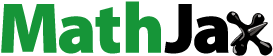
ABSTRACT
The effects of elevated aluminium (Al) concentrations on parameters indicating Al toxicity and Al detoxification were evaluated in two alfalfa populations from different acidic soil environment. Plants were grown in nutrient solutions at pH 4.5, 5.5 and 6.0, with different levels of Al contamination for 30 days. Shoot heights and root activity were significantly decreased in the Al treatment while free proline concentration was significantly increased. Furthermore, citric acid and oxalic acid concentrations tend to increase with increasing Al concentration. Different responses were also observed between the two populations. Shoot height, free proline concentration and root activity were significantly higher for alfalfa plants collected from an acidic soil environment. These plants also exhibited higher concentrations of citric and oxalic acids when exposed to 100 μM Al at pH 4.5. Overall, the results indicate that alfalfa plants grown on acidic soils might develop mechanisms to be less affected by high Al concentration.
Introduction
Alfalfa (Medicago sativa L.) has high protein and highly digestible fibre contents (Li et al., Citation2010) and is one of the most important forage crops in the world with nearly 32 million hectares cultivated chiefly in temperate regions (Du et al., Citation2009). Alfalfa is mainly cultivated in temperate regions, with the United States of America, Canada, Argentina, and China accounting for about 60% of the production (Hong et al. Citation2009). The increasing alfalfa consumption in the world necessitates to expand its production area in non-traditional cultivation areas, whereas about 40% of the world’s arable lands are acidic lands (Foy et al. Citation1978). Under acidic soil condition, Al3+ toxicity might pose a serious agricultural problem (Kochian Citation1995). Soil pH is strongly related to the levels of soil plant-available aluminium (Moir and Moot Citation2010). Especially when the soil pH drops below 5, the octahedral hexahydrate , more commonly referred to as Al3+, becomes soluble in the soil solution and might interfere with a wide range of physical and cellular process (Kinraide Citation1991; Martins, et al. Citation2013), resulting in the inhibition of plant growth and functions (Giannakoula et al. Citation2010; Ikka et al. Citation2013). The first reaction of plants grown on soils with elevated Al concentration was a decreasing root growth (Foy Citation1988). Furthermore, the dry weight of alfalfa was significantly reduced by Al additions, especially under acidic pH values (Langer et al. Citation2009). Shoot growth of plants may be negatively affected (Larsen et al. Citation1997). Exposed to Al, seedlings exhibited a reduced leaf size, and terminal buds, for example in sugar maple (Thornton et al. Citation1986).
Some plant species and cultivars show tolerance to Al toxicity. The mechanism of Al tolerance has been categorised into external or exclusion and internal detoxification mechanism (Matsumoto Citation2000; Kochian et al. Citation2004; Kochian et al. Citation2005). An early study in wheat (Triticum aestivum) reported that organic acids play a role both in Al exclusion, via release from root and Al detoxification in the symplsam, where organic acid such as citric acid and malic acid could chelate Al and reduce or prevent its toxic effects at the cellular level, in particular protecting enzyme activity internally in the plant from deleterious effect of Al (Delhaize et al., Citation1993). In plants like maize, the Al-induced citric acid exudation responses are regarded as a potential Al-exclusion mechanism (Mariano and Keltjens Citation2003). However, the type and amount of organic acids released in response to Al vary widely among plant species and even cultivars within species (Zheng et al. Citation1998; Kochian et al. Citation2004). Dong et al. (Citation2004) observed that soybeans exposed to Al stimulated the release of citric acid, whereas a phosphorus (P) deficiency increased the exudation of malate and oxalate instead. Due to the important role of organic acids in internal and external Al detoxification (Ma and Hiradate Citation2000), they are suitable indicators for the ability of plants to react on elevated Al concentrations.
In addition, aluminium has also been observed to increase free proline concentrations under acidic condition in maize (Khan et al. Citation2000) and Plantago almogravensis and Plantago algarbiensis (Martins et al. Citation2013). Proline is generally assumed to serve as a physiologically compatible solute that accumulates as needed to maintain a favourable osmotic potential between the cell and its surroundings (Pollard and Wyn Jones Citation1979; Ashraf and Foolad Citation2007). Proline is also involved in alleviating cytosolic acidosis associated with several stresses (Kurkdjian and Guern Citation1989), therefore, proline accumulation can serve as a marker of plant response to stressful environments.
Alfalfa is sensitive to Al toxicity as a result performance is limited (Dall’Agnol et al. Citation1996; Langer et al. Citation2009; Khu et al. Citation2010). Its yield and stand duration in acid soils are compromised because of inhibited root growth and reduced nitrogen fixation (Hartel and Bouton Citation1989). According to Barone et al. (Citation2008), enhanced plant growth under aluminium-toxic conditions can be obtained in transgenic alfalfa plants expressing citrate synthase. However, no commercially available Al-tolerant alfalfa cultivar has been developed at present (Khu et al. Citation2010); there is still little information known about the mechanisms by which alfalfa responds to and resist Al stress. In our study, two populations of alfalfa that adapted to different soil pH value, respectively, were employed as experimental materials (). The aim of this investigation was to (a) determine root activity, free proline and organic acid accumulation as affected by Al rhizotoxicity; (b) evaluate whether alfalfa plants grown on acidic soils develop mechanisms to tolerate high Al concentration.
Table 1. The origin of the investigated two alfalfa populations and soil conditions from the collection area.
Materials and methods
Plant material and growth conditions
Two alfalfa populations–Dali and Tonghai were used in this study (). Seeds were collected from the Yunnan-Guizhou Plateau, southwestern China. After surface sterilisation of the seeds with HgCl2 (0.1%), they were put on filter paper saturated with distilled water in Petri dishes and incubated in a growth chamber at 25°C for 4 days. Then, seedlings were selected for uniformity and then transplanted to polypropylene pots containing the nutrient solution (Hoagland and Arnon Citation1959). The nutrient solutions consisted of 2.5 mM KNO3, 2.5 mM Ca(NO3)2, 1 mM MgSO4, 0.5 mM KH2PO4, 0.04 mM Fe(III)EDTA, 0.009 mM MnCl2·4H2O, 0.4 μM ZnSO4·7H2O, 0.3 μM CuSO4·5H2O, 0.0451 mM H3BO3, 0.072 μM NH4Mo 5H2O. Nutrient solutions were continuously aerated with pumps and renewed every 5 days. Three Al levels (0, 50 and 100 μM) added in the form of aluminium chloride (AlCl3) and three levels of pH (4.5, 5.5 and 6.0) were tested. The pH was kept constant by daily additions of 0.1 M hydrochloric acid (HCl) or sodium hydroxide (NaOH) as required. The experiment was conducted in a controlled-environment growth chamber for 30 d at 25°C with a 60 to 75% relative humidity and a 12 h light photoperiod.
Measurement of shoot height and free proline concentration
Plant growth was expressed as shoot height, measured after 30 days of Al treatment.
Free proline was measured as described by Bates et al. (Citation1973). The fully expanded and exposed leaves (0.2 g) were homogenised in 5 ml of 3% sulphosalicylic acid solution. After centrifugation, 2 ml supernatant, 2 ml glacial acetic acid and 2 ml 2.5% acid ninhydrin solution were added into a test tube covered with a Teflon cap. The absorbance of the free proline concentration was measured at 520 nm. The proline concentration was expressed as mmol g−1 fresh weight (FW).
Determination of the root activity
Root activity was quantified by measuring root dehydrogenase activity using triphenyltetrazolium chloride (TTC) reduction technique (Joslin and Henderson Citation1984), which is related positively to respiration capacity of different tissues like root. To determine the amount of live or dead roots in a sample, roots were first washed with deionised water, and fresh weight was determined. Roots then were placed into test tubes with 0.4% (w/v) TTC in 0.06 M Na2HPO4–KH2PO4 (pH 7.0) and tubes were incubated in the dark at 37°C water bath for 1 h. Formazan a water-insoluble red substance, which was formed from the reduction of TTC by dehydrogenase enzymes in living tissues, was extracted in ethyl acetate. The absorbance of the extract was recorded at 485 nm with a spectrophotometer (Shimadzu Scientific Instrument, Columbia, MD, USA). A regression of absorbance against root weight was determined for live roots to calculate the percentage of live root tissue among all roots in dry weight, which is considered the root activity.
Determination of organic acid
After 30 days of growth, shoots, leaves and roots were harvested and washed by deionised water. After mixing, shoots, leaves and roots (0.25 g) were ground in 0.5 M HCl, then solutions were bathed boiled water 20 min. In order to quantify the concentration of organic acids (oxalic, citric and malic acid), extracts were lyophilised and the residue re-dissolved in 5 ml of deionised water, after filtration (0.45 μm) HPLC analysis was carried out. Separation was achieved on a 250 × 4 mm reverse phase column (Merck, LiChrospher 100 RP-18, 5-μm particle size). Sample solutions (10 μL) were injected into the column, and a 5%CH3OH-0.1 ml KH2PO4 (pH 2.7) solution was used for isocratic elution at a flow rate of 0.8 ml min−1 with UV detection at 214 nm. Preliminary studies with standard organic acids indicated that recovery of the organic anions was about 98%. The concentration of organic acids was analysed by HPLC referred to Ma et al. (Citation1997).
Experimental design and data analysis
The experimental design was a randomised complete block with three replications. Statistical analyses were performed using the statistical software package for social science (SPSS) version 13. Two-way analyses of variance (ANOVA) were used to test the main effects of the Al treatment, populations and their interaction. Within each population, pairwise comparisons were made using the Student-Newman-Keuls multiple range tests. Differences were considered significant at the P < .05 level.
Results
Effect of Al on shoot height
For both populations, shoot heights were significantly decreased by 50 and 100 μM Al treatment, whereas a stronger inhibition was observed in the 100 µM Al treatment (). After 30 days, the growth was almost completely inhibited under the highest Al concentration. Also, the shoot height between the two populations differed significantly. Compared to Tonghai, shoot height under Al stress was about 39% lower in Dali. Moreover, the interaction between pH and Al has a significant effect on shoot height of Dali. A further decrease was observed when plants were treated by the highest Al and the lowest pH.
Figure 1. Shoot height of two alfalfa populations–Dali and Tonghai exposed to different aluminium concentrations and pH values. Each value is a mean ± SE. Different lower case letters denote statistically significant differences between the Al treatments (P .05) according to the Student-Newman-Keuls multiple range test. The results of analysis of variance are abbreviated as follows: Al = effect of the Al treatment; pH = effect of the pH treatment; and Al × pH = Al ×pH interaction effect.
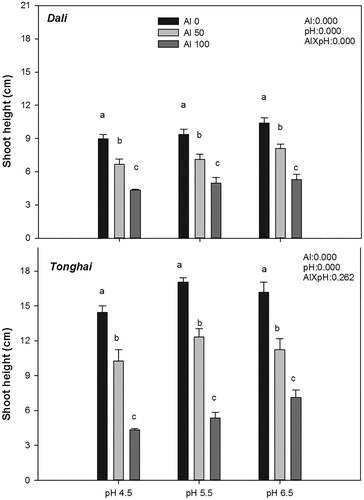
Effect of Al on free proline concentration
When plants were exposed to 50 and 100 µM Al, the free proline concentration was significantly increased in both alfalfa populations (P < .001) (), particularly at pH 4.5. However, with the increase of pH, less free proline was accumulated among all the investigated material. Furthermore, the magnitude of the response differed between pH values. At pH 4.5, free proline of Tonghai population in the 100 µM Al treatment accumulated to almost twice the amount of treatment without Al, whereas such responses were not observed at pH 6.5. The effect of Al toxicity on the free proline concentration was stronger in the Tonghai population compared to the Dali population.
Figure 2. Free proline concentrations of two alfalfa populations–Dali and Tonghai exposed to different aluminium concentrations and pH values. Each value is a mean ± SE. Different lower case letters denote statistically significant differences between the Al treatments (P < .05) according to the Student-Newman-Keuls multiple range test. The results of analysis of variance are abbreviated as follows: Al = effect of the Al treatment; pH = effect of the pH treatment; and Al × pH = Al × pH interaction effect.
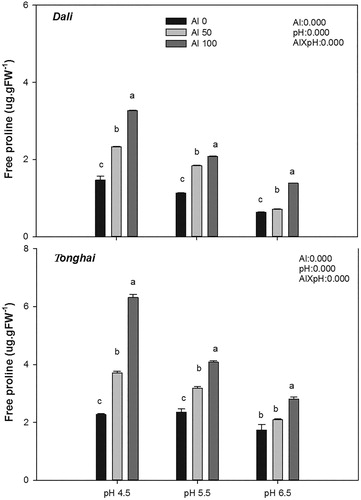
Effect of Al on root activity
For both populations, root activity decreased in parallel with the decrease of pH values. In the Dali population, root activity at pH 4.5 was significantly decreased in the 100 μM Al treatment (P < .05), but not in the 50 μM Al treatment. At all pH level, Tonghai showed the highest root activity (). In the 100 μM Al treatment at pH 4.5, the root activity of Tonghai population was 246% significantly higher than the root activity of the Dali population (P < .001).
Figure 3. Root activity of the two alfalfa populations–Dali and Tonghai exposed to different aluminium concentrations and pH values. Each value is a mean ± SE. Different lower case letters denote statistically significant differences between the Al treatments (P < .05) according to the Student-Newman-Keuls multiple range test. The results of analysis of variance are abbreviated as follows: Al = effect of the Al treatment; pH = effect of the pH treatment; and Al × pH = Al × pH interaction effect.
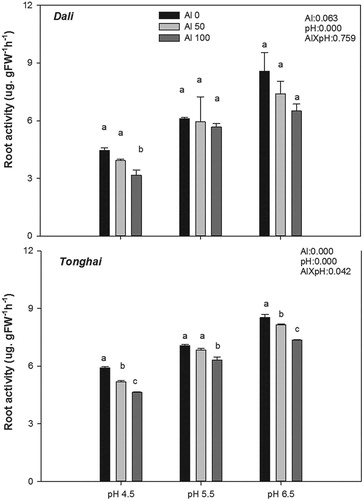
Effect of Al on organic acid accumulation
For both populations, citric acid contents were significantly affected by the Al concentration and the pH value; the citric acid content in the plants was significantly increased when exposed to 100 µM Al at pH 5.5 or 6.5 (). However, a significant difference was observed between the two populations. The citric acid content under Al stress in the Tonghai population was significantly higher than that in the Dali population (P < .001). Moreover, at pH 4.5, a concentration of 100 μM Al significantly increased the citric acid content in the Tonghai population by 90% compared to the control, whereas such effect was not observed in the Dali population.
Table 2. Citric acid, oxalic acid and malic acid concentrations in the plants of the alfalfa populations–Dali and Tonghai grown in nutrient solutions with different pH values and aluminium concentrations.
The oxalic acid concentration increased significantly in response to increasing Al concentrations; this effect was stronger at low pH values. At pH 4.5, the oxalic acid in the Tonghai population was three times higher than the control when exposed to 100 μM Al concentration; In contrast, the oxalic acid content in Dali was just increased by 40% compared to the control. However, the magnitude of increase depended on the alfalfa population, with concentrations in Tonghai population being significantly higher than in Dali population (P < .001).
Malic acid concentration was significantly affected by the pH value as well as the Al treatment. In Dali, malic acid tended to increase with increasing Al concentrations at pH 5.5 and 6.5, but not at pH 4.5. Particularly at pH 4.5, the malic acid concentration was significantly decreased by the 100 µM Al treatment while being increased by the 50 µM Al treatment. In addition, no significant difference in malic acid concentration was found between Tonghai and Dali populations.
Discussion
Aluminium has long been believed to be one of the major factors that limiting the worldwide crop production. Al has been a potential threat to affect legume forge production such as alfalfa in the world. Our results indicated that shoot height, free proline concentration, root activity as well as tissue concentrations of organic acids of M. Sativa growing in nutrient solutions were significantly affected by concentrations of 50 and 100 μM Al at different pH levels compared to plants grown without Al treatment,
The growth of the plant is widely studied concerned the toxicity of Al, thereat most investigations targeted on roots as this is the directly affected organ. It is found that exposure of root apex to Al causes a rapid inhibition of root growth (Ryan et al. Citation1993; Langer et al. Citation2009) as a result of Al-induced inhibition of H+-ATPase activity (Ahn et al. Citation2002), and depolarisation of the membrane (Pavlovkin et al. Citation2009). However, the suppressed effect on root depended on the legume species (Berenji et al. Citation2017).
Studies conducted so far indicate that there is little knowledge about Al effects on the aboveground parts (shoots and leaves) of plants. In our study, shoot growth was significantly inhibited by Al, especially at a pH value of 4.5. According to Rengel (Citation1996), the effects of Al3+ toxicity on shoots, such as restricted growth, become evident only after root growth is limited by exposure to toxic Al3+ levels in the rhizosphere, leading to mineral nutrition deficiencies in the aboveground tissues. Thus, the reduction in shoot growth and crop yield due to Al toxicity seems to be a long-term effect (Taylor Citation1988). In our study, we could show that the shoot height under Al stress differs significantly for different alfalfa populations; the Tonghai population which was collected from more acidic soil pH values (4.46) exhibited a larger shoot height than the Dali population collected from soils with a higher pH value. Also, Langer et al. (Citation2009) found that different alfalfa cultivars are significantly different affected in terms of shoot biomass under Al stress. Therefore, the magnitude of growth reduction depended on the alfalfa population. Similar results were also reported in high bush blueberry by Reyes-Díaz et al. (Citation2010) who investigated the effects of Al in nutrient solutions on relative growth rate. Moreover, different tolerance to Al toxicity between species was found in lotus and falcate lucerne (Moir et al. Citation2016).
In term of free proline concentrations, they were significantly increased in the experimental population in response to 50 and 100 µM Al. However, it was found that free proline concentration was more influenced by 100 µM Al than by 50 µM Al treatments. According to Giannakoula et al. (Citation2008; Citation2010) and Khan et al. (Citation2000), more increase of free proline was related to aluminium tolerance of plants. Increase in proline level suggests that anti-stress mechanism of alfalfa is efficient to control changes induced by aluminium, as also found by Yang and Cheng (Citation2001) in Mungbean as well as in P. almogravensis and P. algarbiensis (Martins et al. Citation2013).
Root activity brings about effective nutrients absorption at the root for growth and yield of crops. In our study root activity of alfalfa was significantly affected by Al treatment, particularly at pH 4.5. Root activity is not only related to the aerobic respiration of roots but also associated with dehydrogenase activity of tricarboxylic acid (TCA) cycle that regulates sugar and mineral absorption in plants (Onanuga et al. Citation2012). According to the study of Atkin et al. (Citation2000), a decrease in the rate of root growth lowers the overall requirements for respiratory ATP, thus the rate of total respiration. The decrease of root activity might attribute to the inhibition of root growth.
Many studies focused on the exudations of organic acid (Zheng et al. Citation1998; Dong et al. Citation2004; Langer et al. Citation2009). Organic acids like citric acid, oxalic acid and malic acid are considered to effectively detoxify Al (Kochian et al. Citation2004), and their content has been correlated with aluminium tolerance in higher plants. On the other hand, Takeda et al. (Citation1985) and Langer et al. (Citation2009) found that Al can accumulate to very high levels in shoot or root, leaves, for example in leaves of Buckwheat, the Al concentration was as high as 15,000 µg Al g−1 dry weight when the plant was grown on acid soils (Ma and Hiradate Citation2000). Therefore, organic acids with Al-chelating ability play an important role in the detoxification of Al both externally and internally (Ma and Hiradate Citation2000). It is equally important to learn the accumulation conditions of organic acid in plants. According to De la Fuente et al. (Citation1997), over production of citric acid was shown to result in aluminium tolerance in plants like transgenic tobacco (Nicotiana tabacum) and papaya (Carica papaya). Enhanced plant growth under acidic, aluminium-toxic conditions can be obtained in transgenic alfalfa plants expressing citrate synthase Barone et al. (Citation2008), Moreover, the citrate content of alfalfa and Eucalyptus increased with increasing Al application (Langer et al. Citation2009; Ikka et al. Citation2013). Our results are in agreement with these findings. At the same time, in our study, the content of citric and oxalic acid was much higher in Tonghai than in Dali population (P < .001) and increased in the presence of Al much more in the former than in the latter, suggesting that the increased content of these organic acids contributed to the Al resistance of Tonghai population. Role of organic acid to detoxify Al is different. Ma et al. (Citation1997) and Shen et al. (Citation2002) showed that most of the Al in plants of buckwheat was complexed with oxalate in a 1:3 Al-oxalate complex. Later, they found that al being transported to the shoot is complexed with citrate, not oxalate (Ma and Hiradate Citation2000). Based on the study of Andrade et al. (Citation2011), the Al-sensitive wheat cultivar had a higher concentration of malic acid regardless of the presence of Al. Considered no significant difference in the concentration of malic acid was found between Tonghai and Dali populations, hence, we believe that citric acid is much more effective at detoxifying Al in alfalfa than malic acid.
Conclusions
In our study, some growth and physiological properties of alfalfa were greatly affected by increases of Al content in growing solutions. The observed differences between the study populations in shoot height, free accumulation and root activity as well as content of citric, oxalic acid indicate the Tonghai population is more tolerant to Al stress than the Dali population. Therefore, we concluded that alfalfa plants grown on acidic soils might develop mechanisms to be less affected by high Al concentration.
Disclosure statement
No potential conflict of interest was reported by the authors.
Additional information
Funding
References
- Ahn SJ, Sivaguru M, Chung GC, Rengel Z, Matsumoto H. 2002. Aluminum- induced growth inhibition is associated with impaired efflux and influx of H+ across the plasma membrane in root apices of squash (Cucurbita pepo). Journal of Experimental Botany. 53:1959–1966. doi: 10.1093/jxb/erf049
- Andrade LRM, Ikeda M, Amaral LIV, Ishizuka J. 2011. Organic acid metabolism and root excretion of malate in wheat cultivars under aluminium stress. Plant Physiology and Biochemistry. 49:55–60. doi: 10.1016/j.plaphy.2010.09.023
- Ashraf M, Foolad MR. 2007. Role of glycine betaine and proline in improving plant abiotic stress resistance. Environmental and Experimental Botany. 59:206–216. doi: 10.1016/j.envexpbot.2005.12.006
- Atkin OK, Edwards EJ, Loveys BR. 2000. Response of root respiration to changes in temperature and its relevance to global warming. New Phytologist. 147:141–154. doi: 10.1046/j.1469-8137.2000.00683.x
- Barone P, Rosellini D, LaFayette P, Bouton J, Veronesi F, Parrott W. 2008. Bacterial citric acid synthase expression and soil aluminum tolerance in transgenic alfalfa. Plant Cell Reports. 27:893–901. doi: 10.1007/s00299-008-0517-x
- Bates LS, Waldren RP, Teare ID. 1973. Rapid determination of free proline for water-stress studies. Plant and Soil. 39:205–207. doi: 10.1007/BF00018060
- Berenji S, Moot DJ, Moir JL, Ridgway HJ, Rafat A. 2017. Dry matter yield, root traits, and nodule occupancy of lucerne and Caucasian clover when grown in acidic soil with high aluminium concentrations. Plant and Soil. 416:227–241. doi: 10.1007/s11104-017-3203-3
- Dall’Agnol M, Bouton JH, Parrott WA. 1996. Screening methods to develop alfalfa germplasms tolerant of acid aluminum toxic soils. Crop Science. 36:64–70. doi: 10.2135/cropsci1996.0011183X003600010011x
- De la Fuente JM, Ramírez-Rodrı´guez V, Cabrera-Ponce JL, Herrera-Estrella L. 1997. Aluminum tolerance in transgenic plants by alteration of citric acid synthesis. Science. 276:1566–1568. doi: 10.1126/science.276.5318.1566
- Delhaize E, Craig S, Beaton CD, Bennet RJ, Jagadish VC, Randall PJ. 1993. Aluminum tolerance in wheat (Triticum aestivum L.) I. Uptake and distribution of aluminum in root apices. Plant Physiology. 103:685–693. doi: 10.1104/pp.103.3.685
- Dong D, Peng X, Yan X. 2004. Organic acid exudation induced by phosphorus deficiency and/or aluminium toxicity in two contrasting soybean genotypes. Physiologia Plantarum. 122:190–199. doi: 10.1111/j.1399-3054.2004.00373.x
- Du WH, Tian XH, Cao ZZ, Humphries A. 2009. Effects of micronutrients on seed yield and yield components of alfalfa. Journal of Plant Nutrition. 32:809–820. doi: 10.1080/01904160902787909
- Foy CD. 1988. Plant adaptation to acid aluminum toxic soils. Communications in Soil Science and Plant Analysis. 19:959–987. doi: 10.1080/00103628809367988
- Foy CD, Chaney RL, White MC. 1978. The physiology of metal toxicity in plants. Annual Review of Plant Physiology. 29:511–566. doi: 10.1146/annurev.pp.29.060178.002455
- Giannakoula A, Moustakas M, Mylona P, Papadakis I, Yupsanis T. 2008. Aluminum tolerance in maize is correlated with increased levels of mineral nutrients, carbohydrates and proline, and decreased levels of lipid peroxidation and Al accumulation. Journal of Plant Physiology. 165:385–396. doi: 10.1016/j.jplph.2007.01.014
- Giannakoula A, Moustakas M, Syros T, Yupsanis T. 2010. Aluminum stress induces up-regulation of an efficient antioxidant system in the Al-tolerant maize line but not in the Al-sensitive line. Environmental and Experimental Botany. 67:487–494. doi: 10.1016/j.envexpbot.2009.07.010
- Hartel PG, Bouton JH. 1989. Rhizobium meliloti inoculation of alfalfa selected for tolerance to acid, aluminum-rich soils. Plant and Soil. 116:283–285. doi: 10.1007/BF02214560
- Hoagland DR, Arnon DI. 1959. The water culture method for growing plants without soil. California Agricultural Experiment Station. 347:1–32.
- Hong FC, Lu XS, Gao HW. 2009. Alfalfa science. Beijing: China Agriculture Press.
- Ikka T, Ogawa T, Li D, Hiradate S, Morita A. 2013. Effect of aluminum on metabolism of organic acids and chemical forms of aluminum in root tips of Eucalyptus camaldulensis Dehnh. Phytochemistry. 94:142–147. doi: 10.1016/j.phytochem.2013.06.016
- Joslin JD, Henderson GS. 1984. The determination of percentages of living tissue in woody fine root samples using triphenyltetrazolium chloride. Forest Science. 30:965–970.
- Kinraide TB. 1991. Identity of the rhizotoxic aluminum species. Plant and Soil. 134:167–178. doi: 10.1007/BF00010729
- Khan AA, McNeilly T, Collins JC. 2000. Accumulation of amino acids, proline, and carbonhydrates in response to aluminum and manganese stress in maize. Journal of Plant Nutrition. 23:1303–1314. doi: 10.1080/01904160009382101
- Khu D-M, Reyno R, Brummer EC, Bouton JH, Han YH, Monteros MJ. 2010. QTL mapping of aluminum tolerance in Tetraploid alfalfa. In: C. Huyghe, editor. Sustainable Use of genetic diversity in forage and turf breeding. Dordrecht: Springer Science+Business Media B.V; p. 438.
- Kochian LV. 1995. Cellular mechanisms of aluminum toxicity and resistance in plants. Annual Review of Plant Physiology. 46:237–260. doi: 10.1146/annurev.pp.46.060195.001321
- Kochian LV, Hoekenga OA, Pineros MA. 2004. How do crop plants tolerate acid soils?–Mechanisms of aluminum tolerance and phosphorous efficiency. Annual Review of Plant Biololgy. 55:459–493. doi: 10.1146/annurev.arplant.55.031903.141655
- Kochian LV, Pineros MA, Hoekenga OA. 2005. The physiology, genetics and molecular biology of plant aluminum resistance and toxicity. Plant and Soil. 274:175–195. doi: 10.1007/s11104-004-1158-7
- Kurkdjian A, Guern J. 1989. Intracellular pH-measurement and importance in cell-activity. Annual Review of Plant Physiology. 40:271–303. doi: 10.1146/annurev.pp.40.060189.001415
- Langer H, Cea M, Curaqueo G, Borie F. 2009. Influence of aluminum on the growth and organic acid exudation in Alfalfa cultivars grown in nutrient solution. Journal of Plant Nutrition. 32:618–628. doi: 10.1080/01904160802715430
- Larsen PB, Kochian LV, Howell SH. 1997. Al inhibits both shoot development and root growth in ak3, an Al-sensitive Arabidopsis mutant. Plant Physiology. 114:1207–1214. doi: 10.1104/pp.114.4.1207
- Li R, Shi F, Fukuda K, Yang Y. 2010. Effects of salt and alkali stresses on germination, growth, photosynthesis and ion accumulation in alfalfa (Medicago sativa L.). Journal of Soil Science and Plant Nutrition. 56:725–733. doi: 10.1111/j.1747-0765.2010.00506.x
- Ma JF, Hiradate S. 2000. Form of aluminum for uptake and trans-location in buckwheat (Fagopyrum esculentum Moench). Planta. 211:355–360. doi: 10.1007/s004250000292
- Ma JF, Zheng SJ, Matsumoto H. 1997. Specific secretion of citric acid induced by Al stress in Cassia tora L. Plant and Cell Physiology. 38:1019–1025. doi: 10.1093/oxfordjournals.pcp.a029266
- Mariano ED, Keltjens WG. 2003. Evaluating the role of root citrate exudation as a mechanism of aluminium resistance in maize genotypes. Plant and Soil. 256:469–479. doi: 10.1023/A:1026106714644
- Martins N, Gonçalves S, Romano A. 2013. Metabolism and aluminum accumulation in Plantago almogravensis and P. algarbiensis in response to low pH and aluminum stress. Biologia Plantarum. 57:325–331. doi: 10.1007/s10535-012-0271-3
- Matsumoto H. 2000. Cell biology of aluminum toxicity and tolerance in higher plants. International Review of Cytology. 200:1–46. doi: 10.1016/S0074-7696(00)00001-2
- Moir JL, Moot DJ. 2010. Soil pH, exchangeable aluminium and lucerne yield responses to lime in a South Island high country soil. Proceedings of the New Zealand Grassland Association. 72:191–196. doi: 10.33584/jnzg.2010.72.2788
- Moir JL, Jordan P, Moot DJ. 2016. Phosphorus response and optimum pH ranges of twelve pasture legumes grown in an acid upland New Zealand soil under glasshouse conditions. Journal of Soil Science and Plant Nutrition. 16:438–460.
- Onanuga AO, Jiang PA, Adl S. 2012. Effect of effect of phytohormones, phosphorus and potassium on cotton varieties (Gossypium hirsutum) root growth and root activity grown in hydroponic nutrient solution. Journal of Agricultural Science. 4:93–110.
- Pavlovkin J, Pal’ove-Balang P, Kolarovič L, Zelinová V. 2009. Growth and functional responses of different cultivars of Lotus corniculatus to aluminum and low pH stress. Journal of Plant Physiology. 166:1479–1487. doi: 10.1016/j.jplph.2009.03.005
- Pollard A, Wyn Jones RG. 1979. Enzyme activities in concentrated solutions of glycinebetaine and other solutes. Planta. 144:291–298. doi: 10.1007/BF00388772
- Rengel Z. 1996. Uptake of aluminum by plant cells. New Phytologist. 134:389–406. doi: 10.1111/j.1469-8137.1996.tb04356.x
- Reyes-Díaz M, Inostroza-Blancheteau C, Millaleo R, Cruces E, Wulff-Zottele C, Alberdi M, De la Luz Mora M. 2010. Long-term aluminum exposure effects on physiological and biochemical features of highbush Blueberry cultivars. Journal of the American Society for Horticultural Science. 135:212–222. doi: 10.21273/JASHS.135.3.212
- Ryan PR, Ditomaso JM, Kochian LV. 1993. Aluminum toxicity in roots: An investigation of spatial sensitivity and the role of the root cap. Journal of Experimental Botany. 44:437–446. doi: 10.1093/jxb/44.2.437
- Shen R, Ma J, Kyo M, Iwashita T. 2002. Compartmentation of aluminium in leaves of an Al-accumulator, Fagopyrum esculentum Moench. Planta. 215:394–398. doi: 10.1007/s00425-002-0763-z
- Takeda K, Kariuda M, Itoi H. 1985. Blueing of sepal colour of Hydrangea macrophylla. Photocehmistry. 24:2251–2254. doi: 10.1016/S0031-9422(00)83019-8
- Taylor GJ. 1988. Metal ions in biological systems: Aluminum and its role in biology. In: Sigel H., Sigel A, editor. The physiology of aluminum tolerance. New York: Marcel Dekker; p. 165–198.
- Thornton FC, Schaedle M, Raynal DJ. 1986. Effect of aluminum on the growth of sugar maple in solution culture. Canadian Journal of Forest Research. 16:892–896. doi: 10.1139/x86-159
- Yang YH, Chen SM. 2001. Physiological effects of aluminum/calcium rations on aluminum toxicity of Mungbean seedling growth. Journal of Plant Nutrition. 24:585–597. doi: 10.1081/PLN-100104982
- Zheng SJ, Ma JF, Matsumoto H. 1998. Continuous secretion of organic acids is related to aluminium resistance during relatively long term exposure to aluminium stress. Physiologia Plantarum. 103:209–214. doi: 10.1034/j.1399-3054.1998.1030208.x