ABSTRACT
Nitrogen (N) is an essential nutrient for plant growth. In grazed pasture systems in New Zealand, N fertiliser is regularly applied to maintain high productivity of ryegrass (Lolium perenne L.)/white clover (Trifolium repens L.) pastures. Several decades of trials/research have established the scientific basis for N management and N fertiliser application in grass/clover pasture systems. However, despite, or perhaps because of the rich diversity of this work, it isn’t always easy to see the full picture of this research; the seminal research and sometimes more recent advances are not always easily discoverable. This paper therefore seeks to amalgamate and summarise some of the vast amount of research on N fertiliser use in grazed pasture systems undertaken in New Zealand over the last 50 years. It provides an overview of research, raising the awareness of key findings, and importantly providing a pathway for decision makers to find and access the research on N fertiliser use and management. It also assists in the identification of knowledge gaps for new avenues of enquiry that could improve the efficiency of N fertiliser use and reduce environmental impacts of N loss from grazed pasture systems.
Introduction
Nitrogen (N) fertiliser is widely used to ensure high productivity of grazed pasture systems in New Zealand and is considered to be integral to maintaining the economic viability of these systems (Journeaux et al. Citation2019). Nitrogen is an essential plant nutrient required for grass/clover pasture (Hay and Porter Citation2006). Historically, ryegrass (Lolium perenne L.)/white clover (Trifolium repens L.) pasture in New Zealand relied on N supplied from symbiotic N fixation from white clover to support its productive potential (Syers Citation1982; Walker and Ludecke Citation1982), with measured N fixation rates of 50–300 kg N ha−1 yr−1 (Hoglund et al. Citation1979; Ledgard et al. Citation2001). However, research has established that even well managed grass/clover pastures remain deficient in N for much of the year (Carran Citation1978; Field and Ball Citation1978; Steele Citation1982), possibly because the average white clover content of New Zealand pastures rarely exceeds 20% (on an annual pasture production basis) (Ettema and Ledgard Citation1992). This is too low to support the N requirements of grass/clover pasture, which Thomas (Citation1992) estimated should be between 20% and 45% of the pasture sward. As a result, it has been shown that New Zealand grass/clover pasture responds readily to the application of N fertiliser (Steele et al. Citation1981; Roberts and Thomson Citation1989).
Several decades of N fertiliser trials and research has established the scientific basis for N management and fertiliser application in grass/clover pasture-based systems in New Zealand. This research has been published in numerous scientific articles and technical reports addressing a wide range of issues relevant to N fertiliser application and management. In addition, there have been reviews of the research findings relevant to specific aspects of managing N fertiliser, such as quantifying pasture N response (Ball and Field Citation1982; O’Connor Citation1982; Ledgard Citation1989; Sun et al. Citation2008), biological N fixation (Ledgard and Steele Citation1992; Sun et al. Citation2008), N losses from soil (de Klein et al. Citation2001; Cameron et al. Citation2013), and the application of technology to improve N fertiliser use (Saggar et al. Citation2013; Di and Cameron Citation2018). Further, organisations such as the Fertiliser Association of New Zealand (FANZ) and DairyNZ have produced guidelines and technical reports for land managers based on these research findings (Shepherd and Lucci Citation2011).
Despite, or perhaps because of this rich diversity of work, it is not always easy to see the full picture of N fertiliser research; the original research and more recent advances, published in both scientific articles and technical reports aren’t always easily discoverable. Further, other aspects haven’t been recently reviewed (e.g. plant growth regulators, interaction of N fertiliser and legumes).
The aim of this paper therefore is to amalgamate and summarise some of the published New Zealand based research on N fertiliser use in grazed pasture-based agricultural systems over the last 50 years. This is timely given the increasing attention and scrutiny N fertiliser use in grazed systems is receiving in New Zealand by the general public as well as local and central government. We will seek to provide an overview of N research, raising the awareness of key research findings, providing pathways for decision makers to find and access existing N research, and allowing the identification of gaps in our knowledge for new avenues of enquiry. Because of the vastness of the topic, the paper is intentionally constrained in its scope. The focus is primarily on the direct effects of application of N fertiliser in mainly grass/clover pasture systems, although still acknowledging and highlighting the indirect effects that are intertwined with the application of N fertiliser. It is also restricted to pasture productivity (kg dry matter (DM) kg−1 N fertiliser applied), and not animal productivity.
Nitrogen fertiliser use in grazed pasture-based systems in New Zealand
In many temperate regions, intensive pasture-based systems have relied on production from pure grass swards, with a dependance on heavy and continued inputs of inorganic N fertiliser (Field and Ball Citation1978). In contrast, intensive mixed grass/clover pasture-based systems in New Zealand have relied on N supplies from symbiotic N fixation, particularly white clover (Ball et al. Citation1979a). Several reasons have been suggested for this, although the most important was the success of grass/clover-based pastures enabling a high level of production for grazing ruminants (Syers Citation1982). However, a combination of relatively strong prices for agricultural products and a worldwide oversupply of affordable cheap N fertiliser in the mid-1960s and again in the early 1970s, and the construction of an ammonia urea plant at Kapuni in South Taranaki in 1982 coincided with a steady increase in N fertiliser use in New Zealand (Rogers and Little Citation1982) (). The increase was notable in grass/clover pasture systems during the 1980s, prompted by an increasing amount of research that showed pasture responded readily to the application of N fertiliser (Steele et al. Citation1981; Syers Citation1982; Ball Citation1989; Roberts and Thomson Citation1989).
Figure 1. Nitrogen (N) fertiliser consumption in New Zealand between 1970–2021 (sourced from Rogers and Little (Citation1982) and the Fertiliser Association of New Zealand) and cow numbers between 1974–2021 (sourced from Livestock Improvement Corporation Limited).
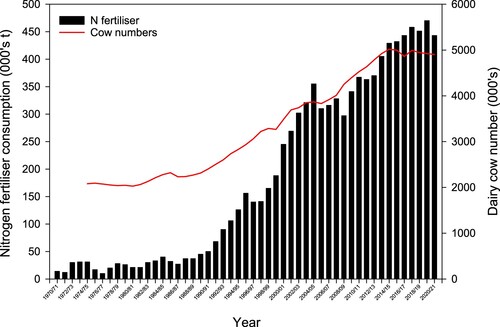
The large increase in dairy cow numbers in New Zealand since the 1990s (Livestock Improvement Corporation Limited, DairyNZ Limited Citation2021) accelerated the use of N fertiliser (). This also coincided with the arrival of clover root weevil (Sitona obsoletus) that led to an even greater reliance on the use of N fertiliser (Barratt et al. Citation1996). Nitrogen fertiliser was initially used to enhance the establishment of new pasture, and then during spring or autumn to extend pasture growth during the cool season (Monaghan et al. Citation2005; Catto and Roberts Citation2021). More recently, the pattern of N fertiliser use on dairy farms has changed, with N being applied all-year-round following the cows’ grazing rotation (Chapman et al. Citation2018a). Although interestingly, there were examples N fertiliser (10–15 kg N ha−1) being applied after each grazing by some farmers in the early 1990s (Gazzard and Bint Citation1992). In sheep and beef systems, N use has also increased (Stats Citation2021), albeit slower due to lower pasture response to N (kg of DM kg−1 N). Total N inputs from synthetic fertiliser on sheep and beef farms are relatively low at around 14 kg N ha−1 yr−1 for a whole-farm New Zealand average (Beef + Lamb New Zealand Citation2020). On these farms, N fertiliser use has predominantly targeted crop and specialty areas of the farm, although increasingly it is also being used in autumn and spring to support feed deficits (Lambert et al. Citation2012; Catto and Roberts Citation2021).
A range of ammonium-containing and forming compounds are currently used as N fertilisers in grass/clover pasture systems in New Zealand. These include ammonium sulphate ((NH4)2SO4), urea ((NH2)2CO), mono-ammonium phosphate (NH4H2PO4) and diammonium phosphate ((NH4)2HPO4) (Stats Citation2021). In dairy and sheep and beef systems in 2019, urea (253, 373 t/yr) was the main N fertiliser used in New Zealand, with lesser amounts of diammonium phosphate (33, 117 t/yr) and ammonium sulphate (16, 500 t/yr) (Stats Citation2021). Currently, it is estimated that the dairy sector uses 63% of New Zealand’s total N fertiliser, with sheep and beef, deer, arable and horticulture estimated to use the remaining 28%, 1%, 6% and 2%, respectively (Fertiliser Association of New Zealand Citation2020).
Quantifying pasture nitrogen fertiliser response
Although early research demonstrated the beneficial effect from using N fertiliser in grazed pasture systems in New Zealand (e.g. Hudson and Woodcock Citation1931; O’Connor Citation1961; Scott Citation1963; During and Weeda Citation1968), it was described by Syers (Citation1982) as ‘not substantial or consistent’. It wasn’t until the late 1960s/early 1970s that a structured programme of research evaluating the response of N fertiliser in pasture systems commenced in New Zealand. This was driven largely by the small but increasing use of N fertiliser in pasture systems in New Zealand. This reportedly stimulated an increasing number of questions/inquiries to government scientists by Dairy Board consulting officers and MAF advisors relating to its use, for which at the time there was only a very limited knowledge base (Syers Citation1982; Ball Citation1989).
Seasonality of nitrogen fertiliser application
The initial focus of research was on quantifying the response of pasture to N fertiliser (kg DM kg−1 of N fertiliser applied) and the factors which effect the variability in N response. Of particular interest was the response of pasture to N fertiliser applied to offset seasonal N deficiency in grass/clover pasture (O’Connor and Gregg Citation1971). This was prompted by promising earlier findings reported by Lynch (Citation1953, Citation1967) and During and Weeda (Citation1968) of enhanced N response from late winter/early spring applications of N fertiliser to pasture.
As result, between 1969 and 1974 a series of national field trials was established examining response rates of N fertiliser and the effect of time of application in late winter/spring (Spring) and late summer/autumn (Autumn) to mixed grass-clover pastures (O’Connor Citation1982). In brief, 405 experiments were conducted over a six-year period at a series of lowland sites. Each experiment consisted of four rates of lime ammonium nitrate (now termed calcium ammonium nitrate) equivalent to 0, 25, 50 and 100 kg of N fertiliser ha−1, replicated six times. Trials were established over consecutive months in spring and autumn on high producing grass/clover pastures. On all trials, single applications of N fertiliser were applied to pasture that had previously been trimmed to a height of two to three cm and allowed to recover for a few days. Trial areas were fenced to exclude stock and DM yield measurements were made when pasture regrowth was about 15 cm (usually a four to six-week interval).
The results from these trials have been reported in numerous papers, workshops and conferences (e.g. O’Connor and Gregg Citation1971; O'Connor and Cumberland Citation1973; O’Connor Citation1973; O'Connor Citation1974; Williams and Paterson Citation1983), and comprehensively summarised by O'Connor (Citation1982). The trials confirmed the findings of earlier research that response to spring application of N fertiliser was generally higher and more reliable than N applied in autumn. For instance, for spring N fertiliser application (25 kg N ha−1), the average pasture response (over three months) ranged from 3.6 to 24.1 kg DM kg−1 N (mean 13.1 kg DM kg−1 N). For autumn N applications the average pasture response ranged from 2.8 to 20.0 kg DM kg−1 N (mean 7.0 kg DM kg−1 N). It was also found that there was considerable variability due to differences between sites and seasons and there were regional differences in the responsiveness of pasture to N fertiliser (). However, there didn’t appear to be any clear reason for the differences. For example, there were no consistent differences between warmer and cooler regions, although responses were minimal when soils were very dry (O’Connor Citation1982).
Table 1. Pasture dry matter (DM) increases (kg ha−1) for spring applied nitrogen (N) fertiliser and nitrogen use efficiency (kg DM kg−1 N applied) (adapted from O’Connor Citation1982).
Numerous studies undertaken since the national trial series on lowland sites across New Zealand confirmed the earlier findings, reporting that pasture response from late winter/early spring application of N fertiliser was generally higher and more reliable than N applied in autumn (Harris et al. Citation1973; Holmes and Wheeler Citation1973; Steele et al. Citation1981; Ball and Field Citation1982; Roberts and Thomson Citation1989; Smith et al. Citation2000). For example, Feyter et al. (Citation1985) measured pasture production in five harvests in consecutive months from March to August over two years after the application of N fertiliser (0, 25, 50, 100 kg ha−1). Pasture response to N fertiliser in harvest one was greatest from the August application ().
Figure 2. Effect of nitrogen (N) fertiliser (50 kg ha−1) applied in March, June or August on daily pasture production, expressed as difference from the control. Arrows indicates the month of N fertiliser application (adapted from Feyter et al. Citation1985).
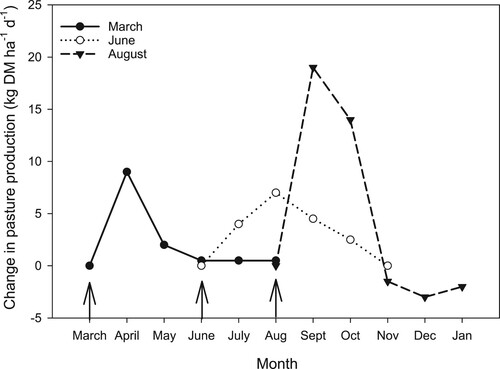
Hill country pastures are also chronically N deficient and readily respond to N fertiliser (Lambert and Clark Citation1986; Gillingham et al. Citation1998). A review of pasture response to the application of N fertiliser for a series of hill country trials by Ball et al. (Citation1982) showed that N response efficiencies ranged from 7 to 33 kg DM kg−1 N. Several other studies confirmed hill country pastures are responsive to N fertiliser and pasture growth could be increased at most times of the year (Sherlock and O' Connor Citation1973; Ball et al. Citation1976; Ball et al. Citation1978; Luscombe Citation1980; Lambert et al. Citation2003). However, as at lowland pasture sites, the greatest increase in pasture growth was often found from late winter/early spring application of N fertiliser (Luscombe Citation1979, Citation1980; Ball et al. Citation1982; Ledgard et al. Citation1983; Parker et al. Citation1989; Gillingham et al. Citation2007; Citation2003; Puha et al. Citation2008; Hoogendoorn et al. Citation2017). Sherlock and O' Connor (Citation1973) measured the pasture response to N fertiliser (25, 50, 100 kg N ha−1) applied monthly between April and October at a hill country trial in Te Kuiti. They found the best percentage and total DM responses were obtained from early spring applications of N, and least in winter, with no response to N applied in June ().
Figure 3. Pasture production from the application of nitrogen (N) fertiliser applied over the April to October (adapted from Sherlock and O' Connor Citation1973).
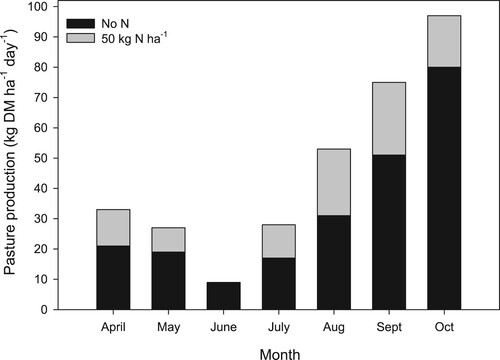
Korte (Citation1988) found average response rates for N fertiliser (25, 50, 100 kg N ha−1) applied to five East Coast Hill country farms in the North Island varied four-fold depending on the month of fertiliser application. Response rates were 6.7, 8.6, 3.8, 12.0, 12.3, 11.6 and 8.5 kg DM kg−1 N from N fertiliser applied in March, April, May, June, July, August and September, respectively. Large N fertiliser response rates (17–34 kg extra DM kg−1 N applied) were obtained by Lambert and Clark (Citation1986) when urea (up to 50 kg N ha−1) was applied in late autumn, and pasture production response extended through winter into spring. Lower response rates were found by Ledgard et al. (Citation1983) at the Whatawhata Hill Country Research Station, where urea (up to 90 kg N ha−1) applied to pasture in autumn resulted in < 8 kg of extra DM kg−1 N and < 20 kg extra DM kg−1 N from N applied in early spring. At the Ballantrae trial site, Lambert et al. (Citation2003) found that the application of 400 kg N ha−1 (eight applications of 50 kg N ha−1) to historically low, medium and high fertility (P status) areas of hill country increased annual pasture production from 9.2 to 17.1 t DM ha−1 averaged across the fertility blocks over two years of measurement. Similar N fertiliser response rates of 18–22 kg extra DM kg−1 N applied were observed across high fertility sites. Significant responses to N fertiliser occurred in all four seasons of each year, with highest responses recorded in spring and summer.
In general, trials have highlighted that better N responses in late winter/early spring are due to plants being N deficient. This is because from late autumn to early spring, lower temperatures restrict clover growth and soil microbial activity, and therefore lower N fixation and soil N mineralisation, resulting in less N available for the grass (Mitchell and Lucanus Citation1962; Field and Ball Citation1978), along with drainage during the winter removing plant available N. In contrast, the poorer response in late summer/autumn is due to limited soil moisture, and also higher soil mineral N concentrations and a higher proportion of clover supplying N to the pasture (Ball and Field Citation1982; Keeney and Gregg Citation1982; O’Connor Citation1982).
Although there are some exceptions (e.g. Korte Citation1988), often there is no or only a low response to N fertiliser applied in mid-winter as noted by Feyter et al. (Citation1985) and Sherlock and O' Connor (Citation1973). This is because N uptake in grass/clover pasture is very temperature sensitive, and only readily occurs in ryegrass once the soil temperature exceeds about 4°C (Hoglund Citation1980). For example, in a trial undertaken at Kirwee in Canterbury, Hoglund (Citation1980) showed that despite repeated applications of N fertiliser from mid-June onwards, there was no pasture growth response until 15 August when the minimum soil temperature was above 4°C.
Strategic application of nitrogen fertiliser
The findings from the series of national N fertiliser response trials and subsequent research highlighted that the application of N fertiliser had the potential to be used as a management tool for manipulating seasonal pasture growth. This means that assuming pasture deficits are identified early enough in the season, the application of N fertiliser can be used ‘strategically’ to increase pasture production in grazed grass/white clover systems during parts of the year to overcome or reduce the foreseeable deficits in animal feed supply (Field and Ball Citation1978; Steele et al. Citation1981).
This has been demonstrated by Roberts et al. (Citation1992) who investigated the effect of strategic N fertiliser application on pasture growth in a three-year trial in South Taranaki. There is a disconnect between pasture growth and feed requirements for dairy cows, resulting in periods of the year where there is a feed deficit (). Roberts et al. (Citation1992) suggested the application N fertiliser (40 kg of N ha−1) (period A), could help increase pasture growth to bridge a late winter/early spring feed deficit, and the application of N fertiliser (60 kg N ha−1 period B), could help maximise the amount of pasture production during spring, some of which could then be conserved and fed back to animals over the late summer/autumn period ().
Figure 4. Pasture dry matter production and cow feed requirements and timing of strategic application of nitrogen (N) fertiliser at a trial site in south Taranaki (adapted from Roberts et al. Citation1992).
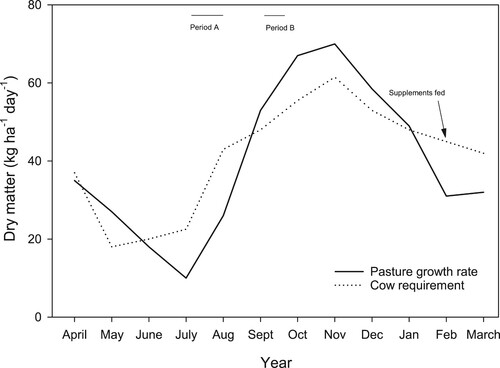
Rate of nitrogen fertiliser
The rate at which N fertiliser (kg N ha−1) is applied has a large effect not only on grass/clover pasture production, but also N fertiliser use efficiency (kg DM kg−1 N). Typically, as the rate of N fertiliser applied increases, the lower the pasture N response (). This was observed in the series of national N fertiliser trials, where, as the rate of N applied in spring from a single application increased from 25 to 100 kg N ha−1, the pasture DM yield increased (kg ha−1) but the relative pasture response (kg DM kg−1 N) declined on average from 12 to 7 kg DM kg−1 N, respectively (O’Connor Citation1982) (). This was consistent for N fertiliser applied in both the spring and autumn. For example, at one of the national N trials noted above, Williams and Paterson (Citation1983) measured pasture yield responses to N fertiliser at two sites in the Maruia Valley in the spring, and one site near Hokitika and one in the Grey Valley in the autumn over two years. While significant pasture yield responses from the application of up to 100 kg N ha−1 were measured for all three sites, the application of 25 kg N ha−1 produced 67–86% of the maximum yield, whereas 50 kg N ha−1 produced 77–98%, indicating only a modest advantage in using higher rates of N fertiliser (Williams and Paterson Citation1983).
Figure 5. Effect of rate of nitrogen (N) fertiliser applied on pasture response in the Waikato (adapted from Roberts et al. Citation1992).
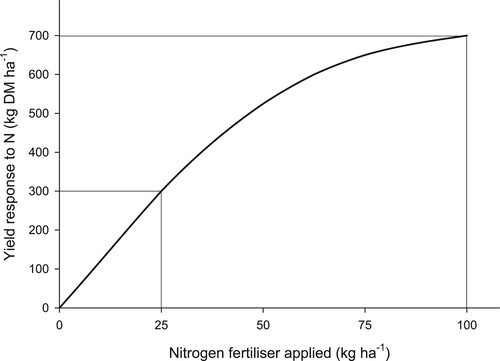
Other studies have reported similar findings (Sherlock and O' Connor Citation1973; Ball et al. Citation1978; Steele et al. Citation1981; Ball and Field Citation1982; Ledgard and Saunders Citation1982; Eltilib and Ledgard Citation1988; Ledgard Citation1989; Gillingham et al. Citation2007). In a series of trials in Gisborne-East Coast, a region not represented in the national N trials, Korte (Citation1988) showed the pasture response to N fertiliser decreased from 10.0 to 8.1 kg DM kg−1 N as N fertiliser applied to an old established pasture increased from 25 to 100 kg ha−1. In Taranaki, Roberts and Thomson (Citation1989) measured DM production at 28-day intervals from plots that received 12 rates of N fertiliser (0–100 kg N ha−1 in 10 kg increments and 125 and 150 kg N ha−1) applied in early September in each of two years. They found that the efficiency of response declined from 10 to 4 (kg DM kg ha−1 N) as the N application rate increased. Using average grass/clover pasture DM yield data from trials undertaken in the Waikato region obtained from a national N trial database (Rajendram et al. Citation2009), Shepherd and Lucci (Citation2012) showed the response of pasture to N fertiliser began to decrease at N fertiliser rates above 60 kg N ha−1 (depending on conditions). Feyter et al. (Citation1985) reported that although pasture DM yields were still highest when 100 kg N ha−1 of N was applied, the efficiency of N was higher at lower N fertiliser application rates (i.e. 25–50 kg N ha−1) ().
Table 2. Total response to nitrogen (N) fertiliser applied in March to August. Values in brackets show efficiency of the N applied (kg DM kg−1 N) (adapted from Feyter et al. Citation1985).
Because of the reported decline in N response efficiency at higher rates of N fertiliser application, and importantly the negative effect on clover growth (discussed later in the paper), a maximum single application rate of 50 kg N ha−1 is often generally recommended (Roberts et al. Citation1992; Anon Citation2008).
Frequency of nitrogen fertiliser application
Trials indicate no advantage in applying smaller, regular amounts of N fertiliser compared to larger amounts less often on total pasture production. Penno (Citation1993) set up a short-term grazing trial where pasture plots received either a single 50 kg N ha−1 application of N fertiliser in September or five 10 kg N ha−1 applications after grazing. It was found the single 50 kg N ha−1 treatment produced 28% higher net herbage accumulation between 22 September and 12 November (). The five 10 kg N ha−1 treatment produced 37% higher net herbage accumulation between 12 November and 11 January. However, despite a different pattern of accumulation, at the end of the trial there was no difference in total pasture production between the N treatments. In a more recent study, Ley (Citation2020) compared the pasture response to 25 kg N ha−1 of N fertiliser applied either once per month or 6.25 kg N ha−1 once per week to grass/clover field plots between September and June. Both treatments produced similar pasture DM yields and pasture quality throughout the growing season.
Figure 6. Average pasture mass resulting from 50 kg N ha−1 applied 10 September (50 × 1), and 5 × 10 kg N ha−1 applied between 10 September and 18 December (adapted from Penno Citation1993).
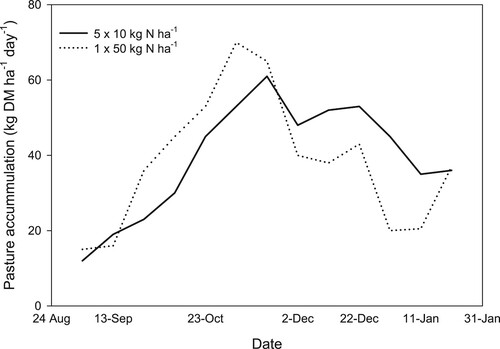
Form of nitrogen fertiliser type on pasture production
As reported, there are a range of different types of N fertiliser used in New Zealand. The consensus is there is little difference in N response between different forms of N fertiliser (e.g. ammonium-containing and forming compounds, and nitrate) applied in pasture-based systems (Ball Citation1970; During Citation1972; Roberts et al. Citation1992). This assumes any other nutrients in some N fertiliser (e.g. sulphur in ammonium sulphate and phosphorus in diammonium phosphate) are compensated for when comparing between N only fertiliser (e.g. urea). Ball and Field (Citation1982), for example, compared the effect of three N fertilisers (in multiple applications totalling 360 kg N ha−1) on pasture production and found no significant difference between the form of N applied ().
Table 3. Pasture response to three different forms of nitrogen (N) fertiliser (adapted from Ball and Field Citation1982) applied in multiple applications at a total of 360 kg N ha−1 yr−1.
A possible exception is when N fertiliser is applied to pasture at low soil temperatures. Craighead et al. (Citation1997) reported that nitrate-based fertilisers (calcium ammonium nitrate, ammonium sulphate nitrate) were more effective than urea when applied in early spring to cold soils (3–5°C) at two trial sites in South Canterbury, although there was inconsistency in response between the three years of the trial. Craighead et al. (Citation1997) suggests the low soil temperatures inhibit soil biological activity and the nitrification of ammonium fertiliser to nitrate. Although temperature may be an important factor (Hoglund Citation1980), studies often find that when ryegrass is supplied with equal amounts of ammonium and nitrate N, both forms of N are taken up at almost identical rates (Bailey Citation1998).
Residual effect of nitrogen fertiliser
The uptake of N by the grass component of a mixed pasture sward is generally more rapid than the growth response. This occurs where conditions restrict grass growth (e.g. cold, wet soils) or when the interval between N fertiliser application and harvest is insufficient to fully allow N uptake (O’Connor Citation1982). Potentially this can result in a build-up of N in the soil and lead to a positive residual (or carry over) effect on pasture response.
O’Connor (Citation1982) summarised the size of the residual effect from 114 trials that applied 25 kg N ha−1 of fertiliser monthly in the spring and in the autumn, and then measured the pasture response over three pasture cuts. Although there was variability, the percentage of the total pasture response occurring in the first harvest after N application tended to be lower from a June/July application of N fertiliser than from September/October (). While the total pasture response in the first harvest in February/March after N application tended to be higher than late autumn April/May N fertiliser application (). Overall, the better the pasture growth straight after N fertiliser application, the smaller the residual effect (O’Connor Citation1982).
Table 4. Residual effects of applying nitrogen (N) fertiliser (25 kg ha−1) to pasture in spring and autumn (adapted from O’Connor Citation1982).
Other studies have also identified a residual effect from N fertiliser applied to pastures at different rates and times of year across different regions of New Zealand (Sherlock and O' Connor Citation1973; Smith et al. Citation2000). At the Ballantrae hill country research station, Luscombe (Citation1979) measured a large and persistent residual N response (kg DM kg−1 N) in three cuts following an autumn/early winter application of N fertiliser (40, 80, 160 kg N ha−1) (). Ledgard et al. (Citation1988) reported that 50 kg N ha−1 of fertiliser applied to pasture either in May, June or August in a trial in the Waikato region resulted in an increase in pasture growth during the first two or three harvests, but then there was a subsequent reduction in pasture growth which lasted for two to three harvests. Roberts and Thomson (Citation1989) found a residual N fertiliser response in the second harvest of a pasture amended in July with N fertiliser (0, 50, 100 kg N ha−1) in a trial in South Taranaki, however by the third harvest there was negative N response. Feyter et al. (Citation1985) found a similar result where after a positive response to N in cuts one and two there was a negative response in cuts four and five. It has been suggested the depression in response could be a result of several factors including a decrease in the proportion and growth of white clover and a deficiency of other plant nutrients, particularly in low producing pasture (Ball and Field Citation1982; O’Connor Citation1982). In contrast, Matthew et al. (Citation1986) found that the residual pasture response from an autumn application of N fertiliser (30 and 60 kg ha−1) from urea was small and not statistically significant when measured 12 weeks after application. The results of these trials highlight that although pasture growth is improved immediately after applying N fertiliser, positive carry over effects can sometimes extend into the next growing period following grazing/harvesting. However, results are variable, and in some instances, there are negative responses in pasture growth in subsequent grazing/harvesting.
Table 5. Nitrogen (N) fertiliser response efficiencies (kg DM kg−1 N) calculated from cumulative pasture production (adapted from Luscombe Citation1979).
Cultivars
There has been a limited amount of research investigating the response of different ryegrass and white clover cultivars (discussed in a later section) to the application of N fertiliser. Bahmani et al. (Citation2001) compared the productivity of ‘Ellett’ ryegrass, a Mangere ecotype from which many modern cultivars have been bred, and ‘Grasslands Ruanui’ an older Hawke's Bay ecotype in a field trial that received 240 and 180 kg ha−1 of N fertiliser in year one and two respectively. Differences in productivity between cultivars were associated with differing plant morphologies and sward structure. It was suggested the morphological and productivity differences are linked to a greater leaf elongation rate in ‘Ellett’ than in ‘Grasslands Ruanui (Bahmani et al. Citation2000), and that the increased leaf elongation rate could explain the lower tiller density and larger tiller size of ‘Ellett’ Bahmani et al. (Citation2001). Ellett ryegrass produced greater average yields (13%) and consistently higher tiller weight and lower tiller density than Ruanui, although overall Ruanui produced more tillers than Ellett in responses to N fertiliser. This may be important because ryegrass cultivars with different morphologies and tillering responses to N fertiliser may require different grazing management to optimise their performance.
Slope and aspect
In hill country, pasture response to N fertiliser has often been improved on sunny (north facing) and steep slopes compared to shady (south-facing) gentler slopes (Ball et al. Citation1982; Ledgard et al. Citation1983; Gillingham et al. Citation1998; Lambert et al. Citation2003; Gillingham et al. Citation2007, Citation2008a, Citation2008b; Puha et al. Citation2008). Luscombe (Citation1979, Citation1980, Citation1981) undertook a series of trials at the Ballantrae hill country research station near Woodville that allowed a comparison of the response of pasture to different rates of N fertiliser (0, 10, 20, 40, 80, 160 kg N ha−1) in relation to aspect and slope of the site. Luscombe (Citation1979) found pasture response to N in spring was greater on cool, south-westerly aspect slopes than warm, north-easterly aspect slopes. However, in a follow up study, Luscombe (Citation1980) reported aspect appeared to have no significant effect on N response. Luscombe (Citation1981) also found there was no difference in pasture response from N fertiliser applied to flat (0–10°) and steep sites (10–25°). Gillingham et al. (Citation1998) investigated the relationship between N response, topography and P fertiliser history in a large-scale field trial established at Waipawa, in Hawke’s Bay. Pasture growth rates, species composition, and soil moisture levels were measured on easy and steep slopes of generally north – and south-facing aspects in two pairs of farmlets that had a low or high soil Olsen P status, one of each pair also receiving 30 kg N ha−1 yr−1 in winter. The N fertiliser response was found to be about twice as high on north than south aspects (), and very high on the steep, north aspect slopes. Gillingham et al. (Citation1998) reports this was related to differences in soil moisture between sites with different slope/aspect, which affected the persistence of clover in the pasture and in turn the response pattern to N fertiliser. For instance, the greater persistence of clover on easy slopes and on south aspects reflected the better soil moisture conditions throughout most of the year (Gillingham et al. Citation1998). In comparison, steeper slopes with northerly aspects, characterised by shallower soils with lower water holding capacity can’t support clover survival over the drier summer months. As a result, pastures are severely N deficient and therefore respond well to low rates of N fertiliser after soil moisture increases during winter (Roberts and White Citation2016).
Table 6. Average annual pasture response (kg DM kg−1 N) from the application of nitrogen (N) fertiliser (30 kg N ha−1) on contrasting topographic land units from 1995–2000 at Waipawa (adapted from Gillingham et al. Citation2003).
The trial at Waipawa was expanded with six more trials undertaken over three years on hill country sites on the east coast of both the North and South Island to evaluate pasture responses to N fertiliser in soils with a range of P concentrations (Gillingham et al. Citation2007, Citation2008a, Citation2008b). The response to N fertiliser applied mid/late winter on easy slopes ranged from 1.6 to 17.9 kg DM kg−1 N (Gillingham et al. Citation2007) and on steep slopes between 3.7 and 14.9 kg DM kg−1 N (Gillingham et al. Citation2008b) from N fertiliser applications of 90 kg N ha−1. There was little difference in the average pasture response to N fertiliser applied at 30 kg N ha−1 and at 90 kg N ha−1 to steep slopes at each site (Gillingham et al. Citation2008b). In contrast, on the gentler sloping sites at the same locations, the average N response declined as the rate of fertiliser N applied increased (Gillingham et al. Citation2007). Gillingham et al. (Citation2008b) suggests that soil on steep slopes was more deficient in N than soil on easier slopes. Except for one of the seven sites, the pasture N response on the easy slopes was higher than the adjacent steep sites, and there was no difference at the Waipawa site. This was different to the earlier findings at the site (Gillingham et al. Citation1998). The results of these trials highlight that slope and aspect of a site can influence pasture production and response to N fertiliser because they affect factors such as soil depth, water holding capacity, soil moisture and soil temperature, which in turn influence pasture composition, in particular the growth and survival of legumes (Ledgard et al. Citation1983).
Effect of soil P in hill country
Despite the contribution of N from legumes to pasture in hill country, pasture often remains responsive to the application of fertiliser N. Numerous factors, including low legume productivity and N fixation inputs (Grant and Lambert Citation1979), and animal nutrient transfer effects contribute to N deficiency in hill country pastures (Luscombe Citation1981). Overcoming soil fertility constraints on clover growth however has also been found to be particularly important in improving pasture response to N fertiliser (Gillingham et al. Citation2007). Of particular importance are nutrients such as P, essential for both good growth of legumes such as clover and N2 fixation, which drives pasture production. Phosphorus fertiliser inputs are required to assist legume growth on nearly all New Zealand hill country farms. For example, at the Waipawa hill country trial, Blennerhassett (Citation2002) found that regular application of high rates of N on all slopes and aspects, under both low and high soil P conditions, increased pasture production by 7000–8000 kg DM ha−1 (). However, the response to N was greater at the high soil P concentration. Blennerhassett (Citation2002) indicates that the presence of a P response when N was applied suggests that the addition of P fertiliser was overcoming an actual P deficiency in grass by stimulating clover growth.
Figure 7. The effect of nitrogen (N) fertiliser application on annual pasture production at both low and high soil phosphorus (P) concentrations. Average of easy and steep slopes and north and south facing aspects at the Waipawa trial site (adapted from Blennerhassett Citation2002).
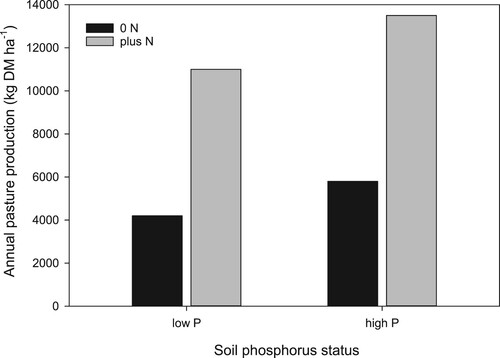
Interaction of nitrogen fertiliser and legumes
White clover is an important legume species in mixed grass/clover pasture systems because of its high quality as an animal feed, and its ability to biologically fix atmospheric N through a symbiosis with Rhizobium bacteria (Ledgard and Steele Citation1992; Caradus et al. Citation1996). Grass/clover pasture for example can produce herbage DM yields equivalent to pure ryegrass pasture that have received 300–600 kg ha−1 yr−1 of N fertiliser (Ball and Field Citation1985). The productivity of white clover in a grass/clover pasture can vary greatly depending on a range of factors including soil properties, climatic conditions, pests and disease (Hoglund et al. Citation1979; Harris Citation1987; Clark and Harris Citation1996; Chapman et al. Citation2018a). Two important management practices affecting productivity are pasture grazing management (Brock and Hay Citation1996) and the use of N fertiliser (Ledgard and Steele Citation1992). Many studies in New Zealand have investigated the interaction between the application of N fertiliser and the proportion and production of white clover and its impact on N2 fixation in grass/clover pasture.
Proportion of clover
Although there have been a few exceptions (e.g. Matthew et al. Citation1986; Morris and McRae Citation1990; Smith et al. Citation2000), generally the application of N fertiliser decreases the proportion of white clover in grass/clover pasture (Lambert et al. Citation2012; Chapman et al. Citation2018b) (). This has been reported in trials that have controlled pasture by mowing (O’Connor Citation1982; Crush et al. Citation1983) as well as those that have relied on grazing animals (O'Connor and Cumberland Citation1973; Ledgard et al. Citation2001), which is important because as noted, pasture management affects the composition and productivity of white clover in a grass/clover pasture. In the most comprehensive study, O’Connor (Citation1982) reported the proportion of clover decreased on average from 18% to 12% and grass increased from 75% to 82% from a N fertiliser application of 100 kg ha−1 in a summary of 158 mowed pasture field trials located across New Zealand. They also found that the decrease in the proportion of clover was most noticeable for the first 25 kg N ha−1 of N fertiliser applied, that it did not always result in a decrease in the yield of clover and the response was the same if N was applied in autumn or spring. O’Connor (Citation1982) also noted there was no obvious regional differences. This contrasts with the earlier findings of O'Connor and Cumberland (Citation1973) for a series of nine trials comparing pasture response to a high rate of N fertiliser (420 kg ha−1 yr−1), where trial sites located in northern North Island retained clover contents above 20%, while in South Island trials clover was reduced from 20% to less than 5%. O’Connor (Citation1982) later attributed this to differences in temperature between regions, but variation within a region suggests that defoliation frequency and intensity may also be important.
Table 7. Field trials that have assessed the effect of nitrogen (N) fertiliser application on the proportion (%) of clover in a grass/clover pasture sward.
Clover production
The application of N fertiliser to a grass/clover pasture in most instances results in an increase in DM production in the grass component of the pasture (). However, the production of clover in the grass/clover mix generally decreases (Harris et al. Citation1973; Ball et al. Citation1978; Ledgard and Saunders Citation1982; Luscombe and Fletcher Citation1982; Risk Citation1982; Ball and Field Citation1985; Feyter et al. Citation1985; Caradus et al. Citation1993; Gillingham et al. Citation1998; Ledgard et al. Citation2001; Gillingham et al. Citation2008b).
Figure 8. Annual herbage dry matter (DM) yield from the application of nitrogen (N) fertiliser (adapted from Ball Citation1989).
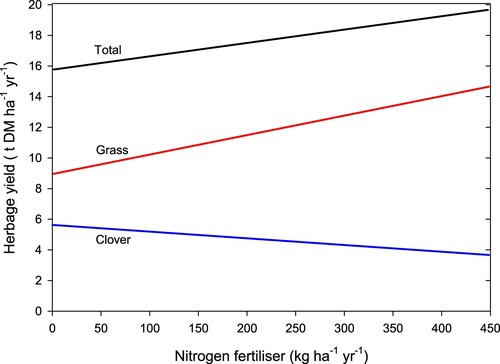
In a two-year farmlet study, Crush et al. (Citation1982) found that in grass/clover pasture receiving 100–150 kg N ha−1 yr−1 as urea, overall N fertiliser use decreased clover yields by 17%. Caradus et al. (Citation1993) reported clover growth decreased by an average of 38% over three years following the application of 225 kg N ha−1 yr−1 from urea in a sheep grazed trial that included a mix of 15 white clover cultivars grown with perennial ryegrass. Ledgard et al. (Citation1995) also compared the productivity of different white clover cultivars grown with perennial ryegrass that received no N or 390 kg N ha−1 yr−1 from urea applied at 30 kg N ha−1. Compared to the no N treatment, white clover production decreased as a result of N fertiliser application by 8%, 17%, and 30% in years one, two, and three respectively. The effect of N fertiliser reducing clover growth was greatest in the seasons where pasture growth was greatest e.g. spring 28% and summer 13% (). In large (0.09 ha) cattle grazed plots in Southland, Monaghan et al. (Citation2005) found on average over four years, clover biomass decreased by 20%, 35% and 48% in plots that received the 100, 200, and 400 kg N ha−1 fertiliser respectively compared with the 0 N treatment. More recently, Chapman et al. (Citation2018b) combined DM data from several sites across New Zealand to explore the main effects of clover and N fertiliser treatments on grass/clover pasture productivity. They reported that on average over three years from sites in Canterbury and the Waikato, clover yields in a grass/clover pasture decreased from 2400 to 1090 kg DM ha−1 yr−1 from the application of high (225–325 kg N ha−1) and low (50–100 kg N ha−1) rates of N fertiliser respectively. Chapman et al. (Citation2018b) also calculated that compared to a grass monoculture, a grass/clover mixture produced an additional 2400 kg DM ha−1 yr−1 in the low-N treatment and an additional 1200 kg DM ha−1 yr−1 in the high-N treatment.
Figure 9. Seasonal production by white clover in grazed grass/clover pastures as affected by nitrogen (N) fertiliser application. Data are the mean over three years (adapted from Ledgard et al. Citation1995).
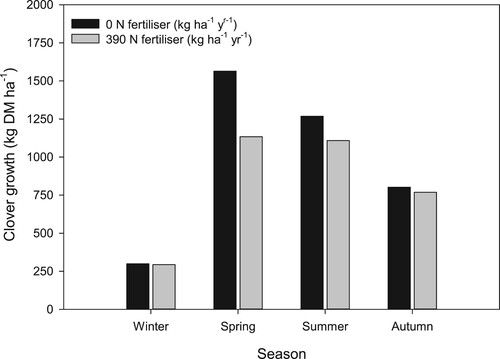
The application of N fertiliser has also been found to affect clover production on hill country, although compared to lowland sites responses are often more variable and inconsistent, depending on the year, P status of the soil, and on slope and aspect. Lambert and Clark (Citation1986) reported the late autumn application of N fertiliser (37 and 50 kg N ha−1 yr−1) to low and high fertility (P status) hill country pasture sites decreased clover production by 19% and 28%, respectively. Gillingham et al. (Citation2008b) measured the pasture response to N fertiliser (up to 90 kg N ha−1) applied mid-late winter to soils with different soil P status at sites on the east coast of both North and South Islands. It was found there was no response in early spring, thought to be due to the lower activity of clover at this time of year. A similar finding was made by Caradus et al. (Citation1993) who found no significant effect of N fertiliser application on clover production during the cooler seasons of autumn and winter. There was, however, a consistent decrease in clover production in late spring-autumn with increasing N fertiliser.
Several mechanisms have been reported for the decline in clover production from the application of N fertiliser. These include reduced clover stolon number and length (Harris and Clark Citation1996) and less stolon branching due to shading by grasses, which in a mixed pasture are more efficient at taking up fertiliser N from the soil than legumes (Murphy and Ball Citation1985; Caradus and Chapman Citation1991; Harris et al. Citation1995; Hepp et al. Citation2003). This highlights that a critical management practice in reducing clover suppression is tight grazing to control pasture cover, particularly in the spring (Harris and Clark Citation1996). Another important factor affecting clover production is the competition from grasses for moisture and nutrients (viz P) (Gillingham et al. Citation1998).
Biological N2 fixation
Biological N2 fixation in grass/clover pasture can also be affected by the application of N fertiliser (Crush et al. Citation1982; Ledgard and Saunders Citation1982; Risk Citation1982; Ledgard and Steele Citation1992; Chapman et al. Citation2020). While it has been found that the application of low rates of N fertiliser (< 50 kg N ha−1) may have little effect (Steele and Percival Citation1984; Eltilib and Ledgard Citation1988), more commonly, the application of higher rates of N fertiliser inhibits biological N2 fixation by microbes associated with legumes such as white clover (Ledgard et al. Citation1996a).
Luscombe and Fletcher (Citation1982) measured the effect of N fertiliser (0, 25, 50, 100 kg N ha−1) applied as urea in early spring on pasture yield, species composition and N2 fixation under grazing at the Ballantrae hill country research site. There was a decrease in clover yields and N2 fixation rates in the spring, summer and autumn after the spring N fertiliser application. Decreases were most notable during summer when clover yields and N2 fixation were 41% and 52% lower respectively on sites that received the highest rate of N fertiliser. Ledgard et al. (Citation1996a) compared N2 fixation in white clover cultivars grown with perennial ryegrass over three years that received either no N or 390 kg N ha−1 yr−1. Fertiliser N application decreased annual N2 fixation from 111 to 47 kg N ha−1 (mean for all cultivars), with the decline occurring predominantly in spring and summer (). In a more recent study, Ledgard et al. (Citation2001) found fertiliser N application (0, 200, 400 kg N ha−1 yr−1) decreased the average proportion of clover N derived from N2 fixation from 77% in the 0 N treatment to 43–48% in the low and high animal stocked treatments receiving 400 N ha−1 yr−1. On average, it was calculated to be a decrease in total N2 fixation from 154 to 39–53 kg N ha−1 yr−1. Ledgard et al. (Citation2001) estimated that the total annual N2 fixation decreased by an average of 0.27 kg N fixed per 1 kg of N fertiliser applied, mainly due to the decrease in clover production. Using data from two grazed sites in Canterbury and the Waikato, Chapman et al. (Citation2020) reported that for each 1 kg reduction in N fertiliser used, N2 fixation increased by 0.50–0.55 kg N.
Figure 10. Seasonal N2 fixation by white clover in grazed clover/grass pastures as affected by the application of nitrogen (N) fertiliser. Data are the mean of nine clover cultivar (adapted from Ledgard et al. Citation1996a).
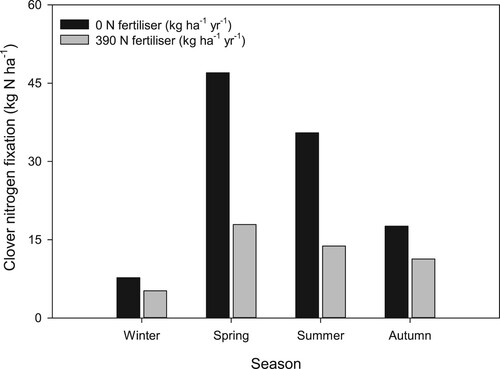
The decrease in N2 fixation in a grass/clover pasture from the application of N fertiliser is attributed to several factors (Caradus et al. Citation1996; Harris and Clark Citation1996). A decrease in the proportion of clover in a mixed grass/clover pasture will result in less N2 being fixed. The prolonged application of N fertiliser reduces infections and subsequent nodule formation by rhizobia in the soil (Mytton Citation1987). It can also restrict the development of nodules (Sprent Citation1979), something reported by Harris and Clark (Citation1996) in clover receiving increasing rates of N fertiliser (up to 400 kg N ha−1). Further, the presence of a ready supply of available soil mineral N sourced from N fertiliser (or dung and urine from grazing animals) favours uptake of mineral N by clover, because this is considered an ‘energetically less expensive’ process than fixing atmospheric N (Moustafa et al. Citation1969). As a result, clover substitutes fixing atmospheric N2 for mineral soil N (Hoglund and Brock Citation1987; Ledgard and Steele Citation1992; Ledgard et al. Citation1996a). However, importantly, Harris and Clark (Citation1996) note that although the reduction in N2 fixation activity through reduced clover content and nodulation is a longer-term effect, the substitution of mineral N for fixed N2 is a temporary effect, and N fixation re-commences when the soil N levels are depleted (Mundy Citation1990).
Clover morphology
The application of N fertiliser has also been found to affect clover plant morphology. Caradus et al. (Citation1993) showed the growing point density, stolon length and dry weight decreased in 15 white clover cultivars that received > 225 kg ha−1 yr−1 of N fertiliser. In a farmlet study the effects of high rates of N fertiliser (200 and 400 kg N ha−1) on white clover content and clover plant morphology in a mixed grass/clover pasture were monitored over five years (Harris and Clark Citation1996; Ledgard et al. Citation2001). Although 200 kg N ha−1 yr−1 had little effect on clover plant morphology, plants that received 400 kg N ha−1 yr−1 were generally smaller, with fewer, shorter stolons developing from fewer axillary buds. Further, the nodule number and mass per plant or per unit of root weight decreased with increasing rates of N application which reduced the potential for N2 fixation (Harris and Clark Citation1996).
Clover cultivars
The detrimental effects of N fertiliser on clover production and persistence stimulated interest in determining whether there are differences in the response between different white clover cultivars under different N regimes. Caradus et al. (Citation1993) found little difference in tolerance to N fertiliser amongst 15 white clover cultivars applying low to moderate rates of N fertiliser (25–225 kg N ha−1 yr−1) on pastures with a low clover component (5–14% of total pasture DM). Ledgard et al. (Citation1996a) compared the productivity of nine white clover cultivars grown with perennial ryegrass in a three-year field trial that received either 0 or 390 kg ha−1 yr−1 of N fertiliser. They found about a three-fold variation in annual N2 fixation between white clover cultivars at both N fertiliser treatments (), and N2 fixation was positively correlated with clover production.
Figure 11. Interaction between clover cultivar and rate of nitrogen (N) fertiliser application rate (0 or 390 kg N ha−1 yr−1) on the annual amount of N2 fixed by clover (adapted from Ledgard et al. Citation1996a).
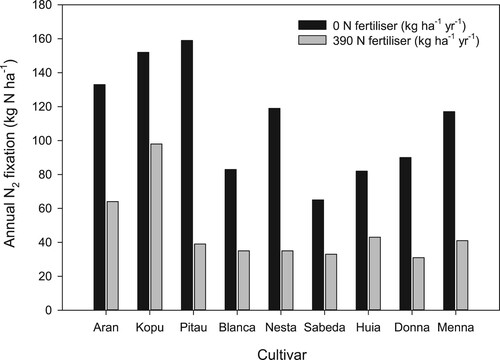
Nitrogen losses
In grazed grass/clover pasture systems, mineral-N derived from the application of N fertiliser can be lost directly or indirectly via three main processes including (i) nitrate (NO3-) leaching, (ii) ammonia (NH3) volatilisation and (iii) denitrification (i.e. transformations of N into gaseous forms such as di-nitrogen (N2) and nitrous oxide (N2O)) ().
Figure 12. Nitrogen transformation and losses from soil (adapted from Cardenas et al. Citation2019).
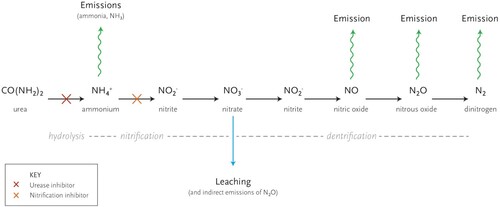
Nitrate leaching losses
One of the concerns with the use of N fertiliser is the increased potential for NO3– leaching losses from soil into water. This not only represents a loss of plant available N in soil, but also a threat to the wider environment and to human health (Ryden et al. Citation1984; WHO Citation1984; Goulding et al. Citation2008). The amount of NO3– that is leached from the soil depends on its concentration in soil solution and the drainage volume (Di and Cameron Citation2002). These are affected by both the source of N and a range of soil and environmental factors and management practices (Cameron et al. Citation2013).
Historically, the view in New Zealand was that leaching loss of N from grass/clover pastures was relatively small because of the ability of pasture to accumulate large amounts of N from fertiliser inputs or fixed by legumes (Steele Citation1982). This ensured that NO3– concentrations in soil solution remained relatively low. However, reports of elevated N concentrations measured in groundwater (Baber and Wilson Citation1972; Taranaki Catchment Commission Citation1987) and leachate (Goh et al. Citation1979) from intensively grazed pasture systems in the Waikato, Taranaki and Canterbury regions in the 1970s highlighted that N loss via leaching from these systems may be important. This was confirmed by subsequent research in the late 1970s where mineral N concentrations were measured down the soil profile below grazed grass/clover pasture sites that received N fertiliser. Holland and During (Citation1977) for example found that NO3– from sodium nitrate fertiliser (400 kg N ha−1) applied to a free draining sandy loam moved to a depth of 400 mm.
This was the catalyst for a large amount of research that has been ongoing over the last four decades in New Zealand, that have measured, and modelled nitrate leaching losses from intensively grazed pasture systems and identified the soil and environmental factors and management practices affecting leaching loss. A large amount of this research has been captured in various papers (e.g. Ledgard et al. Citation2001; Di and Cameron Citation2002; Monaghan et al. Citation2007; Cameron et al. Citation2013; Monaghan and de Klein Citation2014; Selbie et al. Citation2015; Watkins and Shepherd Citation2015; Vogeler et al. Citation2016).
The research has been wide ranging, including quantifying N leaching losses from different sources of N including fertiliser (Di et al. Citation1998; Silva et al. Citation1999; Di and Cameron Citation2002), farm dairy effluent (Cameron et al. Citation1996; Di et al. Citation1998; Silva et al. Citation1999; Monaghan and Smith Citation2004) and cow and sheep urine (Monaghan et al. Citation1989; Silva et al. Citation2005; Citation1999; Buckthought et al. Citation2015a). The effect of timing and rate of application of N on leaching losses has also been measured (Steele et al. Citation1984; Harris et al. Citation1994; Ledgard et al. Citation1996b; Ledgard et al. Citation1999; Silva et al. Citation1999). Environmental factors and management practices that affect NO3– leaching have also been a focus, including the effect of soil type (Beale et al. Citation2021), animal type (Hoogendoorn et al. Citation2011) and stocking rate (Ledgard et al. Citation1999; Monaghan et al. Citation2002; Citation2005; Sprosen et al. Citation2002; Shepherd et al. Citation2014), different types of irrigation (Di et al. Citation1998), climatic conditions (rainfall) and grazing management (Betteridge et al. Citation2007; Christensen et al. Citation2012; Shepherd et al. Citation2014; Monaghan et al. Citation2016). Leaching losses have been measured at both intensive lowland sites (Monaghan and Smith Citation2004) and hill country sites (Crofoot et al. Citation2010; Hoogendoorn et al. Citation2017). There has also been extensive research on the application of technologies to decrease NO3– leaching loss, and these are discussed in the technology section of the paper.
A key finding from research on NO3– leaching is that while the amount of fertiliser N applied to pasture affects the amount of leaching, it is the N excreted by animals, particularly urine in intensively grazed pasture systems that is the main factor affecting losses (Ledgard et al. Citation2000; Monaghan et al. Citation2005). This is because the application of N fertiliser generates more forage, enabling higher stocking rates, higher consumption and thus more N excretion to the soil in dung and urine (Di and Cameron Citation2002). The majority of N (70–90%) is in the form of urea, some of which can be volatilised, as discussed in the next section on N losses (Di and Cameron Citation2000), but most is nitrified and accumulates in soil as NO3-. Because the N loading from urine is high (e.g. 610 kg N ha−1; Selbie et al. Citation2015), and greater than plant N requirements, the remaining NO3– can be lost by denitrification or leached when there is drainage from the soil (Fraser et al. Citation1994).
This has been well illustrated by studies that have measured N leaching losses from soil amended with N fertiliser, cow urine and combinations of both fertiliser and urine (e.g. Ledgard et al. Citation1999; Di and Cameron Citation2002). In a lysimeter study using a sandy loam soil under a grass/cover pasture, Silva et al. (Citation1999) reported N leaching losses of 3.6 kg ha−1 yr−1 from the control and losses of 8 and 17.4 kg ha−1 from soil amended with 200 and 400 kg N ha−1 from urea, applied in four split applications. In comparison, N losses from a urine treatment applied at 1000 kg N ha−1 was 124 kg ha−1 yr−1, and losses increased to 190 kg N ha−1 when urine was amended with 200 kg N ha−1 of urea. Another important finding from the research on NO3– leaching is that even when no N fertiliser is applied, there will still be N leaching loss due to animal urinary excretion from the surplus protein in forage grazing animals consume, along with variable amounts N mineralised from soil organic matter.
Ledgard et al. (Citation1999, Citation2000) showed in a farmlet study stocked at 3.3 cows ha−1 that received N fertiliser (urea) at 0, 200, 400 kg ha−1 yr−1, on average over five years, N leaching losses from the control were c. 30 kg N ha−1 yr−1 ().
Figure 13. Effect of rate of nitrogen (N) fertiliser application on nitrate leaching in dairy pasture stocked at 3.3 cows ha−1. Data are the mean for five years (adapted from Ledgard et al. Citation1999; Citation2000).
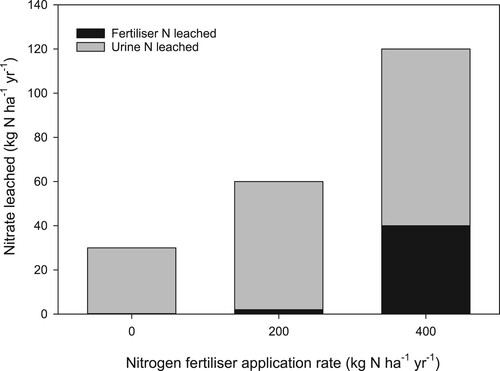
Research also shows that in general, the direct leaching of N from fertiliser in grazed pasture is low if application rates are not excessive (i.e. < 200 kg N ha−1 yr−1) and are synchronised with periods when pasture requires N and is actively growing (Silva et al. Citation1999; Ledgard et al. Citation2000; Di and Cameron Citation2002; Monaghan et al. Citation2005). Di et al. (Citation1998) used lysimeters to measure NO3– leaching from a sandy loam soil under a grass/clover pasture amended with 400 kg N ha−1 from ammonium chloride applied annually over two years. Nitrate-N losses ranged between 28 and 48 kg ha−1 (8.7–9.8% of the N applied from fertiliser). Silva et al. (Citation1999) reported N fertiliser leaching losses were < 5% of the N applied to a stony soil amended with urea at 200 and 400 kg N ha−1. Ledgard et al. (Citation1999, Citation2000) showed that direct N leaching losses from N fertiliser were minimal from pasture receiving 200 kg N ha−1 yr−1, and approximately 10% of the N fertiliser applied receiving 400 kg N ha−1 yr−1 (). Cookson et al. (Citation2000) measured the contribution of autumn 15N-labelled fertiliser (50 kg N ha−1) to NO3– leaching losses from a soil that was cultivated and sown with ryegrass over two years. Nitrogen fertiliser was found to directly contribute 7.8–8.6% of the total amount of N leached from soil. However, larger N leaching losses from the application of N fertiliser have been reported. Ledgard et al. (Citation1988) measured the amount of inorganic 15N with depth down the soil profile in a pasture soil after it had received labelled 15N urea (50 kg N ha−1) in May, June and August. They calculated that up to one-third of the N fertiliser applied in May/June was lost via leaching. Ledgard et al. (Citation1988) reported that the direct leaching losses of fertiliser N generally only occur when applied in late-autumn and winter and that avoiding applying N fertiliser during this period will likely minimise direct fertiliser N losses.
Ammonia volatilisation
Ammonia volatilisation is the loss of gaseous ammonia (NH3) to the atmosphere and can occur whenever there is free NH3 at the soil surface (Bolan et al. Citation2004a). This may be from the application of animal urine and faeces, the mineralisation of soil organic matter and plant residues, as well as from the application of N fertiliser such as urea (Cameron et al. Citation2013). Under moist conditions, urea applied to soil from fertiliser can be hydrolysed (within 1–2 days) to produce ammonium carbonate by the enzyme urease (Zaman et al. Citation2013a) (). The ammonium carbonate quickly dissociates to ammonium (NH4) (Bolan et al. Citation2004a). Under alkaline conditions (pH >7) the ammonium will be converted to ammonia gas, which may then be volatilised to the atmosphere. Ammonia volatilisation is unwanted because it is an agronomic loss of N from the soil/plant system and a threat to the wider environment. This is because the majority of the NH3 that is volatilised is re-deposited back to land or surface water and can cause acidification and eutrophication of ecosystems (Bolan et al. Citation2004a), and it can also be an indirect source of N2O (Haynes and Sherlock Citation1986; Saggar et al. Citation2013).
There has been a large amount of research undertaken in New Zealand investigating NH3 loss from N fertilisers applied to grazed pasture. This has included quantifying losses, the factors which affect losses, along with the testing of management strategies and technologies to mitigate NH3 losses from N fertiliser (discussed in the technology section). Although losses of N due to NH3 volatilisation vary, they are typically c. < 15% of the N applied from a single moderate application of urea (<40 kg N ha−1) (). However, greater losses have been reported at higher urea application rates ().
Table 8. Ammonia (NH3) volatilisation losses measured from the urea, ammonium sulphate (AS), and diammonium phosphate (DAP) fertiliser applied to pasture soils in New Zealand.
Because urine is a concentrated solution of urea (up to 10 g N L−1) (Cameron et al. Citation2013), urine deposited during animal grazing is also an important source of NH3 loss. Various studies have measured NH3 volatilisation at the urine patch scale from sheep, beef and dairy grazed pasture in New Zealand (Ball et al. Citation1979b; Carran et al. Citation1982; Sherlock and Goh Citation1984; Di and Cameron Citation2004; Zaman and Nguyen Citation2012). As a proportion of the N in the urine, NH3 losses ranged between 1.5% and 38%, a similar range as to those lost from the application of N fertiliser.
A range of factors have been found to affect NH3 loss including the type of N fertiliser applied and the timing of application, soil properties, climate and the application of irrigation (Black et al. Citation1984, Citation1985a, Citation1985b, Citation1987a; Selvarajah et al. Citation1989; Zaman et al. Citation2013a). Black et al. (Citation1985b) measured NH3 emissions from urea, diammonium phosphate, ammonium sulphate and calcium ammonium nitrate (30 kg N ha−1) applied to pasture across a range of seasons. They reported average percent losses of NH3 were highest from urea (11.9%), then diammonium phosphate (5.3%), ammonium sulphate (1.0%) and calcium ammonium nitrate (0.8%). This is because the hydrolysis of urea to ammonium carbonate produces a greater pH rise than ammonium-based fertiliser. Black et al. (Citation1987a) investigated the influence of rainfall and irrigation on the amount of NH3 volatilised from urea applied to pasture. They found that the application of 10–16 mm of rainfall within eight hours of urea application decreased the amount of NH3 loss by 80%. This is because the rainfall/irrigation water washed urea below the soil surface that ensured the surface NH3 concentration was low. Zaman et al. (Citation2013a) also showed the importance of the amount and timing of irrigation on NH3 emission after the application of urea to a pasture soil. Delaying irrigation for 48 hr after the application of urea resulted in average NH3 losses of 23% and 28% for urea applied at 30 and 60 kg N ha−1, respectively. Even when 5 or 10 mm of irrigation was applied eight hours after applying urea, average NH3 losses were still between 11% and 14%. It was suggested that to minimise NH3 losses, 5–10 mm of irrigation should ideally be applied within eight hours of applying urea, although current advice is the application of 10 mm or more water within eight hours (Ants Roberts pers. Comm.). Selvarajah et al. (Citation1993) measured NH3 loss from the application of 100 kg N ha−1–to 11 soils with contrasting properties. Ammonia losses ranged between 4.4% and 53.9% of the N applied and it was concluded soils that have low chemical resistance to the alkalinity build-up caused by the urea hydrolysis products produced higher NH3 losses. Black et al. (Citation1985b) also investigated whether mixing urea with an acidifying agent such as sulphur (S) (S coated urea) could reduce the risk of NH3 loss. The results from two applications to pasture were mixed, with no effect after the application of urea in April but a significant decrease after the application in September, although soil pH was not different after either application. It was concluded that any beneficial effect on volatilisation from the presence of elemental S would be small.
An important finding from these studies is that although NH3 losses from N fertiliser (or urine) can be significant, the factors which affect losses are well known and tested, and strategies to manage NH3 losses are available. Further, new technologies are also available that can minimise NH3 losses from urea and are discussed in the technology section.
Emission of nitrous oxide and di-nitrogen gas
Along with NH3, gaseous losses of N2, N2O, and to a lesser extent nitric oxide (NO) also represent a significant loss of N from the soil/plant system (Cameron et al. Citation2013). Nitrous oxide is also a potent greenhouse gas (GHG) and a contributor to stratospheric ozone depletion (Sutton et al. Citation2014). Most of the N2O, N2, and NO lost from soil is produced biologically, mainly due to the processes of nitrification and denitrification (de Klein and van Logtestijn Citation1996; Cameron et al. Citation2013) (). Nitrification results in the accumulation of NO3– in soil, which is the substrate for denitrification and production of N2O and N2 (McLaren and Cameron Citation1996).
Emissions of N2O are controlled by interactions between different soil physical and chemical properties, soil microbiological populations, climate, and animal management practices (Whitehead and Edwards Citation2015). A focus of research in New Zealand (and elsewhere) has been calculating N2O emissions factors (EF) (proportion of the N fertiliser that is emitted as N2O) under different conditions (e.g. climate, soils etc.) and rates of N fertiliser application. The EF are used in mandatory GHG inventory reporting. In addition, there has been a lot of research focused on the testing of strategies to mitigate N2O losses from different N fertilisers which are discussed in the technology section.
Calculation of N2O EFs have been made for different types of N fertiliser applied to pasture soils including urea (de Klein et al. Citation2004; Luo et al. Citation2007; Kelliher et al. Citation2014; van der Weerden et al. Citation2016b) and non-urea fertilisers including ammonium phosphate, ammonium sulphate, potassium nitrate and diammonium phosphate (Bhandral et al. Citation2007; Zaman et al. Citation2008). Kelliher et al. (Citation2014) undertook a meta-analysis of the results from 22 field experiments where urea had been predominantly applied at a rate of 50 kg N ha−1 to calculate a New Zealand specific EF value. The mean EF and standard error were 0.48 ± 0.13%. Van der Weerden et al. (Citation2016b) updated the meta-analysis of Kelliher et al. (Citation2014) with the addition of four new data values and calculated a mean EF (95% confidence limits) for urea of 0.59% (0.14–1.02%) This value for urea is used for GHG inventory reporting in New Zealand.
Compared to urea, few studies have calculated EF for non-urea N fertilisers. Zaman et al. (Citation2008) reported an EF value of 1% for diammonium phosphate (150 kg ha−1 yr−1), similar to the EF value determined for the corresponding urea treatment (1.1%). Bhandral et al. (Citation2007) calculated an EF of about 1.1% for urea and ammonium sulphate and 9.9% for potassium nitrate applied at 600 kg N ha−1 to pasture soil (compacted) in the Manawatu. Leahy et al. (Citation2019) reports emissions of N2O from N fertiliser have been calculated at 4% of total agricultural emissions in New Zealand in 2017.
As previously reported, in grazed pasture systems there are large inputs of excreta (dung and urine). As a consequence, many more studies have investigated N2O losses from soils amended with urine than N fertiliser in New Zealand (e.g. Haynes and Sherlock Citation1986; Müller Citation1995; Clough et al. Citation1998, Citation1996; Bolan et al. Citation2004b; Clough et al. Citation2005; Di and Cameron Citation2008, Citation2006, Citation2003; Hoogendoorn et al. Citation2008; Saggar et al. Citation2009; Qiu et al. Citation2010; de Klein et al. Citation2011, Citation2001; van der Weerden et al. Citation2014, Citation2011; Buckthought et al. Citation2015b). The findings from many of these studies have recently been summarised by Di and Cameron (Citation2018). A recent meta-analysis of N2O EFs from these studies based on livestock type and slope class report EF values of 0.08% for sheep urine on medium and steep slopes and 0.98% for dairy cattle on flatland and low slopes (van der Weerden et al. Citation2020). Compared to N2O from N fertiliser, emissions from the deposition of animal excreta are a larger source from pasture systems, representing 17% of New Zealand’s agricultural emissions in 2017 (Leahy et al. Citation2019).
Technology and innovation
A range of technologies and innovations have been identified and tested that are designed to both improve the pasture response from N fertiliser and also minimise direct and indirect losses from it.
Plant growth regulators
Plant growth regulators (PGR) are a group of naturally occurring organic compounds that have been shown to influence a wide range of physiological processes in plants including stem elongation and leaf expansion, flowering, fruiting and seed germination (Davies Citation1995). The majority of research in grazed pasture systems in New Zealand has focused on assessing the efficacy of the PGR Gibberellic acid (GA). Interest has centered around the application of GA to potentially reduce the use of N fertiliser, while at the same time maintaining or enhancing DM production (Whitehead and Edwards Citation2015), particularly in the shoulder of the seasons when cool temperatures limit pasture growth (Woods et al. Citation2016). Another potential benefit is the production of pasture with a lower N concentration, decreasing the N intake by grazing animals and N output in urine, thereby reducing N loss and the related environmental effects (Whitehead and Edwards Citation2015).
Research has focused on (i) the response of pasture DM production to application of GA (Edmeades Citation2009; Matthew et al. Citation2009; van Rossum et al. Citation2013; Zaman et al. Citation2014; Bryant et al. Citation2016), (ii) the effect of seasonality on DM production (Ball et al. Citation2012; Parsons et al. Citation2013), (iii) changes in the pasture composition (van Rossum et al. Citation2013; Bryant et al. Citation2016), (iv) effect on herbage N concentration and quality (Ghani et al. Citation2014; Zaman et al. Citation2014; Bryant et al. Citation2016) and (v) the estimated effects on N losses from NO3– leaching and N2O emissions (Ghani et al. Citation2014; Woods et al. Citation2016).
The key findings from these studies are that GA can stimulate extra pasture production in late winter/early spring and late autumn/early winter, when natural pasture growth is limited by cooler soil temperatures. Timing is an important factor and ideally GA should be applied one to five days after grazing, when there is sufficient herbage available for uptake, and then harvested within 40–50 days (Edmeades and McBride Citation2012). Where GA has been applied with N fertiliser the effects have been shown to be additive (Jiang et al. Citation2011; van Rossum et al. Citation2013; Zaman et al. Citation2014). Although GA used both with and without N fertiliser increases pasture yield within the first three weeks after application, it can be at the expense of reduced DM yields in subsequent weeks (Matthew et al. Citation2009).
The effectiveness of applying GA with urea and cow urine on N losses appears to be inconclusive. Preliminary modelling by Ghani et al. (Citation2014) indicated reduced N excretion by animals consuming GA treated pasture and a decrease in urine-N leaching of up to 29% for a Waikato case study dairy farm. However, Di et al. (Citation2016) showed that the application of GA to urine-treated lysimeters (700 kg N ha−1) with Italian ryegrass (Lolium multiflorum), lucerne (Medicago sativa) or grass/clover pastures did not result in lower N2O emissions or decrease NO3– leaching losses (Woods et al. Citation2016).
Coated nitrogen fertiliser products
There has been considerable research in New Zealand on N fertilisers amended with bio-active chemicals or organic and inorganic products to slow down transformations and losses (volatilisation, denitrification, leaching, runoff) of N fertiliser once it is in the soil (Edmeades Citation2015). Of particular interest has been the application of urease and nitrification inhibitors to N fertiliser and urine patches in pastures and polymer-coated controlled release N fertiliser products.
Urease inhibitors
As noted, the loss of N from soil due to NH3 volatilisation from the application of N fertiliser (urea) and urine in particular from grazed pasture systems can be significant. One way to reduce NH3 volatilisation is to coat urea fertiliser with a urease inhibitor (UI). Inhibitors are natural or synthetic compounds that can slow, for a period of one to two weeks, the rate of conversion of urea to NH3/NH4 by inhibiting the activity of the urease enzyme (Saggar et al. Citation2013) (). Among the different UI’s available, N-(n-butyl) thiophosphoric triamide (NBPT) has been the most widely researched in New Zealand (Saggar et al. Citation2013; Zaman et al. Citation2013a), although there has been limited assessment of the effectiveness of other inhibitors such as N-(2-Nitrophenyl) phosphoric triamide (2-NPT) (Adhikari et al. Citation2018). Laboratory and field-based pasture trials in New Zealand have measured the effect of urea fertiliser applied at different rates and treated with NBPT on NH3 emissions. Zaman et al. (Citation2013a) measured NH3 emissions over a two-week period from a soil that received two rates of urea (30 and 60 kg N ha−1) and urea (60 kg N ha−1) amended with 0.025% NBPT. Daily NH3 emissions in the urea treatments declined rapidly after peaking on day three, reflecting the fast hydrolysis of urea (Zaman et al. Citation2013a). In comparison, NH3 losses from urea-nBTPT applied at 60 kg N ha−1 slowly increased after day two, reached their maximum on day five and declined (). On average over the two-week period, urea treated with nBTPT emitted 47% less NH3 than un-treated urea. On average the application of NBPT at 0.025% has been found to reduce ammonia emissions by c. 44.7% (), with a 95% confidence of interval of 39–50% (Saggar et al. Citation2013).
Figure 14. Daily ammonia (NH3) emissions over a two-week period after the application of two rates of urea (30 and 60 kg ha−1) and urea (60 kg ha−1) treated with the urease inhibitor nBTPT (0.025%) to a pasture soil (adapted from Zaman et al. Citation2013a).
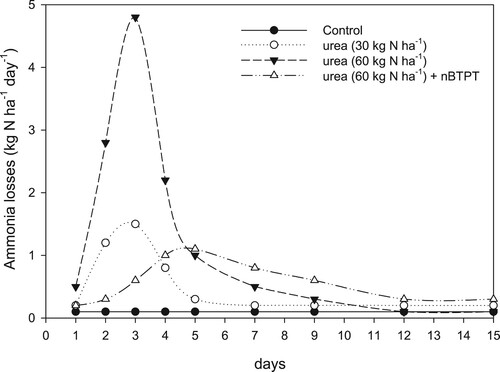
Table 9. Reduction in ammonia (NH3) emissions with application of the urease inhibitor N-(n-butyl) thiophosphoric triamide (nBTPT) to pastoral soils in New Zealand (adapted from Saggar et al. Citation2013).
The effect of applying urea treated with nBTPT on pasture productivity and N use efficiency has also been investigated. In a series of field trials across New Zealand, Zaman et al. (Citation2013b) found treating granular urea with nBTPT increased both pasture productivity and N use efficiency. It was reported to be due to a range of factors including reduced losses of N via NH3 volatilisation, an increased dispersion of urea, a reduced rate of nitrification and increased plant uptake of N, either in urea or NH4 form (Zaman et al. Citation2013b).
Ammonia emissions from animal urine amended with NBPT at 0.025% has also been investigated (Menneer et al. Citation2008; Singh et al. Citation2008; Zaman et al. Citation2009; Zaman and Blennerhassett Citation2010; Zaman and Nguyen Citation2012). The urease inhibitor has been shown to decrease NH3 losses by c. 53% with a 95% confidence interval of 33–73% (Saggar et al. Citation2013).
The effect of applying both urease and nitrification inhibitors (NI) (discussed in the next section) on N use efficiency has also been explored (Zaman et al. Citation2013c). Zaman and Blennerhassett (Citation2010) showed that nitrification and UI applied together with urine decreased emissions of NH3 (48% and 51%), N2O (55% and 63%) and NO3– leaching (56% and 42%) in autumn and spring, respectively. There were also increases in pasture production (13% and 17%) and N uptake (7% and 18%) in autumn and spring, respectively.
Nitrification inhibitors
Nitrification inhibitors (NI) are chemical compounds that can slow the first stage of nitrification, temporarily delaying the bacterial oxidation of NH4 to NO3– in the soil () (Amberger Citation1989). The increase in soil N retained in the NH4 form means more N is available to be assimilated by plants that may then potentially mean greater DM yield, as well as a reduction in N losses via NO3– leaching and N2O emissions (Moir et al. Citation2007; Smith et al. Citation2008; Monaghan et al. Citation2009; Carey et al. Citation2012; Cameron et al. Citation2014; Di and Cameron Citation2018).
Many compounds have been shown to have NI properties (e.g. 3,4-dimethylpyrazole phosphate (DMPP) and [2-chloro-6-(trichloromethyl) pyridine] (nitrapyrin) (Di and Cameron Citation2016). However, dicyandiamide (DCD) has been by far the most frequently investigated NI in New Zealand pasture systems (Di and Cameron Citation2018), with a smaller amount of research investigating a liquid formulation of 3,4-dimethylpyrazole phosphate (DMPP) (Di and Cameron Citation2012, Citation2011).
As has previously been noted, direct leaching losses of NO3– or atmospheric emissions of N2O from N fertiliser are small compared to losses from urine returned by the grazing animal (Silva et al. Citation1999; Di and Cameron Citation2002). Consequently, only a few studies in New Zealand have measured the effect of applying DCD with N fertiliser on N loss. Van der Weerden et al. (Citation2016a) compared N2O losses from pasture in four regions of New Zealand that had contrasting soils and climatic conditions that were amended with 50 kg N ha−1 of urea with and without the addition of 0.02 kg DCD kg−1 N. It was found that as a proportion of the N added, N2O-N losses in urea fertiliser ranged from 0.03% to 1.52%. In comparison, the effect of applying DCD was mixed, with a 90% reduction at the site where the cumulative N2O emission for urea fertiliser was greatest. However, DCD had no effect at the other three sites. It was speculated this was possibly because emissions were already very low making it difficult to detect differences between treatments.
In comparison, over the last 20 years a large body of research has focused on quantifying the effectiveness of applying DCD on N2O emissions, NO3– leaching and on pasture yield from urine inputs in grazed pasture systems. There has also been considerable research identifying and testing the factors that control the effectiveness of DCD including the properties of the NI, soil temperature, rainfall, effects of different soil properties (pH, CEC) and timing and rate of DCD application. Several recent reviews have comprehensively summarised much of this research (Di and Cameron Citation2016; Di and Cameron Citation2018).
In brief, the application of DCD has been shown to be a highly effective technology for reducing NO3– leaching across a wide range of soil and environmental conditions. Studies using undisturbed soil lysimeters to measure the effect of applying DCD to urine patches showed that although there is variability, the average reduction in NO3– leaching was 50% (± 3 SEM) (Di and Cameron Citation2016 and references therein). Integrated measures of nitrate leaching from both the urine patch and non-urine areas across a paddock using either suction cups or drainage plots showed the application of DCD reduced NO3– leaching by about 30% (± 6 SEM) (Di and Cameron Citation2016). However, the effectiveness of DCD is influenced by factors such rainfall, temperature (Kelliher et al. Citation2008) and the frequency and timing of DCD application (Menneer et al. Citation2008). The application of DCD to grazed pasture soils can decrease N2O losses from urine patches in soil from both nitrification and denitrification processes (Di and Cameron Citation2008). Results from a national series of trials capturing a wide range of soil and environmental conditions showed that the application of DCD decreased N2O emissions from animal urine-N affected soil by an average of 57% (±2) (Di and Cameron Citation2016). Because DCD increases the amount of N retained in the soil/plant system, potentially this can result in an increase in pasture response to N and plant growth. However, because pasture growth can be affected by a wide range of soil and environmental factors, along with the uneven distribution of urine patches across a paddock, there is often considerable variability in yield effects from the application of DCD (Ledgard et al. Citation2014). Nonetheless, Carey et al. (Citation2012) collated data on pasture response from DCD applied to 132 paddocks from 37 farms located across New Zealand. They found an overall increase in DM production from the application of DCD of 19%.
However, importantly, in 2011 the discovery of DCD residues in milk resulted in DCD being withdrawn from commercial use in New Zealand. This was despite DCD being shown to pose little or no health risks to either grazing animals or humans (OECD Citation2003). In the meantime, there is ongoing research to identify and commercialise new NI’s (de Klein et al. Citation2022).
Controlled release nitrogen fertiliser
Controlled release fertilisers are coated with an organic or inorganic product designed to delay the release of N from the fertiliser granule, so potentially the N is supplied to the plant at a rate that matches demand and reduces the risk of N loss from the soil-plant system (Guinto et al. Citation2018).
Several controlled release N fertiliser products have been developed and tested in New Zealand pasture systems, with variable results. Steele and Dawson (Citation1980) undertook a series of five trials in Northland designed to assess the rate, timing, and form of N fertiliser on pasture botanical composition and production at pasture establishment or after pasture renovation. It was found that two controlled-release N fertilisers i.e. Isobutylidene diurea and Organiform applied at 50 kg N ha−1 performed no better than urea. Bishop et al. (Citation2008) compared the effectiveness of 5% and 7% polyurethane coated urea, urea coated with DCD, and un-coated urea on DM yield of Italian ryegrass, herbage N content, and N concentrations in drainage over a four-month field trial. It was reported that none of the treatments, including the polyurethane coated urea were significantly better than untreated urea in terms of pasture DM production. There was however lower NO3– leaching losses from soil treated with polyurethane coated urea compared to untreated urea (Bishop et al. Citation2008).
More recently, several studies have investigated the effectiveness of a polymer coated, controlled-release urea-based N fertiliser called Smartfert® (Edmeades Citation2015; Edmeades and McBride Citation2017; Guinto et al. Citation2018; Dawson et al. Citation2019). Edmeades (Citation2015) reported that Smartfert® applied once at two rates (25 or 50 kg N ha−1) compared with the same rates of N applied once as urea over 5–6 months increased N use efficiency (kg DM kg−1 N applied) by 5–50%, depending on the site and the rate of N application. Further testing of Smartfert® was undertaken by Edmeades and McBride (Citation2017) across five trial sites over two years in which the effect of single applications of different rates of Smartfert® on pasture production and pasture N concentrations were measured, relative to the same rates of SustaiN (urea which contains a UI), along with a comparison of multiple applications of SustaiN with a single application of Smartfert®. The key findings from these trials were DM production was higher at two of the sites (Rotorua and Canterbury) that received Smartfert® relative to the same rate of N applied as SustaiN, although this depended on the rate of N applied and the year. At one site (Northland), the application of SustaiN significantly increased pasture production relative to Smartfert®. In two trials (Northland and Rotorua), pasture DM production from a single application of Smartfert® (100 kg N ha−1) was the same as three consecutive applications of 33 kg N ha−1 of SustaiN, and at a third trial site (Canterbury) production from the application of Smartfert® was significantly better. In addition, N use efficiency of Smartfert® was significantly greater than for SustaiN in two of the trials (Canterbury and Rotorua) at the lower rate of N (30 kg N ha−1).
More recently, Dawson et al. (Citation2019) compared pasture production at two sites in South Westland treated with Smartfert® (60 kg N ha−1) in combination with SustaiN (40 kg N ha−1) in one application, and SustaiN that was applied after every cut over a three-month period at rates of 40, 30 and 30 kg N ha−1, respectively. At one of the sites, total DM production was significantly higher in the SustaiN treatment compared to the Smartfert® treatment, although there was no difference between treatments at the other site. However, when reporting pasture production across the trial period (day 49, 76, 103), Smartfert® and the SustaiN treatment produced similar amounts of pasture up to day 76 at both sites. At day 103, DM production in the SustaiN treatment was higher than the Smartfert® treatment. Dawson et al. (Citation2019) suggest the release of the Smartfert® was either not keeping up with plant demand or had fully released its 60 kg N ha−1, in addition to the 40 kg N ha−1 in the SustaiN. It appears the effectiveness of controlled release fertilisers are variable and only in a few studies has a small increase in N efficiency been found.
Fertigation
Fertigation is the process of applying liquid/dissolved fertiliser with irrigation water to a crop or pasture. Liquid fertilisers are made by dissolving solid granular fertilisers in water through agitation of the solution or heating the water to assist dissolution (Morton et al. Citation2019). The perceived benefits of applying N via fertigation over granular fertiliser are the ability to maintain or enhance DM production by smaller and more frequent N fertiliser applications, the direct incorporation of N into the soil thereby minimising N loss via volatilisation and the possibility of maintaining a lower, but constant N concentration in soil solution to reduce NO3– leaching losses (Ley Citation2020).
Only limited research has been undertaken in New Zealand comparing the effect of applying granular and liquid N fertilisers to pasture systems. These have been small (6 × 2 m), mowed plots trials, where N fertiliser has been applied at rates between 20–40 kg N ha−1, that have focused on the effects on pasture production and quality (Carey Citation2009; McCloy Citation2009; Ley Citation2020). The most comprehensive study compared a monthly application of urea (25 kg N ha−1) dissolved in water with a monthly application of solid urea (25 kg N ha−1) that received irrigation either immediately or two days after N application on pasture production and quality (Ley Citation2020). Both N treatments produced similar DM yield and pasture quality at all harvests across the growing season. In another trial, 25 kg N ha−1 dissolved in water was applied either once per month or once per week (6.25 kg N ha−1 week−1) to determine if smaller gaps between application timing increased pasture response to N fertiliser (Ley Citation2020). It was also found that the larger monthly and smaller weekly N application produced similar DM yield and pasture quality across the growing season. These results are consistent with the finding of two earlier trials (e.g. Carey Citation2009; McCloy Citation2009) where there was no significant difference in pasture DM yield between the application of liquid or solid forms of urea.
Fine particle application
Fine particle application (FPA) fertilisers are made by fine grinding solid granular fertilisers to between 100 and 200 μm particle size and adding water (30–40% by weight) to produce a fluid mixture of dissolved fertiliser and fine fertiliser particles that can be boom-sprayed onto pasture either in an aerial or ground application (Morton et al. Citation2019). It has been suggested FPA of N fertiliser could improve N use efficiency and pasture production compared with the application of granular N fertiliser due to a combination of factors including a more uniform application of the N fertiliser, easier N uptake through both roots and leaves, and more efficient N metabolism (Dawar et al. Citation2011; Quin et al. Citation2015, Citation2006).
A recent review of New Zealand trials investigating the relative pasture response of FPA compared to granular application of N fertiliser has been completed (Morton et al. Citation2019). It found that of the trials that could statistically analyse their data, only in two of the three trials (Dawar et al. Citation2012, Citation2011) was there a significant pasture yield response to the application of FPA over a granular N fertiliser (). In a more recent trial not included in the review, Risk and Dawson (Citation2019) compared the effect of FPA N and granular N applied at two rates (25 or 50 kg N ha−1) in spring and autumn on pasture production at a site in Southland (). It was found there was no significant difference in pasture production harvested at four and eight weeks post N application between FPA and granular N applied either in spring or autumn at either rate of N fertiliser. In five other trials (i.e. Quin et al. Citation2006, Citation2015, Citation2017; Mackay Citation1996; Zaman and Blennerhassett Citation2009) that have compared pasture response from the FPA of N with granular urea, four reported an increase in pasture yield from FPA N. However, Morton et al. (Citation2019) noted the findings of these trials should be treated with caution because there was no statistical analysis of the data. Morton et al. (Citation2019) concluded that when FPA N fertiliser is applied to pasture at the same rate of N under the same environmental conditions and carefully controlled trial protocols, there doesn’t appear to be any significant difference in terms of pasture production between applying a fertiliser as FPA or its granular equivalent.
Table 10. Trials comparing the effect of fine particle application (FPA) and granular application of nitrogen (N) fertiliser on pasture response (adapted from Morton et al. Citation2019).
Precision agriculture and digital technologies
Precision agriculture uses remote and proximal sensing methods to collect data to help delineate and monitor within-field variably in soil and plant properties (Hedley Citation2015). This data can then be linked to variable rate technologies (VRT) and used to inform on farm spatial and temporal inputs such as N fertiliser. This has been made possible by the development and advancement of a range of technologies over the last couple of decades including geographic positioning systems (GPS), soil and crop sensors, geographic information systems (GIS), and the improvement in software and precision application of VRT (Hedley Citation2015; Shepherd et al. Citation2018). This technology has the potential to enhance productivity and minimise environmental effects from the application of N fertiliser. It also has the benefit of being able to generate data (e.g. type, rate and date of fertiliser application) that can be used for compliance reporting, increasingly being required by regulators in New Zealand.
Hedley (Citation2015) summarised some of the key advances in precision agriculture, some of which may benefit on-farm N fertiliser management in grazed pasture systems. Early advances in precision agriculture came about with the implementation of GPS/GIS and variable rate application of fertiliser from both ground and aerial vehicles that allowed the precise application of nutrients according to the agronomic requirement of the pasture or crop. Fertiliser application maps are loaded into a vehicle’s on-board computer system which control the release rate of the fertiliser being applied to the soil. In addition, exclusion zones (e.g. native bush, open waterways, wetlands) are included in the farm plan so that fertiliser is not applied to areas where it is not required.
There has been ongoing development in VRT to improve the precision of delivery of inputs such as fertiliser. The advent of automated flow control of aerial topdressing application systems has allowed more accurate spreading of fertiliser from aircraft (White et al. Citation2017). This has been tested in hill country pastures across farms in New Zealand (Murray and Yule Citation2007; Morton et al. Citation2016; White et al. Citation2017; Grafton et al. Citation2021). There have also been developments in the control of ground spreading of fertiliser (Lawrence et al. Citation2005; Hedley Citation2015; Wigley et al. Citation2017). For example, variable application placement control which incorporates a variable spreading disc speed and electrical adjustment of the delivery system allows the spreading width of fertiliser being applied from both sides of a truck to be individually controlled (Hedley Citation2015).
Another focus of research has been around developing and testing of optical sensors that use reflectance indices to collect data on pasture quality, quantity and foliar-N uptake that can be used to inform variable rate application of N fertiliser (Pullanagari et al. Citation2011, Citation2012, Citation2013; McVeagh et al. Citation2012; Roberts et al. Citation2015; King et al. Citation2021). Wigley et al. (Citation2017) tested the performance of various vegetation indices (i.e. Simple Ratio (SR), Water Index (WI), Normalised Difference Vegetation Index (NDVI)) measured from optical reflectance sensors to estimate biomass yield and N-uptake in an irrigated dairy pasture that had received different rates of N fertiliser (0, 10, 20, 40, 80 kg N ha−1). It was found that the sensors were able to detect differences in biomass and foliar N-uptake following defoliation of grazed pastures. Of the measured indices, SR showed the most linear and consistent relationships to N-uptake and DM yields over a range of foliar-N conditions, and it was concluded these optical sensors have potential to inform a real-time variable rate fertiliser application system.
Technology such as the C-DAX Pasturemeter (C-Dax Citation2021) have also been developed that use optical sensors to determine pasture height and measure pasture cover (Dalley et al. Citation2009; Dennis et al. Citation2015). It can be used as a stand-alone pasture meter or equipped with GPS to map pasture production to provide information for on farm feed budgeting and strategic variable N input decisions (Yule et al. Citation2013; Hedley Citation2015). King et al. (Citation2021) for example used variable rate application (VRA) technology to apply N fertiliser as a function of the pasture mass present at the time of application, generated using C-DAX across five trial farms over one season. However, on average there was no statistical difference between VRA of N fertiliser and N applied uniformly across the paddock on pasture production. There are also other technologies available such as ‘Pasture Reader’ (Dalley et al. Citation2009) that uses sonar technology for measuring pasture DM that could also assist in precision application of N fertiliser to pasture.
Remote systems (e.g. drones, LiDAR, and satellite imagery) to measure pasture are also being investigated (Bensemen Citation2013). For example, pasture cover can be measured by satellite through SPACE (Satellite Pasture and Cover Evaluation). This technology utilises Planet’s constellation of Dove satellites comprising over 130 satellites and field analysis software from Farmshots to provide an estimate of pasture cover by using algorithms to convert a NDVI reading to an estimate of pasture biomass (Macdonald Citation2017; Milsom et al. Citation2019; Anderson et al. Citation2020). These technologies have the potential to allow spatial N fertiliser application to match localised differences in N responsiveness.
Conclusions
This paper amalgamates and summarises some of the vast amount of research on N fertiliser use in grazed pasture systems undertaken in New Zealand over the last 50 years. It provides an overview of the research to raise awareness of the key findings, and importantly, to provide a pathway for decision makers to readily find and access the research on N fertiliser use and management. The key findings are:
Well-managed grass/clover pastures can respond to N fertiliser application, but the response is variable and depends on the rate, timing, carry-over effect of N fertiliser, and site conditions such as slope, aspect, soil fertility status and pasture composition.
The best time of year to apply N fertiliser to grazed pasture depends on when extra feed is required by the animal, and the ability of the pasture to respond to the application of N.
The response to late winter/early spring application of N fertiliser is generally higher and more reliable than N applied in autumn.
The application of N fertiliser can negatively affect the clover content in grass/clover pasture due to reduced stolon number and length, less stolon branching, inhibited biological N2 fixation, and increased competition for moisture and nutrients by the grass component in the sward.
Nitrogen derived from the application of fertiliser can be lost directly or indirectly (N excreted from animals) from soils under grazed pasture through NO3– leaching, NH3 volatilisation and N2O from denitrification.
Direct leaching from the application of N fertiliser is low if application rates are not excessive and are synchronised with active growth of the pasture.
Urine excreted by animals is the main factor affecting NO3– leaching losses.
Ammonia volatilisation losses from fertiliser are typically <15% of the N applied, while emissions of N2O from N fertiliser are approximately 4% of total agricultural emissions in New Zealand.
New technologies such as coated N fertiliser products, plant growth regulators, advances in precision agriculture and digital technologies have potential to be applied to grazed pasture systems to optimise N fertiliser use and minimise environmental impacts.
With increasing expectations from consumers and constraints by regulators on the impact of using N fertiliser, it is likely there will still need to be innovation in the future to develop new N fertiliser products and technologies to further minimise environmental impacts and improve the efficiency of N fertiliser use.
Acknowledgments
Knowledge advisors Cathy Peck and Deirdre Congdon for compiling and providing access to the many scientific papers and reports. Alice Baillie for drafting . Dr Stewart Ledgard and Dr Diana Selbie for comments on an earlier draft of the paper.
Disclosure statement
No potential conflict of interest was reported by the author(s).
Additional information
Funding
References
- Adhikari KP, Saggar S, Hanly JA, Guinto DF. 2018. Laboratory evaluation of urease inhibitors 2-NPT and nBTPT in reducing ammonia emissions from cattle urine applied in dairy-grazed pasture soils. In: LD Currie and CL Christensen, editors. Farm environmental planning – science, policy and practice. http://flrc.massey.ac.nz/publications.html. Occasional Report No. 31. Fertiliser and Lime Research Centre, Massey University, Palmerston North, New Zealand. 9 pages.
- Amberger A. 1989. Research on dicyandiamide as a nitrification inhibitor and future outlook. Communications in Soil Science and Plant Analysis. 20:1933–1955.
- Anderson G, Rawlings M, Ogle G. 2020. Mitigation of saturation in satellite pasture measurement via incorporation of a statistical pasture growth model. Journal of New Zealand Grasslands. 82:191–198.
- Anon. 2008. Nitrogen – principles of applying nitrogen fertiliser to pasture. DairyNZ Farm Fact 7-1. http://www.dairynz.co.nz/file/fileid/37255. Accessed 31 July 2012.
- Baber HL, Wilson AT. 1972. Nitrate pollution of groundwater in the Waikato region. Chemistry in New Zealand. 36:179–183.
- Bahmani I, Hazard L, Varlet-Grancher C, Betin M, Lemaire G, Matthew C, Thom ER. 2000. Differences in tillering of long- and short-leaved perennial ryegrass genetic lines under full light and shade treatments. Crop Science. 40:1095–1102.
- Bahmani I, Thom ER, Matthew C, Lemaire G. 2001. Productivity of grazed perennial ryegrass dairy pastures from different ecotypes under nitrogen and irrigation treatments. New Zealand Journal of Agricultural Research. 44:123–133.
- Bailey JS. 1998. Varying the ratio of (15) N-labelled ammonium and nitrate-N supplied to perennial ryegrass: effects on nitrogen absorption and assimilation, and plant growth. New Phytologist. 140:505–518.
- Ball CC, Parsons AJ, Rasmussen S, Shaw C, Rowarth JS. 2012. Seasonal differences in the capacity of perennial ryegrass to respond to gibberellin explained. In Proceedings of the New Zealand Grassland Association, Gore, New Zealand pp. 183–188.
- Ball PR. 1989. An introduction to the role of nitrogen fertilizers in New Zealand farming systems. In: White RE, Currie LD, editors. Nitrogen in New Zealand agriculture and horticulture. Occasional report no. 3. Palmerston North, New Zealand: Fertilizer and Lime Research Centre, Massey University; p. 1.
- Ball PR, Field TRO. 1982. Response to nitrogen as affected by pasture characteristics, season and grazing management. In: Lynch PB, editor. Nitrogen fertilisers in New Zealand agriculture. Auckland, New Zealand: Ray Richards Publisher; p. 45–64.
- Ball PR, Field TRO. 1985. Productivity and economics of legume-based pastures and grass swards receiving fertilizer nitrogen in New Zealand. In: Barnes RF, Ball PR, Brougham RW, Marten GC, Minson DJ, editors. Forage legumes for energy-efficient animal production. Springfield, USA: NTIS; p. 47–55.
- Ball PR, Luscombe PC, Grant DA. 1982. Nitrogen on hill country. In: Lynch PB, editor. Nitrogen fertilisers in New Zealand agriculture. Auckland, New Zealand: Ray Richards Publisher; p. 133–148.
- Ball PR, Molloy LF, Ross DJ. 1978. Influence of fertiliser nitrogen on herbage dry matter and nitrogen yields, and botanical composition of a grazed grass-clover pasture. New Zealand Journal of Agricultural Research. 21:47–55.
- Ball R. 1970. The use of nitrogenous fertilisers. II. Department of Scientific and Industrial Research Experiments. Dairyfarming Annual, Massey University. 72–81.
- Ball R, Brougham RW, Brock JL, Crush JR, Hoglund JH, Carran RA. 1979a. I. Introduction and general methods. New Zealand Journal of Experimental Agriculture. 7:1–5.
- Ball R, Inglis JAH, Mauger JH. 1976. Tactical application of fertiliser nitrogen to offset a seasonal feed shortage on a heavily stocked sheep farm in southern Hawke's Bay. Proceedings of the New Zealand Grassland Association. 37:166–181.
- Ball R, Keeney DR, Theobald PW, Nes P. 1979b. The nitrogen balance in urine-affected areas of New Zealand pasture. Agronomy Journal. 71:309–314.
- Barratt BIP, Barker GM, Addison PJ. 1996. Sitona lepidus Gyllenhal (Coleoptera: Curculionidae), a potential clover pest new to New Zealand. New Zealand Entomologist. 19:23–30.
- Beale ND, Talbot WD, Cameron KC, Di HJ, Narbey R. 2021. Measurement of nitrate leaching losses from lysimeters on a dairy farm following conversion from forestry. New Zealand Journal of Agricultural Research. DOI: 10.1080/00288233.2021.1977661.
- Beef + Lamb New Zealand. 2020. Compendium of New Zealand farm facts: 2020. 44th ed. Beef + Lamb New Zealand, Economic Service. https://beeflambnz.com/sites/default/files/data/files/Compendium-2020.pdf.
- Bensemen M. 2013. Assessment of standing herbage dry matter using a range imaging system [Master’s thesis]. New Zealand: University of Waikato.
- Betteridge K, Hoogendoorn CJ, Thorrold BS, Costall DA, Ledgard SF, Park-Ng ZA, Theobald PW. 2007. Nitrate leaching and productivity of some farming options in the Lake Taupo catchment. New Zealand Grassland Association. 69:123–129.
- Bhandral R, Saggar S, Bolan NS, Hedley MJ. 2007. Transformation of nitrogen and nitrous oxide emission from grassland soils as affected by compaction. Soil and Tillage Research. 94:482–492.
- Bishop PA, Liu HY, Hedley MJ, Loganathan P. 2008. New Zealand made controlled release coated urea increases winter growth rates of Italian ryegrass with lower N leaching than uncoated urea. Proceedings of the New Zealand Grassland Association. 70:85–89.
- Black AS, Sherlock RR, Cameron KC, Smith NP, Goh KM. 1985a. Comparison of three field methods for measuring ammonia volatilization from urea granules broadcast on to pasture. Journal of Soil Science. 36:271–280.
- Black AS, Sherlock RR, Smith NP. 1987a. Effect of timing of simulated rainfall on ammonia volatilization from urea, applied to soil of varying moisture content. Journal of Soil Science. 38:679–687.
- Black AS, Sherlock RR, Smith NP. 1987b. Effect of urea granule size on ammonia volatilization from surface-applied urea. Fertiliser Research. 11:87–96.
- Black AS, Sherlock RR, Smith NP, Cameron KC, Goh KM. 1984. Effect of previous urine application on ammonia volatilisation from 3 nitrogen fertilisers. New Zealand Journal of Agricultural Research. 27:413–416.
- Black AS, Sherlock RR, Smith NP, Cameron KC, Goh KM. 1985b. Effects of form of nitrogen, season and urea application rate on ammonia volatilisation from pastures. New Zealand Journal of Agricultural Research. 28:469–474.
- Blennerhassett JD. 2002. Pasture growth constraints on dry steep east coast hill country [Ph.D. thesis]. Palmerston North, New Zealand: Massey University.
- Bolan N, Saggar S, Singh J. 2004a. The role of inhibitors in mitigating nitrogen losses in grazed pasture. NZ Soil News. 52:52–58.
- Bolan NS, Saggar S, Luo J, Bhandral R, Singh J. 2004b. Gaseous emissions of nitrogen from grazed pastures: processes, measurements and modelling, environmental implications, and mitigation. Advances in Agronomy. 84:37–120.
- Brock JL, Hay MJM. 1996. A review of the role of grazing management on the growth and performance of white clover cultivars in lowland New Zealand pastures. Agronomy Society of New Zealand Special Publication No. 11 / Grassland Research and Practice Series No. 6.
- Bryant RH, Edwards GR, Robinson B. 2016. Comparing response of ryegrass-white clover pasture to gibberellic acid and nitrogen fertiliser applied in late winter and spring. New Zealand Journal of Agricultural Research. 59:18–31.
- Buckthought L, Clough TJ, Cameron KC, Di HJ, Shepherd MA. 2015a. Urine patch and fertiliser N interaction: effects of fertiliser rate and season of urine application on nitrate leaching and pasture N uptake. Agriculture, Ecosystems and Environment. 203:19–28.
- Buckthought LE, Clough TJ, Cameron KC, Di HJ, Shepherd MA. 2015b. Fertiliser and seasonal urine effects on N2O emissions from the urine-fertiliser interface of a grazed pasture. New Zealand Journal of Agricultural Research. 58:311–324.
- Cameron KC, Di HJ, Moir JL. 2013. Nitrogen losses from the soil/plant system: a review. Annals of Applied Biology. 162:145–173.
- Cameron KC, Di HJ, Moir JL. 2014. Dicyandiamide (DCD) effect on nitrous oxide emissions, nitrate leaching and pasture yield in Canterbury, New Zealand. New Zealand Journal of Agricultural Research. 57:251–270.
- Cameron KC, Rate AW, Noonan MJ, Moore S, Smith NP, Kerr LE. 1996. Lysimeter study of the fate of nutrients following subsurface injection and surface application of dairy pond sludge to pasture. Agriculture, Ecosystems and Environment. 58:187–197.
- Caradus JR, Chapman DF. 1991. Variability of stolon characteristics and response to shading in two cultivars of white clover (trifolium repens L.). New Zealand Journal of Agricultural Research. 34:239–247.
- Caradus JR, Pinxterhuis JB(INA), Hay RJM, Lyons T, Hoglund JH. 1993. Response of white clover cultivars to fertiliser nitrogen. New Zealand Journal of Agricultural Research. 36:285–295.
- Caradus JR, Woodfield DR, Stewart AV. 1996. Overview and vision for white clover. Agronomy Society of New Zealand Special Publication No. 11 /Grassland Research and Practice Series No. 6.
- Cardenas LM, Yan M, Misselbrook T, Chadwick DR. 2019. Impacts of inhibitor use on nitrous oxide and ammonia emissions, nitrate leaching and resulting crop yields. Proceedings of the International Fertiliser Society 836.
- Carey P. 2009. Effectiveness of using fertigation N compared to solid urea fertiliser-N on pasture growth. Report to Ballance Agri-Nutrients. 2.
- Carey PL, Jiang S, Roberts AHC. 2012. Pasture dry matter responses to the use of a nitrification inhibitor: a national series of New Zealand farm trials. New Zealand Journal of Agricultural Research. 55:63–72.
- Carran RA. 1978. Soil nitrogen and pasture management. Proceedings of the New Zealand Grassland Association. 40:44–50.
- Carran RA, Roger Ball P, Theobald PW, Collins MEG. 1982. Soil nitrogen balances in urine-affected areas under two moisture regimes in Southland. New Zealand Journal of Experimental Agriculture. 10:377–381.
- Catto WD, Roberts AHC. 2021. Soil and nutrient management: the practitioner’s perspective. Resilient Pastures – Grassland Research and Practice Series. 17:243–252.
- C-Dax Rapid Pasture Assessment Smart Technologies. 2021. Available: http://www.cdax.com/Images/featureproducts/c-dax_presentationof_pm.pdf.
- Chapman D, Pinxterhuis I, Ledgard S, Parsons T. 2020. White clover or nitrogen fertiliser for dairying under nitrate leaching limits. Animal Production. 20:78–83.
- Chapman DF, Crush JR, Lee JM, Cosgrove GP, Stevens DR, Rossi L, Popay AJ, Edwards GR, King WM. 2018b. Implications of grass–clover interactions in dairy pastures for forage value indexing systems. 6. Cross-site analysis and general discussion. New Zealand Journal of Agricultural Research. 61:255–284.
- Chapman DF, Lee JM, Rossi L, Cosgrove GP, Stevens DR, Crush JR, King WM, Edwards GR, Popay AJ. 2018a. Implications of grass–clover interactions in dairy pastures for forage value indexing systems. 1. Context and rationale. New Zealand Journal of Agricultural Research. 61:119–146.
- Christensen CL, Hedley MJ, Hanly JA, Horne DJ. 2012. Nitrogen loss mitigation using duration-controlled grazing: field observations compared to model outputs. Proceedings of the New Zealand Grassland Association. 74:115–121.
- Clark AD, Harris SL. 1996. White clover or nitrogen fertiliser for dairying? In: Woodfield DR, editor. White clover: New Zealand’s competitive edge. Grassland research and practice series no. 6. Palmerston North, New Zealand: New Zealand Grassland Association; p. 107–114.
- Clough TJ, Ledgard SF, Sprosen MS, Kear MJ. 1998. Fate of 15N labelled urine on four soil types. Plant and Soil. 199:195–203.
- Clough TJ, Sherlock RR, Cameron KC, Ledgard SF. 1996. Fate of urine nitrogen on mineral and peat soils in New Zealand. Plant and Soil. 178:141–152.
- Clough TJ, Sherlock RR, Rolston DE. 2005. A review of the movement and fate of N2O in the subsoil. Nutrient Cycling in Agroecosystems. 72:3–11.
- Cookson WR, Rowarth JS, Cameron KC. 2000. The effect of autumn applied 15N-labelled fertilizer on nitrate leaching in a cultivated soil during winter. Nutrient Cycling in Agroecosystems. 56:99–107.
- Craighead MD, Hayward JA, Howie AM. 1997. Evaluation of nitrate fertilisers as nitrogen sources for spring pasture. Proceedings of the New Zealand Grassland Association. 59:131–135.
- Crofoot AN, Crofoot EW, Hoogendoorn CJ, Litherland AJ, Garland CB. 2010. N-leaching in hill country; farmer led research. Proceedings of the New Zealand Grassland Association. 72:55–60.
- Crush JR, Cosgrove GP, Brougham RW. 1982. The effect of nitrogen fertiliser on clover nitrogen fixation in an intensively grazed Manawatu pasture. New Zealand Journal of Experimental Agriculture. 10:395–399.
- Crush JR, Cosgrove GP, Brougham RW. 1983. Nitrogen fixation during 1979-81 in 2 pastures on the Manawatu Plains. New Zealand Journal of Experimental Agriculture. 11:17–20.
- Dalley D, Clark D, Pairman D, Dynes R, Yule I, King W, Mata G. 2009. Technologies for measuring grass/crops. In: Proceedings of the South Island Dairy Event. Invercargill, New Zealand; p. 134–151.
- Davies PJ. 1995. The plant hormones: their nature, occurrence, and functions. In: Davies PJ, editor. Plant hormones: physiology, biochemistry and molecular biology. Dordrecht, The Netherlands: Kluwer; p. 1–12.
- Dawar K, Zaman M, Rowarth JS, Blennerhassett J, Turnbull MH. 2011. Urease inhibitor reduces N losses and improves plant bio-availability of urea applied in fine particle and granular forms under field conditions. Agriculture, Ecosystems and Environment. 144:41–50.
- Dawar K, Zaman M, Rowarth JS, Turnbull MH. 2012. Applying urea with urease inhibitor (N-(nbutyl thiophosphoric triamide) in fine particle application improves nitrogen uptake in ryegrass (Lolium perenne L.). Soil Science and Plant Nutrition. 58:309–318.
- Dawson AE, Turner P, Gorrie D. 2019. The effect of controlled release nitrogen fertiliser on pasture production on two dairy farms in Whataroa, South Westland. Occasional Report No. 32. (Eds. L.D. Currie and C.L. Christensen). Fertiliser and Lime Research Centre, Massey University, Palmerston North, New Zealand. 7 p. http://flrc.massey.ac.nz/publications.html.
- de Klein CAM, Bowatte S, Simon PL, Arango J, Cardenas LM, Chadwick DR, Pijlman J, Rees RM, Richards KG, Subbarao GV, Whitehead D. 2022. Accelerating the development of biological nitrification inhibition as a viable nitrous oxide mitigation strategy in grazed livestock systems. Biology and Fertility of Soils. 58:235–240.
- de Klein CAM, Cameron KC, Di HJ, Rys G, Monaghan RM, Sherlock RR. 2011. Repeated annual use of the nitrification inhibitor dicyandiamide (DCD) does not alter its effectiveness in reducing N2O emissions from cow urine. Animal Feed Science and Technology. 166–167:480–491.
- de Klein CAM, Li Z, Sherlock RR. 2004. Determination of the N2O emission factor from animal excreta or urea, following a winter application in two regions of New Zealand. Report for MAF Policy, Wellington, New Zealand, 31.
- de Klein CAM, Sherlock RR, Cameron KC, van der Weerden TJ. 2001. Nitrous oxide emissions from agricultural soils in New Zealand—a review of current knowledge and directions for future research. Journal of the Royal Society of New Zealand. 31:543–574.
- de Klein CAM, van Logtestijn RSP. 1996. Denitrification in grassland soils in The Netherlands in relation to irrigation, nitrogen application rate, soil water content and soil temperature. Soil Biology and Biochemistry. 28:231–237.
- Dennis SJ, Talyor AAL, O’Neill K, Clarke-Hill W, Dynes RA, Cox A, can Koten C, Jowett TWD. 2015. Pasture yield mapping: why & how. Journal of New Zealand Grasslands. 77:41–46.
- Di HJ, Cameron KC. 2000. Calculating nitrogen leaching losses and critical nitrogen application rates in dairy pasture systems using a semi-empirical model. New Zealand Journal of Agricultural Research. 43:139–147.
- Di HJ, Cameron KC. 2002. Nitrate leaching in temperate agroecosystems: sources, factors and mitigating strategies. Nutrient Cycling in Agroecosystems. 46:237–256.
- Di HJ, Cameron KC. 2003. Mitigation of nitrous oxide emissions in spray-irrigated grazed grassland by treating the soil with dicyandiamide, a nitrification inhibitor. Soil Use and Management. 19:284–290.
- Di HJ, Cameron KC. 2004. Treating grazed pasture soil with a nitrification inhibitor, eco-n™, to decrease nitrate leaching in a deep sandy soil under spray irrigation – a lysimeter study. New Zealand Journal of Agricultural Research. 47:351–361.
- Di HJ, Cameron KC. 2006. Nitrous oxide emissions from two dairy pasture soils as affected by different rates of a fine particle suspension nitrification inhibitor, dicyandiamide. Biology and Fertility of Soils. 42:472–480.
- Di HJ, Cameron KC. 2008. Sources of nitrous oxide from 15N-labelled animal urine and urea fertiliser with and without a nitrification inhibitor, dicyandiamide (DCD). Australian Journal of Soil Research. 46:76–82.
- Di HJ, Cameron KC. 2011. Inhibition of ammonium oxidation by a liquid formulation of 3,4- dimethylpyrazole phosphate (DMPP) compared with a dicyandiamide (DCD) solution in six New Zealand grazed grassland soils. Journal of Soils and Sediments. 11:1032–1039.
- Di HJ, Cameron KC. 2012. How does the application of different nitrification inhibitors affect nitrous oxide emissions and nitrate leaching from cow urine in grazed pastures? Soil Use and Management. 28:54–61.
- Di HJ, Cameron KC. 2016. Inhibition of nitrification to mitigate nitrate leaching and nitrous oxide emissions in grazed grassland: a review. Journal of Soils and Sediments. 16:1401–1420.
- Di HJ, Cameron KC. 2018. Ammonia oxidisers and their inhibition to reduce nitrogen losses in grazed grassland: a review. Journal of the Royal Society of New Zealand. 48:127–142.
- Di HJ, Cameron KC, Moore S, Smith NP. 1998. Nitrate leaching from dairy shed effluent and ammonium fertiliser applied to a free-draining pasture soil under spray or flood irrigation. New Zealand Journal of Agricultural Research. 41:263–270.
- Di HJ, Cameron KC, Podolyan A, Edwards GR, de Klein CAM, Dynes R, Woods R. 2016. The potential of using alternative pastures, forage crops and gibberellic acid to mitigate nitrous oxide emissions. Journal of Soils and Sediments. 16:2252–2262.
- During C. 1972. Fertiliser and soil in New Zealand farming. New Zealand Department of Agriculture Bulletin (Vol. 409). Wellington: Government Printer.
- During C, Weeda WC. 1968. Nitrogenous fertiliser in grassland farming. New Zealand Journal of Agriculture. 116:32–37.
- Edmeades DC. 2009. ProGibb-SG. An independent assessment of the science. A report to NuFarm Ltd. Hamilton, New Zealand.
- Edmeades DC. 2015. The evaluation of a controlled release nitrogen fertiliser. Journal of New Zealand Grasslands. 77:147–152.
- Edmeades DC, McBride RM. 2012. Evaluating the agronomic effectiveness of fertiliser products. Proceedings of the New Zealand Grassland Association. 74:217–224.
- Edmeades DC, McBride RM. 2017. Further field evaluation of the controlled release nitrogen fertiliser Smartfert. Journal of New Zealand Grasslands. 79:73–78.
- Eltilib AM, Ledgard SF. 1988. Production and nitrogen fixation by ‘grasslands kopu’ and ‘grasslands huia’ white clovers under different nitrogen regimes. New Zealand Journal of Agricultural Research. 31:325–330.
- Ettema PJ, Ledgard SF. 1992. Getting the best out of white clover. Proceedings of the Ruakura Farmers’ Conference. 44:72–76.
- Fertiliser Association of New Zealand. 2020. Fertiliser use in New Zealand; [accessed 1 May 2022]. https://www.fertiliser.org.nz/site/about/fertiliser_use_in_nz.aspx#phosphorus-use.
- Feyter C, O'Connor MB, Addison B. 1985. Effects of rates and times of nitrogen application on the production and composition of dairy pastures in Waikato District, New Zealand. New Zealand Journal of Experimental Agriculture. 13:247–252.
- Field TRO, Ball PR. 1978. Tactical use of fertiliser nitrogen. Proceedings of the Agronomy Society of New Zealand. 8:129–133.
- Fraser PM, Cameron KC, Sherlock RR. 1994. Lysimeter study of the fate of nitrogen in animal urine returns to irrigated pasture. European Journal of Soil Science. 45:439–447.
- Gazzard M, Bint J. 1992. Experience with nitrogen – is it feed or fertiliser? Proceedings of the Ruakura Farmers’ Conference. 44:84–86.
- Ghani A, Ledgard S, Wyatt J, Catto W. 2014. Agronomic assessment of gibberellic acid and cytokinin plant growth regulators with nitrogen fertiliser for increasing dry matter production and reducing the environmental footprint. Proceedings of the New Zealand Grassland Association. 76:177–182.
- Gillingham AG, Gray MH, Smith D. 1998. Pasture responses to phosphorus and nitrogen fertilisers on dry hill country. Proceedings of the New Zealand Grassland Association. 60:135–140.
- Gillingham AG, Morton JD, Gray MH. 2007. Pasture responses to phosphorus and nitrogen fertilisers on east coast hill country: total production from easy slopes. New Zealand Journal of Agricultural Research. 50:307–320.
- Gillingham AG, Morton JD, Gray MH. 2008a. Pasture responses to phosphorus and nitrogen fertilisers on east coast hill country: 2. Clover and grass production from easy slopes. New Zealand Journal of Agricultural Research. 51:85–97.
- Gillingham AG, Morton JD, Gray MH, Roberts AHC. 2008b. Pasture responses to phosphorus and nitrogen fertilisers on east coast hill country: 3. Production from steep slopes. New Zealand Journal of Agricultural Research. 51:425–437.
- Gillingham AG, Sheath GW, Gray MH, Webby RW. 2003. Management and nitrogen fertiliser options for increased pasture productivity in dryland hill systems. Legumes for Dryland Pastures. 43‒50.
- Glassey C, Roach CG, Lee JM, Clark DA. 2013. The impact of farming without nitrogen fertiliser for ten years on pasture yield and composition, milksolids production and profitability; a research farmlet comparison. Proceedings of the New Zealand Grasslands Association. 75:71–78.
- Goh KM, Edmeades DC, Hart PBS. 1979. Direct field measurements of leaching losses of nitrogen in pasture and cropping soils using tension lysimeters. New Zealand Journal of Agricultural Research. 22:133–142.
- Goulding K, Jarvis S, Whitmore A. 2008. Optimizing nutrient management for farm systems. Philosophical Transactions of the Royal Society of London. Series B, Biological Sciences. 363:667–680.
- Grafton MCE, Irwin ME, Sandoval-Cruz EA. 2021. Measuring the response of variable bulk solid fertiliser application by computer-controlled delivery from aircraft. New Zealand Journal of Agricultural Research. DOI: 10.1080/00288233.2021.1936573.
- Grant DA, Lambert MG. 1979. Nitrogen fixation in pasture V. Unimproved North Island hill country, “Ballantrae”. New Zealand Journal of Experimental Agriculture. 7:19–22.
- Guinto DF, Stafford A, Blennerhassett J, Catto W. 2018. Dry matter yield response of pasture to enhanced efficiency nitrogen fertilisers. In: Currie LD, Christensen CL, editors. Farm environmental planning – science, policy and practice. Occasional report no. 31. Palmerston North, New Zealand: Fertiliser and Lime Research Centre, Massey University; p. 5; http://flrc.masseys.ac.nz/publications.html.
- Harris AJ, Brown KR, Turner JD, Johnston JM, Ryan DL, Hickey MJ. 1973. Some factors affecting pasture growth in Southland. New Zealand Journal of Experimental Agriculture. 1:139–163.
- Harris SL, Clark DA. 1996. Effect of high rates of nitrogen fertiliser on white clover growth, morphology, and nitrogen fixation activity in grazed dairy pasture in northern New Zealand. New Zealand Journal of Agricultural Research. 39:149–158.
- Harris SL, Clark DA, Waugh CD, Clarkson FH. 1995. Nitrogen fertiliser effects on white clover in dairy pastures. Agronomy Society of New Zealand Special Publication No. 11 /Grassland Research and Practice Series No. 6.
- Harris SL, Penno JW, Bryant AM. 1994. Effects of high rates of nitrogen fertiliser on dairy pastures and production. Proceedings of the New Zealand Grassland Association. 56:27–31.
- Harris W. 1987. Population dynamics and competition. In: Baker MJ, Williams WM, editors. White clover. Wallingford: CAB International; p. 203–298.
- Hawke MF. 2007. Pasture responses to rates of nitrogen products on ash and peat soils in the Waikato. Report to Ballance Agri-Nutrients. 6 p.
- Hay RJM, Porter JR. 2006. The physiology of crop yield. Oxford: Blackwell Publishing Ltd; p. 1–275.
- Haynes RJ, Sherlock RR. 1986. Gaseous losses of nitrogen. In: Haynes RJ, editor. Mineral nitrogen in the plant – soil system. New York, NY, USA: Academic Press; p. 242–302.
- Hedley C. 2015. The role of precision agriculture for improved nutrient management on farms. Journal of the Science of Food and Agriculture. 95:12–19.
- Hepp C, Valentine I, Hodgson J, Gillingham AG, Kemp PD. 2003. Effects of grass suppression on legume abundance during two contrasting seasons in a summer-dry hill country site. In: Proceedings of the New Zealand Grassland Association Symposium—legumes for dryland pastures. Grassland research and practice series no. 11. Lincoln University; p. 123–130.
- Hoglund J. 1980. Nitrogen use on south island dairy farms. In: Proceedings of the Lincoln College Farmers’ Conference. Lincoln; p. 420–428.
- Hoglund JH, Brock JL. 1987. Nitrogen fixation in managed grasslands. In: Snaydon RW, editor. Managed grasslands: analytical studies. ecosystems of the world 17B. Amsterdam.: Elsevier; p. 187–196.
- Hoglund JH, Crush JR, Brock JL, Ball R, Carran RA. 1979. Nitrogen fixation in pasture XII. General discussion. New Zealand Journal of Experimental Agriculture. 7:45–51.
- Holland PT, During C. 1977. Movement of nitrate-N and transformations of urea-M under field conditions. New Zealand Journal of Agricultural Research. 20:479–488.
- Holmes CW, Wheeler JS. 1973. The use of nitrogenous fertilisers on the seasonal supply dairy farm, no. 3 dairy unit. Dairy Farming Annual, Massey University; p. 45–54.
- Hoogendoorn CJ, Betteridge K, Ledgard SF, Costall DA, Park ZA, Theobald PW. 2011. Nitrogen leaching from sheep, cattle and deer grazed pastures in the Lake Taupo catchment in New Zealand. Animal Production Science. 51:416–425.
- Hoogendoorn CJ, de Klein CAM, Rutherford AJ, Letica S, Devantier BP. 2008. The effect of increasing rates of nitrogen fertiliser and a nitrification inhibitor on nitrous oxide emissions from urine patches on sheep grazed hill country pasture. Australian Journal of Experimental Agriculture. 48:147–151.
- Hoogendoorn CJ, Lambert MG, Devantier BP, Theobald PW, Park ZA. 2017. Nitrogen fertiliser application rates and nitrogen leaching in intensively managed sheep grazed hill country pastures in New Zealand. New Zealand Journal of Agricultural Research. 60:154–172.
- Hudson AW, Woodcock JW. 1931. Nitrogen dressings on dairy pastures. Experiments on production, seasons 1928-29 and 1929-30. New Zealand Journal of Experimental Agriculture. 42:99–115.
- Jiang S, Carey PL, Roberts A, Kerse G. 2011. Comparison of three plant growth regulators and urea on a Canterbury dairy pasture. In: Currie LD, Christensen CL, editors. Adding to the knowledge base for the nutrient manager: occasional report no. 24. Fertiliser and Lime Research Centre. Palmerston North, New Zealand: Massey University; p. 1–5.
- Journeaux P, Wilton J, Archer L, Ford S, McDonald G. 2019. The value of nitrogen fertiliser to the New Zealand economy. Prepared for the Fertiliser Association of New Zealand.
- Keeney DR, Gregg PEH. 1982. Nitrogen fertilisers and the nitrogen cycle. In: Lynch PB, editor. Nitrogen fertilisers in New Zealand agriculture. Auckland, New Zealand: Ray Richards Publisher; p. 19–28.
- Kelliher FM, Clough TJ, Clark H, Rys G, Sedcole JR. 2008. The temperature dependence of dicyandiamide (DCD) degradation in soils: a data synthesis. Soil Biology and Biochemistry. 40:1878–1882.
- Kelliher FM, Cox N, van der Weerden TJ, de Klein CAM, Luo J, Cameron KC, Di HJ, Giltrap D, Rys G. 2014. Statistical analysis of nitrous oxide emission factors from pastoral agriculture field trials conducted in New Zealand. Environmental Pollution. 186:63–66.
- King WM, Pletnyakov P, Taylor A, Werner A, Ekanayake C. 2021. Variable-rate application of fertiliser – a tool for improving farm systems and environmental performance? Journal of New Zealand Grasslands. 83:201–214.
- Korte CJ. 1988. Nitrogen fertilizer responses on Gisborne-East coast pastures. Proceedings of the Agronomy Society of New Zealand. 18.
- Korte CJ, Gray MH, Smith DR. 1996. DAP slurry evaluations in Hawke’s Bay. Proceedings of the New Zealand Grassland Association. 57:127–132.
- Lambert MG, Clark DA. 1986. Effects of late-autumn nitrogen application on hill country pastures and sheep production. Proceedings of the New Zealand Grassland Association. 47:211–215.
- Lambert MG, Mackay AD, Devantier BP, McDougall DB, Barker DJ, Park-Ng ZA. 2003. Redefining the production potential of hill pastures using fertiliser nitrogen. Proceedings of the New Zealand Grassland Association. 65:29–34.
- Lambert MG, Roberts AHC, Morton JD. 2012. Nitrogen use on hill country: lessons from the national wise use of nitrogen focus farm project. In: Currie LD, Christensen CL, editors. Advanced nutrient management: gains from the past goals for the future. Occasional report no. 25. Palmerston North, New Zealand: Fertiliser and Lime Research Centre, Massey University; p. 9. http://flrc.massey.ac.nz/publications.html
- Lawrence H, Yule I, Jones J, Hedley M. 2005. Testing the viability of existing ground spread fertiliser spreaders to perform variable rate fertilisation. In: Stafford JV, editor. Proceedings of the 5th European Conference on Precision Agriculture/2nd European Conference, Precision Livestock Farming, Uppsala, Sweden. Adelaide, Australia: Curran Associates; p. 655–663.
- Leahy SC, Kearney L, Reisinger A, Clarke H. 2019. Mitigating greenhouse gas emissions from New Zealand pasture-based livestock farm system. Journal of New Zealand Grasslands. 81:101–110.
- Ledgard SF. 1989. Nitrogen fertiliser Use on pastures and crops. Ruakura: MAFTech.
- Ledgard SF, Brier GJ, Gillingham AG, Sheath, GW. 1983. Influence of some soil and pasture components on the growth of hill country pastures 2. Response to nitrogen fertiliser. New Zealand Journal of Experimental Agriculture. 11:251–256.
- Ledgard SF, Clark DA, Sprosen MS, Brier GJ, Nemaia EKK. 1996b. Nitrogen losses from grazed dairy pasture, as affected by nitrogen fertiliser application. Proceedings of the New Zealand Grassland Association. 57:21–25.
- Ledgard SF, de Klein CAM, Crush JR, Thorrold BS. 2000. Dairy farming, nitrogen losses and nitrate-sensitive areas. Proceedings of the New Zealand Society of Animal Production. 60:256–260.
- Ledgard SF, Luo J, Sprosen MS, Wyatt JB, Balvert SF, Lindsey SB. 2014. Effects of the nitrification inhibitor dicyandiamide (DCD) on pasture production, nitrous oxide emissions and nitrate leaching in Waikato, New Zealand. New Zealand Journal of Agricultural Research. 57:294–315.
- Ledgard SF, Penno JW, Sprosen MS. 1999. Nitrogen inputs and losses from clover/grass pastures grazed by dairy cows, as affected by nitrogen fertiliser application. Journal of Agricultural Science Cambridge. 132:215–225.
- Ledgard SF, Saunders MH. 1982. Effects of nitrogen fertiliser and urine on pasture performance and the influence of phosphorus and potassium status. New Zealand Journal of Agricultural Research. 25:541–547.
- Ledgard SF, Sprosen MS, Penno JW, Rajendram GS. 2001. Nitrogen fixation by white clover in pastures grazed by dairy cows: temporal variation and effects of nitrogen fertilization. Plant and Soil. 229:177–187.
- Ledgard SF, Sprosen MS, Steele KW. 1996a. Nitrogen fixation by nine white clover cultivars in grazed pasture, as affected by nitrogen fertilization. Plant and Soil. 178:193–203.
- Ledgard SF, Sprosen MS, Steele KW, West CP. 1995. Productivity of white clover cultivars under intensive grazing, as affected by high nitrogen fertiliser application. New Zealand Journal of Agricultural Research. 38:473–482.
- Ledgard SF, Steele KW. 1992. Biological nitrogen fixation in mixed legume/grass pastures. Plant and Soil. 141:137–153.
- Ledgard SF, Steele KW, Feyter C. 1988. Influence of time of application on the fate of 15N-labelled urea applied to dairy pasture. New Zealand Journal of Agricultural Research. 31:87–91.
- Ley TWS. 2020. Can fertigation increase nitrogen use efficiency in NZ dairy pastures? [thesis submitted in partial fulfilment of the requirement for the degree of a Master of Agricultural Science]. Lincoln University.
- Livestock Improvement Corporation Limited, DairyNZ Limited. 2021. New Zealand dairy statistics 2020-21. https://www.dairynz.co.nz/media/5794941/nz-dairy-statistics-2020-21-web.pdf
- Luo J, Ledgard SF, Lindsey SB. 2007. Nitrous oxide emission from application of urea on New Zealand pasture. New Zealand Journal of Agricultural Research. 50:1–11.
- Luo J, Sprosen M, Wise B, Lindsey L. 2010. The effectiveness of Sustain® in reducing ammonia volatilisation losses. Report to Summit QuinPhos. 8 pp.
- Luscombe PC. 1979. Nitrogen fertiliser responses on hill country pastures. Proceedings of the New Zealand Grassland Association. 41:155–162.
- Luscombe PC. 1980. Seasonal nitrogen deficiency in hill country pastures. New Zealand Journal of Experimental Agriculture. 8:117–122.
- Luscombe PC. 1981. Fertiliser nitrogen responses on two hill pastures of different phosphate fertiliser history. New Zealand Journal of Experimental Agriculture. 9:57–61.
- Luscombe PC, Fletcher RH. 1982. Nitrogen fertiliser on grazed hill pastures. Proceedings of the New Zealand Grassland Association. 43:171–181.
- Lynch PB. 1953. Nitrogen fertiliser trials by Department of Agriculture. Proceedings Waikato Nitrogen Conference. Hamilton; p. 17–19.
- Lynch PB. 1967. A survey of the responses of pastures and crops to nitrogenous fertilisers in New Zealand. 11th Technical Conference of the New Zealand Fertiliser Manufacturers Research Association. 35–40.
- Macdonald D. 2017. Dairy data: utilising technology for decision making in pasture-based dairy farming. Nuffield Australia 46p. https://www.nuffieldscholar.org/reports.
- Mackay AD. 1996. Wairarapa Fertiliser Demonstration. AgResearch final report, 6 p.
- Matthew C, Chu ACP, Korte CJ. 1986. Improved pasture response to nitrogen applied in winter after application of urea in autumn. New Zealand Journal of Experimental Agriculture. 14:51–56.
- Matthew C, Hofmann WA, Osborne MA. 2009. Pasture response to gibberellins: a review and recommendations. New Zealand Journal of Agricultural Research. 52:213–225.
- McCloy BL. 2009. Liquid urea on pasture – Burnham, NZ Arable Trial Code Pa0819, Confidential report prepared for Ballance AgriNutrients.
- McLaren RG, Cameron KC. 1996. Soil science. 2nd ed. Australia and New Zealand: Oxford University Press; p. 304.
- McVeagh P, Yule IJ, Grafton M. 2012. Pasture yield mapping from your groundspread truck. In: Currie LD, Christensen CL, editors. Advanced nutrient management: gains from the past – goals for the future. Occasional report no. 25. Palmerston North, New Zealand: Fertilizer and Lime Research Centre, Massey University. http://flrc.massey.ac.nz/publications.html
- Menneer JC, Sprosen MS, Ledgard SF. 2008. Effect of timing and formulation of dicyandiamide (DCD) application on nitrate leaching and pasture production in a Bay of Plenty pastoral soil. New Zealand Journal of Agricultural Research. 51:377–385.
- Milsom A, Bell O, Bailey K, Brown SC, Barton RA, Moreno Garcia CA, Chen A, Bryant RH, Maxwell MR, Eady CC. 2019. Assessing the ability of a stationary pasture height sensing device to estimate pasture growth and biomass. Journal of New Zealand Grasslands. 81:61–68.
- Mitchell KJ, Lucanus R. 1962. Growth of pasture under controlled environment. III. Growth at various levels of constant temperature with 8 and 16 h of uniform light per day. New Zealand Journal of Agricultural Research. 5:135–144.
- Moir JL, Cameron KC, Di HJ. 2007. Effects of the nitrification inhibitor dicyandiamide on soil mineral N, pasture yield, nutrient uptake and pasture quality in a grazed pasture system. Soil Use and Management. 23:111–120.
- Monaghan RM, Cameron KC, McLay CDA. 1989. Leaching losses of nitrogen from sheep urine patches. New Zealand Journal of Agricultural Research. 32:237–244.
- Monaghan RM, de Klein CAM. 2014. Integration of measures to mitigate reactive nitrogen losses to the environment from grazed pastoral dairy systems. Journal of Agricultural Science. 152:S45–S56.
- Monaghan RM, Hedley MJ, Di HJ, McDowell RW, Cameron KC, Ledgard SF. 2007. Nutrient management in New Zealand pastures – recent developments and future issues. New Zealand Journal of Agricultural Research. 50:181–201.
- Monaghan RM, Paton RJ, Drewry JJ. 2002. Nitrogen and phosphorus losses in mole and tile drainage from a cattle-grazed pasture in eastern Southland. New Zealand Journal of Agricultural Research. 45:197–205.
- Monaghan RM, Paton RJ, Smith LC, Drewry JJ, Littlejohn RP. 2005. The impacts of nitrogen fertilisation and increased stocking rate on pasture yield, soil physical condition and nutrient losses in drainage from a cattle-grazed pasture. New Zealand Journal of Agricultural Research. 48:227–240.
- Monaghan RM, Smith LC. 2004. Minimising surface water pollution resulting from farm-dairy effluent application to mole-pipe drained soils. II. The contribution of preferential flow of effluent to whole-farm pollutant losses in subsurface drainage from a West Otago dairy farm. New Zealand Journal of Agricultural Research. 47:417–429.
- Monaghan RM, Smith LC, Ledgard SF. 2009. The effectiveness of a granular formulation of dicyandiamide (DCD) in limiting nitrate leaching from a grazed dairy pasture. New Zealand Journal of Agricultural Research. 52:145–159.
- Monaghan RM, Smith LC, Muirhead RW. 2016. Pathways of contaminant transfers to water from an artificially-drained soil under intensive grazing by dairy cows. Agriculture, Ecosystems and Environment. 220:76–88.
- Morris ST, McRae AF. 1990. Evaluation of nitrogen fertiliser in a beef production system. Proceedings of the New Zealand Grassland Association. 51:89–92.
- Morton J, Tillman R, Morton A. 2019. Review of research on pasture yield responses to fine particle application of fertiliser in New Zealand. New Zealand Journal of Agricultural Research. 62:210–223.
- Morton JD, Roberts AHC. 2006. Pasture production responses to fertiliser on renovated west coast Pakihi soils. Proceedings of the New Zealand Grassland Association. 68:331–338.
- Morton JD, Stafford AD, Gillingham AG, Old A, Knowles O. 2016. The development of variable rate application of fertiliser from a fixed wing topdressing aircraft. Hill Country – Grassland Research and Practice. Series. 16:163–168.
- Mouat MCH, Hay MJM, Crush JR, Nes P, Dunlop J, Hart AL. 1987. Effects of nitrogen and phosphorus applications on pasture growth and composition on a recent alluvial soil with low phosphorus retention. New Zealand Journal of Experimental Agriculture. 15:143–146.
- Moustafa E, Ball R, Field TRO. 1969. The use of acetylene reduction to study the effect of nitrogen fertiliser and defoliation on nitrogen fixation by field-grown white clover. New Zealand Journal of Agricultural Research. 12:691–696.
- Muir PD, Thomson BC, Smith NB. 2006. Applying nitrogen as a granule or slurry – does application method really matter? Report on 2005/2006 Smedley Station Trial. On-Farm Research Ltd, 6 p. New Zealand Grassland Association 57: 127–132.
- Müller C. 1995. Nitrous oxide emission from intensive grassland in Canterbury, New Zealand [Unpublished PhD thesis]. Lincoln, New Zealand: Lincoln University.
- Mundy GN. 1990. Recovery of nitrogen fixation in clover following a nitrogen fertiliser application. Institute for Sustainable Agriculture Research Report 1989–90: 109.
- Murphy PM, Ball PR. 1985. Effect of early season nitrogen on nitrogen fixation in a ryegrass/white clover pasture. Proceedings International Grassland Congress. 15:460–461.
- Murray RI, Yule IJ. 2007. Developing variable rate application technology: scenario development and agronomic evaluation. New Zealand Journal of Agricultural Research. 50:53–63.
- Mytton L. 1987. The role of rhizobium in hill clover establishment. In: Hardcastle JEY, editor. Science and better use of grassland. Agricultural and Food Research Council; p. 8–9.
- O’Connor MB. 1973. Nitrogen on pastures in New Zealand. Proceedings 14th Technical Conference New Zealand Fertiliser Manufactures Research Association, 68–73.
- O'Connor MB. 1974. Conclusions from MAF trials with nitrogen. New Zealand Fertiliser Journal. 43:18.
- O’Connor MB. 1982. Nitrogen fertilisers for the production of out-ot-season grass. In: Lynch PB, editor. Nitrogen fertilisers in New Zealand agriculture. p. 65–76.
- O'Connor MB, Cumberland GLB. 1973. Nitrogen responses on pasture. Proceedings of the Ruakura Farmers’ Conference. 137–144.
- O’Connor MD, Gregg PEH. 1971. Nitrogen fertiliser trials on pasture. Proceedings of the New Zealand Grassland Association. 33:26–34.
- O’Connor OCKF. 1961. Nitrogen and grassland production of the midaltitude zone of Canterbury, New Zealand. I. The effects of different levels of nitrogen fertiliser on herbage and nitrogen yields of cultivated pastures. New Zealand Journal of Agricultural Research. 4:686–697.
- OECD. 2003. Cyanoguanidine. SIDS initial assessment report. Arona, Italy: UNEP Publications; p. 75.
- Parker WJ, Tillman RW, Gray DI. 1989. Management considerations in nitrogen fertiliser usage on a Wairarapa sheep farm. In: White RE, Currie LD, editors. Occasional report no. 3. Palmerston North, New Zealand: Fertilizer and Lime Research Centre, Massey University; p. 65–78.
- Parsons AJ, Rasmussen S, Liu Q, Xue H, Ball C, Shaw C. 2013. Plant growth - resource or strategy limited: insights from responses to gibberellin. Grass and Forage Science. 68:577–588.
- Penno JW. 1993. A comparison of the effect of split and single applications of nitrogen fertiliser on dairy production. Proceedings of the New Zealand Society of Animal Production. 53:33–35.
- Puha MR, King WM, Burggraaf VT, Roberts AHC. 2008. Pasture production gains from strategic winter nitrogen applications on a North Island sheep and beef hill country farm. Proceedings of the New Zealand Grassland Association. 70:117–122.
- Pullanagari RR, Yule I, King W, Dalley D, Dynes R. 2011. The use of optical sensors to estimate pasture quality. International Journal on Smart Sensing and Intelligent Systems. 4:125–137.
- Pullanagari RR, Yule IJ, Tuohy MP, Hedley MJ, Dynes RA, King WM. 2012. In-field hyperspectral proximal sensing for estimating quality parameters of mixed pasture. Precision Agriculture. 13:351–369.
- Pullanagari RR, Yule IJ, Tuohy MP, Hedley MJ, Dynes RA, King WM. 2013. Proximal sensing of the seasonal variability of pasture nutritive value using multispectral radiometry. Grass Forage Science. 68:110–119.
- Qiu W, Di HJ, Cameron KC, Chengxiao H. 2010. Nitrous oxide emissions from animal urine as affected by season and a nitrification inhibitor dicyandiamide. Journal of Soils and Sediments. 10:1229–1235.
- Quin BF, Gillingham AG, Baird D, Spilsbury S, Gray M. 2015. A comparison under grazing of pasture production, pasture N content and soil mineral N levels between granular urea ONEsystem® on two contrasting dairy farms in New Zealand. Proceedings of the New Zealand Grassland Association. 77:259–267.
- Quin BF, Harold S, Spilsbury S, Bates G. 2017. Commercialisation of ONEsystem® (wetted NBPTtreated prilled urea) in New Zealand and Victoria, Australia). In: Currie LD, Hedley MJ, editors. Science and policy: nutrient management challenges for the next generation. Occasional report no. 30. Palmerston North, New Zealand: Fertiliser and Lime Research Centre, Massey University; p. 10.
- Quin BF, Rowarth JS, Blennerhassett JD, Crush JR, Cornforth IS. 2006. Removing the barriers to improved response to fertiliser N – the plants perspective. In: Currie LD, Hanly JA, editors. Implementing sustainable nutrient management strategies in agriculture. Fertiliser and Lime Research Centre occasional report no. 19. Palmerston North: Massey University; p. 368–382.
- Rajendram GS, Waller J, Cameron C, Longhurst R, Luo J, Ghani A, O'Connor M. 2009. Nitrogen fertiliser trial database: a valuable resource. Climatic factors and first cut response to nitrogen application. In: Currie LD, Lindsay CL, editors. Nutrient management in a rapidly changing world. Palmerston North, NZ: Fertiliser and Lime Research Centre, Massey University; p. 191–204.
- Risk JT, Dawson AE. 2019. Comparing fine particle and granular nitrogen response on Southland pastures. In: Currie LD, Christensen CL, editors. Nutrient loss mitigations for compliance in agriculture. Occasional report no. 32. Palmerston North, New Zealand: Fertiliser and Lime Research Centre, Massey University. http://flrc.massey.ac.nz/ publications.html
- Risk WH. 1982. The use of nitrogen fertilisers on the Southland Plains. In: Lynch PB, editor. Nitrogen fertilisers in New Zealand agriculture. p. 149–158.
- Roberts AHC, Ledgard SF, O’Connor MB, Thomson NA. 1992. Effective use of N fertiliser—research and practice. Proceedings of the Ruakura Farmers’ Conference. 44:77–83.
- Roberts AHC, Thomson NA. 1989. Use of nitrogen fertiliser for intensive dairy production. In: White RE, Currie LD, editors. Nitrogen in New Zealand agriculture and horticulture. Occasional report no. 3. Palmerston North, New Zealand: Fertilizer and Lime Research Centre, Massey University; p. 42–55.
- Roberts AHC, White MD. 2016. From there to where? Past, present and future soil fertility management on hill country farms. Hill Country – Grassland Research and Practice Series. 16:127–136.
- Roberts J, Suarez Infiesta A, Schäbitz B, Fourie J, Werner A. 2015. Active optical sensing of canopies in pasture management. Journal of New Zealand Grasslands. 77:35–40.
- Rogers J, Little ECS. 1982. Historical summary of the use of nitrogen fertilisers in New Zealand. In: Lynch PB, editor. Nitrogen fertilisers in New Zealand agriculture. p. 159–170.
- Ryden J, Ball P, Garwood E. 1984. Nitrate leaching from grassland. Nature. 311:50–53.
- Saggar S, Luo J, Giltrap DL, Maddena M. 2009. Nitrous oxide emission processes, measurements, modeling and mitigation. In: Sheldon AI, Barnhart EP, editors. Nitrous oxide emissions research progress. New York, NY, USA: Nova Science Publishers Inc; p. 1–66.
- Saggar S, Singh J, Giltrap DL, Zaman M, Luo J, Rollo M, Kim D-G, Rys G, Van der Weerden TJ. 2013. Quantification of reductions in ammonia emissions from fertiliser urea and animal urine in grazed pastures with urease inhibitors for agricultural inventory: New Zealand as a case study. Science of the Total Environment. 465:136–146.
- Scott RS. 1963. Nitrogen fertilisers for out-of-season growth. New Zealand Journal of Agriculture. 106:117–121.
- Selbie DR, Buckthought LE, Shepherd MA. 2015. The challenge of the urine patch for managing nitrogen in grazed pasture systems. Advances in Agronomy. 129:229–292.
- Selvarajah N, Sherlock RR, Cameron KC. 1989. Effect of different soils on ammonia volatilization losses from surface applied urea granules. In: White RE, Currie, LD, editors. Nitrogen in New Zealand agriculture and horticulture. Occasional report no. 3. Palmerston North, New Zealand: Fertilizer and Lime Research Centre. Massey University; p. 145–156.
- Selvarajah N, Sherlock RR, Cameron KC. 1993. Ammonia volatilisation potentials of New Zealand agricultural soils broadcast with granular urea. In: Land treatment collective papers for technical session no. 8, c/o Forest Research Institute. Rotorua, New Zealand.
- Shepherd M, Lucci G. 2012. Fertiliser use: responses to nitrogen and phosphorus. DairyNZ Technical Series. Issue 12.
- Shepherd M, Philips P, Lucci G, Macdonald KA, Glassey C, Roach C, Woodward S, Griffiths W. 2014. Comparison of two dairy grazing systems in the Waikato region of New Zealand: early results on nitrate leaching. Lisbon: 18th Nitrogen Workshop.
- Shepherd M, Turner JA, Small B, Wheeler D. 2018. Priorities for science to overcome hurdles thwarting the full promise of the ‘digit agriculture’ revolution. Journal of the Science of Food and Agriculture. 100:5083–5092.
- Shepherd MA, Lucci GM. 2011. Nitrogen fertiliser advice – what progress can we make? In: Currie LD, Christensen CL, editors. Adding to the knowledge base for the nutrient manager. Occasional report no. 24. Palmerston North, New Zealand: Fertiliser and Lime Research Centre, Massey University; p. 11. http://flrc.massey.ac.nz/publications.html
- Sherlock RR, Goh KM. 1984. Dynamics of ammonia volatilization from simulated urine patches and aqueous urea applied to pasture I. Field experiments. Nutrient Cycling in Agroecosystems. 5:181–195.
- Sherlock RR, O' Connor BM. 1973. The use of nitrogen on hill country. Proceedings of the New Zealand Grassland Association. 35:52–62.
- Silva R, chr K, Di H, Hendry T. 1999. A lysimeter study of the impact of cow urine, dairy shed effluent and nitrogen fertilizer on drainage water quality. Australian Journal of Soil Research. 37:357–369.
- Silva RG, Cameron KC, Di HJ, Jorgensen EE. 2005. A lysimeter study to investigate the effect of dairy effluent and urea on cattle urine N losses, plant uptake and soil retention. Water, Air and Soil Pollution. 164:57–78.
- Singh J. 2007. The role of inhibitors in mitigating nitrogen losses from cattle urine and nitrogen fertiliser inputs in pastures [Unpublished PhD Thesis]. New Zealand: Massey University.
- Singh J, Bolan NS, Saggar S, Zaman M, et al. 2008. The role of inhibitors in controlling the bioavailability and losses of nitrogen. In: Naidu R, editor. Chemical bioavailability in terrestrial environment. Amsterdam: Elsevier; p. 329–362.
- Smith LC, de Klein CAM, Monaghan RC, Catto WD. 2008. The effectiveness of dicyandiamide in reducing nitrous oxide emissions from a cattle-grazed, winter forage crop in Southland, New Zealand. Australian Journal of Experimental Agriculture. 48:160–164.
- Smith LC, Morton JD, Catto WD, Trainor KD. 2000. Nitrogen responses on pastures in the southern South Island of New Zealand. Proceedings of the New Zealand Grassland Association. 62:19–23.
- Sprent JI. 1979. The biology of nitrogen-fixing organisms. London: McGraw-Hill Publishers.
- Sprosen M, Ledgard S. 2008. A comparison of the effectiveness of two coated urea products in reducing volatilsation losses from urea. Report for Ballance Agri-Nutrients, February 2008; p. 10.
- Sprosen M, Ledgard SF, Lindsey SB, Macdonald KA. 2002. Effect of stocking rate on leaching of nitrate and associated nutrients. In: Currie LD, Loganathan P, editors. Dairy farm soil management.
- Stats NZ. 2021. Fertilisers – nitrogen and phosphorus. https://www.stats.govt.nz/indicators/fertilisers-nitrogen-and-phosphorus.
- Steele KW. 1982. Nitrogen in grassland soils. In: Lynch PB, editor. Nitrogen fertilisers in New Zealand agriculture. New Zealand Institute of Agricultural Science; p. 29–44.
- Steele KW, Dawson TE. 1980. Nitrogen as an aid to pasture establishment and renovation in Northland. New Zealand Journal of Experimental Agriculture. 8:123–129.
- Steele KW, Judd MJ, Shannon PW. 1984. Leaching of nitrate and other nutrients from a grazed pasture. New Zealand Journal of Agricultural Research. 27:5–11.
- Steele KW, O’Connor MB, Ledgard SF. 1981. Efficient use of nitrogen fertiliser. Proceedings of the Ruakura Farmers’ Conference. 33:53–56.
- Steele KW, Percival NS. 1984. Nitrogen fertiliser application to pastures under Pinus radiata. New Zealand Journal of Agricultural Research. 27:49–55.
- Sun X, Luo N, Longhurst B, Luo J. 2008. Fertiliser nitrogen and factors affecting pasture responses. The Open Agriculture Journal. 2:35–42.
- Sutton MA, Skiba UM, van Grinsven HJM, Oenema O, Watson CJ, Williams J, Hellums DT, Maas R, Gyldenkaerne S, Pathak H, Winiwarter W. 2014. Green economy thinking and the control of nitrous oxide emissions. Environmental Development. 9:76–85.
- Syers JK. 1982. Introduction. In: Lynch PB, editor. Nitrogen fertilisers in New Zealand agriculture. p. 11–18.
- Taranaki Catchment Commission. 1987. Nitrates in groundwater: Taranaki regional survey. Stratford: Taranaki Catchment Commission; p. 1–42.
- Theobald P, Ledgard S, Sprosen M. 2008. The effectiveness of two coated urea products in improving the efficiency of urea applied to a Manawatu silt loam soil. Report for Ballance Agri-Nutrients, June 2008; p. 13.
- Theobald PW, Ball PR. 1984. Nitrogen lost by ammonia volatilisation, and the effectiveness of urea and ammonium sulphate fertilisers. Proceedings of the New Zealand Grassland Association. 45:236–238.
- Thomas R. 1992. The role of legumes in the nitrogen cycle of productive and sustainable pastures. Grass and Forage Science. 47:133–142.
- Trainor K. 2007. Data sheet report to Ballance Agri-Nutrients on effectiveness of FPA and granular forms of urea; p. 1.
- van der Weerden TJ, Cox N, Luo J, Di HJ, Podolyan A, Phillips RL, Saggar S, de Klein CAM, Ettema P, Rys G. 2016b. Refining the New Zealand nitrous oxide emission factor for urea fertiliser and farm dairy effluent. Agriculture, Ecosystems and Environment. 222:133–137.
- van der Weerden TJ, Luo J, Di HJ, Podolyan A, Phillips RL, Saggar S, de Klein CAM, Cox N, Ettema P, Rys G. 2016a. Nitrous oxide emissions from urea fertiliser and effluent with and without inhibitors applied to pasture. Agriculture, Ecosystems and Environment. 219:58–70.
- van der Weerden TJ, Luo J, Styles T, Wise B, Lindsay S. 2011. N fertilisers amended with inhibitors to reduce N2O and NH3 losses. Report to Ballance Agri-Nutrients. 30 p.
- van der Weerden TJ, Manderson A, Kelliher FM, de Klein CAM. 2014. Spatial and temporal nitrous oxide emissions from dairy cattle urine deposited onto grazed pastures across New Zealand based on soil water balance modelling. Agriculture, Ecosystems and Environment. 189:92–100.
- van der Weerden TJ, Noble AN, Luo J, de Klein CAM, Saggar S, Giltrap D, Gibbs J, Rys G. 2020. Meta-analysis of New Zealand's nitrous oxide emission factors for ruminant excreta supports disaggregation based on excreta form, livestock type and slope class. Science of The Total Environment. 732.
- van Rossum MH, Bryant RH, Edwards GR. 2013. Response of simple grass-white clover and multi- species pastures to gibberellic acid or nitrogen fertiliser in autumn. Proceedings of the New Zealand Grassland Association. 75:145–150.
- Vogeler I, Lucci G, Shepherd M. 2016. An assessment of the effects of fertilizer nitrogen management on nitrate leaching risk from grazed dairy pasture. Journal of Agricultural Science. 154:407–424.
- Walker TW, Ludecke TE. 1982. An economic evaluation of the use of nitrogen fertilisers on field crops in New Zealand. In: PB Lynch, editor. Nitrogen fertilisers in New Zealand agriculture. N.Z. Institute of Agricultural Science, Wellington; p. 95–119.
- Watkins N, Shepherd M. 2015. A compendium of New Zealand pasture farmlet experiments measuring nitrogen leaching. In: Currie LD, Burkitt LL, editors. Moving farm systems to improved attenuation. Occasional report no. 28. Palmerston North, New Zealand: Fertilizer and Lime Research Centre, Massey University.
- White MD, Metherell AK, Roberts AHC, Meyer RE, Cushnahan TA. 2017. Economics of a variable rate fertilizer strategy on a Whanganui hill country station. Journal of New Zealand Grasslands. 79:119–224.
- Whitehead D, Edwards G. 2015. Assessment of the application of gibberellins to increase productivity and reduce nitrous oxide emissions in grazed grassland. Agriculture, Ecosystems and Environment. 207:40–50.
- WHO. 1984. Guidelines for drinking-water quality, volume 1. Recommendations. Geneva, Switzerland: World Health Organization.
- Wigley K, Owens J, Trethewey J, Ekanayake D, Roten R, Werner A. 2017. Optical sensors for variable rate nitrogen application in dairy pastures. Journal of New Zealand Grasslands. 79:223–227.
- Williams PH, Paterson J. 1983. Response of pastures to nitrogen fertiliser applied in autumn and spring on the West Coast of the South Island, New Zealand. New Zealand Journal of Experimental Agriculture. 11:247–250.
- Woods RR, Cameron KC, Edwards GR, Di HJ, Clough TJ. 2016. Effects of forage type and gibberellic acid on nitrate leaching losses. Soil Use and Management. 32:565–572.
- Yule I, Lawrence H, Mackenzie C, Hedley C, Grafton M, Pullanagari R. 2013. Case studies which demonstrate the financial viability of precision dairy farming, in Proceedings of the 22nd International Grassland Congress ‘Revitalising Grasslands to Sustain our Communities’, 15–19 September 2013, Sydney. NSW Dept of Primary Industries, Australia. Printed by PBA Printers in conjunction with CSIRO Publishing, pp. 610–613.
- Zaman M, Blennerhassett JD. 2009. Can fine particle application of fertilisers improve N use efficiency in grazed pastures. In: Currie LD, Lindsay CL, editors. Nutrient management in a rapidly changing world. Occasional report no. 22. Palmerston North, New Zealand: Fertiliser and Lime Research Centre, Massey University; p. 257–264.
- Zaman M, Blennerhassett JD. 2010. Effects of the different rates of urease and nitrification inhibitors on gaseous emissions of ammonia and nitrous oxide, nitrate leaching and pasture production from urine patches in an intensive grazed pasture system. Agriculture, Ecosystems and Environment. 136:236–246.
- Zaman M, Ghani A, Kurepin LV, Pharis RP, Khan S, Smith TJ. 2014. Improving ryegrass-clover pasture dry matter yield and urea efficiency with gibberellic acid. Journal of the Science of Food and Agriculture. 94:2521–2528.
- Zaman M, Nguyen ML. 2012. How application timings of urease and nitrification inhibitors affect N losses from urine patches in pastoral system. Agriculture, Ecosystems and Environment. 156:37–148.
- Zaman M, Nguyen ML, Blennerhassett JD, Quin BN. 2008. Reducing NH3, N2O and NO3-−N losses from a pasture soil with urease or nitrification inhibitors and elemental S-amended nitrogenous fertilisers. Biology and Fertility of Soils. 44:693–705.
- Zaman M, Saggar S, Blennerhassett JD, Singh J. 2009. Effect of urease and nitrification inhibitors on N transformation, gaseous emissions of ammonia and nitrous oxide, pasture yield and N uptake in grazed pasture system. Soil Biology Biochemistry. 41:1270–1280.
- Zaman M, Saggar S, Stafford AD. 2013a. Mitigation of ammonia losses from urea applied to a pastoral system: the effect of nBTPT and timing and amount of irrigation. New Zealand Grassland Association. 75:121–126.
- Zaman M, Zaman S, Adhinarayanan C, Nguyen ML, Nawaz S, Dawar KM. 2013b. Effects of urease and nitrification inhibitors on the efficient use of urea for pastoral systems. Soil Science and Plant Nutrition. 59:649–659.
- Zaman M, Zaman S, Nguyen ML, Smith TJ, Nawaz S. 2013c. The effect of urease and nitrification inhibitors on ammonia and nitrous oxide emissions from simulated urine patches in pastoral system: a two-year study. Science of the Total Environment. 465:97–106.