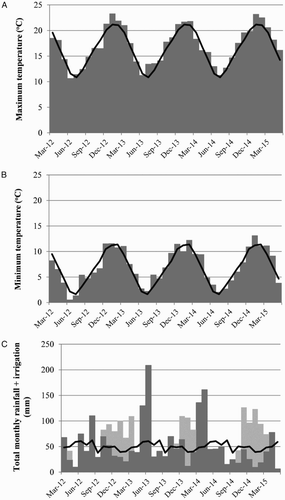
The number of germinable buried seeds was estimated from soil samples collected prior to establishment of the experiment using the method described by Tozer et al. (Citation2014). This revealed substantially greater numbers of weed grass and other weed seeds compared with clover seeds (1141, 989 and 418 seeds/m2 for weed grass, weed and clover seeds, respectively). No ryegrass seeds were detected. The most abundant weed grasses were Poa spp., while the most abundant weeds were shepherd's purse (Capsella bursa-pastoris), chickweed (Stellaria media), fleabane (Conyza bonariensis) and fathen (Chenopodium album).
In late February 2012, existing pasture was eradicated with glyphosate herbicide (1.1 kg a.i./ha) plus Accelerate™ organo-silicone penetrant at 100 mL/100 L water. The site was then ploughed, power-harrowed and rolled, and dutch-harrowed and rolled. In late March 2012, perennial ryegrass seed was sown using a cone seeder (row spacing 15 cm). Seeding rates were 20 and 28 kg/ha for the diploid and tetraploid cultivars used, respectively. All ryegrass seed was treated with Poncho® which contained systemic insecticides to deter insect attack on seedlings before endophyte alkaloid production commenced. A 50:50 mix of Superstrike®-coated Kopu II and Tribute white clover seed was also sown by hand at 7 kg/ha (equivalent to 4 kg/ha bare seed) in the ‘with clover’ treatment plots. Superstrike® contains Rhizobia, molybdenum, lime, a nematicide and Poncho®. The plots were then rolled again with a Cambridge roller. Slug bait (0.4 kg metaldehyde/ha) was applied after sowing. Snowfall in early June 2012 caused frost-damage to the sown white clover; thus the clover mixture was re-sown by hand in the ‘with clover’ plots in early September to ensure a strong clover presence.
Treatments
The ryegrass cultivars and phenotypes, and target N fertiliser rates, used in the experiment are described in Tables 1 and 2 in Chapman et al. (Citation2018). Nitrogen fertiliser was applied as urea (46:0:0:0) in multiple applications of 25 or 33 kg N/ha for the Low and High N treatments, respectively. The actual annual totals differed slightly from the targets with the High N and Low N treatments receiving 314 and 101 kg N/ha/year on average over the three years, respectively.
Endophyte infection frequency in perennial ryegrass was measured in April every year in the Low N with clover treatment. Fifty perennial ryegrass tillers per sub-plot were randomly sampled from three of the five replicates. Tillers were cut at ground level with a scalpel. The sap from the cut base of the tiller was squeezed onto nitrocellulose blotting paper before colour development that confirmed the presence/absence of endophyte (Hahn et al. Citation2003). Throughout the three years, endophyte infection frequency of all cultivars remained high (>66%).
Grazing management
Plots were rotationally grazed by dairy cows as described by Chapman et al. (Citation2018). The first grazing took place 157 days after sowing (31 August 2012), and there were between 25 and 26 grazings over the three years for the Low N treatments and 29 grazings for the High N treatments.
During the peak of seedhead production (late spring/early summer) when clumps of herbage were left ungrazed around dung pats and urine-affected areas, plots were mown to a stubble height of ∼7 cm after grazing. This occurred four times over the three years (November/December 2013, August 2014, November 2014 and December 2014).
Fertiliser application
Before sowing and on an annual basis, soil cores (25 mm diameter) were taken across each replicate to 75-mm depth and analysed for pH, phosphorus (P), potassium (K), calcium (Ca), magnesium (Mg), sodium (Na) and sulphate sulphur (S). To maintain nutrient levels in the optimum range for pasture growth, 66, 41, 80 and 146 kg/ha of P, K, S and Ca were applied during 2012/2013 and 109, 125, 176 and 242 kg/ha of P, K, S and Ca was applied during 2013/2014.
Herbicide application
All plots were sprayed with Preside® herbicide (40 g flumetsulam/ha) plus Uptake™ surfactant (1 L/ha) in water (230 L/ha) in September 2012. To eradicate clover, the ‘without clover’ treatment plots were sprayed with Banvel® 200 (400 g dicamba/ha) or T-Max™ (60 g/ha aminopyralid) plus Uptake™ surfactant (1 L/ha) when clover content was visually assessed at 5%. This occurred three times during the experiment (March 2013, December 2013 and October 2014). To achieve similar broadleaf weed control, the ‘with clover’ treatment plots were sprayed at the same time with Preside® herbicide (50–65 g/ha flumetsulam) plus Uptake™ surfactant (1 L/ha).
Adjusted herbage accumulation
Herbage mass was estimated before each grazing by cutting one 6 × 1.5 m strip from each sub-plot using a Haldrup F-55 forage harvester (Haldrup GmbH, Ilshofen, Germany) set to a height of 5 cm above the ground (minimum cut height available). To avoid harvesting the same area in consecutive grazings, the position of the strip was rotated within each sub-plot. The fresh weight of the cut herbage was taken using the on-board weighing system, and a representative sample (∼1000 g fresh weight) was taken. In the laboratory, the sample was blended, and a sub-sample (∼150 g fresh weight) was oven-dried at 95°C to constant weight (∼48 hours) to allow calculation of herbage biomass harvested per hectare.
Within a day of each grazing, sward height (in units of 0.5 cm) was measured using a rising plate meter (Jenquip, Feilding, New Zealand). Forty readings were taken in a ‘W’ pattern across each sub-plot and post-grazing herbage mass calculated using the equation:(1) where sward height is in units of 0.5 cm.
Harvested herbage mass was then adjusted for each plot to account for variation in the post-grazing herbage mass within and between treatments using the equation:(2) where pre-graze masst = 1 is measured before grazing using the Haldrup harvester as described above, 1900 is the mass (kg DM/ha, to ground level) remaining after the Haldrup harvest cut as derived from Equation (1) (where sward height is fixed by the harvester), and post-graze masst−1 is the mass remaining after the previous grazing as derived from Equation (1) (where sward height was variable). Thus, systematic and random variation in post-grazing herbage mass was accounted for by either adding to (in the case of post-grazing mass at the previous grazing ≤ 1900 kg DM/ha) or subtracting from (in the case of the previous post-grazing mass ≥ 1900 kg DM/ha) the pre-graze mass measured by cutting. Technically, this describes the amount of herbage grown since the previous grazing that was harvested by animals at each grazing event (and cumulatively, when summed for seasons and years). It is synonymous with ‘herbage accumulation’ as used throughout this paper.
Seasonal adjusted herbage accumulation for each sub-plot was then calculated from the adjusted herbage accumulation rate data for individual harvests partitioned within the following periods as defined by the FVI (Chapman et al. Citation2017). For the Canterbury site these periods were: winter (1 June to 31 July), early spring (1 August to 30 September), late spring (1 October to 30 November), summer (1 December to 28/29 February) and autumn (1 March to 31 May). Total annual adjusted herbage accumulation was also calculated for the period 1 June–31 May.
Botanical composition and nutritive value
Representative samples of herbage from each sub-plot were processed for botanical composition and nutritive value characteristics in late spring, summer and late autumn (October/November, January and April/May, respectively). From the blended sample collected from the Haldrup harvester, one sub-sample (∼15 g fresh weight) was dissected into the following categories: perennial ryegrass, white clover, unsown species and dead material. Dissected sub-samples were oven-dried at 95°C to constant weight (approximately 48 hours) and weighed to determine botanical composition as a proportion of above-residual herbage biomass on a DM basis. A second sub-sample (∼150 g fresh weight) was oven-dried at 60°C to constant weight (approximately 48 hours) and ground to pass through a 1-mm sieve (ZM200 rotor mill, Retsch GmbH, Hann, Germany). Dried samples were analysed for crude protein (CP) and neutral detergent fibre (NDF) concentration, organic matter digestibility (OMD), and metabolisable energy (ME) density using near infrared spectroscopy (Corson et al. Citation1999) by feedTECH, AgResearch Grasslands, Palmerston North. The ME density was derived directly from predicted OMD on the basis of an in vitro cellulase digestibility assay which was calibrated against in vivo standards (Corson et al. Citation1999).
Population density
The density of perennial ryegrass tillers and white clover growing points was calculated from the total number of ryegrass tillers and white clover growing points counted in five randomly placed frames (5 × 20 cm) per sub-plot in autumn each year.
Invertebrate populations
Samples were collected between mid-February and late March each year from 2013 to 2016 to assess populations of pest insects and nematodes. Sample collection and processing procedures are described in Chapman et al. (Citation2018).
Statistical analysis
Herbage-related data were analysed using REML in GenStat (VSN International Ltd. Citation2013), including replicate, main plot within replicate, and plot as random effects, and cultivar, N and clover treatments, and their interactions as fixed effects. Multiple comparisons between cultivars were made using the Tukey's least significant difference test. For botanical composition and white clover growing point data, both untransformed and angular transformed (botanical composition) or square root transformed (growing point) data were analysed; slightly different conclusions were reached for some analyses. Therefore, significance values and letters to indicate significant differences presented in the Tables and Figures are from the analysis of transformed data, while percentages and standard error of the difference between means (s.e.d.) are from the analysis of untransformed data for ease of interpretation.
Phenotypic contrasts (i.e. morphology and heading date) of the cultivars were also included in the analysis to aid interpretation of cultivar effects. Significant phenotypic contrasts were considered to be valid (i.e. both cultivars were considered to be behaving consistently for the variable of interest) if both cultivars in one group were ranked higher/lower than the contrasting group. Significant phenotypic contrasts where cultivars from both groups were interspersed throughout the ranking have not been presented here as cultivars within the group are not considered to be consistent in the variable of interest. Data have not been presented in the Results section for statistically significant, valid phenotypic contrasts. However, means for each contrast (see Table 1 in Chapman et al. Citation2018) can be calculated from the relevant cultivar means presented in Tables 2, 4, 5 and 7.
Densities of individual insect species that were present in at least 50% of plots and the total pest densities were analysed by ANOVA with log transformation used if necessary to stabilise the variance. Total pest densities represented the sum of all insect pests that were enumerated, which therefore excluded root aphid (Aploneura lentisci) for which only an incidence was determined. A repeated measures ANOVA was also carried out to investigate changes in populations in response to clover and N treatments over the four years of sampling. Results for plant feeding nematode species on the four cultivars with clover that were sampled are presented as numbers per 100 g of soil to ensure valid comparison between different years when soil moisture levels differed. Data were analysed by ANOVA with log transformation as required to normalise the data.
Results
Climate
Annual rainfall plus irrigation water was 957, 1077 and 776 mm/year for the 2012/2013, 2013/2014 and 2014/2015 years, respectively. Total Penman potential evapotranspiration exceeded rainfall plus irrigation water for six to eight months during early spring to autumn each year. The resulting water deficits were less severe than those experienced at the Waikato site (−278, −246 and −283 mm, respectively; compared with Lee et al. Citation2018).
Adjusted herbage accumulation: cultivar level
Adjusted herbage accumulation data for a total of 17 combinations of years × seasons were available for analysis. Of these 17 analyses, there was no evidence of significant three-way interactions between cultivar, N treatment and clover treatment.
Effects of N and clover, and their interaction
During the three years, the main effects of N and of clover on seasonal and total annual adjusted herbage accumulation were each significant on 15 out of 17 occasions (). The site was clearly responsive to additional N fertiliser, and sowing pastures with clover also increased adjusted herbage accumulation once the clover had well established, resulting in greater total annual adjusted herbage accumulation during all three years.
Table 1. Seasonal and total annual adjusted herbage accumulation (kg DM/ha) from pastures sown with (plus) or without (minus) clover, and receiving High or Low rates of nitrogen (N) fertiliser annually.
Significant interactions between N and clover were identified on 11 occasions (). These interactions were generally the result of a strong response in adjusted herbage accumulation in the Low N plus clover treatment, resulting in seasonal or total annual adjusted herbage accumulation for this treatment combination that were markedly higher than the Low N minus clover treatment, and similar to one or both of the High N treatments.
The apparent efficiency of the annual herbage accumulation response to additional N fertiliser differed between the clover treatments. The apparent response to additional N fertiliser was consistently greater in the without clover treatment (18.2, 17.2 and 21.5 vs. 4.8, 9.8 and 4.0 kg DM/kg of additional N in 2012/2013, 2013/2014 and 2014/2015, respectively).
Effects of cultivar, and their interactions with N and clover
A significant effect of perennial ryegrass cultivar on seasonal and total annual adjusted herbage accumulation was identified on 10 occasions (). One50 AR37 and Prospect AR37 were generally among the highest ranked cultivars, while Abermagic AR1 was generally among the lowest ranked cultivars. The difference in total annual adjusted herbage accumulation between the lowest and highest ranked cultivars ranged from 0.9 to 1.8 t DM/ha/year.
Table 2. Seasonal and total annual adjusted herbage accumulation (kg DM/ha) from pastures sown with eight different perennial ryegrass cultivars with contrasting morphology and heading dates.
The cultivar × N interaction was significant in winter 2013 (P < .01; ). This scaling-type interaction arose because although the adjusted herbage accumulation of the cultivars increased when they received high rates of N fertiliser compared with low rates, the increase was significant for all cultivars with the exception of Kamo AR37 and Commando AR37. There were no significant clover × cultivar interactions.
Figure 2. Significant nitrogen (N) × cultivar interaction detected in the seasonal adjusted herbage accumulation data during winter 2013. Pastures were sown with eight different perennial ryegrass cultivars and received High (▪) or Low (□) rates of N fertiliser annually. The error bars indicate the maximum standard error of the difference for comparing N treatment means for a cultivar as well as cultivars within each N treatment.
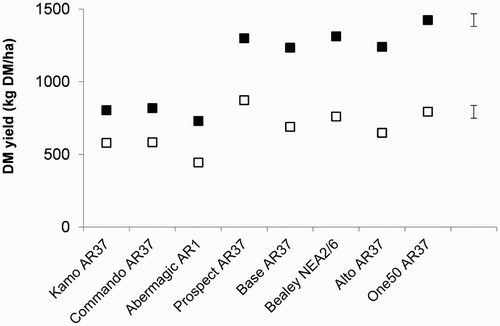
Adjusted herbage accumulation: phenotype contrast level
Morphology contrast
Adjusted herbage accumulation of cultivars representing the dense, fine-leaved phenotype (Abermagic AR1, Prospect AR37) was significantly greater than for cultivars representing the open, broad-leaved phenotype (Base AR37, Bealey NEA2/6) on four of the 17 occasions (early spring 2012 and late spring 2012, 2013 and 2014; P < .05; ). No significant N × clover × morphology group, N × morphology group or clover × morphology group interactions were identified.
Heading date contrast
Adjusted herbage accumulation of cultivars representing the late season heading date group (Alto AR37, One50 AR37) was significantly greater than cultivars representing the mid-season heading date group (Commando AR37, Kamo AR37) on four occasions (autumn 2013, summer 2013/2014, autumn 2015 and the 2014/2015 year; P < .05; ). Conversely, the mid-season heading date group had greater adjusted herbage accumulation than the late season heading date group during early spring 2012 (P < .001; ).
In winter 2013, a scaling-type interaction between N treatment and heading date group was identified whereby the adjusted herbage accumulation of cultivars was greater when they received high rates of N fertiliser compared with low rates, however the increase in herbage accumulation was greater for the late season heading date group compared with the mid-season heading date group (P < .001; data not shown). No significant N × clover × heading date group or clover × heading date group interactions were identified.
Nutritive value: cultivar level
No N × clover × cultivar interactions for CP or ME concentration were detected during the three years; however, there was one for NDF concentration (autumn 2015; P < .05; Supplementary Table S1).
During the three years, additional N fertiliser often increased herbage CP concentration, though effects on NDF concentration and ME density were sporadic and variable (). Sowing pastures with clover also generally increased herbage CP concentration and reduced NDF concentration (). Significant interactions between N and clover were common (). In many cases, the interaction was the result of an increase in CP concentration in the Low N plus clover treatment, resulting in values for this treatment combination that were markedly higher than the Low N minus clover treatment, and similar to one or both of the High N treatments. The opposite was generally true for NDF concentration, with the interaction generally the result of a decrease in NDF in the Low N plus clover treatment. This resulted in values for this treatment combination that were markedly lower than the Low N minus clover treatment, and similar to or lower than the High N treatments. Interactions between N and clover were only evident on three occasions for ME density, and again these were due to an increase in ME density in the Low N plus clover treatment.
Table 3. Seasonal nutritive value characteristics from pastures sown with (plus) or without (minus) clover, and receiving High or Low rates of nitrogen (N) fertiliser annually.
Herbage CP and NDF concentration and ME density varied with cultivar (). The two tetraploids, Base AR37 and Bealey NEA2/6, and the diploid Abermagic AR1 were generally among the highest ranked cultivars for ME density, while Commando AR37, Kamo AR37 and Prospect AR37 were consistently the lowest ranked cultivars.
Table 4. Seasonal nutritive value characteristics from pastures sown with eight different perennial ryegrass cultivars.
Of the nine analyses for each nutritive value variable, the clover × cultivar term was significant for NDF concentration in summer 2012/2013 (P < .001; A), late spring 2013 (P < .05; B) and summer 2014/2015 (P < .05; C), and significant for ME density in summer 2012/2013 (P < .01; D) and summer 2013/2014 (P < .001; E). The interactions for NDF concentration arose for one of two reasons: either because NDF concentration was similar across clover treatments for the majority of the cultivars, whilst other cultivars (Commando AR37 and/or Kamo AR37) had greater NDF concentration in the without clover treatment compared with the with clover treatment (summer 2012/2013, summer 2014/2015); or because while the difference in NDF concentration between the two clover treatments was not significant, the magnitude of the difference differed between cultivars (late spring 2013). Similarly, the interactions for ME density arose because while ME was similar across clover treatments for the majority of the cultivars, other cultivars (Kamo AR37 and/or Commando AR37) had lower ME density in the without clover treatment compared with the with clover treatment (summer 2012/2013, summer 2013/2014). No evidence of significant interactions between clover treatment and cultivar was found for CP concentration.
Figure 3. Significant clover × cultivar interactions detected in neutral detergent fibre (NDF) concentration data during (A) summer 2012/2013, (B) late spring 2013 and (C) summer 2014/2015, and in metabolisable energy (ME) density data during (D) summer 2012/2013 and (E) summer 2013/2014. Pastures were sown with eight different perennial ryegrass cultivars with (●) or without (○) clover. The error bars indicate the maximum standard error of the difference for comparing clover treatment means for a cultivar as well as cultivars within each clover treatment.
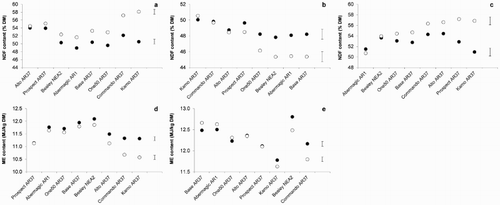
Nutritive value: phenotype contrast level
Morphology contrast
In late spring 2013, CP concentration was greater in herbage from the dense, fine-leaved cultivar group compared with that from the open, broad-leaved group (P < .05; ). There was no effect of morphology group on NDF concentration or ME density, or any significant N × morphology group or clover × morphology group interactions for any of the three nutritive value variables.
Heading date contrast
An effect of heading date group on CP concentration was identified on seven occasions (summer 2012/2013, P < .01; autumn 2013, P < .05; late spring 2013, P < .05; summer 2013/2014, P < .001; autumn 2014, P < .001; summer 2014/2015, P < .05; autumn 2015, P < .001). In summer and autumn of all three years, herbage from pastures sown with mid-season heading cultivars had greater CP concentration than that from pastures sown with late season heading cultivars, with the opposite during late spring 2013 (). In summer 2013/2014 there was also a clover × heading date group interaction (P < .05; data not shown) whereby in the mixed pastures the CP concentration of herbage from pastures sown with mid- and late heading cultivars was similar, but in the monocultures herbage from pastures sown with mid-season heading cultivars had greater CP concentration than that from late season heading cultivars. No interactions between N treatment and heading date were identified in CP concentration data.
In autumn 2014, NDF concentration was greater in herbage from pastures sown with late season heading cultivars compared with mid-season heading cultivars (P < .05) while the opposite occurred in late spring 2014 (P < .001; ). No N × heading date or clover × heading date interactions were identified in NDF concentration data.
ME density was greater in herbage from pastures sown with late season heading cultivars compared with mid-season heading cultivars in autumn 2013, late spring 2014 and autumn 2015 (P < .01; ). In summer 2012/2013, there was a clover × heading date interaction (P < .01; data not shown), whereby ME density was greater in pastures sown with clover compared with those without clover, and the increase was greater for mid-season heading cultivars than late season heading cultivars. Clover × heading date interactions also existed in autumn 2013 (P < .05) and summer 2013/2014 (P < .01; data not shown), in which ME density from late season heading cultivars was similar regardless of whether they were sown with or without clover, however herbage from the mid-season heading date group had greater ME density when they were sown with clover. No N × heading date interactions were identified in ME density data.
Botanical composition: cultivar level
Differences were common in the botanical composition of pasture from the four main treatments (). Pastures receiving high rates of N fertiliser had greater perennial ryegrass content than those receiving low rates of N during eight of the nine year × season combinations (P < .01), as well as containing less dead material (P < .05; four occasions) and other unsown species (P < .05; five occasions). Looking at the with clover treatments only, the main effect of N on clover content was significant for seven of the nine occasions (P < .05), with a trend towards significance on the other two occasions (late spring 2014, P = .06; summer 2014/2015, P = .07). On these nine occasions, mixed swards receiving low rates of N fertiliser contained more than double the clover content of those receiving high rates of N fertiliser.
Figure 4. Seasonal botanical composition of pastures during late spring (LSp), summer (Sum) and autumn (Aut). The four treatments were: HN+c, High nitrogen (N) plus clover; HN-c, High N minus clover; LN+c, Low N plus clover; and LN-c, Low N minus clover. Components include perennial ryegrass (grey), white clover (white), unsown species (black) and dead material (diagonal lines).
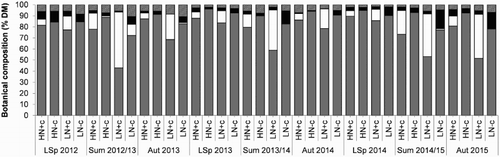
The main effect of cultivar on perennial ryegrass and clover contents was significant for five and four of the nine occasions, respectively (). There were no significant interactions between N and cultivar for perennial ryegrass or clover content during the three years.
Table 5. Seasonal perennial ryegrass and white clover content (% of above residual herbage DM) of pastures sown with eight different perennial ryegrass cultivars.
Botanical composition: phenotype contrast level
No effect of morphology group was found on the clover content of pastures, and there were no N × morphology group interactions.
The heading date contrast affected clover content on four occasions (summer 2012/2013, autumn 2013, summer 2013/2014 and autumn 2015; P < .05). In each case, the clover content of mixed pastures sown with mid-season flowering cultivars was greater than that of mixed pastures sown with late season flowering cultivars (). There were no N × heading date group interactions.
Perennial ryegrass tiller density
Nitrogen × clover × cultivar interactions were evident in the perennial ryegrass tiller density data in two out of the three years (P < .05; Supplementary Table S1).
No evidence of N × clover interactions was found in the perennial ryegrass tiller density data. A positive effect of N fertiliser on tiller density was evident at the end of the first year (P < .001; ); beyond which tiller density was similar across N treatments. Pastures sown as mixed swards with clover had a lower density of perennial ryegrass tillers than those sown as monocultures during each of the three years (P < .001).
Table 6. Perennial ryegrass and white clover population density in pastures sown with (plus) or without (minus) white clover, and receiving high or low rates of nitrogen (N) fertiliser annually.
Bealey NEA2/6 consistently had the lowest tiller density, while Kamo AR37, Commando AR37 and Abermagic AR1 were among the densest (P < .001; ). No evidence of clover × cultivar interactions was found in the tiller density data.
Table 7. Perennial ryegrass and white clover population density in pastures sown with eight different ryegrass cultivars with contrasting morphology and heading dates.
White clover growing point density
A negative effect of N fertiliser on clover growing point density was observed in the with clover treatments throughout the experiment (). Growing point density was similar across cultivars in autumn 2013 but differed among cultivars in autumn 2014 (P < .05; ). No N × cultivar interactions in white clover growing point density were detected in any of the three years.
Invertebrate pests
During the 4 years of sampling, the invertebrate pest population was dominated by clover root weevil (CRW) (Sitona obsoletus), although there were significant numbers of porina (Wiseana spp.) larvae present in 2013, the first year of the trial. These larvae were very small and only found at the surface, and were thus likely to be the result of a recent deposition of eggs. Plus clover treatments had significantly higher densities of CRW in 2013, 2015 and 2016 compared to minus clover treatments (data were too sparse to analyse in 2014) (). This difference was only significant for the Low N treatments in which populations overall were significantly higher than in High N treatments. Across all 4 years, populations were higher under Low N plus clover treatments (P = .008). There was significant annual variation in populations (P = .002) and significant interactions between year and clover treatment (P < .001) and year and N treatment (P = .005) (data not presented). The only significant effect of cultivar was apparent in a Year × N × Cultivar interaction (P = .002) reflecting a particularly high CRW population in the Base AR37 Low N plus clover treatment (450/m2), compared with a mean of 148/m2 for the other cultivars in the same treatment combination, with no such difference and much lower populations in the High N plus clover treatments. Populations of porina in 2013 were not affected by the presence of clover but were significantly higher under High N than Low N (43/m2 cf. 23/m2; SED 6.6; P = .01). Densities of both Argentine stem weevil and grass grub larvae remained low throughout the 4 years (<10/m2 for ASW; grass grub larvae increased to 14.4/m2 in clover plots in 2016). Nevertheless these insects contributed to total pest populations which were significantly higher under plus clover than minus clover in all years and in Low N treatments compared with High N in 2015.
Table 8. Density (number/m2 (Log N + 12/m2)) of CRW in pastures sown with (plus) or without (minus) white clover, and receiving High or Low rates of nitrogen (N) fertiliser annually.
Besides CRW, other root inhabiting pests present were the root aphid (Aploneura lentisci) and plant parasitic nematodes. A low incidence of root aphid in the first year (<5%) built up to an average of 14% of samples infested in 2015 and 2016, but data were too sparse for analysis. The plant feeding nematode fauna were comprised mainly of Pratylenchus and Paratylenchus species with low numbers of Heterodera. Meloidogyne species were absent from this site. Pratylenchus populations on Base AR37 (Log(n+0.045)/g dry soil – 1.35) were similar to those on Bealey NEA2 (1.04) but significantly greater than those on Abermagic AR1 (0.75) and One50 AR37 (0.49) (df 48, SED = 0.255, P = .029). Cultivar had no effect on numbers of Paratylenchus or Heterodera spp. Base AR37 also had the higher populations of all plant feeding nematodes (884/100 g dry soil) compared with Abermagic AR1 (507), Bealey NEA2 (587) and One50 AR37(468) (df 48, SED 1.283, P = .028). With the exception of 2014 when all nematode species occurred in low numbers, populations of Pratylenchus spp. increased between 2013 and 2016, whereas both Paratylenchus and Heterodera declined (data not presented).
Discussion
The complete absence of clover × cultivar interactions in herbage accumulation at this site supports the hypothesis behind this investigation, that is, that the relative yield rankings of perennial ryegrass cultivars do not differ when they are grown as monocultures or in mixtures with white clover (Chapman et al. Citation2018). The general strong presence of clover in the mixture treatments created marked differences in the productive properties of mixtures compared with monocultures, thus satisfying one of the key conditions required to provide a rigorous test of the hypothesis (Chapman et al. Citation2018). In summer, white clover contributed between 30% and 50% of total pasture dry matter in the Low N mixture treatment, and between 10% and 20% in the High N mixture treatment, over the three years of the study (). These contributions suggest the clover populations ( and ) were sufficiently strong to allow competitive or other interactions between the grass and the clover to emerge if they were present.
The high clover content in the Low N pastures also resulted in higher densities of CRW compared with High N clover pastures in two of the four years of monitoring, and overall. Measured in summer – early autumn, and with the advantage of irrigation that is known to increase reproduction (Addison et al. Citation1998), there was the potential for larval populations to increase substantially and cause significant damage to clover in the following winter and spring period (Gerard et al. Citation2010). It is possible, therefore, that higher CRW populations recorded in Low N than in High N clover treatments in autumn 2013 contributed to a difference in adjusted herbage accumulation between these treatments in late spring 2013. Very high populations of CRW recorded in autumn 2015, especially in Base AR37, may have had a similar effect. Populations of other pests that are favoured by the presence of clover and can affect pasture composition such as grass grub remained very low throughout this trial. Plant-feeding nematodes also did not occur in high numbers.
While significant effects of cultivar on the clover content (% DM) of the mixtures were recorded in four of the nine seasons when botanical composition was assessed (), these were evidently not large enough to affect the relative yielding rankings of the associated grasses observed in the monocultures. The phenotype contrast embedded in the experimental design showed that the general trend was towards higher clover content in pastures sown with mid-season heading cultivars compared with late-heading cultivars. This heading date effect was significant in two out of the three summers, and two out of the three autumns in which botanical composition was measured. The negative relationship between clover content in mixtures and heading date of the associated ryegrass is well-established from UK studies (Collins and Rhodes Citation1989; Davies and Fothergill Citation1990; Gilliland Citation1996).
For New Zealand ryegrass cultivars, the relationship between heading date and yield generally goes in the opposite direction (Lee et al. Citation2012) to that between heading date and clover content. The top-ranking bands in the FVI are dominated by late heading cultivars (Chapman et al. Citation2017). In the heading date contrast included in the present study, the herbage accumulation of the late heading cultivars was greater than that from the mid-season cultivars in four out of the 17 seasonal/total annual data sets available (autumn two occasions, summer one occasion, total annual one occasion). The obvious explanation for the negative relationship between heading date and clover content is that the higher yielding potential of later heading material leads to greater competition between grass and clover, particularly in the transition between spring and summer when clover is normally responding to increasing temperatures. It is well-known that breeding for later heading in perennial ryegrass has led to increased grass yields in late spring and early summer compared with mid-season heading types (Stewart Citation2006; Lee et al. Citation2012). This shift in seasonal growth pattern could restrict clover population expansion and subsequent growth in the warm seasons of the year when clover has a relative competitive advantage through its higher optimum temperature for growth compared with ryegrass (Mitchell Citation1956).
While clover × cultivar interactions were not evident for herbage accumulation, significant interactions were observed for nutritive value. These were all scaling interactions rather than re-ranking interactions, and reflected two factors: 1) the well-known species differences in fibre (NDF, generally lower in clover than grass) and crude protein (generally higher in clover); and 2) the trend towards higher clover content in mid-season cultivars compared with late heading cultivars. In general, pastures based on the mid-season cultivars Kamo AR37 and Commando AR37 had much lower NDF concentration and higher ME density in summer when mixed with clover than when grown as a monoculture. While the trend was in the same direction for other cultivars, the magnitude of the difference was much smaller (). The scaling interaction was not always driven by just the mid-season cultivars (Prospect and Bealey were also involved on occasions, ), but they were involved in all cases. This indicates that the use of a scaling factor for nutritive value performance values for mid-season cultivars may be justified in the FVI to account for cultivar × clover interactions in mixtures, assuming nutritive value is defined using an energy-supply related measure (ME density, or fibre concentration which is the major compositional factor influencing ME density) and will be evaluated routinely in monocultures (as is the case for DM yield).
Total annual herbage accumulation measured here was lower than typically assumed for fully irrigated pastures on silt loam soils in coastal Canterbury (closer to 18–20 t DM/ha per year, DairyNZ Citation2008). Yields in the range 16–18 t DM/ha have been recorded in closely monitored dairy systems trials at Lincoln (Chapman et al. Citation2012) and at the Lincoln University Demonstration Dairy Farm (van Bysterveldt and Christie Citation2007). The lower herbage accumulation recorded here was most likely related to the recent agricultural use of the paddock in which the experiment was conducted. It had a long history of cropping and had been in grazed pasture for less than one year before the ground was prepared for sowing the experiment (Rossi Citation2017). Hence, soil organic matter levels were likely low compared with those commonly found under long-term pasture. The low crude protein concentration of herbage in the Low N minus clover treatment (, mean 14.8%, that is, less than 2.5% N which is indicative of N deficiency, Whitehead Citation2000) and the strong apparent N response efficiency observed in the High N minus clover treatment (average across all seasons and years of approximately 18 kg DM/kg N applied in fertiliser) are all indicative of low organic N levels and likely dominance of immobilisation over mineralisation processes in the soil.
The apparent low baseline soil N status of the site probably reduced the competitive advantage of the grass relative to the clover compared with what might be expected at a site with a longer history under grazed pasture. This could, therefore, have influenced grass-clover interactions throughout the experiment, but any such influence should have been positive for the test of the hypothesis since there was a clear opportunity for clover to achieve yield parity with ryegrass in some situations (e.g. in summer in the Low N plus clover treatment, ). Despite this, relative yield rankings of ryegrass cultivars and phenotype groupings were the same in the mixtures as in the monocultures ().
The total annual herbage accumulation data show an increasing trend over time (), suggesting that the balance between immobilisation and mineralisation was changing as would be expected when soil use changes from cropping to grazing (e.g. Nguyen et al. Citation1995). This transition would have been enhanced in the plus clover treatments compared with the minus clover treatments through biological nitrogen fixation. There is circumstantial evidence for this in , which shows that mean herbage accumulation in the minus clover treatments increased by 1.7 t DM/ha per year (17%) from year 1 to year 3, whereas it increased by 3.2 t DM/ha per year (27%) in the plus clover treatments.
White clover DM yield and associated N fixation rates would have been the driving force behind these differences (Whitehead Citation1995). Pasture botanical composition was assessed only once per season in the main growing seasons of the year, rather than at every grazing event. Nonetheless, this information can be used to interpolate likely clover herbage accumulation in the mixtures, albeit with the caveat that short term shifts in species composition within seasons could have occurred. Total annual clover herbage accumulation estimated using this method was 3250 and 1250 kg DM/ha per year (mean across all three years) in the Low N plus clover and High N plus clover treatments, respectively. Assuming white clover fixes 40 kg N/tonne clover DM in irrigated pastures in Canterbury growing around 14 t DM/ha/year (Crush Citation1979), an additional 130 and 50 kg N/ha per year could have been added to the soil-pasture system through fixation in the respective treatments. Approximately 45% of N fixed by clover is transferred to grass in grazed New Zealand dairy pastures (Ledgard Citation1991) implying an additional 55–60 and 20–25 kg N/ha per year was available for grass growth in the respective treatments. Using the ryegrass herbage accumulation data and the apparent N response efficiencies of grass derived from the Low N versus High N monoculture treatments, Chapman et al. (Citation2016) calculated that grass must have benefitted from an additional 53 and 16 kg N/ha per year in the respective treatments. These values align well with the calculations above, which are based on previously published N fixation efficiencies and N transfer rates.
Herbage accumulation in the final year, 2014/2015, may have been limited by dry soil conditions in spring 2014 when low rainfall was not supplemented by irrigation (C). Estimated annual soil water deficits of 250–280 mm (see the Climate sub-section in Results) indicate that irrigation did not fully remove soil moisture limitations during late spring, summer and autumn in any of the three years. This may also have contributed to the lower than expected total annual herbage accumulation recorded in the experiment, since irrigation efficiency is a major factor controlling pasture yield in Canterbury (Rickard and McBride Citation1986).
The apparent low background soil organic N content and resulting strong grass growth responses to N provided an opportunity to gauge whether perennial ryegrass cultivars differ in their N fertiliser response. The N × cultivar interaction term was significant in only one of the 17 seasonal/total annual data sets available, viz winter 2013. Therefore, there is little evidence to suggest there are genetic differences in the responsiveness of perennial ryegrass to added N, at least within the range of cultivars and N rates used here. Like the clover × cultivar interactions in nutritive value, the winter 2013 result was also a scaling interaction (). It appeared to be driven by a separation of cultivars bred using germplasm from north-west Spain (Prospect, Base, Bealey, Alto and One50) from New Zealand ecotypes (Kamo and Commando) and the one northern European cultivar included in the experiment (Abermagic). Herbage accumulation in the High N rate treatment was approximately double that of the low N rate for the former group of cultivars, whereas the yield difference between treatments in cultivars in the latter grouping was much less.
The greater winter growth of ryegrass material from north-west Spain is well known (Stewart Citation2006), and is reflected in the relative winter DM yield performance values in the FVI – where cultivars with some Spanish germplasm consistently rank much higher than those without (Chapman et al. Citation2017; https://www.dairynz.co.nz/feed/pasture-renewal/select-pasture-species/cultivar-selector-tool/). However, it is important to note that, in this experiment, N fertiliser was not applied during winter. Therefore, any growth difference in winter must be a result of carry-over effects of differences in soil N status or pasture composition from earlier in the year. The latter is relevant here, since mixtures based on Kamo and Commando (which are also mid-season heading) supported greater white clover contents than the other cultivars () and, therefore, more N would have been fixed and available for grass growth.
Abermagic doesn't fit this mould. It is a late heading cultivar, and supported similar clover contents to the other late heading cultivars. It was bred from ecotypes from high latitude zones of the northern hemisphere and its performance values for winter and early spring DM yield in the FVI are low for all New Zealand regions (1- or 2-star band, in 5-band rating system, Chapman et al. Citation2017; https://www.dairynz.co.nz/feed/pasture-renewal/select-pasture-species/cultivar-selector-tool/). Thus, both genetic differences in growth at low temperatures, and differences between heading dates in sward clover content, are likely to be involved.
These types of interactions in pastures highlight the challenges involved in ensuring that multi-trait evaluation systems for grasses adequately capture the results that farmers are likely to see in their farm systems. It is impossible to meet this challenge in full for every region, let alone every farm. However, information of the type gained from experiments such as that reported here helps provide a scientific basis to any decisions that are made to ensure that the evaluation system is robust.
Conclusion
This experiment supports the hypothesis that the relative herbage accumulation rankings of ryegrass cultivars do not differ when ryegrass is grown in monoculture or in mixtures with white clover within three years of sowing. Therefore, there is no clear case for adjusting herbage accumulation performance values in the FVI. Significant clover × cultivar interactions in NDF concentration and ME density of the whole pasture were, however, observed on several occasions. These were driven by the effects of ryegrass cultivar, particularly heading date, on the clover content of mixtures. Performance values for nutritive value of mid-season cultivars may need to be scaled in the FVI to account for these interactions in farm systems.
Supplementary Table 1. Three-way interactions between nitrogen, clover and cultivar.
Download MS Word (27.5 KB)Acknowledgements
Sincere thanks to the large number of technical and farm staff involved and to Barbara Dow (DairyNZ) for statistical analyses. Thanks also to Dr David Hume and Anouck de Bonth (AgResearch) for endophyte infection analysis and to Lily Ouyang (AgResearch) for assessment of the existing seedbank.
Disclosure statement
No potential conflict of interest was reported by the authors.
ORCID
David F. Chapman http://orcid.org/0000-0002-6997-2380
Julia M. Lee http://orcid.org/0000-0002-2442-2086
Additional information
Funding
References
- Addison PJ, Willoughby BE, Hardwick S, Gerard PJ. 1998. Clover root weevil: observations on differences between 1997 and 1998 summer populations in the Waikato. Proceedings of the 51st New Zealand Plant Protection Conference, 1–4.
- Brougham RW, Ball PR, Williams WM. 1978. The ecology and management of white-clover based pastures. In: Wilson JR, editor. Plant relations in pastures. East Melbourne: CSIRO; p. 309–324
- Chapman DF, Bryant JR, Olayemi ME, Edwards GR, Thorrold BS, McMillan WH, Kerr GA, Judson G, Cookson T, Moorhead A, et al. 2017. An economically based evaluation index for perennial and short-term ryegrasses in New Zealand dairy farm systems. Grass and Forage Science. 72:1–21. doi: 10.1111/gfs.12213
- Chapman D, Clark D, Beukes P, Edwards G, Dalley D, Pinxterhuis I, Romera A, Shepherd M, Cameron K, Di H, et al. 2012. Strategies for increasing system productivity while controlling environmental footprint: a common systems research approach in two regions of New Zealand. In: Jacobs J, editor. Proceedings of the 5th Australasian Dairy Science Symposium. p. 417–421.
- Chapman DF, Lee JM, Rossi L, Cosgrove GP, Stevens DR, Crush JR, King WM, Edwards GR. 2018. Implications of grass-clover interactions in dairy pastures for forage value indexing systems. 1. Context and rationale. New Zealand Journal of Agricultural Research. doi:10.1080/00288233.2017.1402353.
- Chapman DF, Lee JM, Rossi L, Edwards GR, Pinxterhuis JB, Minnee EMK. 2016. White clover: the forgotten component of high-producing pastures? Animal Production Science. 57:1269–1276 doi: 10.1071/AN16453
- Collins RP, Rhodes I. 1989. Yield of white clover populations in mixture with contrasting perennial ryegrasses. Grass and Forage Science. 44:111–115 doi: 10.1111/j.1365-2494.1989.tb01918.x
- Corson DC, Waghorn GC, Ulyatt MJ, Lee J. 1999. NIRS: forage analysis and livestock feeding. Proceedings of the New Zealand Grassland Association. 61:127–132.
- Crush JR. 1979. Nitrogen fixation in pasture. IX. Canterbury Plains, Kirwee. New Zealand Journal of Experimental Agriculture. 7:35–38. doi: 10.1080/03015521.1979.10426159
- DairyNZ. 2008. DairyNZ Farmfact 1–15 Using the rising plate meter. Hamilton: DairyNZ Ltd
- DairyNZ. 2016. New Zealand dairy statistics 2015–16. Hamilton: DairyNZ Ltd.
- Davies DA, Fothergill M. 1990. Productivity and persistence of white clover grown with three perennial ryegrass varieties and continuously stocked with sheep. In: Soil-grassland-animal relationships. Proceedings of 13th General Meeting of the European Grassland Federation, Banska Bystrica, Czechoslovakia, Jun 25–29, 1990, Volume 2. p. 157–162.
- East R, Willoughby BE. 1980. Effects of pasture defoliation in summer on grass grub (Costelytra zealandica) populations. New Zealand Journal of Agricultural Research. 23:547–562. doi: 10.1080/00288233.1980.10417882
- Easton HS, Baird DB, Cameron NE, Kerr GA, Norriss M, Stewart AV. 2001. Perennial ryegrass cultivars: herbage yield in multi-site plot trials. Proceedings of the New Zealand Grassland Association. 63:183–188.
- Eerens JPJ, Hardwick S. 2003. Factors affecting clover root weevil (Sitona lepidus) numbers and clover production. New Zealand Journal of Agricultural Research. 46:305–310. doi: 10.1080/00288233.2003.9513558
- Gerard PJ, Goldson SL, Hardwick S, Addison PJ, Willoughby BE. 2010. The bionomics of an invasive species Sitona lepidus during its establishment in New Zealand. Bulletin of Entomological Research. 100:339–346. doi: 10.1017/S0007485309990411
- Gilliland TJ. 1996. Assessment of perennial ryegrass variety compatibility with white clover under grazing. Plant Varieties and Seeds. 9:65–75.
- Hahn H, Huth W, Schöberlein W, Diepenbrock W. 2003. Detection of endophytic fungi in Festuca spp. by means of tissue print immunoassay. Plant Breeding. 122:217–222. doi: 10.1046/j.1439-0523.2003.00855.x
- Hewitt AE. 1998. New Zealand soil classification. Landcare Research Science Series No. 1. Lincoln: Manaaki Whenua, Landcare Research.
- Hunt WF, Gaynor DL. 1982. Argentine stem weevil effects on Nui and Ruanui ryegrasses grown with two levels of nitrogen/water nutrition. New Zealand Journal of Agricultural Research. 25:593–599. doi: 10.1080/00288233.1982.10425225
- Kerr GA, Chapman DF, Thom ER, Matthew C, Van Der Linden A, Baird DB, Johnston E, Corkran JR. 2012. Evaluating perennial ryegrass cultivars: improving testing. Proceedings of the New Zealand Grassland Association. 74:127–136.
- Ledgard SF. 1991. Transfer of fixed nitrogen from white clover to associated grasses in swards grazed by dairy cows, estimated using 15N methods. Plant and Soil. 131:215–223. doi: 10.1007/BF00009451
- Lee JM, Chapman DF, Popay AJ, Wims CM, Griffiths WM. 2018. Implications of grass-clover interactions in dairy pastures for forage value indexing systems. 2. Waikato. New Zealand Journal of Agricultural Research. doi:10.1080/00288233.2017.1394330.
- Lee JM, Matthew C, Thom ER, Chapman DF. 2012. Perennial ryegrass breeding in New Zealand: a dairy industry perspective. Crop and Pasture Science. 63:107–127. doi: 10.1071/CP11282
- Mitchell KJ. 1956. Growth of pasture species under controlled environment. 1. Growth at various levels of constant temperature. New Zealand Journal of Science and Technology. Section A. 38:203–216
- Nguyen Ml, Haynes RJ, Goh KM. 1995. Nutrient budgets and status in three pairs of conventional and alternative mixed cropping farms in Canterbury, New Zealand. Agriculture, Ecosystems and Environment. 52:149–162. doi: 10.1016/0167-8809(94)00544-O
- Rickard DS, McBride SD. 1986. Irrigated and non-irrigated pasture production at Winchmore, 1960–1985. Technical Report No. 21. Canterbury, New Zealand: Winchmore Irrigation Research Station, Ministry of Agriculture and Fisheries.
- Rossi L. 2017. Interactions between pasture species and management and their implications for evaluating perennial ryegrass (Lolium perenne) cultivars in dairy systems [PhD thesis]. Lincoln University.
- Stewart AV. 2006. Genetic origins of New Zealand perennial ryegrass (Lolium perenne) cultivars. In: Mercer CF, editor. Advances in Pasture Breeding. Grassland Research and Practice Series No. 12; p. 55–61.
- Tozer KN, Carswell K, Griffiths WM, Crush JR, Cameron CA, Chapman DF, Popay A, King W. 2017. Growth responses of diploid and tetraploid perennial ryegrass (Lolium perenne) to soil-moisture deficit, defoliation and a root-feeding invertebrate. Crop and Pasture Science. 68:632–642 doi: 10.1071/CP17154
- Tozer, KN, Chapman DF, Bell NL, Crush JR, King WM, Rennie GM, Wilson DJ, Mapp NR, Rossi L, Aalders LT, et al. 2014. Botanical survey of perennial ryegrass-based dairy pastures in three regions of New Zealand: implications for ryegrass persistence. New Zealand Journal of Agricultural Research. 57:14–29. doi: 10.1080/00288233.2013.863785
- van Bysterveldt A, Christie R. 2007. Dairy farmer adoption of science demonstrated by a commercially focussed demonstration farm. In: Chapman DF, Clark DA, Macmillan KL, Nation DP, editors. Proceedings of the 3rd Australasian Dairy Science Symposium. p. 535–540.
- VSN International. 2013. Genstat for windows. 16th ed.Hemel Hempstead (UK): VSN International.
- Whitehead DC. 1995. Grassland nitrogen. Wallingford (UK): CAB International.
- Whitehead DC. 2000. Nutrient elements in grasslands. Soil-plant-animal relationships. Wallingford (UK): CAB International.