ABSTRACT
In identifying endophytes for use in pastures in New Zealand there have been two strategies used, either exclusion of ergopeptine and lolitrem alkaloids or, retaining some ergopeptine expression to enhance the stability of natural defences against invertebrate pests. Both have their strengths and weaknesses. It is a matter of balancing these to ensure the end-user has access to a product that satisfies their needs – a persistent pasture with low to nil animal toxicity in terms of animal production and welfare. The range and intensity of ergot alkaloids in grazed pasture on both pasture and ruminants is reviewed, with emphasis on New Zealand. Ergot alkaloids associated with pasture are produced by associations between certain Epichloë endophyte strains and temperate grasses. Ergot alkaloids have been shown to improve persistence of pasture through providing resistance/deterrence to insect pests as well as deterring grazing animals. However, ergovaline is toxic to grazing animals. Some commercially available ryegrass-endophyte associations can produce ergovaline concentrations close to those found in associations between ryegrass and the standard endophyte. It is feasible to eliminate ergot alkaloids from pasture grasses in New Zealand as endophyte strains are commercially available that do not express ergovaline and yet still provide excellent pest resistance.
Introduction
Pasture species from Europe, mainly England, were purposefully brought to New Zealand in the nineteenth century as the early European settlers experimented with various forages in different parts of the country primarily for wool, meat and dairy production (Cockayne Citation1912; Daly Citation1973; Stewart Citation2006). Grass species (family Poaceae) that were trialled included Cock's-foot (Dactylis glomerata), perennial ryegrass (Lolium perenne), fine fescues (Festuca ovina, F. rubra and F. rubra var. commutata) (Star and Brooking Citation2006) and tall fescue (F. arundinacea) which arrived later via Australia (Easton et al. Citation1994).
Fungal endophyte species of the Epichloë genus (family Clavicipitaceae), previously known as Acremonium and Neotyphodium, reside in the seed of several of grass species and were unknowingly introduced to New Zealand when grass seed was imported (Johnson et al. Citation2013). Epichloë spp. are largely seed transmitted, obligate mutualists that may confer advantageous traits to their host and allow the plant to be more resilient to some abiotic stresses, such as drought (Hume et al. Citation1993; Malinowski and Belesky Citation2000; He et al. Citation2013).
Epichloë endophytes are known to produce four major classes of bioactive alkaloids. These are the ergot alkaloids which include ergovaline, the indole diterpenes which include lolitrem B (Gallagher et al. Citation1984; Miles et al. Citation1994) and epoxy janthitrems (Finch et al. Citation2012, Citation2013), pyrrolizidines which include the lolines, and the pyrrolopyrazine metabolites which include peramine (Porter Citation1995; Siegel and Bush Citation1996; Lane et al. Citation2000; Schardl et al. Citation2013; Panaccione et al. Citation2014; Berry et al. Citation2019). Different ergot alkaloids are produced by several fungi in nine genera in the Clavicipitaceae, Aspergillaceae, Arthrodermataceae (Trichocomaceae) families (Gerhards et al. Citation2014), although all have the same early steps before their pathways diverge to produce different end products (Robinson and Panaccione Citation2015). Specifically, in the Clavicipitaceae to which the Epichloë belong, Robinson and Panaccione (Citation2015) noted that diversity is generated by the presence or absence of lysergyl peptide synthetases, which interact to make lysergic acid amides and ergot alkaloids. The diversity of ergot alkaloids has resulted from gene gains, gene losses, and gene sequence changes during the evolutionary development of Epichloë (Young et al. Citation2015).
In New Zealand, the naturally-occurring standard endophyte in ryegrass, Epichloë festucae var. lolii, expresses a wide range of metabolites. Some of these compounds, such as peramine, (Lane et al. Citation2000; Schardl et al. Citation2013) are beneficial to the pastoral system as they protect the host plant from herbivory (Hume et al. Citation2009; Johnson et al. Citation2013). However, other endophyte-expressed metabolites, such as lolitrem B and ergot alkaloids are detrimental (Seman et al. Citation1990) as they are associated with animal diseases including ryegrass staggers, fescue toxicosis (Hemken et al. Citation1984) and heat stress (Prestidge Citation1993; Strickland et al. Citation2011; di Menna et al. Citation2012) as well as losses in animal production (Schmidt and Osborn Citation1993; Browning Citation2000; Waller Citation2009; Strickland et al. Citation2011).
Under New Zealand environmental conditions, the presence of an endophyte is essential due to insect pest pressures, but the detrimental effects of endophytes can be minimised by identifying and selecting endophytes with a favourable chemical profile and inoculating these endophytes into modern grass cultivars (Johnson et al. Citation2013). It is widely accepted that the elimination of lolitrem B, the causative agent of ryegrass staggers, is desirable due to the significant impacts of ryegrass staggers on animal health and welfare (Fletcher and Harvey Citation1981). Although it is also possible to eliminate ergot alkaloids, the causative agent of several animal health and welfare issues including heat stress (Eady et al. Citation2017) from New Zealand pastoral systems, there is not a clear consensus among New Zealand seed companies marketing endophytes as to whether this is the best strategy. Of all the ergot alkaloids found in Epichloë-infected-grass associations, ergovaline is the most abundant and is considered the most potent towards mammals (Rowan et al. Citation1990; Rottinghaus et al. Citation1991; Oliver et al. Citation1994; Bush et al. Citation1997; Klotz Citation2015). This review will critically evaluate the data available to determine whether (a) ergovaline producing endophyte strains can be advantageous to the pastoral industries, and (b) if so, is there a threshold level below which it is safe when in pastures fed to ruminants. The focus will be on the use of Epichloë endophytes in perennial ryegrass and the data collected in New Zealand studies. However, endophyte-infected tall fescue (F. arundinacea) has been much more extensively studied especially in the USA as ergot alkaloids have long been recognised to severely affect animal health. This research will therefore also be incorporated into this review as the experience of overseas groups will aid our interpretation of New Zealand results.
Ergovaline in New Zealand pastures
Ergot alkaloids
Ergot alkaloids are a group of nitrogenous compounds derived from L-tryptophan. These mycotoxins are mainly produced by Claviceps species (over 80 compounds) but also by other fungi and higher plants (Schiff Citation2006). The ergot alkaloid pathway () associated with Epichloë endophytes is well researched and understood (Porter et al. Citation1981; Garner et al. Citation1993; Schardl et al. Citation2006, Citation2009; Guerre Citation2015; Young et al. Citation2015). Ergot alkaloids can be divided into four groups based on their chemical structure: clavines (e.g. chanoclavine, agroclavine), lysergic acid, lysergic acid amides (e.g. ergonovine, ergine) and ergopeptines (e.g. ergovaline, ergotamine, ergocornine, ergocristine, ergosine, ergocryptine) (Schardl et al. Citation2006; Gerhards et al. Citation2014).
Figure 1. Biosynthesis of ergot alkaloids associated with endophyte–plant symbioses. Double arrows indicate one or more omitted intermediates. Relevant enzymes associated with catalysis are indicated. At the branch point, combinations of peptide synthetases LpsA, LpsB, and LpsC are required to produce ergot alkaloids or lysergic acid amides (Lorenz et al. Citation2009; Ortel & Keller Citation2009). For the ergopeptines, R1, R2, and R3 refer to the side-groups of the three amino acids linked to lysergic acid to create the various final products.
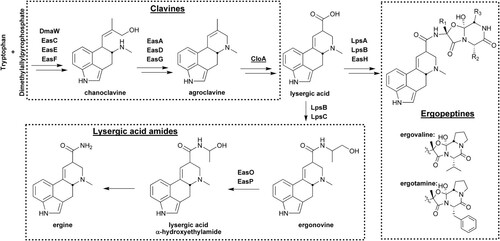
Ergovaline is the most prevalent and toxic ergot alkaloid in endophyte-infected grasses and is therefore the most widely studied and understood (Lyons et al. Citation1986; Schardl et al. Citation2009).
Ergovaline has three stereoisomers (ergovaline, ergovalinine and aci-ergovaline) and two derivatives (dehydroergovaline and dehydroergovalinine) (Lane Citation1999). However, ergovaline is not the only ergot alkaloid produced by Epichloë endophytes in perennial ryegrass and tall fescue (Lane et al. Citation1999; Duringer et al. Citation2007), and there is evidence that some of these other compounds may contribute to the animal toxicosis often attributed to ergovaline (Gadberry et al. Citation2003; Duringer et al. Citation2007). Other ergot alkaloids, including ergotamine, are found at very low levels in Epichloë-infected ryegrass using HPLC analysis (Rowan et al. Citation1990) and also when using mass spectrometry (Rowan and Shaw Citation1987), while low concentrations of ergosine, ergonine, ergocryptine, ergocrystine and ergocornine have also been detected (Lyons et al. Citation1986; Schardl et al. Citation2006).
Lysergic acid amides, including ergine and ergonovine, are produced late in the ergovaline pathway (Fleetwood et al. Citation2007) and ergine has been detected in grasses infected with some Epichloë endophytes (Lane et al. Citation1999) (). Lysergic acid amides have also been found in Epichloë-infected sleepygrass (Stipa robusta) in the southwestern USA (Petroski et al. Citation1992), and in drunken horse grass (Achnatherum inebrians) from China at levels of 2900 μg/g DM (Miles et al. Citation1996). Lysergic acid amides can induce stupor in grazing animals (Lane Citation1999).
Clavines such as chanoclavine have also been identified in Epichloë-infected ryegrass and tall fescue (Porter et al. Citation1981; Lane et al. Citation1999; Fleetwood Citation2007) (). Clavines are derived from dimethylallyl tryptophan (DMAT), an early product in the ergovaline pathway (Panaccione et al. Citation2001). Chanoclavine is devoid of any ergot-like animal toxicity (Berde and Schild Citation1978) and has been shown to be non-toxic to mice even at high concentrations (Finch et al. Citation2019). Since ergot-alkaloids of the clavine class have been shown to deter the feeding of fall armyworm (Spodoptera frugiperda) (Clay and Cheplick Citation1989) they are considered worthy of further investigation for biological activity against insects (Lane et al. Citation1999; Schardl et al. Citation2009).
Measurement of ergovaline–sampling and methods of analysis
The methods of sampling herbage for ergovaline analysis are of critical importance because of the uneven distribution of ergovaline within and between plants. This is further described in the next section, but in summary ergovaline is most highly concentrated in the crown, lower part of the leaf tiller (pseudostem) and in flower heads (). Therefore, sampling height is critical, along with the stage of reproductive maturity (Rottinghaus et al. Citation1991; Davies et al. Citation1993; Blackwell and Keogh Citation1999). Variation between individual tillers can exceed the level of variation observed between individual plants (Mace et al. Citation2014), therefore sampling needs to account for this variation also.
Table 1. Ergovaline concentrations (μg/g DW) in different plant parts of ryegrass: (a) standard endophyte grown in the field in the Manawatu, and harvested in late spring (November 1993) (Study 1 from Lane et al. Citation1997a); (b) cv. ‘Grasslands Nui’ infected with strain AR42 (Lp19), noted as a low ergovaline expressing strain (Study 2 from Spiering et al. Citation2002); (c) strain 187BB showing extremely high ergovaline levels (Study 3 from Lane et al. Citation1997c); (d) standard endophyte from a 3 to 4-year-old pasture in Northland harvested from mid-spring to mid-autumn (Study 4 from Blackwell and Keogh Citation1999); (e) total herbage analysis of ‘Grasslands Nui’ with standard endophyte grown in Southland and harvested throughout the year (Study 5 from Rowan et al. Citation1990).
A further complication is determining not just the concentration in the plant as a whole, but more particularly the concentration in the parts of the plant likely to be consumed by a grazing ruminant. This in itself can be variable and is determined by a combination of stocking rate and the amount of feed available. Therefore, sampling to a standard height at or above ground level is important for developing a time series to follow changes in ergovaline concentrations but may not necessarily reflect the amount consumed by a grazing animal. Unfortunately, some studies measuring ergovaline concentrations have not recorded height of harvest above ground.
After sampling it is important to have samples frozen and dried as soon as possible and subsequently stored at low temperatures (≤ −20°C) or else ergovaline concentrations will decline over the first 24-hours post-harvest (Lea et al. Citation2014). In addition, UV light and heat can reduce ergovaline concentrations within hours (Kaur et al. Citation2014; Lea et al. Citation2014). In the appropriate low temperature conditions (e.g. −20°C) samples may be stored until analysed with little deterioration of alkaloids (Lea et al. Citation2014). Solvent, particle size, and time used for extraction also has a significant impact on efficiency of extraction (Ji et al. Citation2014).
In ryegrass conserved for silage or hay, ergovaline concentrations decline the longer herbage is field dried or stored (Fletcher Citation2005; Hume et al. Citation2007a). Consistent with this, a two-year study in the USA showed that ergovaline concentrations in stockpiled herbage of Kentucky 31 tall fescue infected with standard endophyte decreased by approximately 85% between December and March each year (Kallenbach et al. Citation2003).
Ergopeptine alkaloids were first identified in perennial ryegrass grown in New Zealand by Rowan and Shaw (Citation1987). Procedures for ergot alkaloid analysis commonly comprise liquid chromatography with tandem mass spectrometry (LC-MS/MS) and liquid chromatography with fluorescence detection (LC-FLD) (Shelby and Kelley Citation1992; Garner et al. Citation1993; Ma et al. Citation1993; Porter Citation1995; Rasmussen et al. Citation2012; Crews Citation2015). It is acknowledged that while ergovaline is the biologically active form of the ergopeptine compounds, isomerisation with its 8-epimer, ergovalinine, can take place variably in the plant, in stored samples, and during analysis (Lane Citation1999). Ergovaline concentrations in perennial ryegrass herbage grown and analysed in New Zealand are often between 0.3 and 1 μg/g DM (Easton et al. Citation1996), which is about 60% of the concentrations found in USA tall fescue pastures (Lyons et al. Citation1986; Garner et al. Citation1993). In New Zealand, the accepted methodology has been to measure ergovaline as the sum of both isomers (e.g. Easton et al. Citation1996) whereas this is not the case in the USA. Therefore, Lane (Citation1999) postulated that the ergovaline values reported from New Zealand studies may be 30%–40% higher than comparative measurements made in the USA. This fact should be considered when comparing ergovaline concentrations reported from studies originating in the USA and New Zealand.
Urinary ergot alkaloid excretion (creatinine) has been used, in preference to serum prolactin levels, for health assessment of steers grazing endophytic pastures, where a significant negative relationship (r2 = 0.86) between alkaloid excretion and average daily weight gain has been established (Hill et al. Citation2000). ELISA technology has also been developed to measure lysergol compounds in animal urine (Garthwaite et al. Citation1994) and this includes all the ergot alkaloids, so this assay gives a linear response to the amount of ergovaline ingested.
Distribution of ergovaline in the plant
Field grown perennial ryegrass diploids cv. ‘Grasslands Marsden’ and ‘Grasslands Samson’, along with their respective tetraploids, cv. ‘Grasslands Greenstone’ and the selection ‘4N’, together with a third diploid, cv. ‘Yatsyn’ were sampled in April 1998 and February 1999. This showed ergovaline concentrations of below 0.3 μg/g DM in leaf lamina, but in pseudostems levels varied from between 0.6 μg/g DM (‘Greenstone’) and 1.6 μg/g DM (‘Yatsyn’) in 1998 and between 1.7 μg/g DM (‘Samson’) and 2.8 μg/g DM (4N) in 1999 (Popay et al. Citation2003). ‘Marsden’ and ‘Greenstone’ are long-rotation hybrid ryegrasses (L. boucheanum syn. L. hybridum) while ‘Samson’, ‘4N’ and ‘Yatsyn’ are perennial ryegrasses (L. perenne). All were infected with standard endophyte except ‘Greenstone’ which was infected with a selected endophyte which produces ergovaline and peramine but not lolitrem B.
Ergovaline was monitored in endophyte-infected perennial ryegrass ‘Samson’ over a three-year period in southern France which showed that concentrations were strongly influenced by the stage of maturity of the plant with maximum concentrations measured at the fully ripe stage (Repussard et al. Citation2014). In this study ergovaline levels were similar in the tiller base and leaf blade ranging from 0.014 to 0.362 μg/g DM in the base of the plant, and 0.008–0.366 μg/g DM in the leaf blade, but were highest in the seed head/ flowering stem with levels ranging from 0.042 to 6.241 μg/g DM. In New Zealand, ergovaline concentrations have been shown to be not only highest in the flowering stem and seed-head, but also high in the basal sheath of the plant (). Heat stress symptoms are more severe in lambs forced to graze lower into the sward (Bluett et al. Citation2001a), due to the presence of higher levels of ergovaline, particularly in this case at ambient temperatures of 26°C–28°C. Davies et al. (Citation1993) was able to show in ryegrass containing standard endophyte grown in Canterbury that ergovaline levels in the lower pseudostem were 1.4 and 1.5 μg/g DM (in March and April, respectively) compared with 0.3 and 0.2 μg/g DM in the first leaf. Others have also found ergovaline higher in the basal regions of both the tiller and sheath than in the lamina of perennial ryegrass and tall fescue (Lyons et al. Citation1986; Rottinghaus et al. Citation1991; Azevedo et al. Citation1993; Keogh et al. Citation1996; Lane et al. Citation1997a; Watson et al. Citation1999; Spiering et al. Citation2002), and almost absent in dead leaf material (Lowe et al. Citation2008). Ergovaline also occurs at biologically significant levels (over 1 μg/g DM when plants are fertilised with N, P and K) in the roots of tall fescue (Azevedo et al. Citation1993).
Level of ergovaline expression in New Zealand pastures
Ergovaline expression is influenced by plant host genetics, Epichloë strain genetics, the interaction between host and Epichloë strain genetics, and the environment in which the plant is being grown.
Plant species effects on ergovaline expression
The strain of Epichloë endophyte most commonly credited with causing ergovaline expression in perennial ryegrass has been referred to by a number of different terms including ‘standard’, ‘wild-type’, ‘toxic’ and ‘common’ endophyte. In this paper it will be referred to as standard endophyte. This endophyte was first identified in the late eighteenth Century (Persoon Citation1798) and further characterised in the 1930s (Sampson Citation1933). It was probably introduced to New Zealand by European colonists in the nineteenth century. However, it was not until the 1980s that the alkaloid expression of the standard endophyte was elucidated to show that it produced at least three alkaloids–peramine, lolitrem B and ergovaline (Easton et al. Citation2001). Tall fescue with a toxic endophyte strain occurs along roadsides in New Zealand (Cunningham Citation1948; Wilson Citation1975).
Although ergovaline is found in both Epichloë-infected perennial ryegrass and tall fescue (TePaske et al. Citation1993; Gadberry et al. Citation2003) signs of ergovaline toxicity are rarely reported in livestock fed endophyte-infected ryegrass (Easton et al. Citation2001). This could be due to the co-occurrence of lolitrem B which may mask the impact of ergot-alkaloids. It has been suggested in the literature that ‘the toxic threshold of ergovaline was lower in endophyte-infected ryegrass than in endophyte-infected tall fescue’ (Guerre Citation2015). However, we believe that the original data was misinterpreted. In these studies, lactating ewes fed endophyte-infected ryegrass hay containing 851 µg/kg DM of ergovaline for 28 days suffered no ill effects whereas, under the same experimental conditions, lactating ewes fed endophyte-infected tall fescue hay containing 497 µg/kg DM ergovaline showed adverse effects. This therefore suggests that the toxic threshold of ergovaline is actually lower in endophyte-infected tall fescue rather than endophyte-infected ryegrass (Gadberry et al. Citation2003; Zbib et al. Citation2014b, Citation2015).
In tall fescue forage, ergovaline can make up 80% of the ergot alkaloids (Belesky et al. Citation1988) and in seed 50% (Guerre Citation2015) although this can be variable and much lower percentages have been reported. (Gadberry et al. Citation2003; Dillard et al. Citation2019). In perennial ryegrass herbage ergovaline may make up 70% of the ergot alkaloids (W.J. Mace unpublished data) but again this can be variable. It has been proposed that the array of ergot alkaloids (), in particular ergopeptides found in Epichloë infected ryegrass, maybe less varied than in infected tall fescue, although the biological significance of non-peptide ergot alkaloids, such as ergine and chanoclavine-I, cannot be dismissed (Lane et al. Citation1999). In this study they found that dehydroergovaline occurred in immature inflorescences of tall fescue at about 41% to 85% of the concentrations of ergovaline, but was not present in ryegrass infected with endophyte. Shelby et al. (Citation1997) reported that for tall fescue, in addition to ergovaline, and the known ergopeptides, ergosine and ergonine (as minor components), two unusual modified ergopeptides, dehydroergovaline and aci-ergovaline were present (). They suggested that dehydroergovaline and aci-ergovaline may be derived from the modification of ergovaline by plant enzymes rather than being direct products of the endophyte itself. These plant enzymes may be lacking in perennial ryegrass as dehydroergovaline has not been detected in this association leading to the suggestion that the difference in toxicity between Epichloë-infected ryegrass and tall fescue may be due, at least in part, to differences in the chemistry of the associations.
Table 2. Mean total (µg/g DM) and proportional ergot alkaloids in three plant parts of tall fescue measured using HPLC analysis (derived from Shelby et al Citation1997).
Ergine, a lysergic acid amide, has been reported in Epichloë-infected tall fescue seed () at similar concentrations to that of ergovaline (TePaske et al. Citation1993; Shelby et al. Citation1997; Gadberry et al. Citation2003). In contrast, ergine has been tentatively identified in Epichloë-infected perennial ryegrass seed but at only 7% of the concentration of ergovaline (TePaske et al. Citation1993; Gadberry et al. Citation2003). Ergine has also been identified in New Zealand Epichloë-infected perennial ryegrass leaf material at on average about the same levels as that of ergovaline, ranging from 0.14 to 12.65 µg/kg DM across 25 genotypes infected with the same endophyte strain (Mace et al. Citation2014). Other lysergic acid amides, if they occur at all, are at very low concentrations in perennial ryegrass herbage (Ball et al. Citation1997).
Clavine alkaloids (), including chanoclavine-I, have been identified in E. coenophiala in culture (Porter et al. Citation1981), and subsequently in planta in tall fescue (Lyons et al. Citation1986) and ryegrass (Mace et al. Citation2014), and in seed of endophyte-infected tall fescue and perennial ryegrass of New Zealand origin (Lane et al. Citation1999).
Epichloë genetic strain effects on ergovaline expression
Alkaloids can be independently regulated and are controlled by both plant and endophyte genotype (Roylance et al. Citation1994). There is a tendency for asexual, seed-transmitted Epichloë endophyte strains infected into ryegrass to express higher levels (between 2.3 and 5.4 μg/g DM) of ergovaline compared with horizontally transmitted sexual endophytes (e.g. Epichloë typhina) that can express zero ergovaline (Siegel et al. Citation1990). A strong positive relationship (r2 = 0.73) between levels of lolitrem B and ergovaline has been observed across 459 ryegrass straw samples in the USA (Hovermale and Craig Citation2001). This study indicated that when 2 μg/g DM of lolitrem B is reached, approximately 0.175 μg/g DM ergovaline is present suggesting that the first clinical sign observed when animals ingest endophyte-positive perennial ryegrass will be staggers. This of course begs the question of what happens when ryegrass containing a lolitrem B free but ergovaline producing strain is ingested?
Although the standard endophyte in perennial ryegrass is characterised by the presence of ergovaline, lolitrem B and peramine, an early screen of 23 Epichloë strains from different populations of perennial ryegrass, predominately from Europe (Christensen et al. Citation1993), found that 9% expressed lolitrem B and peramine but no ergovaline, 26% had peramine and ergovaline but no lolitrem B, 48% had only lolitrem B and ergovaline, and 17% expressed none of these three alkaloids. This allowed the characterisation (in terms of genetic diversity and chemical profiles) of strains that express only ergovaline and not lolitrem B such as AR6 (also known as 187BB) (Easton et al. Citation2001; Fletcher Citation2012), and AR5 (originally marketed as ‘Endosafe’ (Thom et al. Citation2012) but now marketed as ‘Endo5’) (Popay and Hume Citation2011). Other characterised strains which express ergovaline, such as NEA2, have been commercialised (Stewart et al. Citation2014). While this endophyte brand is a mix of NEA2 and NEA6 it is promoted as expressing only low levels of lolitrem B and ergovaline they have the potential to produce toxic concentrations under adverse environmental conditions and have reached 0.9 μg/g DM of ergovaline in autumn (Fletcher Citation2012; Fletcher et al. Citation2017).
Some of these characterised Epichloë endophyte strains associated with ryegrass, such as AR5, AR6 and some NEA brand endophytes, that either have been or are commercially available, can produce ergovaline concentrations similar to those found in ryegrass with its standard endophyte and depending on host genetics can reach 1.1 μg/g DM (van Zijll de Jong et al. Citation2008; Logan et al. Citation2015). This can result in heat stress responses similar to that of the standard endophyte (Fletcher et al. Citation2017).
Interactions between ryegrass host and Epichloë genetics on ergovaline expression
Plant host and endophyte strain, and interactions between these factors, can modify the quantity and type of alkaloids produced (Agee and Hill Citation1994; Latch Citation1994; Ball et al. Citation1995; Adcock et al. Citation1997; Easton et al. Citation2002). This variation can occur at both genotype and cultivar level. A 10-fold range of ergovaline concentration was observed amongst 19 genotypes from the same cultivar of ryegrass infected with the same endophyte (Latch Citation1994). Latch in the same study also noted that when endophyte strain 187BB (also known as AR6) was inoculated into the diploid cultivar ‘Grasslands Pacific’, the expression of ergovaline was much higher than when inoculated into ‘Grasslands Greenstone’, an autotetraploid hybrid ryegrass cultivar. This was most apparent during flowering under warm ambient conditions, or with regrowth soon after the harvest of seed crops (Tapper and Latch Citation1999). As a result, cv. ‘Grasslands Pacific’ Endosafe was withdrawn from the market, but cv. ‘Grasslands Greenstone’ continued to be marketed (Fletcher Citation2012). Davies et al. (Citation1993) also noted large variations in ergovaline content with strain 187BB inoculated into ‘Nui’, ‘Ruanui’ and ‘Greenstone’. In ‘Greenstone’, ergovaline expression was only 1.1 and 1.4 μg/g DM (in March and April, respectively) but in ‘Nui’ and ‘Ruanui’ the average ergovaline expression level was 4.8 and 3.9 μg/g DM, respectively.
As has been shown for tall fescue (Hill et al. Citation1991; Hill Citation1993; Agee and Hill Citation1994; Roylance et al. Citation1994; Adcock et al. Citation1997; Hiatt and Hill Citation1997), the host ryegrass genome has significant control over the concentrations of ergopeptides produced by the endophyte in herbage (Easton et al. Citation2002). This study also showed that parent-progeny correlation coefficients were high and narrow-sense heritability was estimated as 0.70 for ergovaline. It was also estimated that 41% of the genetically controlled variation in ergovaline concentrations in ryegrass was a function of mycelial mass.
Therefore, it is not unexpected that the level of ergovaline can be low in one plant and high in another, such that when one strain of endophyte was inoculated into 19 endophyte-free Nui seedlings the ergovaline expression ranged from 3 to 27 μg/g DM among plants (Tapper and Latch Citation1999). Similarly, it has been demonstrated that by phenotypic selection, the level of ergovaline in tall fescue breeding lines infected with standard endophyte E. coenophiala can be reduced in progeny (Agee and Hill Citation1994). The variation in ergovaline between ryegrass plants led both Rowan et al. (Citation1990) and Easton et al. (Citation2002) to suggest that it may be possible to select endophyte strains for high or low levels of ergovaline. This has been the strategy behind the release of some of the NEA branded endophytes (Eady et al. Citation2017). While this may be partly correct, it is now accepted that the level of ergovaline expression can be driven by the host plant genetics (Easton et al. Citation2002) and also by environment (e.g. Barker et al. Citation1993; Repussard et al. Citation2014; Dillard et al. Citation2019). Selection for ergovaline levels in tall fescue is also highly heritable (Adcock et al. Citation1997; Hill et al. Citation2002). In contrast to other studies, ploidy and ryegrass species had no effect on ergovaline concentrations in the lamina or pseudostem in a field study in Taranaki (Popay et al. Citation2003).
Environmental effects on ergovaline expression
Several biotic and abiotic variables including season, can impact on the level of ergovaline expression. The ergovaline concentration of an endophytic ryegrass pasture in southern France varied considerably from 0.526 to 2.322 μg/g DM over three years suggesting that abiotic factors play a key role in the expression of ergovaline levels in endophyte-infected perennial ryegrass (Repussard et al. Citation2014). The highest concentrations of ergovaline were observed in spring during ryegrass anthesis (Easton et al. Citation1996), under high summer temperatures (McCulley et al. Citation2014; Fuchs et al. Citation2017) with a further peak in the autumn (Fletcher et al. Citation1994; Easton et al. Citation1996; Fletcher et al. Citation2001; Bluett et al. Citation2005; Moate et al. Citation2012; Thom et al. Citation2013), particularly after defoliation. Blackwell and Keogh (Citation1999) in an on-farm study in Northland found that ergovaline concentrations were higher in summer and in autumn than in spring. A similar trend was shown over a two-year period in the Manawatu () and in Australia (Woodburn et al. Citation1993) but in Southland, the highest levels of ergovaline were noted in late spring (Eerens et al. Citation1998).
Figure 2. Mean (± SEM) ergovaline concentrations in ryegrass leaf blade and leaf sheath components of the standard endophyte infected pasture, grown in the Manawatu region, during 1997 and 1998 (taken from Watson et al. Citation1999). Average maximum daily temperature for summer (December to February) in each year was 22.3°C and 22.8°C, respectively; for autumn (March to May) 18.2°C and 19.9°C; for winter (June to August) 12.7°C and 13.8°C; and for spring (September to November) 16°C and 17.3°C. Data provided by the Meteorological Service of New Zealand Ltd..
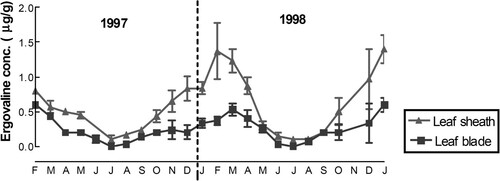
However, even within a season ergovaline levels in pastures can vary greatly, and unpredictably. Easton et al. (Citation1996) sampled 39 sites on 18 farms in Northland between January and April 1995. While the mean did not vary greatly throughout the season, the percentage of pastures with ergovaline concentrations above 1 μg/g DM was 0% in March, 30% in February, and between 15% and 20% in January and April. They concluded that in seasons that combine adequate soil moisture with warm temperatures and high levels of mineralised (or fertiliser) nitrogen (Lyons et al. Citation1986), the biological activity of the endophyte remained high and this was reflected in the high ergovaline concentrations observed. Hume et al. (Citation2016) reviewed literature to date on changes in endophyte and alkaloid concentrations in response to experimental warming and CO2 increase, and concluded that warming resulted in increased endophyte prevalence and concomitant increase in alkaloid concentration. Plants suffering from water deficit have elevated ergovaline levels (Lane et al. Citation1997b), which in some studies have resulted in a doubling of the ergovaline concentration (Barker et al. Citation1993).
Impacts of N supply on ergovaline levels have been variable. Ergovaline concentrations can be elevated by N fertilisation (Lyons et al. Citation1986; Rottinghaus et al. Citation1991; Lane et al. Citation1997b; Reed et al. Citation2011; Repussard et al. Citation2014), ambient temperature and drought (Arechavaleta et al. Citation1992; Barker et al. Citation1993; Eerens Citation1996). Fletcher et al. (Citation2001) showed that 40% to 50% of the variation in ergovaline levels in ryegrass could be explained by soil temperature (at 1 m depth). Hunt et al. (Citation2005) showed a negative correlation between the production of ergovaline and N fertilisation at ambient CO2, but a positive effect at elevated CO2, when coupled with high N. They proposed that this seemingly peculiar result could be related to the requirement for carbohydrates, as well as N, in alkaloid biosynthesis. However, Belesky et al. (Citation1988) and Rasmussen et al. (Citation2007) showed no N fertilisation effect on ergovaline concentrations while Azevedo et al. (Citation1993) showed elevated ergovaline levels in leaf blade, stem, crown and roots of tall fescue when fertilised with N, P and K.
Even in Ireland with its mild climate it has been noted that an awareness is required of the potential adverse effect of ergot alkaloids from perennial ryegrass infected with endophytic fungi on animal health and performance (Canty et al. Citation2014).
Ergovaline effects on pest resistance
Ergovaline is known to deter adult Argentine stem weevil (ASW) (Listronotus bonariensis) (Popay et al. Citation1990; Popay and Wyatt Citation1995). However, the benefits of this bioactivity are reduced by the fact that it is the larvae of ASW that causes greater damage to pasture (Kelsey Citation1958) and Popay and Lane in unpublished work (quoted in Popay and Ball Citation1998) found that ergovaline had no effect on ASW larval growth or development. It may, however, have a role in reducing oviposition in the pseudostem (A.J. Popay unpublished data). Some other ergot alkaloids can affect ASW with ergocryptine deterring feeding by weevil adults and larvae, and ergotamine affecting adult feeding (Dymock et al. Citation1989).
Ergovaline and other ergot alkaloids (ergovalinine, ergotamine, α-ergosine, and α-ergocryptine) are described as having strong anti-feeding activity to adult African black beetle (Heteronychus arator) (Ball et al. Citation1997; Wheatley et al. Citation2003; Hume et al. Citation2007b) compared with the simpler lysergic acid amides and clavines (Prestidge and Ball Citation1993; Ball et al. Citation1997). Ball et al. (Citation1997) established that the ergopeptine concentration threshold required for significant black beetle feeding deterrence lay between 1.0 and 5.0 μg/g DM, which is within the ergovaline concentration range encountered in planta at certain times of the year (Rowan et al. Citation1990; Davies et al. Citation1993; Fletcher et al. Citation1994; Ball et al. Citation1995; Easton et al. Citation1996). Interestingly, when Fleetwood et al. (Citation2007) deleted an endophyte gene responsible for the synthesis of ergovaline, resulting in the elimination of ergovaline and lysergic acid amides, and the accumulation of lysergic acid and other clavine intermediates, feeding deterrence to African black beetle was still observed with plants infected with the mutant strain. This work therefore suggests that the clavine intermediates of ergot alkaloids may be responsible for black beetle deterrence. Contrary to this however, clavines and simple lysergic acid derivatives were determined using a choice test as having no anti-feeding activity towards adult African black beetle, while lysergic acid amide and ergonovine had only weak anti-feeding activity (Ball et al. Citation1997). In this study it was the ergopeptine alkaloids ergotamine, ergovaline, ergosine and ergocryptine that provided the reduced feeding at 5 μg/g DM. Deterrence of the adult beetle by ergovaline is thought to reduce oviposition in the field leading to reductions in highly damaging root-feeding larval populations (Prestidge et al. Citation1994) although this tends to only delay population build-up. Siegel et al. (Citation1989) commented that ergovaline has not been measured at biologically significant levels in root tissues, but Lane et al. (Citation1997a) did measure ergovaline in root tissues () although there was no measure of effects on African black beetle larval feeding in that study. Additionally, the known potent bioactive compound, loline which does get into the root system, has been shown to have a variable effect on black beetle feeding ranging from being not significant (Popay and Ball Citation1998) to a significant effect on larval feeding (Barker et al. Citation2015).
Ergovaline produced both by the standard and AR6 endophytes may be a possible factor in reducing root aphid numbers (Aploneura lentisci) in perennial ryegrass (Popay and Gerard Citation2007). Patterson et al. (Citation1991) showed that wet weight concentrations of 5 and 10 µg/g of ergotamine deterred feeding by larvae of the Japanese beetle (Popillia japonica) but equivalent dry weight concentrations would be unrealistically high. In addition, ergovaline appears to have neither a deterrent nor toxic effect on either grass grub (Costelytra zealandica) or porina (Wiseana spp.), both native insects that can be destructive to ryegrass pastures in New Zealand (Popay and Ball Citation1998).
Despite ergovaline affecting Pratylenchus spp. in in vitro motility tests, the elimination of certain complex ergot alkaloids (ergovaline and lysergic acid amides) in one gene knockout strain, or complete elimination of ergot alkaloids in another, did not affect the ability of the endophyte to suppress populations of nematodes in in planta tests (Panaccione et al. Citation2006b).
Ergovaline effects on animals
Known effects of ergovaline on animals
In general terms there are a number of important effects of ergovaline in consumed feed on animal physiology and metabolism as reviewed by Klotz (Citation2015) and Klotz and Nicol (Citation2016). Klotz (Citation2015) concluded that the impacts of ergot alkaloids on livestock are not caused by the sole action of a single toxin but rather the combined impact and synergistic action of multiple ergot alkaloids. Flieger et al. (Citation2019) further postulated that ergochrome compounds, such as secalonic acids A-C may contribute to the overall toxicity caused by ergot alkaloids.
Ergot alkaloids in feed can impact on feed intake, body temperature and respiration rate, general physiology, and overall animal production.
Feed deterrent effect
Ergovaline has a deterrent effect on grazing animals (Pennell and Rolston Citation2003). This has been demonstrated in cattle where a study showed animals grazing endophyte-infected tall fescue had lower feed intakes than animals grazing endophyte-free tall fescue (Stuedemann et al. Citation1989; Seman et al. Citation1990). Similarly, another study in the USA showed that cattle fed endophyte-infected tall fescue hay, and cattle fed endophyte-free hay supplemented with pure ergotamine, had lower feed intakes than cattle fed endophyte-free tall fescue hay both at thermoneutral and elevated temperatures (Osborn et al. Citation1992).
This feed deterrent effect has also been observed in rats where animals fed endophyte-infected tall fescue seed had a decreased food intake under both thermoneutral and heat stress environments (Roberts et al. Citation2002). Furthermore, Filipov et al. (Citation1998) showed that endophyte-infected tall fescue reduced the food consumption and weight gain of rabbits in comparison to nil endophyte controls. Later work by Panaccione et al. (Citation2006a) using endophyte-infected perennial ryegrass with altered ergot alkaloid profiles showed that ergovaline was the compound primarily responsible for appetite suppression in rabbits. This deterrent trait has been the basis of using ergovaline expressing endophytes in turf ryegrass and fescues to deter small birds, rabbits and rodents at airports and recreational areas to avoid bird strike and situations where small animals are regarded as a nuisance (Pennell et al. Citation2010, Citation2017a, Citation2017b; Finch et al. Citation2016).
Elevated body temperature and respiration rates
Elevation of rectal temperature and respiration rate has been observed in cattle fed endophyte-infected and ergotamine diets at high temperatures although not at under thermoneutral conditions (Osborn et al. Citation1992). A similar effect has been observed in sheep where reduced food intakes of perennial ryegrass infected with standard endophyte have been associated with lower animal performance (Edwards et al. Citation1993). However, separately from effects of feed intake or environmental temperature, ergot alkaloids from fescue seed can affect the cardiovascular system of steers (Oliver et al. Citation2000; Eisemann et al. Citation2014). To counteract overheating in warm and humid conditions, the respiration rate and salivation of the animal increases in an attempt to cool itself–these are recognised as heat stress symptoms in these animals (Brookbanks et al. Citation1985; Easton et al. Citation1996).
Effects on physiological systems
Schiff (Citation2006), Strickland et al. (Citation2011, Citation2012) and Dellafiora et al. (Citation2015) provide thorough reviews concerning the mechanisms (toxicodynamics) by which the ergot alkaloids may affect the physiological systems of animals (and humans), resulting in health and/or production deficiencies. Ergovaline has a significant effect on biogenic amines that occur naturally through the enzymatic removal of a carboxyl group (-COOH) from an amino acid. Many amines (e.g. dopamine, histamine, and serotonin) have powerful physiological effects on ruminants/mammals through their action as neurotransmitters (Purves et al. Citation2012). Ergot alkaloids, including ergovaline, bind these biogenic amines and stop them functioning, and as a result, can cause persistent vasoconstriction (Rhodes et al. Citation1991; Dyer Citation1993; Larson et al. Citation1995; Strickland et al. Citation1996; Larson et al. Citation1999; Oliver Citation2005; Aiken and Flythe Citation2014), decreased heart rate and increased blood pressure (Aiken et al. Citation2007, Citation2009). This explains why the animal’s ability to regulate their core body temperature is weakened (Hemken et al. Citation1981; Spiers et al. Citation2005; Eisemann et al. Citation2014) and reduces blood flow to extremities, such as tails, ears and hooves (Solomons et al. Citation1989; Rhodes et al. Citation1991; McCollough et al. Citation1994; Oliver Citation1997) which can result in gangrene and death (Goodman Citation1952; Jensen et al. Citation1956; Maag and Tobiska Citation1956; Garner and Cornell Citation1978; Bush et al. Citation1979; Bacon et al. Citation1986; Thompson and Stuedemann Citation1993). Steers fed ergot alkaloid containing tall fescue had decreased serum concentrations of cholesterol, globulin (increased albumin/ globulin ratio), prolactin, total protein, and copper (Oliver et al. Citation2000).
A series of studies by Klotz with tall fescue examined the potency and efficacy of selected ergot alkaloids to induce constriction of the bovine lateral saphenous vein in vitro (Klotz et al. Citation2006, Citation2007, Citation2008, Citation2010). These studies showed that ergovaline is approximately 1000 times more potent (concentrations at which constriction occurs) in causing lateral saphenous veins to constrict in comparison to lysergic acid (Klotz et al. Citation2006, Citation2007, Citation2008). Ergot alkaloids as agonists of dopamine can reduce serum prolactin levels (Fletcher and Barrell Citation1984; Porter et al. Citation1985; Strickland et al. Citation1994; Fletcher et al. Citation1996, Citation1997; Oliver Citation1997) and in doing so can affect temperature regulation (Nicoll and Bryant Citation1972; Faichney and Barry Citation1986; Speirs et al. Citation1995; Fletcher et al. Citation1997) resulting in heat stress (hyperthermia) in warm humid conditions or hypothermia in sub-zero conditions (Craig et al. Citation2015). Reduced prolactin may also impair immune function (Rever Citation1993; Dawe et al. Citation1997), reduce milk yield and lower conception rates and fertility (Kramer et al. Citation1999; Browning Citation2000). Thompson et al. (Citation1993) showed that ergovaline may be responsible for serum prolactin decline in animals grazing endophyte-infected tall fescue. It has been noted that the threshold level for suppression of prolactin by ergovaline can be less than 0.5 μg/g DM (Debessi et al. Citation1993; Fletcher and Easton Citation1997). However, unlike Strickland et al. (Citation1994), Piper et al. (Citation1997) showed that feeding either ergovaline (at 2.5 μg /g DM) or ergine (at 0.5 μg /g DM) to rats had no impact on serum prolactin levels. They concluded that alkaloids other than ergovaline and ergine may play a more important role in affecting feed intake, weight gains and serum prolactin.
Effects on animal production
The deterrent grazing effect of ergovaline mentioned above along with reduced nutritive value, and heat stress can result in poor animal live weight production gains, milk production and growth rates (Peters et al. Citation1992; Watson et al. Citation1999; Browning Citation2012; Duckett et al. Citation2014) leading to significant costs to agriculture (Hoveland Citation1993).
Effects of ergovaline identified in New Zealand
In New Zealand, the most obvious effect of ryegrass infected with Epichloë endophytes is ryegrass staggers caused by the ingesting of lolitrem B (Fletcher and Harvey Citation1981; Fletcher Citation1982). Due of the co-occurrence of lolitrem B with ergovaline in these associations it can be difficult to isolate the effects of just ergovaline. However, RH Watson (pers. comm. in a report to Meat and Wool NZ 2007) concluded that in standard endophyte pasture the presence of ergovaline is a greater factor in the reduction of feed intake and changes in diet selection behaviour in sheep than lolitrem B. By showing an increased concentration of ergovaline compared with lolitrem B in pasture over a 14 d grazing period it was deduced that sheep selected components of the pasture that did not contain ergovaline, even though they may have contained relatively high concentrations of lolitrem B, and have been nutritionally inferior to the components being avoided.
Effects of ergovaline have been identified through depressed live weight gain (Hannah et al. Citation1990; Fletcher et al. Citation1991; Fletcher and Sutherland Citation1993b; Browning Citation2012), lowered serum prolactin levels (Thompson et al. Citation1993; Blackwell and Keogh Citation1999), and increased body temperature (Fletcher Citation1993b; Bluett et al. Citation2001a). The possibility also exists of a synergistic effect between the two toxin classes. In some New Zealand studies, there is the suggestion of ergovaline exacerbating ryegrass staggers (Fletcher and Easton Citation1997) while in others there has been no evidence for a synergistic effect of ergovaline to increase the effects of lolitrem B (Finch et al. Citation2018). In Australia, occasional severe perennial ryegrass toxicity (PRGT) is observed in sheep and Reed et al. (Citation2016) concluded that the ingestion of ergot alkaloids combined with the impact of high solar radiation on the animals’ heat load are important factors in this unusually severe form of ryegrass toxicity. The use of endophyte strains such as 187BB in perennial ryegrass (Fletcher Citation1993b; Easton et al. Citation2001) which expresses ergovaline without lolitrem B have become useful tools to understand the direct impact of ergovaline on animals under New Zealand environmental conditions. These studies, which are described in more detail in the section above reviewing 'environmnetal effects on ergovaline expression; indicated that ryegrass staggers may obscure the more subtle symptoms of heat stress possibly linked with the presence of ergot alkaloids. Fletcher (Citation1993a) surmised that at ambient temperatures above 25 °C ergovaline-expressing endophytes in ryegrass may cause heat stress and result in significant losses to animal production, in both sheep and dairy cows.
Nicol and Klotz (Citation2015) highlighted the difficulties in determining the effect of varying concentrations of ergovaline on animals due to (1) inaccurate predictions of ergovaline intake caused by the ergovaline concentration of the diet on offer not representing the concentration in the diet consumed, and (2) dry matter intake rates being ignored or difficult to estimate in field trials. The use of urine lysergol:creatinine ratios as an estimation of the amount of ergovaline consumed by animals shows some promise (Hill et al. Citation2000; Layton et al. Citation2004) at least when comparing studies conducted over time. More details on the various trials on grazing animals are presented below.
Production and welfare effects of ergovaline on grazing animals
Dairy cows
Early documented reports (Brookbanks et al. Citation1985) of what was then called ‘bovine hyperthermia’ was linked to farms in Bay of Plenty and Northland where animals were observed to have excessive salivation, inappetence, rough coats, and would stand with open mouths and protruding tongues, seeking shade and water to stand in. Although all of these farms had tall fescue present, in some instances it was only a small amount along farm races. The condition would occur between November and April and resulted in lost production of up to 50% in one instance. Others have linked decreases in milk production in Northland to heat stress during some periods in summer and the consumption of endophyte alkaloids (Blackwell Citation1999).
Dairy cows in many areas of New Zealand can be affected by heat stress during summer (Easton et al. Citation1996; Keogh et al. Citation1999; Bryant et al. Citation2007b; deNicolo et al. Citation2015). Heat stress occurs when the ‘heat load’ of the animal is greater than its capacity to lose heat due to high ambient temperature and humidity, direct solar radiation and lack of air movement (Blackshaw and Blackshaw Citation1994). The combined effect of temperature and humidity can be measured through a calculated Temperature Humidity Index (THI) (Davis et al. Citation2003). When THI reaches 68 for Friesians and 75 for Jerseys (equivalent to 21 and 25.5 °C respectively at 75% relative humidity) New Zealand cows begin to experience the effects of heat stress (Bryant et al. Citation2007b). Several overseas studies have observed milk yield reductions when the THI exceeds an average of 72 for three consecutive days even when the diet is largely made up of concentrates (Ravagnolo et al. Citation2000; Aharoni et al. Citation2002; West et al. Citation2003). This is related to a reduction in feed intake and a concomitant drop of around 10 g milksolids per day per unit increase in THI (Bryant et al. Citation2007b). Overseas studies have consistently shown that dairy cows respond to heat stress by reducing milk production (Kadzere et al. Citation2002; West Citation2003), again largely due to reduced feed intake. It is also well known that heat stress in ruminants can be exacerbated by ergovaline (Osborn et al. Citation1992; Aldrich et al. Citation1993) through causing vasoconstriction (Klotz et al. Citation2008, Citation2010). In the USA, many trials have shown negative effects of tall fescue endophyte infection on live weight gain, milk production and welfare of cattle (Hoveland et al. Citation1980; Hemken et al. Citation1981; Schmidt et al. Citation1982; Wallner et al. Citation1983; Hemken et al. Citation1984; Stuedemann and Hoveland Citation1988; Crawford et al. Citation1989; Hoveland Citation1993; Schmidt and Osborn Citation1993). Similarly, in Australia, ergovaline at levels of 0.4–0.75 μg/g DM in ryegrass fed as silage has resulted in a significant 4.6 L/cow/day decrease (15%) in milk production (Lean Citation2001). Concomitantly, milk somatic cell count increased significantly, and reproductive performance declined. Body condition score and coat condition were also negatively affected. Valentine et al. (Citation1993) comparing cv. ‘Ellett’ perennial ryegrass with and without endophyte showed significantly lower milk yields (up to 12% lower) when cows were fed the grass with endophyte (producing both ergovaline and lolitrem B).
The importance of night temperatures was recognised by Ansell (Citation1976) who showed that cattle during the day can accumulate heat in their bodies without adverse effects if night temperatures are sufficiently low to allow for the dissipation of heat. Fletcher (Citation1998) therefore proposed that, if night temperatures were high, then cattle could not dissipate the heat in their bodies accumulated during the day, and it may be the impact of high night temperatures that would result in hyperthermia during prolonged hot periods.
As pointed out by Bryant et al. (Citation2007a) in Northland, average milksolids yield is 218 kg per cow compared with Southland, where average milksolids yield is 418 kg per cow (LIC and DairyNZ Citation2019). Mean daily maximum air temperature is more than 5.0 °C higher in the north of the North Island (Kaitaia 16.4°C) than in the south of the South Island (Gore 10.8°C) (National Institute of Weather and Atmospheric Research Citation2018). However, one could also argue that factors other than just temperature are impacting here, including the fact that lower levels of toxic standard endophyte, producing ergovaline, are either required or common in pastures in Southland, compared with northern New Zealand (Widdup and Ryan Citation1992; Eerens et al. Citation1998) due to different insect pest pressures.
A comparison of milk production of dairy cows grazing a ryegrass cultivar infected with the AR1 strain of endophyte (which expresses no toxins) with dairy cows grazing a standard endophyte (expressing both ergovaline and lolitrem B) through two mild summers in Northland (Ussher Citation2003) showed increases in milk yield of AR1 over the standard endophyte of 5% in year 1 and 1.4% in year 2. However, during the months of high temperatures (23°C–25°C average daily maximum temperatures) in January and through to mid-March the herd grazing AR1 pastures produced 21% more milk than those on standard endophyte pastures. Similarly, dairy cows grazing cv. ‘Bronsyn’ ryegrass containing AR1 in the Waikato showed a 8.9% improvement in total milksolids compared to cows grazing ‘Bronsyn’ standard-infected pastures over a 3-year period (318 versus 292 kg/cow, SED = 9.2, P = 0.006) (Bluett et al. Citation2005). During this time, ergovaline levels in the standard endophyte-infected pasture ranged between 0.17 and 0.75 μg/g DM. However, there were no significant effects of endophyte strain on mean or maximum daily cow body temperatures (using a temperature logger mounted in a progesterone-free Controlled Internal Drug Release (CIDR)) in January, February, or March. In a short-term trial, cows grazing ryegrass cultivar ‘Vedette’ infected with the AR1 endophyte produced 8% more milk than those grazing ‘Vedette’ infected with standard endophyte, and this coincided with the maximum levels of ergovaline in the standard endophyte-infected pastures (0.7 ng/ml) (Bluett et al. Citation2001b).
Blackwell and Keogh (Citation1999) (also see Keogh et al. Citation1999) described a trial in Northland comparing two groups of 16 spring-calving, 3-year-old Holstein-Friesian cows grazing pastures either with or without ergovaline and lolitrem B. Although the presence of lolitrem B may have had an influence on this experiment they were able to establish a negative correlation between milk production of the group ingesting ergovaline and lolitrem B in January with the prevailing temperature and humidity conditions during the night, which suggest that ergovaline was having an impact. While there was no relationship between daily maximum temperature and milk production, there was a significant positive relationship (R2 = 0.94) between THI and milk production for cows on the endophyte free pastures, but this relationship was negative (R2 = 0.60) for cows on the pasture containing alkaloids. Over a milking season (1 October to 29 April) cows grazing ergovaline- and lolitrem-free pasture produced 19% more milk solids per cow (measured using herd test data) than cows grazing pastures containing these toxins. Over this period ergovaline concentrations ranged from 0.15 to 0.4 μg/g DM in the leaf blade and from 0.3 to 1.5 μg/g DM in the leaf sheath. The difference in milksolids per cow between animals grazing the two endophyte treatments was greatest between December and late March when ergovaline concentrations were highest, concentrations during this period were between 0.27 and 0.40 μg/g DM in the leaf blade and 0.8 and 1.3 μg/g DM in the leaf sheath. However, not all studies comparing standard endophyte with endophyte free pastures have shown clear and consistent differences for dairy production (Thom et al. Citation1997, Citation1999; Clark et al. Citation1999). Eady et al. (Citation2017) estimated ergovaline intake by cows at three sites across New Zealand and showed that for diploid ryegrass, ‘Bronsyn’ with standard endophyte, ergovaline intake was 0.016–0.056 mg/kg LW0.75/day; and for diploid ryegrass ‘Trojan’ with NEA2, estimated ergovaline intake ranged from 0.01 to 0.029 mg/kg LW0.75/day. In their review Nicol & Klotz (Citation2016) found only four studies showing negative effects of ergovaline intake on production (liveweight gain or milk yield) when intake was below 0.07 mg/kg LW0.75/day, all of which involved standard endophyte, with the potential confounding effect of lolitrem B expression. To allow comparison between units 0.07 mg/kg LW0.75/day is associated with an ergovaline concentration in ryegrass of 0.7 μg/g DM.
Fletcher (Citation1998) reported dairy cows grazing standard Epichloë-infected ryegrass have shown unexplained lameness and swelling of the fetlock, which did not respond to antibiotics.
Cattle
Easton et al. (Citation1996) noted that there were many instances described in New Zealand were ergovaline containing ryegrass pastures resulted in cattle with high rectal temperatures, high respiration rate, panting, drooling of clear mucus from the mouth and nose, extrusion of the tongue, and searching for shade and cool. A loss of condition was reported, with rough coats often coated with mud or faeces. Production losses of up to 50% on a herd basis were reported. To further investigate this Easton et al. (Citation1996) sampled pastures close to ground level in the summer of 1992–1993 from Canterbury, Manawatu, Hawke’s Bay and Taranaki; from pastures in Northland in the summer of 1993–1994; and from pastures in Waikato and near Dannevirke in April 1995. The results did not indicate major differences between ergovaline levels from samples taken from the different regions of New Zealand. They found that 37% of samples had ergovaline concentrations below 0.5 μg/g DM, 34% between 0.5 and 1.0 μg/g DM; 13% between 1.0 and 1.5 μg/g DM; and 16% greater than 1.5 μg/g DM. On six farms where heat stress in cattle was reported,the ergovaline concentrations ranged from 0.1 to 1.8 μg/g DM. In addition, sampling of Waikato pastures in April 1993 showed that 52% of samples had ergovaline concentrations below 0.5 μg/g DM, 30% between 0.5 and 1.0 μg/g DM; 14% between 1.0 and 1.5 μg/g DM; and 4% greater than 1.5 μg/g DM. From this Fletcher (Citation1998) surmised that in regions prone to heat stress these ergovaline levels would be high enough in summer and autumn to exacerbate heat stress symptoms. He also noted the importance of night temperatures as discussed earlier.
Easton et al. (Citation1993, Citation1996) surmised that cattle grazing standard endophyte-infected ryegrass during summer and autumn in northern New Zealand ingest herbage which may contain over 1 μg/g DM ergovaline, and sometimes over 1.5 μg/g DM. While these levels are much lower than those common in roadside tall fescue in New Zealand, they do approach the level of ergovaline found in endophyte-infected tall fescue cultivars in the USA that are well-recognised to cause elevated body temperatures and other symptoms associated with fescue toxicosis. They therefore concluded that in New Zealand, ergovaline levels in standard endophyte-infected perennial ryegrass pastures can reach levels sufficient to cause fescue toxicosis symptoms in livestock if ambient conditions are suitable, and that for many reported cases of bovine idiopathic hyperthermia, endophyte-infected perennial ryegrass pastures are a more likely cause than the very small amounts of volunteer tall fescue available to animals.
Cosgrove et al. (Citation1996) noted that on a cool day (daily max: 17.8 °C), young cattle grazing ryegrass with an endophyte strain 187BB, which expresses ergovaline but no lolitrem B, had small but significantly elevated rectal temperatures compared with standard or nil endophyte treatments. There have been few statistically significant effects on body temperature recorded (Blackwell & Keogh Citation1999; Easton & Couchman Citation1999) despite visible heat stress being observed on-farm (a number of surveillance references are provided by Easton et al. (Citation1996)). Given the severe effects on cattle performance in trials in USA, Lane et al. (Citation1999) concluded that factors other than ergovaline may contribute to the toxicosis induced by endophyte-infected tall fescue. This conclusion was also reached by Gadberry et al. (Citation2003) when comparing the effects of ergovaline alone and ergovaline plus endophytic tall fescue on lambs.
Cosgrove et al. (Citation1996) in their study on the impact of endophyte strains on weaner cattle also noted reduced herbage intake, and diet digestibility in animals grazing ergovaline producing endophytes, but no effects on live weight gain or blood prolactin levels (). Results of this study (Cosgrove et al. Citation1996) indicate that ergovaline intakes of 0.048 mg/kg LW/day are required for ergovaline-related effects in cattle, at least under the environmental temperatures and relative humidity experienced in Manawatu. In contrast, with tall fescue, Debessai et al. (Citation1993) reported reduced feed intake and reduced live weight gain in lambs at daily ergovaline intakes as low as 0.026 mg/kg LW/day.
Table 3. Comparison of three endophyte treatments and their effect on weaner cattle (data were derived from Cosgrove et al. Citation1996).
Cornell et al. (Citation1990) showed that cattle fed herbage with up to 0.2 µg/g DM ergovaline showed a significant increase in rectal temperature when ambient temperatures were at 31 °C or above. In noting that as little as 0.05 µg/g DM ergovaline (fed as endophytic fescue seed) can cause measurable physiological effects in cattle, they concluded that there may be no level of ergovaline that does not cause an effect under some combinations of high ambient temperature and humidity in susceptible animals.
Sheep
The use of diploid perennial ryegrass infected with the 187BB endophyte, expressing ergovaline but no lolitrem B, has demonstrated that in New Zealand conditions, ergovaline alone can depress live weight gain, elevate body temperatures and increase respiration rates of sheep (Fletcher Citation1993a; Fletcher and Easton Citation1997). Indoor trials under thermoneutral conditions have shown that sheep fed ryegrass containing a combination of lolitrem B and ergovaline have lower dry matter intakes, lower plasma prolactin and higher urine output, faecal moisture, rectal temperature and respiration rate compared to sheep fed toxin-free ryegrass (Henry et al. Citation2015). Although this trial utilised a diet containing both ergovaline and lolitrem B, it nevertheless highlights that sheep can exhibit heat stress symptoms even under thermoneutral conditions. Heat stress, measured as increased body temperature and respiration rate, at ambient temperatures in Canterbury, New zealand, during summer/autumn, has been reported in sheep grazing ryegrass with standard endophyte (Fletcher Citation1998; Fletcher et al. Citation1999) (). Furthermore, although rectal temperature of lambs increased with increasing ambient temperature the rectal temperature of lambs grazing ryegrass infected with the 187BB endophyte which had higher concentrations of ergovaline (and no lolitrem B) were consistently higher compared to lambs grazing ryegrass infected with the standard endophyte, which had lower concentrations of ergovaline but did contain lolitrem B, as well as animals grazing endophyte-free ryegrass which contained no toxins (). At an ambient temperature of only 13°C the rectal temperature of the lambs grazing the high ergovaline pastures were approximately 40.55°C compared to the lambs grazing low ergovaline pastures or toxin-free pastures which had rectal temperatures of 40.05°C and 39.95°C, respectively (Fletcher Citation1993b). The respiration rates in this study were also observed to be higher in the animals grazing the 187BB infected pasture compared to the other two treatments. Heat stress symptoms have been observed to be more severe in lambs forced to graze lower into the sward (Bluett et al. Citation1999). Fletcher et al. (Citation1999) concluded from a review of results across New Zealand that heat stress in lambs and sheep due to ergovaline would be especially prevalent in the warm humid conditions of northern New Zealand.
Figure 3. Effect of ryegrass/endophyte association and ambient temperature, in March 1992, on rectal temperature of grazing lambs (from Fletcher Citation1993b). Mean ergovaline content at time of testing were 1.3 μg/g DM for ‘Nui’ wild-type (Δ- __ - Δ), 4.7 μg/g DM for ‘Nui’ 187BB (●____ ●) and zero for ‘Nui’ Nil (□----□) (from Davies et al. Citation1993).
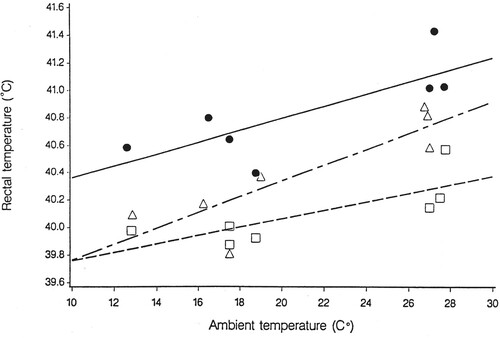
Table 4. Effect of endophyte and ergovaline, in perennial ryegrass, on body temperature and respiration rate of grazing lambs, at ambient temperature of 28°C. (Taken from Fletcher Citation1998).
Fletcher et al. (Citation1991) in a trial in Canterbury demonstrated that after 14 days lambs grazing ryegrass infected with either standard endophyte or 187BB endophyte gained less weight than lambs grazing on endophyte-free ryegrass although only the lambs grazing the standard endophyte treatment lost weight after 28 days. Fletcher (Citation1993b) found that rectal temperatures of lambs transferred to a heat chamber indoors were highest in lambs grazing ryegrasses with high levels of ergovaline. Furthermore, ergovaline in the diet, with or without lolitrem B, increased dag weight, reduced faecal dry matter and increased fly strike of lambs (Fletcher and Sutherland Citation1993a). Fletcher et al. (Citation1999) concluded that many of these symptoms resulting from ingestion of standard endophyte were similar to those described for the ‘summer/autumn ill thrift’ syndrome in New Zealand, resulting in stock growing at a slower than expected rate.
Aiken et al. (Citation2011) using 187BB in perennial ryegrass showed that the auricular artery of lambs was vasoconstricted when they were exposed to ergovaline. In this study the level of ergovaline expression was 0.9–1.0 μg/g DM. The authors concluded that lambs on strain 187BB pasture were more vulnerable to heat stress than lambs grazing endophyte-free pastures. Lambs were removed from 187BB pastures after 19 days and placed on endophyte-free pastures for a further 18 days, but this was not long enough to restore the lamb’s ability to physiologically adjust to increases in ambient temperature. It is generally accepted that one of the main physiological effects attributed to ergot alkaloid is a reduced ability to regulate body temperature (Oliver Citation1997).
It has been noted that the fertility of sheep flocks in New Zealand have been well below their potential (Knight Citation1990). Ewes, in New Zealand, grazing tall fescue with 3.30 ± 0.60 μg/g DM (although quoted as mg/g) ergovaline had significantly lower ovulation rates and number of lambs carried to 90 days of pregnancy compared with ewes grazing nil endophyte pastures (Kramer et al. Citation1999). Watson et al. (Citation1999) concluded that ewes grazing pastures where ergovaline levels exceed 2 μg/g DM are likely to suffer a reduction in reproductive performance. Exposure to ergot alkaloids inutero reduces foetal growth and muscle development in sheep (Duckett et al. Citation2014). This is consistent with studies on small mammals where endophyte-infected tall fescue has been found to reduce reproductive potential in rats (Varney et al. Citation1987, Citation1988) and mice (Zavos et al. Citation1988, Citation1990). Horses are particularly susceptible to ergot alkaloids resulting in increased gestation lengths, agalactia, foal and mare mortality, tough and thickened placentas, weak and dysmature foals, and reduced serum prolactin and progesterone levels (Cross Citation1997). Ergotamine administered to mice, rats and rabbits resulted in maternal toxicity and foetal abnormalities (Grauwiler and Schon Citation1973).
Ergot alkaloids in the food chain
While the majority of studies seeking to understand the impact of ergovaline in animal feed has been focused on the direct effect on the animal itself there is another perspective to consider when animal products (milk and meat) are then consumed by humans. Although regulatory limits are set with regards to permissible levels of ergot/kg grain in the USA, Canada, New Zealand, Australia and the EU, ergot alkaloids are not regulated for food in any country (Anon Citation2005). However, some countries regulate ergot alkaloids in animal feeds (FAO Citation2004). In Canada, guideline limits for ergots in feed are 6 μg/g for pigs, 3 μg/g for dairy cattle, sheep and horses and 9 μg/g for chickens, all of which seem high. In contrast, the limit in Uruguay is much lower at 0.45 μg/g and in Oregon, the ergovaline limit for tall fescue and ryegrass straw exported to Japan as cattle feed has been set at 0.5 μg/g DM (Hume et al. Citation2016).
Ergovaline and lysergic acid are detectable in the urine and faeces of cattle consuming endophytic tall fescue (Stuedemann et al. Citation1998; Schultz et al. Citation2006) with concentration in rumen fluid apparently increasing over time (De Lorme et al. Citation2007; Ayers et al. Citation2009). In fact, Stuedemann et al. (Citation1998) found that 96% of the ergopeptine alkaloids (using lysergic acid for the standard curve) consumed by cattle grazing endophyte-infested tall fescue were excreted via the urine. This led to the proposal that ergovaline is metabolised into lysergic acid that might then be absorbed through the rumen wall (Hill et al. Citation2001). Most of the ergot alkaloids, as weak bases, are amphipathic (i.e. possessing both polar and nonpolar components) (Strickland et al. Citation2011) and their absorption would appear to be limited to the small intestine in non-ruminants (Rothlin Citation1933) and the fore-stomach (Hill et al. Citation2001) and intestine in ruminants. Ruminants appear to handle the metabolism and elimination of ergot alkaloids better than non-ruminants due to a more efficient microbial metabolism before absorption along the gastrointestinal tract (Strickland et al. Citation2011). Using Caco-2 cells derived from human colon carcinoma, Shappell and Smith (Citation2005) showed that ergovaline and its naturally occurring isomer, ergovalinine, readily crossed intestinal cells intact and at similar rates.
LD50s for a number of ergot alkaloid analogues have been determined orally and intravenously (i.v.) using mice, rats and rabbits (Griffith et al. Citation1978). Rabbits were the most sensitive to ergot alkaloids. Ergotamine showed an intravenously administered LD50 of 265 mg/kg in mice with toxicity being 7 and 88 times greater in rats and rabbits, respectively. Not surprisingly oral toxicity, the most relevant route of administration, was considerably lower than that determined by intravenous dosing with the LD50 by gavage being 3200 mg/kg in mice, 1300 mg/kg in rats and 550 mg/kg in rabbits (Griffith et al. Citation1978). Symptoms associated with these acute intoxications include vomiting, diarrhoea, cardio-vascular collapse, convulsions, agony and abortions (Guggisberg Citation1954; Lewin Citation1962). Ergotamine has been fed to rats at concentrations of up to 500 mg/kg for 4 weeks. This study showed a dose-dependent decrease in food intake and weight gain with females being more susceptible than males, leading the authors to conclude that this was likely due to a decreased palatability at concentrations of >100 mg/kg ergotamine. A no-observable-adverse-effect-level (NOAEL) was set at 0.3 mg/kg/day. A further rat study was conducted using appropriate dose rates for a period of 13 weeks. Despite the extended time period, the NOAEL in this study was 0.4 mg/kg/day ergotamine. Similar 4-week studies have been conducted with ergometrine and α-ergocryptine which showed similar NOAELs (0.6 and 0.3 mg/kg/day, respectively). Furthermore, developmental and reproductive toxicity, effects on lactation and genotoxicity have been investigated for some of the ergot alkaloid analogues, although none have been conducted using ergovaline (EFSA Citation2012). Ergotamine has therapeutic applications in humans, most commonly for the treatment of migraine headaches. The usual oral dose for this purpose is 1–2 mg ergotamine per dose with a limit of up to 6 mg/day, 12 mg/week and 20 mg/month.
The risk to human health is a function of both toxicity of the substance as well as the exposure. It is therefore important to consider the amounts of ergovaline which can be feasibly ingested by a consumer. There is no convincing evidence that ergot alkaloids accumulate in edible tissues of animals fed ergot alkaloids. Exposure of dairy cows to 50 g ergot sclerotia per animal (approximately 100 kg body weight) resulted in total ergot alkaloid concentrations in milk of 0.086 mg/litre (Parkheava Citation1979). In another study, dairy cows were fed an alkaloid concentration of 1835 μg/animal/day (equivalent to 3 μg/kg body weight) with no residues of ergot alkaloids in milk (Wolf et al. Citation1995). The authors surmised that the carry-over rate of ergot alkaloids into milk is less than 10% of the ingested dose. Consistent with this hypothesis, Schumann et al. (Citation2009) were unable to detect ergot alkaloids in the milk or blood of cows fed ergot-contaminated concentrate at an alkaloid exposure rate of 4.1–16.3 μg/kg of body weight. However, in contrast, low but detectable concentrations of ergovaline were detected in the milk of lactating ewes fed hay of perennial ryegrass infected with standard endophyte (Zbib et al. Citation2015). Ergovaline could also be detected in the liver and kidneys of these animals but not in the muscle, fat or brain (Zbib et al. Citation2015). Also showing ergovaline residues was Realini et al. (Citation2005) who grazed steers on endophyte-infected tall fescue and detected ergovaline in sub-cutaneous fat tissue.
Overall, the data available on ergovaline residues in animals is limited but the studies that are available point to the presence of only very low concentrations of ergovaline in milk and animal tissue. It is generally accepted that ergot alkaloids in animal tissues at these levels will not be an important source affecting humans (Anon Citation2005).
Threshold concentrations for ergovaline
As detailed earlier it is difficult to accurately compare the results of different studies due to the use of different methodologies of sampling as well as pre-analysis handling and storage of samples. Comparison, and the proposal of a threshold concentration for ergovaline, is further complicated by the common co-occurrence of lolitrem B under New Zealand conditions and the suggestion that the toxic threshold is different in tall fescue and perennial ryegrass. Hume et al. (Citation2016) has summarised the concentrations for a toxic threshold of ergovaline in animal feed for both ruminants and monogastric animals using data published by di Menna et al. (Citation1992), Stamm et al. (Citation1994); Tor-Agbidye et al. (Citation2001), Aldrich-Markham et al. (Citation2003), Fink-Gremmels (Citation2005), Alabdouli et al. (Citation2014), and Zbib et al. (Citation2014a). These toxic thresholds were 0.40–0.75 μg/g DM for cattle; 0.50–0.80 μg/g DM for sheep; and 0.30–0.50 μg/g DM for horses, except for mares in late pregnancy when the safe threshold level is zero. Cornell et al. (Citation1990) reported that even 0.05 μg/g DM ergovaline in tall fescue can cause increased body temperature in cattle. Ergovaline levels of 0.5–1.5 μg/g DM in leaf is considered comparable to those associated with symptoms of toxicity in endophyte-infected tall fescue in south east USA (Easton et al. Citation1993).
In New Zealand, for sheep (hoggets) fed 0.0–0.075 mg ergovaline/kg LW there was a linear decrease in liveweight gain from 110 to 60 g/d (P < 0.01) and 30 days later, from 35 to −130 g/d (P < 0.05); and for cattle (heifers) fed 0.0–0.035 mg ergovaline/kg LW there was a decline in liveweight gain from 1.0 to 0.85 kg/d (P < 0.05) (Layton et al. Citation2004). A study conducted in New Zealand and summarised in 2011 on ‘The Beef Site’ concluded that cattle are slightly less susceptible to ergovaline toxicity than sheep with respect to live weight gain suppression (http://www.thebeefsite.com/articles/2932/vasoconstriction-heat-stress-caused-by-ergot-alkaloids-fescue-toxins/). They provided estimates of ergovaline intake and urine lysergol:creatinine ratios deemed to be toxic and sub-toxic for cattle and sheep ().
Table 5. Estimates of toxic and sub-toxic ergovaline intake levels and concentrations in pasture fed to cattle and sheep, and urine lysergol:creatine ratios.
In a review seeking to understand the impact of ergovaline intake from New Zealand pastures Nicol and Klotz (Citation2016) used studies from nine publications (including the Layton et al. Citation2004 study) to conclude that feed intake or animal production may be depressed when ergovaline intake is greater than 0.07 mg/kg liveweight0.75/day or when ergovaline levels in pasture were 0.7 μg/g DM or higher. They pointed out that both of these levels were much higher than thresholds suggested in the USA for animals feeding on tall fescue where ergovaline effects might occur above intakes rates of 0.035–0.045 mg/kg liveweight0.75/day (Bohnert and Merrill Citation2006) and ergovaline pasture levels of 0.475 μg/g DM (Stamm et al. Citation1994). They concluded that a high proportion of New Zealand pastures should (on average) not contain sufficient ergovaline to provide an ergovaline intake high enough to depress animal productivity or cause animal-welfare concerns. However, Fletcher et al. (Citation1994) indicated that significantly elevated body temperature and respiration of lambs can occur with ergovaline concentrations of only 0.5 μg/g DM. He also commented that the time taken for the body temperature of affected animals to return to normal was slow and observed over a 10-day period on endophyte-free pastures the elevated body temperature of affected animals remained un-changed. He concluded that - ergovaline, even at low concentrations, affects thermo-regulation in lambs and is undesirable in perennial ryegrass-endophyte associations.
In a later report, Fletcher et al. (Citation2017) considered that many farmers do not appreciate the potential for ergovaline to induce animal production problems especially since it is only relatively recently that endophytes which express ergovaline have been marketed. Some of these commercially-available endophytes that express ergovaline can result in increased body temperature and respiration rates similar to that which would occur in animals grazing standard endophyte-infected pastures when exposed to heat stress. However, the severity of ergovaline-induced effects in animals grazing endophyte-infected pastures will depend on farm type, location, balance of toxic and non-toxic pastures in the system, the number and age of sheep exposed to peak toxin levels, climatic conditions and management (Fletcher et al. Citation1999).
Animals suffering from heat stress is a welfare issue that can be avoided through providing shade and reducing the consumption of ergovaline. Other than providing endophyte-free ryegrass pastures which are unlikely to persist in most areas of New Zealand there are several non-ergovaline producing endophytes available (Fletcher Citation1999; Thom et al. Citation2007; Fletcher Citation2012; Caradus et al. Citation2013; Johnson et al. Citation2013; de Bonth et al. Citation2015) with active discovery pipelines searching for further suitable strains. The inclusion of white clover in the grazed diet has also been associated with reducing the impact of ergovaline producing endophytes on milk production (Clark et al. Citation1996; Fletcher Citation1998).
Concluding comments
In identifying endophytes for use in ryegrass pastures in New Zealand there have been two directions pursued. Either -
That the minimum standard should be the exclusion of all ergopeptine alkaloids and lolitrems (Fletcher and Easton Citation1997); or
To enhance the stability of natural defences against invertebrate pests some ergopeptine presence may be beneficial (Popay and Rowan Citation1994; Ball et al. Citation1995; Popay and Wyatt Citation1995).
Both arguments have their strengths and weaknesses and it is a matter of balancing these to ensure the end-user has access to a product that satisfies their needs – a persistent pasture with low to nil animal toxicity in terms of both animal production and welfare. As noted above there is a significant risk in assuming that low ergovaline expressing endophyte strains will have the same low alkaloid expression irrespective of the host genetics and environment in which it is grown (Lane et al. Citation1997b). Endophyte strains that produce no ergovaline and yet provide good grass persistence are available, and widely used (Caradus et al. Citation2013). Future endophyte strains used in New Zealand should seek to avoid any ergovaline expression as one cannot assume that farmers graze swards to ensure that portions of the plant with highest ergovaline content are avoided, and that seasonal spikes in ergovaline content do not occur. In addition, it is predicted that temperatures across New Zealand, particularly in the North Island,will increase (Ministry for the Environment Citation2008, Citation2018) and this alone may exacerbate potential impacts of ergovaline on animal health and welfare.
Disclosure statement
Grasslanz Technology Ltd is the intellectual porperty owner of AR1, Endo5, and AR6 (strain 187BB).
References
- Adcock RA, Hill NS, Bouton JH, Boerma HR, Ware GO. 1997. Symbiont regulation and reducing ergot alkaloid concentration by breeding endophyte-infected tall fescue. Journal of Chemical Ecology. 23:691–704.
- Agee CS, Hill S. 1994. Ergovaline variability in Acremonium-infected tall fescue due to environment and plant genotype. Crop Science. 34:221–226.
- Aharoni Y, Ravagnolo O, Misztal I. 2002. Comparison of lactational responses of dairy cows in Georgia and Israel to heat load and photoperiod. Animal Science. 75:469–476.
- Aiken, GE, Flythe, MD. 2014. Vasoconstrictive responses by the carotid and auricular arteries in goats to ergot alkaloid exposure. Frontiers in Chemistry. 2: Article 101. doi:https://doi.org/10.3389/fchem.2014.00101.
- Aiken GE, Kirch BH, Strickland JR, Bush LP, Looper ML, Schrick FN. 2007. Hemodynamic responses of the caudal artery to toxic tall fescue in beef heifers. Journal of Animal Science. 85:2337–2345.
- Aiken GE, Strickland JR, Looper ML, Bush LP, Schrick FN. 2009. Hemodynamics are altered in the caudal artery of beef heifers fed different ergot alkaloid concentrations. Journal of Animal Science. 87:2142–2150.
- Aiken GE, Sutherland BK, Fletcher LR. 2011. Haemodynamics of lambs grazing perennial ryegrass (Lolium perenne L.) either infected with AR6 novel, wild-type endophyte, or endophyte-free. New Zealand Veterinary Journal. 59:179–184.
- Alabdouli KO, Blythe LL, Duringer JM, Elkhouly A, Kassab A, Askar M, Mohammed EE, Al-Juboori A, Craig AM. 2014. Physiological effects of endophyte-infected perennial ryegrass straw on female camels in the Middle East. Emirates Journal of Food and Agriculture. 26:82–92.
- Aldrich-Markham S, Pirelli G, Craig AM. 2003. Endophyte toxins in grass seed fields and straw effects on livestock. Oregon State University Extension Service, Corvallis, Oregon, p. 1–4, EM 8598.
- Aldrich CG, Patterson JA, Tate JL, Kerley MA. 1993. The effects of endophyte-infected tall fescue consumption on diet utilisation and thermal regulation in cattle. Journal of Animal Science. 71:164–170.
- Anon. 2005. Opinion of the scientific panel on contaminants in food Chain on a request from the Commission related to ergot as undesirable substance in animal feed question N° EFSA-Q-2003-38. The EFSA Journal 225:1–27. https://efsa.onlinelibrary.wiley.com/doi/epdf/10.2903/j.efsa.2005.225.
- Ansell RH. 1976. Maintaining European dairy cattle in the Near East. World Animal review. Published by Food and Agriculture Organisation of United Nations. No. 20: 1–7.
- Arechavaleta M, Bacon CW, Plattner RD, Hoveland CS, Radcliffe DE. 1992. Accumulation of ergopeptide alkaloids in symbiotic tall fescue grown under deficits of soil water and nitrogen fertilizer. Applied Environmental Microbiology. 58:857–861.
- Ayers AW, Hill NS, Rottinghaus GE, Stuedemann JA, Thompson FN, Purinton PT, Seman DH, Dawe DL, Parks AH, Ensley D. 2009. Ruminal metabolism and transport of tall fescue ergot alkaloids. Crop Science. 49:2309–2316.
- Azevedo MD, Welty RE, Craig AM, Bartlett J. 1993. Ergovaline distribution, total nitrogen and phosphorus content of two endophyte-infected tall fescue clones. In: Hume DE, Latch GCM, Easton HS, editors. Proceedings of the 2nd International Symposium on Acremonium/Grass Interactions. Palmerston North: AgResearch; p. 59–62.
- Bacon CW, Lyons PC, Porter JK, Robbins JD. 1986. Ergot toxicity from endophyte-infected grasses: a review. Agronomy Journal. 78:106–116.
- Ball OJ-P, Lane GA, Prestidge RA. 1995. Acremonium lolii, ergovaline and peramine production in endophyte-infected perennial ryegrass. Proceedings New Zealand Plant Protection Conference. 48:224–228.
- Ball OJ-P, Miles CO, Prestidge RA. 1997. Ergopeptine alkaloids and Neotyphodium lolii mediated resistance in perennial ryegrass against adult Heteronychus arator (Coleoptera: Scarabaeidae). Journal of Economic Entomology. 90:1382–1391.
- Barker DJ, Davies E, Lane GA, Latch GCM, Nott HM, Tapper BA. 1993. Effect of water deficit on alkaloid concentrations in perennial ryegrass endophyte associations. In: Hume DE, Latch GCM, Easton HS, editors. Proceedings of the 2nd International Symposium on Acremonium/Grass Interactions. Palmerston North: AgResearch; p. 67–71.
- Barker GM, Patchett BJ, Cameron NE. 2015. Epichloë uncinata infection and loline content afford Festulolium grasses protection from black beetle (Heteronychus arator). New Zealand Journal of Agricultural Research. 58:35–56.
- Belesky DP, Stuedemann JA, Plattner RD, Wilkinson SR. 1988. Ergopeptine alkaloids in grazed tall fescue. Agronomy Journal. 80:209–212.
- Berde B, Schild HO, editors. 1978. Ergot alkaloids and related compounds. New York: Springer-Verlag.
- Berry D, Mace W, Grage K, Wesche F, Gore S, Schardl CL, Young CA, Dijkwel PP, Leuchtmann A, Bode HB, et al. 2019. Efficient nonenzymatic cyclization and domain shuffling drive pyrrolopyrazine diversity from truncated variants of a fungal NRPS. Proceedings of the National Academy of Sciences. 116:25614–25623. doi:https://doi.org/10.1073/pnas.1913080116.
- Blackshaw JK, Blackshaw AW. 1994. Heat stress in cattle and the effect of shade on production and behaviour: a review. Australian Journal of Experimental Agriculture. 34:285–295.
- Blackwell MB. 1999. Ergovaline and heat stress in Northland cows. Dairyfarming Annual. 51:29–40.
- Blackwell MB, Keogh RG. 1999. Endophyte toxins and performance of spring-calving dairy cows in Northland. In: Woodfield DR, Matthew C, editors. Ryegrass endophyte: an essential New Zealand symbiosis. Palmerston North: New Zealand Grassland Association. Grassland Research and Practice Series 7; p. 45–50.
- Bluett SJ, Hodgson J, Kemp PD, Barry TN. 1999. Performance of lambs and the incidence of staggers and heat stress on two perennial ryegrass cultivars over three summers in the Manawatu. In: Woodfield DR, Matthew C, editors. Ryegrass endophyte: an essential New Zealand symbiosis. Palmerston North: New Zealand Grassland Association. Grassland Research and Practice Series 7; p. 143–150.
- Bluett SJ, Hodgson J, Kemp PD, Barry TN. 2001a. Performance of lambs and the incidence of staggers and heat stress on two perennial ryegrass (Lolium perenne) cultivars using a leader-follower rotational grazing management system. Journal of Agricultural Science, Cambridge. 136:99–110.
- Bluett SJ, Thom ER, Clark DA, Macdonald KA, Minnee ENK. 2005. Effects of perennial ryegrass infected with either AR1 or wild endophyte on dairy production in the Waikato. New Zealand Journal of Agricultural Research. 48:197–212.
- Bluett SJ, Thom ER, Clark DA, Tapper BA. 2001b. Milk production and composition from cows grazing perennial ryegrass infected with a novel endophyte (AR1). In: Paul VH, Dapprich P, editors. Proceedings of the 4th International Neotyphodium/Grass Interactions Symposium. Soest (Germany), September 2000. Universitat-Gesamthochschle Paderborn, Abteilung Soest, Fachbereich Agrarwirtschaft; p. 195–199.
- Bohnert D, Merrill M. 2006. Management strategies for use of high-alkaloid grass seed straw. In: Keyserlingk M, editor. Proceedings of the 41st annual Pacific Northwest Nutrition Conference, Vancouver, British Columbia, Canada. Portland (OR): CR Press Inc.; p. 113–125.
- Brookbanks E, Bell K, Fraser D, Kearns M, Sutherland R. 1985. Hyperthermia in cattle associated with tall fescue (Festuca arundinacea). New Zealand Veterinary Journal. 33:57–58.
- Browning R Jr. 2000. Physiological responses of Brahman and Hereford steers to an acute ergotamine challenge. Journal of Animal Science. 78:124–130.
- Browning R Jr. 2012. Effect of wild-type tall fescue endophyte on growth rate and feed consumption in nulliparous meat goat does. Small Ruminant Research. 105:29–32. doi:https://doi.org/10.1016/j.smallrumres.2011.11.025.
- Bryant JR, Lopez-Villalobos N, Pryce JE, Holmes CW, Johnson DL, Garrick DJ. 2007a. Environmental sensitivity in New Zealand dairy cattle of mixed breeds. Journal of Dairy Science. 90:1538–1547.
- Bryant JR, Lopez-Villalobos N, Pryce JE, Holmes CW, Johnson DL. 2007b. Quantifying the effect of thermal environment on three breeds of dairy cattle in New Zealand. New Zealand Journal of Agricultural Research. 50:327–338.
- Bush L, Bowling J, Yates S. 1979. Animal disorders. In: Buckner R, Bush L, editors. Tall fescue. Madison (WI): American Agronomy Society; p. 247–292.
- Bush LP, Wilkinson HH, Schardl CL. 1997. Bioprotective alkaloids of grass-fungal endophyte symbioses. Plant Physiology. 114:1–7.
- Canty MJ, Fogarty U, Sheridan MK, Ensley SM, Schrunk DE, More SJ. 2014. Ergot alkaloid intoxication in perennial ryegrass (Lolium perenne): an emerging animal health concern in Ireland? Irish Veterinary Journal. 2014(67):21.
- Caradus JR, Lovatt S, Belgrave B. 2013. Adoption of forage technologies. Proceedings of New Zealand Grassland Association. 75:39–44.
- Christensen MJ, Leuchtmann A, Rowan DD, Tapper BA. 1993. Taxonomy of Acremonium endophytes of tall fescue (Festuca arundinacea), meadow fescue (F. pratensis) and perennial ryegrass (Lolium perenne). Mycological Research. 97:1083–1092.
- Clark DA, Thom ER, Waugh CD. 1996. Milk production from pastures and pasture silage with different levels of endophyte infection. Proceedings of the New Zealand Society of Animal Production. 56:292–296.
- Clark DA, Thom ER, Waugh CD, Burggraaf VT. 1999. Milk production from perennial ryegrass pastures containing different levels of endophyte. Proceedings of the New Zealand Society of Animal Production. 59:258–259.
- Clay K, Cheplick GP. 1989. Effect of ergot alkaloids from fungal endophyte-infected grasses on fall armyworm (Spodoptera frugiperda). Journal of Chemical Ecology. 15:169–182.
- Cockayne AH. 1912. Perennial rye-grass seed. Journal of the Department of Agriculture. 5:242–245.
- Cornell CN, Lueker JV, Garner GB, Ellis JL. 1990. Establishing ergovaline levels for fescue toxicosis, with and without endoparasites, under controlled climatic conditions. In: Quisenberry SS, Joost RE, editors. Proceedings of the International Symposium on Acremonium/Grass Interactions; Nov 5–7; New Orleans (LA). Baton Rouge (LA): Louisiana Agriculture Experiment Station, ISSN No. 1052-5181. p. 75–79.
- Cosgrove GP, Anderson CB, Berquist TRN. 1996. Fungal endophyte effects on intake, health and liveweight gain of grazing cattle. Proceedings of the New Zealand Grassland Association. 57:43–48.
- Craig AM, Klotz JL, Duringer JM. 2015. Cases of ergotism in livestock and associated ergot alkaloid concentrations in feed. Frontiers in Chemistry 3: Article 8. doi:https://doi.org/10.3389/fchem.2015.00008.
- Crawford RJ Jr, Forwood JR, Belyea RL, Garner GB. 1989. Relationship between level of endophyte infection and cattle gains on tall fescue. Journal of Production Agriculture. 2:147–151.
- Crews C. 2015. Analysis of ergot alkaloids. Toxins. 7:2024–2050. doi:https://doi.org/10.3390/toxins7062024.
- Cross DL. 1997. Fescue toxicosis in horses. In: Bacon C, Hill N, editors. Neotyphodium/Grass Interactions. New York and London: Plenum Press; p. 289–309.
- Cunningham IJ. 1948. Tall fescue grass is poison for cattle. New Zealand Journal of Agriculture. 77:519.
- Daly GT. 1973. The grasslands of New Zealand. In: Langer RHM, editor. Pastures and pasture plants. Wellington: A H and A W Reed Ltd; p. 1–39.
- Davies E, Lane GA, Latch GCM, Tapper BA, Garthwaite I, Towers NR, Fletcher LR, Pownall DB. 1993. Alkaloid concentrations in field- grown synthetic perennial ryegrass endophyte associations. In: Hume DE, Latch GCM, Easton HS, editors. Proceedings of the 2nd International Symposium on Acremonium/Grass Interactions. Palmerston North: AgResearch; p. 72–76.
- Davis MS, Mader TL, Holt SM, Parkhurst AM. 2003. Strategies to reduce feedlot cattle heat stress: effects on tympanic temperature. Journal of Animal Science. 81:649–661.
- Dawe DL, Stuedemann JA, Hill NS, Thompson FN. 1997. Immunosuppression in cattle with fescue toxicosis. In: Bacon CW, Hill NS, editors. Proceeding 3rd Neotyphodium/grass interactions. New York & London: Plenum Press; p. 411–412.
- Debessai W, Luick BR, Cheeke PR. 1993. Effects of feeding endophyte-infected tall fescue seed on lamb performance and serum prolactin. In: Hume DE, Latch GCM, Easton HS, editors. Proceedings of the 2nd International Symposium on Acremonium/Grass Interactions. Palmerston North: AgResearch; p. 111–113.
- De Bonth A, Card S, Briggs L, Faville M, Finch S, Hong W, Johnson L, Liu L, Mace W, Pennell C, et al. 2015. Fungal foray: the pursuit of beneficial endophyte strains for Australasian pastures. In: 9th International symposium on fungal endophytes of grasses. Melbourne. p. 98–99.
- Dellafiora L, Dall’Asta C, Cozzini P. 2015. Ergot alkaloids: from witchcraft till in silico analysis. Multi-receptor analysis of ergotamine metabolites. Toxicology. 2:535–545.
- De Lorme MJ, Lodge-Ivey SL, Craig AM. 2007. Physiological and digestive effects of Neotyphodium coenophialum-infected tall fescue fed to lambs. Journal of Animal Science. 85:1199–1206.
- deNicolo G, Mace WJ, Fletcher L, Daly P, Arthur D. 2015. Ergovaline and heat stress in New Zealand – a case study. In: Proceedings of the 9th International symposium on fungal endophytes of grasses. Melbourne. p. 109–110.
- Dillard SL, Smith SR, Hancock DW. 2019. Variability of ergovaline and total ergot alkaloid expression among endophytic tall fescue cultivars. Crop Science. 59:2866–2875.
- di Menna ME, Finch SC, Popay AJ, Smith BL. 2012. A review of the Neotyphodium lolii / Lolium perenne symbiosis and its associated effects on animal and plant health, with particular emphasis on ryegrass staggers. New Zealand Veterinary Journal. 60:315–328.
- di Menna ME, Mortimer PH, Prestidge RA, Hawkes AD, Sprosen JM. 1992. Lolitrem B concentrations, counts of Acremonium lolii hyphae, and the incidence of ryegrass staggers in lambs on plots of A. lolii-infected perennial ryegrass. New Zealand Journal of Agricultural Research. 35:211–217.
- Duckett, KS, Andrae, JG, Pratt, SL. 2014. Exposure to ergot alkaloids during gestation reduces fetal growth in sheep. Frontiers in Chemistry. 2: Article 68. doi:https://doi.org/10.3389/fchem.2014.00068.
- Duringer JM, Delorme MJM, Lehner A, Craig AM. 2007. A review of the ergot alkaloids found in endophyte-infected tall fescue and perennial ryegrass and their metabolism after ingestion by livestock. In: Popay AJ, Thom ER, editors. Proceedings of the 6th International Symposium on fungal endophyte of grasses, Christchurch, New Zealand. New Zealand Grassland Association Grassland Research and Practice Series No 13; p. 377–382.
- Dyer DC. 1993. Evidence that ergovaline acts on serotonin receptors. Life Sciences. 53:223–228.
- Dymock JJ, Rowan DD, McGee IR. 1989. Effects of endophyte-produced mycotoxins on Argentine stem weevil and the cutworm Graphania mutans. In: Stahle PP, editor. Proceedings of the 5th Australasian Conference on Grassland Invertebrate Ecology. Victoria, Australia; p. 35–43.
- Eady CC, Cockran JR, Bailey KM, Kerr GA, Nicol AM. 2017. Estimation of ergovaline intake of cows from grazed perennial ryegrass containing NEA2 or standard endophyte. Journal of New Zealand Grasslands. 79:189–196.
- Easton HS, Christensen MJ, Eeren JPJ, Fletcher LR, Hume DE, Keogh RG, Lane GA, Latch GCM, Pennell CGL, Popay AJ, et al. 2001. Ryegrass endophyte: a New Zealand Grassland success story. Proceedings of the New Zealand Grassland Association. 63:37–46.
- Easton HS, Couchman JN. 1999. Ryegrass endophyte and cattle growth in Northland. ryegrass endophyte: an essential New Zealand symbiosis. In: Woodfield DR, Matthew C, editors. Ryegrass endophyte: an essential New Zealand symbiosis. Palmerston North: New Zealand Grassland Association. Grassland Research and Practice Series 7. p. 57–62.
- Easton HS, Lane GA, Tapper BA. 1993. Ergovaline in endophyte-infected ryegrass pastures. Proceedings of a symposium on Mycotoxicoses of Grassland Farming, Ruakura, September 1993; Abstract in NZ Veterinary Journal 41: 214.
- Easton HS, Lane GA, Tapper BA, Keogh RG, Cooper BM, Blackwell M, Anderson M, Fletcher LR. 1996. Ryegrass endophyte-related heat stress in cattle. Proceedings of the New Zealand Grassland Association. 57:37–41.
- Easton HS, Latch GCM, Tapper BA, Ball OJ-P. 2002. Ryegrass host genetic control of concentrations of endophyte-derived alkaloids. Crop Science. 42:51–57.
- Easton HS, Lee CK, Fitzgerald RD. 1994. Tall fescue in Australia and New Zealand. New Zealand Journal of Agricultural Research. 37:405–417.
- Edwards GR, Lucas RJ, Johnson MR. 1993. Grazing preference for pasture species by sheep is affected by endophyte and nitrogen fertility. Proceedings of the New Zealand Grassland Association. 55:137–141.
- Eerens JPJ. 1996. The ryegrass endophyte in a cool moist environment [Unpublished PhD thesis]. Lincoln: Lincoln University.
- Eerens JPJ, Lucas RJ, Easton HS, White JGH. 1998. Influence of the ryegrass endophyte (Neotyphodium lolii) in a cool moist environment. I. Pasture production. New Zealand Journal of Agricultural Research. 41:39–48.
- EFSA Panel on Contaminants in the Food Chain (CONTAM). 2012. Scientific opinion on ergot alkaloids in food and feed. EFSA Journal. 10(7):2798 (158 pp).
- Eisemann, JH, Huntington, GB, Williamson, M, Hanna, M, Poore, M. 2014. Physiological responses to known intake of ergot alkaloids by steers at environmental temperatures within or greater than their thermoneutral zone. Frontiers in Chemistry. 2: Article 96. doi:https://doi.org/10.3389/fchem.2014.00096.
- Faichney GJ, Barry TN. 1986. Effects of mild heat exposure and suppression of prolactin secretion on gastro-intestinal tract function and temperature regulation in sheep. Australian Journal of Biological Science. 39:153–157.
- FAO (Food and Agriculture Organization). 2004. Worldwide Regulations for Mycotoxins in food and feed in 2003. Rome, Italy: FAO Food and Nutrition Paper 81.
- Filipov NM, Thompson FN, Hill NS, Dawe DL, Stuedemann JA, Price JC, Smith CK. 1998. Vaccination against ergot alkaloids and the effect of endophyte-infected fescue seed-based diets on rabbits. Journal of Animal Science. 76:2456–2463.
- Finch SC, Fletcher LR, Babu JV. 2012. The evaluation of endophyte toxin residues in sheep fat. New Zealand Veterinary Journal. 60:56–60.
- Finch SC, Munday JS, Sprosen JM, Bhattarai S. 2019. Toxicity studies of chanoclavine in mice. Toxins. 11(5):249.
- Finch SC, Pennell CL, Kerby JWF, Cave VM. 2016. Mice find endophyte-infected seed of tall fescue unpalatable – implications for the aviation industry. Grass and Forage Science. 71:659–666.
- Finch SC, Thom ER, Babu JV, Hawkes AD, Waugh CD. 2013. The evaluation of fungal endophyte toxin residues in milk. New Zealand Veterinary Journal. 61:11–17.
- Finch SC, Vlaming JB, Sutherland BL, van Koten C, Mace WJ, Fletcher LR. 2018. Ergovaline does not alter the level of ryegrass staggers induced by lolitrem B. New Zealand Veterinary Journal. 66:93–97.
- Fink-Gremmels J. 2005. Mycotoxins in forages. In: Diaz DE, editor. The mycotoxin blue book. Nottingham: Nottingham University Press; p. 249–268.
- Fleetwood DJ. 2007. Molecular characterisation of the EAS gene cluster for ergot alkaloid biosynthesis in Epichloë endophytes of grasses [Doctoral dissertation]. Massey University.
- Fleetwood DJ, Scott B, Lane GA, Tanaka A, Johnson RD. 2007. A complex ergovaline gene cluster in Epichloë endophytes of grasses. Applied and Environmental Microbiology. 73:2571–2579.
- Fletcher LR. 1993a. Grazing ryegrass/endophyte associations and their effect on animal health and performance. In: Hume DE, Latch GCM, Easton HS, editors. Proceedings of the 2nd International Symposium on Acremonium/Grass Interactions: Plenary Papers. Palmerston North, New Zealand: AgResearch; p. 115–120.
- Fletcher LR. 1993b. Heat stress in lambs grazing ryegrass with different endophytes. In: Hume DE, Latch GCM, Easton HS, editors. Proceedings of the 2nd International Symposium on Acremonium/grass interactions. Palmerston North, New Zealand: Agresearch; p. 114–118.
- Fletcher LR. 1998. Endophyte on the dairy farm, is it a problem? 75th Jubilee New Zealand Veterinary Association Conference. Dairy cattle and industry. p. 119–132.
- Fletcher LR. 1999. Non-toxic endophytes in ryegrass and their effect on livestock health and production. In: Woodfield DR, Matthew C, editors. Ryegrass endophyte: an essential New Zealand symbiosis. Palmerston North: New Zealand Grassland Association. Grassland Research and Practice Series 7. p. 133–139.
- Fletcher LR. 2005. Managing ryegrass-endophyte toxicoses. In: Roberts CA, West CP, Spiers DE, editors. Neotyphodium in cool-season grasses. Ames (IA): Blackwell Publishing; p. 229–241.
- Fletcher LR. 2012. Novel endophytes in New Zealand grazing systems: The Perfect Solution or a Compromise? In: Young CA, Aiken GE, McCulley RL, Strickland JR, Schardl CL, editors. Epichloae, endophytes of cool season grasses: implications, utilization and biology. Ardmore (OK): Samuel Roberts Noble Foundation; p. 5–13.
- Fletcher R. 1982. Observations of ryegrass staggers in weaned lambs grazing different ryegrass pastures. New Zealand Journal of Experimental Agriculture. 10:203–207.
- Fletcher LR, Barrell GK. 1984. Reduced liveweight gains and serum prolactin levels in hoggets grazing ryegrasses containing Lolium endophyte. New Zealand Veterinary Journal. 32:139–140.
- Fletcher LR, Easton HS. 1997. The evaluation and use of endophytes for pasture improvement. In: Bacon CW, Hill NS, editors. Proceedings 3rd Neotyphodium/Grass Interactions. New York & London: Plenum Press; p. 209–227.
- Fletcher LR, Finch SC, Sutherland BL, deNicolo G, Mace WJ, van Koten C, Hume DE. 2017. The occurrence of ryegrass staggers and heat stress in sheep grazing ryegrass endophyte associations with diverse alkaloid profiles. New Zealand Veterinary Journal. 65:232–241.
- Fletcher LR, Harvey IC. 1981. An association of a Lolium endophyte with ryegrass staggers. New Zealand Veterinary Journal. 29:185–186.
- Fletcher LR, Lane GA, Baird DB, Davies E. 2001. Seasonal variations of alkaloid concentrations in two perennial ryegrass endophyte associations. In: Paul VH, Dapprich PD, editors. Proceedings 4th International Neotyphodium/Grass Interactions Symposium. Soest, Germany: Universitat Paderborn; p. 535–541.
- Fletcher LR, Markham LJ, White SR. 1994. Endophytes an heat tolerance in lambs grazing perennial ryegrass. Proceedings of the New Zealand Grassland Association. 56:265–270.
- Fletcher LR, Popay AJ, Tapper BA. 1991. Evaluation of several lolitrem-free endophyte/ perennial ryegrass combinations. Proceedings of the New Zealand Grassland Association. 53:215–219.
- Fletcher LR, Sutherland BL. 1993a. Flystrike and faecal contamination in lambs grazing endophyte infected ryegrasses. In: Hume DE Latch, Easton HS GCM, editors. Proceedings of the 2nd International Symposium on Acremonium/Grass Interactions. Palmerston North, New Zealand: AgResearch; p. 122–124.
- Fletcher LR, Sutherland BL. 1993b. Liveweight change in lambs grazing perennial ryegrasses with different endophytes. In: Hume DE, Latch GCM, Easton HS, editors. Proceedings of the 2nd International Symposium on Acremonium/Grass Interactions. Palmerston North, New Zealand: AgResearch; p. 125–127.
- Fletcher LR, Sutherland BL, Fletcher CG. 1997. Effect of ambient and black-globe temperature on plasma prolactin levels in ewes grazing endophyte-free and endophyte-infected ryegrass. In: Bacon CW, Hill NS, editors. Symposium on Neotyphodium/Grass Interactions. New York and London: Plenum Press; p. 425–427.
- Fletcher LR, Sutherland BL, Fletcher CG. 1999. The impact of endophyte on the health and productivity of sheep grazing ryegrass based pastures. In: Woodfield DR, Matthew C, editors. Ryegrass endophyte – an essential New Zealand Symbiosis. Napier, New Zealand: New Zealand Grassland Association; p. 11–17. Grassland Research and Practice Series 7.
- Fletcher LR, Sutherland B, Fletcher CG, Easton HS. 1996. The impact of endophyte toxins on the health of grazing sheep – an evolving story. Proceedings Second Pan pacific Veterinary Conference: sheep. p. 31–42.
- Flieger M, Stodůlková E, Wyka SA, Černý J, Grobárová V, Píchová K, Novák P, Man P, Kuzma M, Cvak L, et al. 2019. Ergochromes: heretofore neglected side of ergot toxicity. Toxins (Basel). 11:439. doi:https://doi.org/10.3390/toxins11080439.
- Fuchs B, Krischke M, Mueller MJ, Krauss J. 2017. Plant age and seasonal timing determine endophyte growth and alkaloid biosynthesis. Fungal Ecology. 2:52–58.
- Gadberry MS, Denard TM, Spiers DE, Piper EL. 2003. Effects of feeding ergovaline on lamb performance in a heat stress environment. Journal of Animal Science. 81:1538–1545.
- Gallagher RT, Hawkes AD, Steyn PS, Vleggaar R. 1984. Tremorgenic neurotoxins from perennial ryegrass causing ryegrass staggers Disorder of livestock: structure elucidation of lolitrem B. Journal of the Chemical Society, Chemical Communications. 614–616.
- Garner GB, Cornell CN. 1978. Fescue foot in cattle. In: Wyllie TD, Morehouse LG, editors. Mycotoxic fungi, mycotoxins, mycotoxicoses: an encyclopedic handbook. Vol. 2. New York: Marcel Dekker; p. 45–62.
- Garner GB, Rottinghaus GE, Cornell CN, Testereci H. 1993. Chemistry of compounds associated with endophyte/grass interaction: ergovaline- and ergopeptine-related alkaloids. Agriculture, Ecosystem & Environment. 44(1):65–80.
- Garthwaite I, Sprosen J, Briggs L, Collin R, Towers N. 1994. Food quality on the farm: immunological detection of mycotoxins in New Zealand pastoral agriculture. Food and Agricultural Immunology. 6:123–129.
- Gerhards N, Neubauer L, Tudzynski P, Li S-M. 2014. Biosynthetic pathways of ergot alkaloids. Toxins. 6:3281–3295. doi:https://doi.org/10.3390/toxins6123281.
- Goodman AA. 1952. Fescue foot in cattle in Colorado. Journal of the American Veterinary and Medical Association. 121:289–290.
- Grauwiler J, Schon H. 1973. Teratological experiments with ergotamine in mice rats and rabbits. Teratology. 7:227–236.
- Griffith RW, Grauwiler J, Hodel C, Leist KH, Matter B. 1978. Toxicologic considerations. In: Berde B, Schild HO, editors. Ergot alkaloids and related compounds. Handbook of experimental Pharmacology 49. Berlin, Heidelberg: Springer Verlag; p. 805–838. NY. Chapter 12.
- Guerre P. 2015. Ergot alkaloids produced by endophytic fungi of the genus Epichloë. Toxins. 7:773–790. doi:https://doi.org/10.3390/toxins7030773.
- Guggisberg H. 1954. Mutterkorn. Vom Gift zum Heilstoff. Basel: Verlag S. Karger.
- Hannah SM, Paterson JA, Williams JE, Kerley MS, Miner JL. 1990. Effects of increasing dietary levels of endophyte-infected tall fescue seed on diet digestibility and ruminal kinetics in sheep. Journal of Animal Science. 68:1693–1701.
- He L, Hatier JH, Card SD, Matthew C. 2013. Endophyte-infection reduces leaf dehydration of ryegrass and tall fescue plants under moderate water deficit. Proceeding of the New Zealand Grasslands Association. 75:151–156.
- Hemken RW, Boling JA, Bull LS, Hatton RH, Buckner RC, Bush LP. 1981. Interaction of environmental temperature and anti-quality factors on the severity of summer fescue toxicosis. Journal of Animal Science. 52:710–714.
- Hemken RW, Jackson JA Jr, Boling JA. 1984. Toxic factors in tall fescue. Journal of Animal Science. 58:1011–1016.
- Henry MLE, Kemp S, Clarke IJ, Dunshea FR, Leury BJ. 2015. Production and physiological effects of perennial ryegrass alkaloids under thermoneutral conditions in Merinos. Animal Production Science. doi:https://doi.org/10.1071/AN14731.
- Hiatt EE, Hill NS. 1997. Neotyphodium coenophialum mycelial protein and herbage mass effects on ergot alkaloid concentration in tall fescue. Journal of Chemical Ecology. 23:2721–2736.
- Hill NS. 1993. Physiology of plant- endophyte interactions: implications and use of endophytes in plant breeding. In: Hume DE, Latch GCM, Easton HS, editors. Proceedings of the 2nd International Symposium on Acremonium/Grass Interactions: plenary papers. Palmerston North, New Zealand: Agresearch; p. 161–169.
- Hill NS, Bouton JH, Thompson FN, Hawkins L, Hoveland CS, McCann MA. 2002. Performance of tall fescue germplasms bred for high- and low-ergot alkaloids. Crop Science. 42:518–523.
- Hill NS, Parrott WA, Pope DD. 1991. Ergopeptine alkaloid production by endophytes in a common tall fescue genotype. Crop Science. 31:1545–1547.
- Hill NS, Thompson FN, Stuedemann JA, Dawe DL, Hiatt EE. 2000. Urinary excretion as diagnostic tool for fescue toxicosis in cattle. Journal of Veterinary Diagnostic Investigation. 12:210–217.
- Hill NS, Thompson FN, Stuedemann JA, Rottinghaus GW, Ju HJ, Dawe DL, Hiatt EE III. 2001. Ergot alkaloid transport across ruminant gastric tissues. Journal of Animal Science. 79:542–549.
- Hoveland CS. 1993. Importance and economic significance of the Acremonium endophytes to performance of animals and grass plant. Agricultural Ecosystems and Environment. 44:3–12.
- Hoveland CS, Haaland RL, King CC Jr, Anthony WB, McGuire JA, Smith LA, Grimes HW, Holliman JL. 1980. Steer performance on AP-2 phalaris and Kentucky 31 tall fescue pasture. Agronomy Journal. 72:375–377.
- Hovermale JT, Craig AM. 2001. Correlation of ergovaline and lolitrem B levels in endophyte infected perennial ryegrass (Lolium perenne). Journal of Veterinary Diagnostic Investigation. 13:323–327.
- Hume DE, Cooper BM, Panckhurst KA. 2009. The role of endophyte in determining the persistence and productivity of ryegrass, tall fescue and meadow fescue in Northland. Proceedings of the New Zealand Grassland Association. 71:145–150.
- Hume DE, Hickey MJ, Tapper BA. 2007a. Degradation of endophyte alkaloids in field-dried cut ryegrass herbage. In: Popay AJ, Thom ER, editors. Proceedings of the 6th International Symposium on Fungal Endophytes of Grasses, Christchurch, New Zealand. Grassland Research and Practice Series 13; p. 167–170.
- Hume DE, Popay AJ, Barker DJ. 1993. Effect of Acremonium endophyte on growth of ryegrass and tall fescue under varying levels of soil moisture and Argentine stem weevil attack. In: Hume DE, Latch GCM, Easton HS, editors. Proceedings of the 2nd International Symposium on Acremonium/Grass Interactions. Palmerston North, New Zealand: AgResearch; p. 161–164.
- Hume DE, Ryan DL, Cooper BM, Popay AJ. 2007b. Agronomic performance of AR37-infected ryegrass in northern New Zealand. Proceedings of the New Zealand Grassland Association. 69:201–205.
- Hume DE, Ryan GD, Gibert A, Helander M, Mirlohi A, Sabzalian MR. 2016. Epichloë fungal endophytes for grassland ecosystems. In: Lichfouse E, editor. Sustainable agriculture reviews 19. Switzerland: Springer International Publishing; p. 233–305.
- Hunt MG, Rasmussen S, Newton PCD, Parsons AJ, Newman A. 2005. Near-term impacts of elevated CO2, nitrogen and fungal endophyte-infection on Lolium perenne L. Growth, chemical composition and alkaloid production. Plant Cell and Environment. 28:1345–1354.
- Jensen R, Deem AW, Knaus D. 1956. Fescue lameness in cattle 1. Experimental production of the disease. American Journal of Veterinary Research. 17:196–201.
- Ji H, Fannin F, Klotz J, Bush L. 2014. Tall fescue seed extraction and partial purification of ergot alkaloids. Frontiers in Chemistry. 2: Article1 10. doi:https://doi.org/10.3389/fchem.2014.00110.
- Johnson L, de Bonth A, Briggs L, Caradus J, Finch S, Fleetwood D, Fletcher L, Hume D, Johnson R, Popay A, et al. 2013. The exploitation of epichloae endophytes for agricultural benefit. Fungal Diversity. 60:171–188. doi:https://doi.org/10.1007/s13225-013-0239-4.
- Kadzere CT, Murphy MR, Silanikove N, Maltz E. 2002. Heat stress in lactating dairy cows: a review. Livestock Production Science. 77:59–91.
- Kallenbach RL, Bishop-Hurley GJ, Massie MD, Rottinghaus GE, West CP. 2003. Herbage mass, nutritive value, and ergovaline concentration of stockpiled tall fescue contribution of the Missouri agricultural experiment station and the Arkansas agricultural experiment station. Crop Science. 43:1001–1005.
- Kaur N, Lunn K, Lloyd-West C, De Bonth ACM, Mace WJ. 2014. Characterizing the effect of two different drying techniques (oven and freeze drying) on the concentration of alkaloids in endophyte-infected herbage. In: Roche JR, editor. Proceedings of the 6th Australasian Dairy Science Symposium. Australasian Dairy Science Symposium. Hamilton, New Zealand; p. 266–269.
- Kelsey JM. 1958. Damage in ryegrasses by Hyperodes griseus Hust. New Zealand Journal of Agricultural Research. 1:790–795.
- Keogh RG, Blackwell MB, Shepherd P. 1999. Performance of dairy cows grazing pastures with or without ergovaline and lolitrem B in Northland. Proceedings of the New Zealand Society of Animal Production. 59:254–257.
- Keogh RG, Tapper BA, Fletcher RH. 1996. Distributions of the fungal endophyte Acremonium lolii, and of the alkaloids lolitrem B and peramine, within perennial ryegrass. New Zealand Journal of Agricultural Research. 39:121–127.
- Klotz JL. 2015. Activities and effects of ergot alkaloids on livestock physiology and production. Toxins. 7:2801–2821. doi:https://doi.org/10.3390/toxins7082801.
- Klotz JL, Bush LP, Smith DL, Schafer WD, Smith LL, Arrington BC, Strickland JR. 2007. Ergovaline-induced vasoconstriction in an isolated bovine lateral saphenous vein bioassay. Journal of Animal Science. 85:2330–2336.
- Klotz JL, Bush LP, Smith DL, Schafer WD, Smith LL, Vevoda AO, Craig AM, Arrington BC, Strickland JR. 2006. Assessment of vasoconstrictive potential of d-lysergic acid using an isolated bovine lateral saphenous vein bioassay. Journal of Animal Science. 84:3167–3175.
- Klotz JL, Kirch BH, Aiken GE, Bush LP, Arrington BC, Strickland JR. 2008. Effects of selected combinations of tall fescue alkaloids on the vasoconstrictive capacity of fescue naïve bovine lateral saphenous veins. Journal of Animal Science. 86:1021–1028.
- Klotz JL, Kirch BH, Aiken GE, Bush LP, Strickland JR. 2010. Contractile response of fescue-näive bovine lateral saphenous veins to increasing concentrations of tall fescue alkaloids. Journal of Animal Science. 88:408–415.
- Klotz JL, Nicol AM. 2016. Ergovaline, an endophytic alkaloid. 1. Animal physiology and metabolism. Animal Production Science. 56:1761–1774.
- Knight TN. 1990. Reproductive wastage, a guide for fundamental research: a New Zealand perspective. In: Oldham GM, Martin GR, Purvis IW, editors. Reproductive physiology of the Merino sheep: concepts and consequences. Chapter 5. Perth: The University of Western Australia; p. 11–21.
- Kramer R, Keogh RG, McDonald MF. 1999. Effects of ergovaline in endophyte-infected tall fescue on ewe fertility. Proceedings of the New Zealand Society of Animal Production. 59:263–265.
- Lane GA. 1999. Chemistry of endophytes: patterns and diversity. In: Woodfield DR, Matthew C, editors. Ryegrass endophyte – an essential New Zealand symbiosis. Napier, New Zealand: New Zealand Grassland Association, Grassland Research and Practice Series 7; p. 85–94.
- Lane GA, Ball OJ-P, Davies E, Davidson C. 1997a. Ergovaline distribution in perennial ryegrass naturally infected with endophyte. In: Bacon CW, Hill NS, editors. Proceeding of 3rd Neotyphodium/Grass Interactions. New York: Plenum Press; p. 65–67.
- Lane GA, Christensen MJ, Miles CO. 2000. Coevolution of fungal endophytes with grasses: the significance of secondary metabolites. In: Bacon CW, White JF, editors. Microbial endophytes. New York: Marcel Dekker; p. 341–388.
- Lane GA, Tapper BA, Davies E. 1999. Ergot alkaloids additional to ergovaline in endophyte infected perennial ryegrass and tall fescue in New Zealand. In: Woodfield DR, Matthew C, editors. Ryegrass endophyte: an essential New Zealand symbiosis. Palmerston North: New Zealand Grassland Association. Grassland Research and Practice Series 7; p. 95–100.
- Lane GA, Tapper BA, Davies E, Christensen MJ, Latch GCM. 1997c. Occurrence of extreme alkaloid levels in endophyte-infected perennial ryegrass, tall fescue and meadow fescue. In: Bacon CW, Hill NS, editors. Proceedings 3rd International Neotyphodium/Grass Interactions Symposium. New York and London: Plenum Press; p. 433–436.
- Lane GA, Tapper BA, Davies E, Hume DE, Latch GCM, Barker DJ, Easton HS, Rolston MP. 1997b. Effect of growth conditions on alkaloid concentrations in perennial ryegrass naturally infected with endophyte. In: Bacon CW, Hill NS, editors. Proceedings 3rd International Neotyphodium/Grass Interactions Symposium. New York and London: Plenum Press; p. 179–182.
- Larson BT, Harmon DL, Piper EL, Griffis LM, Bush LP. 1999. Alkaloid binding and activation of D2 dopamine receptors in cell culture. Journal of Animal Science. 77:942–947.
- Larson BT, Samford MD, Camden JM, Piper EL, Kerley MS, Paterson JA, Turner JT. 1995. Ergovaline binding and activation of D2 dopamine receptors in GH4ZR7 cells. Journal of Animal Science. 73:1396–1400.
- Latch GCM. 1994. Influence of Acremonium endophytes on perennial grass improvement. New Zealand Journal of Agricultural Research. 37:311–318.
- Layton DL, Fletcher LR, Litherland AJ, Scannell MG, Sprosen J, Hoogendoorn CJ, Lambert MG. 2004. Effect of ergot alkaloids on liveweight gain and urine lysergol level in ewe hoggets and heifers. Proceedings of the New Zealand Society of Animal Production. 64:192–196.
- Lea K, Smith L, Gaskill C, Coleman R, Smith SR. 2014. Ergovaline stability in tall fescue based on sample handling and storage methods. Frontiers in Chemistry. 2: Article76. doi:https://doi.org/10.3389/fchem.2014.00076.
- Lean IJ. 2001. Association between feeding perennial ryegrass (Lolium perenne cultivar Grasslands impact) containing high concentrations of ergovaline, and health and productivity in a herd of lactating dairy cows. Australian Veterinary Journal. 79:262–264.
- Lewin L. 1962. Gifte und Vergiftungen, Lehrbuch der Toxicologie. 5. Aufl., Karl F. Haug Verlag, Ulm/Donau.
- LIC and DairyNZ. 2019. New Zealand Dairy Statistics 2018–19. www.lic.co.nz/about/dairy-statistics, or www.dairynz.co.nz/dairystatistics.
- Logan CM, Edwards GR, Kerr GA, Williams S. 2015. Ryegrass staggers and liveweight gain of ewe lambs and hoggets grazing four combinations of perennial ryegrass and strains of endophyte. Proceedings of the New Zealand Society of Animal Production. 75:175–178.
- Lorenz N, Haarmann T, Pazoutov S, Jung M, Tudzynski P. 2009. The ergot alkaloid gene cluster: functional analyses and evolutionary aspects. Phytochemistry. 70:1822–1832.
- Lowe KF, Bowdler TM, Hume DE, Casey ND, Tapper BA. 2008. The effect of endophyte on the performance of irrigated perennial ryegrasses in subtropical Australia. Australian Journal of Agricultural Research. 59:567–577.
- Lyons PC, Plattner RD, Bacon CW. 1986. Occurrence of peptide and clavine ergot alkaloids in tall fescue grass. Science. 232:487–489.
- Ma Y, Meyer KG, Afzal D, Agena EA. 1993. Isolation and quantification of ergovaline from Festuca arundinacea (tall fescue) infected with the fungus Acremonium coenophialum by high-performance capillary electrophoresis. Journal of Chromatography. 652:535–538.
- Maag DD, Tobiska JW. 1956. Fescue lameness in cattle. II ergot alkaloids in tall fescue grass. American Journal of Veterinary Research. 17:202–204.
- Mace WJ, Lunn KL, Kaur N, Lloyd-West CM. 2014. Variation in the expression of ergot alkaloids between individual tillers of perennial ryegrass. Frontiers in Chemistry. 2: Article 107. doi:https://doi.org/10.3389/fchem.2014.00107.
- Malinowski DP, Belesky DP. 2000. Adaptations of endophyte-infected cool-season grasses to environmental stresses: mechanisms of drought and mineral stress tolerance. Crop Science. 40:923–940.
- McCollough SF, Piper EL, Moubarak A, Johnson ZB, Petroski RJ, Flieger M. 1994. Effect of tall fescue ergot alkaloids on peripheral blood flow and serum prolactin in steers. Journal of Animal Science. 72(Suppl. 1):144. (Abstract).
- McCulley RL, Bush LP, Carlisle AE, Ji H, Nelson JA. 2014. Warming reduces tall fescue abundance but stimulates toxic alkaloid concentrations in transition zone pastures of the U.S. Frontiers in Chemistry. 2: Article 88. doi:https://doi.org/10.3389/fchem.2014.00088.
- Miles CO, Lane GA, Di Menna ME, Garthwaite I, Piper EL, Ball OJP, Latch GCM, Allen JM, Hunt MB, Bush LP, et al. 1996. High levels of ergonovine and lysergic acid amide in toxic Achnatherum inebrians accompany infection by an Acremonium-like endophytic fungus. Journal of Agriculture and Food Chemistry. 44:1285–1290.
- Miles CO, Munday SC, Wilkins AL, Ede RM, Towers NR. 1994. Large-scale isolation of lolitrem B and structure determination of lolitrem E. Journal of Agricultural and Food Chemistry. 42:1488–1492.
- Ministry for the Environment. 2008. Climate change effects and impacts assessment: A guidance manual for local government in New Zealand. 2 Projections of future New Zealand climate change. https://www.mfe.govt.nz/publications/climate-change/climate-change-effects-and-impacts-assessment-guidance-manual-local-51.
- Ministry for the Environment. 2018. Climate change projections for New Zealand: atmosphere projections based on simulations from the IPCC fifth assessment. 2nd ed. Wellington: Ministry for the Environment.
- Moate PJ, Williams SRO, Grainger C, Hannah MC, Mapleson D, Auldist MJ, Greenwood JS, Popay AJ, Hume DE, Mace WJ, Wales WJ. 2012. Effects of wild-type, AR1 and AR37 endophyte-infected perennial ryegrass on dairy production in Victoria, Australia. Animal Production Science. 52:1117–1130.
- National Institute of Weather and Atmospheric Research. 2018. Annual climate summary 2018. https://niwa.co.nz/sites/niwa.co.nz/files/2018_Annual_Climate_Summary-NIWA.pdf.
- Nicol AM, Klotz JL. 2015. The difficulties in reviewing ergovaline. Proceedings of the New Zealand Society of Animal Production. 75:179.
- Nicol AM, Klotz JL. 2016. Ergovaline, an endophytic alkaloid. 2. Intake and impact on animal production, with reference to New Zealand. Animal Production Science. 56:1775–1786.
- Nicoll CS, Bryant GD. 1972. Physiological and immunological properties of prolactins. Proceedings 4th Tenovus Workshop: Prolactin and Carcinogenesis. p. 28.
- Oliver JW. 1997. Physiological manifestations of endophyte toxicosis in ruminant and laboratory species. In: Bacon CW, Hill NS, editors. Proceedings 3rd International Neotyphodium/Grass Interactions Symposium. New York and London: Plenum Press; p. 311–346.
- Oliver JW. 2005. Pathophysiologic response to endophyte toxins. In: Roberts CA, West CP, Spiers DE, editors. Neotyphodium in cool-season grasses. Ames: Blackwell Publishing; p. 291–304.
- Oliver JW, Schultze AM, Rohrbach BW, Fribourg HA, Ingle T, Waller JC. 2000. Alterations in hemograms and serum biochemical analytes of steers after prolonged consumption of endophyte-infected tall fescue. Journal of Animal Science. 78:1029–1035.
- Oliver JW, Strickland JR, Linnabary RD, Abney LK, Bailey EM, Waller JC, Fribourg HA. 1994. Peripheral blood vessel (bovine) reactivity to the toxins of endophyte-infected tall fescue. Proceedings Tall Fescue Toxicosis workshop, SERAIEG-8, Atlanta (GA). p. 51–53.
- Ortel I, Keller U. 2009. Combinatorial assembly of simple and com-plex D-lysergic acid alkaloid peptide classes in the ergot fungus Claviceps purpurea. Journal of Biological Chemistry. 284:6650–6660.
- Osborn TG, Schmidt SP, Marple DN, Rahe CH, Steenstra JR. 1992. Effect of consuming fungus-infected and fungus-free tall fescue and ergotamine tartrate on selected physiological variables of cattle in environmentally controlled conditions. Journal of Animal Science. 70:2501–2509.
- Panaccione DG, Beaulieu WT, Cook D. 2014. Bioactive alkaloids in vertically transmitted fungal endophytes. Functional Ecology. 28:299–314.
- Panaccione DG, Cipoletti JR, Sedlock AB, Blemmings KP, Shardl CL, Machodo C, Seidel GE. 2006a. Effects of ergot alkaloids on food preference and satiety in rabbits, as assessed with gene-knockout endophytes in perennial ryegrass (Lolium perenne). Journal of Agricultural and Food Chemistry. 54:4582–4587.
- Panaccione D, Kotcon J, Schardl C, Johnson R, Morton J. 2006b. Ergot alkaloids are not essential for endophytic fungus-associated population suppression of the lesion nematode, Pratylenchus scribneri, on perennial ryegrass. Nematology. 8:583–590.
- Panaccione DG, Wang J, Young CA, Schardl CL, Scott B, Damrongkool P. 2001. Elimination of ergovaline from a grass-Neotyphodium endophyte symbiosis by genetic modification of the endophyte. Proceedings of the National Academy of Sciences of the United States of America. 98:12820–12825.
- Parkheava. 1979. Zit. nach: Wolff J, Richter WIF, Spann B. 1995. Mutterkornalkaloide in der Milch? VDLUFA-Schriftenreihe, Kongressband 18: 515–524.
- Patterson CG, Potter DA, Fannin FF. 1991. Feeding deterrency of alkaloids from endophyte-infected grasses to Japanese beetle larvae. Entomologia Experimentalis et Applicata. 61:285–289.
- Pennell CGL, Rolston MP. 2003. Effect of grass endophyte associations on feeding of Canada geese (Branta canadensis). Proceedings of the New Zealand Grassland Association. 65:239–244.
- Pennell CGL, Rolston MP, Baird D, Hume DE, McKenzie CM, Card SD. 2017b. Using novel-grass endophyte associations as an avian deterrent. New Zealand Plant Protection. 70:255–264.
- Pennell CGL, Rolston MP, De Bonth A, Simpson WR, Hume DE. 2010. Development of a bird deterrent fungal endophyte in turf tall fescue. New Zealand Journal of Agricultural Research. 53:145–150.
- Pennell CGL, Rolston MP, Van Koten C, Hume DE, Card SD. 2017a. Reducing bird numbers at New Zealand airports using a unique endophyte product. New Zealand Plant Protection. 70:224–234.
- Persoon CH. 1798. Sphaeria typhina. Icones et descriptiones fungorum minus cognitorum, Lipsiae (1798), p. 21.
- Peters CW, Grigsby KN, Aldrich CG, Paterson JA, Lipsey RJ, Kerley MS, Garner GB. 1992. Performance, forage utilization, and ergovaline consumption by beef cows grazing endophyte fungus-infected tall fescue, endophyte fungus-free tall fescue, or orchard grass pastures. Journal of Animal Science. 70:1550–1561.
- Petroski RJ, Powell RG, Clay K. 1992. Alkaloids of Stipa robusta (sleepygrass) infected with an Acremonium endophyte. Natural Toxins. 1:84–88.
- Piper EL, Gadberry MS, Denard TM, Johnson Z, Flieger M. 1997. Effect of feeding ergovaline and ergine on growing rats. In: Bacon CW, Hill NS, editors. Proceedings 3rd International Neotyphodium/Grass Interactions Symposium. New York: Plenum Press; p. 437–439.
- Popay AJ, Ball OJ-P. 1998. The development of fungal endophytes as a pest management tool for New Zealand grasslands. In: Proceedings 6th Australasian Applied Entomological Research Conference. Vol. 1. p. 374–371.
- Popay AJ, Gerard PJ. 2007. Cultivar and endophyte effects on root aphid, Aploneura lentisei, in perennial ryegrass. New Zealand Plant Protection Journal. 60:223–227.
- Popay AJ, Hume DE. 2011. Endophytes improve ryegrass persistence by controlling insects. Pasture Persistence – Grassland Research and Practice Series. 15:149–156.
- Popay AJ, Hume DE, Davis KL, Tapper BA. 2003. Interactions between endophyte (Neotyphodium spp.) and ploidy in hybrid and perennial ryegrass cultivars and their effects on Argentine stem weevil (Listronotus bonariensis). New Zealand Journal of Agricultural Research. 46:311–319.
- Popay AJ, Prestidge RA, Rowan DD, Dymock JJ. 1990. The role of Acremonium lolii mycotoxins in insect resistance of perennial ryegrass (Lolium perenne). In: Quisenberry SS, Joost RE, editors. Proceedings of the International Symposium Acremonium/Grass Interactions. Baton Rouge: Louisiana Agricultural Experimental Station; p. 44–46.
- Popay AJ, Rowan DD. 1994. Endophytic fungi as mediators of plant-insect interactions. In: Bemays EA, editor. Insect-Plant interactions. Vol. 5. Boca Raton, Florida, USA: CRC Press; p. 83–103.
- Popay AJ, Wyatt RT. 1995. Resistance to Argentine stem weevil in perennial ryegrass infected with endophytes producing different alkaloids. Proceedings of New Zealand Plant Protection Conference. 48:229–236.
- Porter JK. 1995. Analysis of endophyte toxins: fescue and other grasses toxic to livestock. Journal of Animal Science. 73:871–880.
- Porter JK, Bacon CW, Robbins JD, Betowski D. 1981. Ergot alkaloid identification in Clavicipitaceae systemic fungi of pasture grasses. Journal of Agricultural and Food Chemistry. 29:653–657.
- Porter JK, Robbins JD, Norred WP, Garner GB, Bacon CW. 1985. Serum prolactin and brain catecholamine metabolite depression in rats administrated extracts of endophyte-infected fescue. Journal of Agriculture and Food Chemistry. 33:34–36.
- Prestidge RA. 1993. Causes and control of perennial ryegrass staggers in New Zealand. Agriculture, Ecosystems and Environment. 44:283–300.
- Prestidge RA, Ball OJP. 1993. The role of endophytes in alleviating plant biotic stress in New Zealand. In: Hume DE, Latch GCM, Easton HS, editors. Proceedings of the 2nd International Symposium on Acremonium/Grass Interactions: plenary papers. Palmerston North, New Zealand: AgResearch; p. 141–151.
- Prestidge RA, Popay AJ, Ball OJP. 1994. Biological control of pastoral pests using Acremonium spp. endophytes. Proceedings of the New Zealand Grassland Association. 56:33–38.
- Purves D, Augustine GJ, Fitzpatrick D, Hall WC, LaMantia A-S, White, LE, Sunderland, MA. 2012. Neurotransmitters and their Receptors. In: Purves D, Augustine GJ, Fitzpatrick D, Hall WC, LaMantia A-S, White LE, Sunderland, MA, editors. Neuroscience. 5th ed. Sunderland (MA): Sinauer Associates, INC. Publishers. Chapter 6, p 109–140.
- Rasmussen S, Lane GA, Mace W, Parsons AJ, Fraser K, Xue H. 2012. The use of genomics and metabolomics methods to quantify fungal endosymbionts and alkaloids in grasses. Methods in Molecular Biology. 860:213–226.
- Rasmussen S, Parsons AJ, Bassett S, Christensen MJ, Hume DE, Johnson LJ, Johnson RD, Simpson WR, Stacke C, Voisey CR, et al. 2007. High nitrogen supply and carbohydrate content reduce fungal endophyte and alkaloid concentration in Lolium perenne. New Phytologist. 173:787–797.
- Ravagnolo O, Misztal I, Hoogenboom G. 2000. Genetic component of heat stress in dairy cattle, development of heat index function. Journal of Dairy Science. 83:2120–2125.
- Realini CE, Duckett SK, Hill NS, Hoveland CS, Lyon BG, Sackmann JR, Gillis MH. 2005. Effect of endophyte type on carcass traits, meat quality, and fatty acid composition of beef cattle grazing tall fescue. Journal of Animal Science. 83:430–439.
- Reed KFM, Mace WJ, Walker LV, Fletcher LR. 2016. Endophyte metabolites associated with perennial ryegrass toxicosis. Animal Production Science. 56:895–907.
- Reed KFM, Nie ZN, Walker LV, Kearney G. 2011. Fluctuations in the concentration of ergovaline and lolitrem b produced by the wild-type endophyte Neotyphodium lolii in perennial ryegrass Lolium perenne. Pasture. Animal Production Science. 51:1098–1108.
- Repussard C, Zbib N, Tardieu D, Guerre P. 2014. Ergovaline and lolitrem b concentrations in perennial ryegrass in field culture in southern France: distribution in the plant and impact of climatic factors. Journal of Agriculture and Food Chemistry. 62:12707–12712.
- Rever PM. 1993. Prolactin and immunomodulation. American Journal of Medicine. 95:637–644.
- Rhodes MT, Paterson JA, Kerley MS, Garner HE, Laughlin MH. 1991. Reduced blood flow to peripheral and core body temperatures in sheep and cattle induced by endophyte-infected tall fescue. Journal of Animal Science. 69:2033–2043.
- Roberts CA, Spiers DE, Karr A, Benedict HR, Sleper DA, Eichen PA, West CP, Piper EL, Rottinghaus GE. 2002. Use of a rat model to evaluate tall fescue seed infected with introduced strains of Neotyphodium coenophialum. Journal of Agricultural and Food Chemistry. 50:5742–5745.
- Robinson SL, Panaccione DG. 2015. Diversification of ergot alkaloids in natural and modified fungi. Toxins. 7:201–218. doi:https://doi.org/10.3390/toxins7010201.
- Rothlin E. 1933. Über enterale Resoption von Mutterkornalkaloiden und deren spezifische Wirkungweise auf das Kreislaufsystem. Naunyn-Schmiedebergs Archiv für experimentelle Pathologie und Pharmakologie. 171:555–591.
- Rottinghaus GE, Garner GB, Cornell CN, Ellis JL. 1991. HPLC method for quantitating ergovaline in endophyte-infested tall fescue: seasonal variation of ergovaline levels in stems with leaf sheaths, leaf blades, and seed heads. Journal of Agriculture and Food Chemistry. 39:112–115.
- Rowan DD, Shaw GJ. 1987. Detection of ergopeptine alkaloids in endophyte-infected perennial ryegrass by tandem mass spectrometry. New Zealand Veterinary Journal. 35:197–198.
- Rowan DD, Tapper BA, Sergejew NL, Latch GCM. 1990. Ergopeptine alkaloids in endophyte infected ryegrass and fescues in New Zealand. In: Quisenberry S, Joost RE, editors. Proceedings of the 1st International Symposium on Acremonium/Grass Interactions. New Orleans: Louisiana Agricultural Experiment Station; p. 97–99.
- Roylance JT, Hill NS, Agee CS. 1994. Ergovaline and peramine production in endophyte-infected tall fescue: independent regulation and effects of plant and endophyte genotype. Journal of Chemical Ecology. 20:2171–2183.
- Sampson K. 1933. The systemic infection of grasses by Epichloë typhina (Pers.) Tul. Transactions of the British Mycological Society. 18:30–47.
- Schardl CL, Panaccione DG, Tudzynski P. 2006. Ergot alkaloids–biology and molecular biology. The Alkaloids: Chemistry and Biology. 63:45–86.
- Schardl CL, Scott B, Florea S, Zhang D. 2009. Epichloë endophytes: Clavicipitaceous Symbionts of grasses. In: Deising VH, editor. Plant Relationships, 2nd Edition The Mycota. Berlin Heidelberg: Springer-Verlag; p. 275–306.
- Schardl CL, Young CA, Hesse U, Amyotte SG, Andreeva K, Calie PJ, Fleetwood DJ, Haws DC, Moore N, Oeser B, et al. 2013. Plant symbiotic fungi as chemical engineers: Multi-genome analysis of the Clavicipitaceae reveals dynamics of alkaloid loci. PLoS Genetics. 9:e1003323. doi:https://doi.org/10.1371/journal.pgen.1003323.
- Schiff PL. 2006. Ergot and its alkaloids. American Journal of Pharmaceutical Education. 70: Article 98. doi:https://doi.org/10.5688/aj700598.
- Schmidt SP, Hoveland CS, Clark EM, Davis ND, Smith LA, Gumis HW, Holliman JL. 1982. Association of an endophytic fungus with fescue toxicity in steers fed Kentucky 31 tall fescue seed or hay. Journal of Animal Science. 55:1259–1263.
- Schmidt SP, Osborn TG. 1993. Effects of endophyte infected tall fescue on animal performance. Agriculture, Ecosystems & Environment. 44:233–262.
- Schultz CL, Lodge-Ivey SL, Bush LP, Craig AM, Strickland JR. 2006. Effects of initial and extended exposure to an endophyte-infected tall fescue seed diet on faecal and urinary excretion of ergovaline and lysergic acid in mature geldings. New Zealand Veterinary Journal. 54:178–184.
- Schumann B, Lebzien P, Ueberschar K-H, Dänicke S. 2009. Effects of the level of feed intake and ergot contaminated concentrate on ergot alkaloid metabolism and carry over into milk. Molecular and Nutrition Food Research. 53:931–938.
- Seman DH, Stuedemann JA, Breedlove DL, Wilkinson SR, Belesky DP, Thompson FN, Stewart FP. 1990. Differences in grazing behaviour of steers consuming endophyte infected and non-infected tall fescue. In: Quisenberry SS, Joost RE, editors. Proceedings of the International Symposium Acremonium/Grass Interactions. Baton Rouge: Louisiana Agricultural Experimental Station; p. 267–272.
- Shappell NW, Smith DJ. 2005. Ergovaline movement across Caco-2 cells. In Vitro Cellular and Developmental Biology. 41:245–251.
- Shelby RA, Kelley VE. 1992. Detection of ergot alkaloids from Claviceps species in agricultural products by competitive ELISA using a monoclonal antibody. Journal of Agriculture and Food Chemistry. 40:1090–1092.
- Shelby RA, Olsovska J, Havlicek V, Flieger M. 1997. Analysis of ergot alkaloids in endophyte infected tall fescue by liquid chromatography/ electrospray ionisation mass spectrometry. Journal of Agricultural and Food Chemistry. 45:4674–4679.
- Siegel MR, Bush LP. 1996. Defensive chemicals in grass-fungal endophyte associations. In: Romeo JT, Saunders JA, Barbosa P, editors. Phytochemical diversity and Redundancy in Ecological interactions. New York: Plenum Press; p. 81–119.
- Siegel MR, Dahlman DL, Bush LP. 1989. The role of endophytic fungi in grasses: new approaches to biological control of pests. In: Leslie AR, Metcalf RL, editors. Integrated pest management for Turfgrasses and Ornamentals. Washington CG: US-EPA; p. 169–186.
- Siegel MR, Latch GCM, Bush LP, Fannin FF, Rowan DD, Tapper BA, Bacon CW, Johnson MC. 1990. Fungal endophyte-infected grasses: alkaloid accumulation and aphid response. Journal of Chemical Ecology. 16:3301–3315.
- Solomons RN, Oliver JW, Linnabray RD. 1989. Dorsal pedal vein of cattle: Reactivity to selected alkaloids associated with Acremonium coenophialum infected fescue grass. American Journal Veterinary Research. 50:235–238.
- Spiering MJ, Davies E, Tapper BA, Schmid J, Lane GA. 2002. Simplified extraction of ergovaline and peramine for analysis of tissue distribution in endophyte-infected grass tillers. Journal of Agricultural and Food Chemistry. 50:5856–5862.
- Spiers DE, Evans TJ, Rottinghaus GE. 2005. Interaction between thermal stress and fescue toxicosis: animal models and new perspectives. In: Roberts CA, West CP, Spiers DE, editors. Neotyphodium in cool-season grasses. Ames (IA): Blackwell Publishing; p. 243–270.
- Spiers DE, Zhang Q, Eichen PA, Rottinghaus GE, Garner GB, Ellersieck MR. 1995. Temperature-dependent responses of rats to ergovaline derived from endophyte-infected tall fescue. Journal of Animal Science. 73:1954–1961.
- Stamm MM, DelCurto T, Horney MR, Brandyberry SD, Barton RK. 1994. Influence of alkaloid concentration of tall fescue straw on the nutrition, physiology, and subsequent performance of beef steers. Journal of Animal Science. 72:1068–1075.
- Star P, Brooking T. 2006. Fescue to the rescue: Chewings fescue, paspalum, and the application of non-British experience to pastoral practice in New Zealand, 1880–1920. Agricultural History. 80:312–335.
- Stewart AV. 2006. Genetic origins of perennial ryegrass (Lolium perenne) for New Zealand pastures. In: Mercer CF, editor. Breeding for Success: Diversity in Action. Proceedings of the 13th Australasian Plant Breeding Conference; Apr 18–21; Christchurch, New Zealand. p. 11–20. doi:https://doi.org/10.2527/jas.2010-3478.
- Stewart A, Kerr G, Lissaman W, Rowarth J. 2014. Endophyte in ryegrass and tall fescue. In: Davis K, Casey M, editors. Pasture and forage plants for New Zealand Grassland Research and Practice series No 8. Fourth ed. Dunedin, New Zealand: New Zealand Grassland Association; p. 66–77.
- Strickland JR, Bailey EM, Abney LK, Oliver JW. 1996. Assessment of the mitogenic potential of the alkaloids produced by endophyte (Acremonium coenophialum)-infected tall fescue (Festuca arundinacea) on bovine vascular smooth muscle in vitro. Journal of Animal Science. 74:1664–1671.
- Strickland JR, Brown KR, Aiken GE, Klotz JL, Flythe MD. 2012. Ergot alkaloids: Toxicokenetics and vascular effects. In: Young CA, Aiken GE, McCulley RL, Strickland JR, Schardl CL, editors. Epichloae, endophytes of cool season grasses: Implications, Utilization and Biology. Ardmore (OK): Samuel Roberts Noble Foundation; p. 14–19.
- Strickland JR, Cross DL, Birrenkott GP, Grimes LW. 1994. Effect of ergovaline, loline, and dopamine antagonists on rat pituitary cell prolactin release in vitro. American Journal of Veterinary Research. 55:716–721.
- Strickland JR, Looper ML, Matthews JC, Rosenkrans CF, Flythe MD, Brown KR. 2011. BOARD-INVITED REVIEW: St. Anthony’s Fire in livestock: causes, mechanisms, and potential solutions. Journal of Animal Science. 89:1603–1626.
- Stuedemann JA, Breedlove DL, Pond KR, Belesky DD, Tate LP, Thompson FN, Wilkinson SR. 1989. Effect of endophyte (Acremonium coenophialum) infection of tall fescue and paddock exchange on intake and performance of grazing steers. Proceedings of the XVI International Grassland Congress. p. 1243–1244.
- Stuedemann JA, Hill NS, Thompson RA, Fayrer-Hosken RA, Hay WP, Dawe DH, Seman DH, Martin SA. 1998. Urinary and biliary excretion of ergot alkaloids from steers that grazed endophyte-infected tall fescue. Journal of Animal Science. 76:2146–2154.
- Stuedemann JA, Hoveland CS. 1988. Fescue endophyte: history and impact on animal agriculture. Journal of Production Agriculture. 1:39–44.
- Tapper BA, Latch GCM. 1999. Selection against toxin production in endophyte-infected perennial ryegrass. In: Woodfield DR, Matthew C, editors. Ryegrass endophyte: an essential New Zealand symbiosis. Palmerston North: New Zealand Grassland Association. Grassland Research and Practice Series No. 7; p. 107–111.
- TePaske MR, Powell RG, Clement SL. 1993. Analyses of selected endophyte-infected grasses for the presence of loline-type and ergot-type alkaloids. Journal of Agricultural and Food Chemistry. 41:2299–2303.
- Thom ER, Clark DA, Waugh CD. 1999. Endophyte and dairy production in New Zealand: experience at the Dairying Research Corporation. In: Woodfield DR, Matthew C, editors. Ryegrass endophyte: an essential New Zealand symbiosis. Palmerston North: New Zealand Grassland Association. Grassland Research and Practice Series 7; p. 39–44.
- Thom ER, Clark DA, Waugh CD, McCabe RJ, van Vught VT, Koch BJL. 1997. Effects of ryegrass endophyte and different white clover levels in pasture on milk production from dairy cows. In: Bacon CW, Hill NS, editors. Proceedings 3rd International Neotyphodium/Grass Interactions Symposium. New York and London: Plenum Press; p. 443–445.
- Thom ER, Popay AJ, Hume DE, Fletcher LR. 2012. Evaluating the performance of endophytes in farm systems to improve farmer outcomes – a review. Crop and Pasture Science. 63:927–943.
- Thom ER, Waugh CD, Minneé EMK, Waghorn GC. 2007. A new generation of ryegrass endophyte – the first results from dairy cows fed AR37. Proceedings of the 6th International Symposium on Fungal endophytes of Grasses. Grassland Research and Practice Series 13. p. 293–296.
- Thom ER, Waugh CD, Minneé EMK, Waghorn GC. 2013. Effects of novel and wild-type endophytes in perennial ryegrass on cow health and production. New Zealand Veterinary Journal. 61:87–97.
- Thompson FN, Hill NS, Dawe DL, Stuedeman JA. 1993. The effects of passive immunization against lysergic acid derivatives on serum prolactin in steers grazing endophyte-infected tall fescue. In: Hume DE, Latch GCM, Easton H, editors. Proceedings of the 2nd International Symposium on Acremonium/Grass Interactions. Palmerston North, New Zealand: AgResearch; p. 135–137.
- Thompson FN, Stuedemann JA. 1993. Pathophysiology of fescue toxicosis. Agriculture and Ecosystem Environment. 44:263–281.
- Tor-Agbidye J, Blythe LL, Craig AM. 2001. Correlation of endophyte toxins (ergovaline and lolitrem B) with clinical disease: fescue foot and perennial ryegrass staggers. Veterinary and Human Toxicology. 43:140–146.
- Ussher G. 2003. Northland’s pasture toxin project. New Zealand Large Herds Association Annual Conference. 34:62–64.
- Valentine SC, Bartsch BD, Carroll PD. 1993. Production and composition of milk by dairy cattle grazing high and low endophyte cultivars of perennial ryegrass. In: Hume DE, Latch GCM, Easton H, editors. Proceedings of the 2nd International Symposium on Acremonium/ Grass Interactions. Palmerston North, New Zealand: AgResearch; p. 138–141.
- van Zijll de Jong E, Dobrowolski MP, Sandford A, Smith KF, Willocks MJ, Spangenberg GC, Forster JW. 2008. Detection and characterisation of novel fungal endophyte genotypic variation in cultivars of perennial ryegrass (Lolium perenne L.). Australian Journal of Agricultural Research. 59:214–221.
- Varney DR, Kappes CJ, Jones SL, Newsome R, Siegel MR, Zavos PM. 1988. The effect of feeding tall fescue seed infected by Acremonium coenophialum on pregnancy and parturition in female rats. Comparative Biochemistry and Physiology Part C: Comparative Pharmacology. 89:315–320.
- Varney DR, Ndefru M, Jones SL, Newsome R, Siegel MR, Zavos PM. 1987. The effect of feeding endophyte infected tall fescue seed on reproductive performance in female rats. Comparative Biochemistry and Physiology Part C: Comparative Pharmacology. 87:171–175.
- Waller JC. 2009. Endophyte effects on cattle. In: Fribourg HA, Hannaway DB, West CP, editors. Tall fescue for the twenty-first century. Madison (WI): ASA, CSSA, and SSSA; p. 289–310.
- Wallner BM, Booth NH, Robbins JD, Bacon CW, Porter JK, Kiser TE, Wilson R, Johnson B. 1983. Effect of an endophytic fungus isolated from toxic pasture grass on serum prolactin concentrations in the lactating cow. American Journal of Veterinary Research. 44:1317–1322.
- Watson RH, Keogh RG, McDonald MF. 1999. Ewe reproductive performance and growth rate of suckling-lambs on endophyte-infected perennial ryegrass pasture. In: Woodfield DR, Matthew C, editors. Ryegrass endophyte: an essential New Zealand symbiosis. Palmerston North: New Zealand Grassland Association. Grassland Research and Practice Series No. 7; p. 19–26.
- West JW. 2003. Effects of heat-stress on production in dairy cattle. Journal of Dairy Science. 86:2131–2144.
- West JW, Mullinix BG, Bernard JK. 2003. Effects of hot, humid weather on milk temperature, dry matter intake, and milk yield of lactating dairy cows. Journal of Dairy Science. 86:232–242.
- Wheatley WM, Hume DE, Kemp HW, Monk MS, Lowe KF, Popay AJ, Baird DB, Tapper BA. 2003. Effects of fungal endophyte on the persistence and productivity of tall fescue at 3 sites in eastern Australia. In Solutions for a better environment. Proceedings of the 11th Australian Agronomy Conference. Australian Society of Agronomy/The Regional Institute: Gosford, NSW. www.regional.org.au/au/asa/2003/p/12/wheatley.htm.
- Widdup KH, Ryan DL. 1992. Forage potential of wild populations of perennial ryegrass collected from southern New Zealand farms. Proceedings of the New Zealand Grassland Association. 54:161–165.
- Wilson GF. 1975. The performance of dairy cattle grazing two varieties of tall fescue. Proceedings of the New Zealand Grasslanz Association. 36:200–208.
- Wolff J, Richter WIF, Spann B. 1995. Mutterkornalkaloide in der Milch? VDLUFA Schriftenreihe. Kongressband. 18:515–524.
- Woodburn OJ, Walsh JR, Foot JZ, Heazlewood PG. 1993. Seasonal ergovaline concentrations in perennial ryegrass cultivars of differing endophyte status. In: Hume DE, Latch GCM, Easton H, editors. Proceedings 2nd international symposium on Acremonium/ Grass Interactions. Ed. D.E. Hume, G.C.M. Latch & H.S. Easton. p. l00–102.
- Young CA, Schardl CL, Panaccione DG, Florea S, Takach JE, Charlton ND, Moore N, Webb JS, Jaromczyk J. 2015. Genetics, genomics and evolution of ergot alkaloid diversity. Toxins. 7:1273–1302. doi:https://doi.org/10.3390/toxins7041273.
- Zavos PM, Siegel MR, Grove RJ, Hemken RM, Varney DR. 1990. Effects of feeding endophyte-infected tall fescue seed on reproductive performance in male CD-1 mice by competitive breeding. Theriogenology. 33:653–660.
- Zavos PM, Varney DR, Hemken RM, Siegel MR, Bush LP. 1988. Fertilization rates and embryonic development in CD-1 mice fed fungal endophyte-infected tall fescue seed. Theriogenology. 30:461–468.
- Zbib N, Repussard C, Tardieu D, Guerre P. 2014a. Toxicité des mycotoxines produites par des champignons endophytes du genre Neotyphodium. Revue de Médecine Vétérinaire. 165:116–135.
- Zbib N, Repussard C, Tardieu D, Priymenko N, Domange C, Guerre P. 2014b. Ergovaline in tall fescue and its effect on health, milk quality, biochemical parameters, oxidative status, and drug metabolizing enzymes of lactating ewes. Journal of Animal Science. 92:5112–5123.
- Zbib N, Repussard C, Tardieu D, Priymenko N, Domange C, Guerre P. 2015. Toxicity of endophyte-infected ryegrass hay containing high ergovaline level in lactating ewes. Journal of Animal Science. 93:4098–4109.