ABSTRACT
Silvopastoral systems have complex impacts on a diverse range of outcomes, making it essential to design these systems using an integrative approach to maximise positive impacts to farms. This paper comprises firstly a systematic review of global silvopastoral processes, and secondly stakeholder-driven synthesis of key opportunities and challenges for future silvopastoralism situated in the context of New Zealand. The systematic review demonstrated that although under-researched, livestock interactions can have overriding influences on the system, and that the traditional functional traits that are typically deemed important for selection (N2-fixing trees v non N2-fixing trees, evergreen v deciduous) do not show consistent positive impacts on the agroecological environment. From the New Zealand silvopastoral participatory case study, including the stakeholder workshop, we synthesised 5 key principles that should be considered in future system designs. These were: (1) silvopastoral systems are complex and require holistic management; (2) the views, values and experiences of local people are deeply connected to silvopastoral system design; (3) spatial heterogeneity in environmental and social conditions requires locally specific decisions; (4) understanding of ecological processes must underpin all management decisions; and (5) the complexity and spatial heterogeneity present in silvopastoral systems requires high-resolution data and tools.
Introduction
Silvopastoralism is an integrative and holistic land management practice that has great potential for reducing landscape degradation and the environmental impact of farms (Jose and Dollinger Citation2019; Zhu et al. Citation2020; Plieninger et al. Citation2021; Nair et al. Citation2022). Silvopastoralism is a type of agroforestry, where agroforestry is defined as an agricultural system where woody perennials (typically a tree) interacts biologically with a crop that is managed for forage, annual or perennial crop production (Somarriba Citation1992). Silvopasture specifically describes the integration of woody perennials into pasture-livestock systems (Nair Citation2012; Nair et al. Citation2022).
The many positive impacts of silvopastoral systems have been well established globally, as outlined in previous review articles (Jose Citation2009; Jose and Dollinger Citation2019; Pent Citation2020; Zhu et al. Citation2020; Plieninger et al. Citation2021; Smith et al. Citation2022) and summarised in . However, silvopastoral systems do not have universally positive effects (). For example, many silvopastoral systems show increased pasture growth compared to treeless pasture (e.g., Callaway et al. Citation1991; Peri Citation2005; Moreno Citation2008; Rivest et al. Citation2013), while other systems show decreased pasture production (e.g., Guevara-Escobar et al. Citation2007; Benavides et al. Citation2009; Rivest et al. Citation2013; Seddaiu et al. Citation2018). Silvopastoral systems must therefore be designed to maximise the benefits while minimising the negative effects of these systems to ensure they become beneficial land management solutions (Rhoades Citation1996; Casals et al. Citation2014; Jose et al. Citation2019).
Table 1. Review of the positive and negative impacts of silvopastoral systems globally for a range of outcomes.
Silvopastoral landscapes are complex systems involving numerous ecological components and mechanisms of human management (Mancera et al. Citation2018; Venturi et al. Citation2021). Even without considering trees, pastoral agriculture depends on complex interactions between livestock, forage plants, soils, microbes, and humans (Crawford et al. Citation2005; Robinson Citation2009; Bryant and Snow Citation2010; Dean et al. Citation2021). Integrating trees into the system greatly increases this complexity through adding new interactions and linkages, many of which can modify existing biophysical processes within the system () (van Mil et al. Citation2014; Mancera et al. Citation2018).
Figure 1. Ecological interactions between system components with increasing complexity for pasture, livestock and tree interactions in a pastoral system. The direction of the arrow indicates how the component the arrow comes from impacts the component the arrow goes towards. For instance, for number 7, the microclimate impacts livestock through changes to temperature, humidity, PAR and wind.
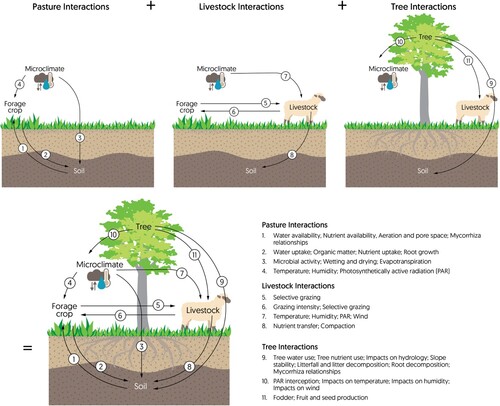
The impacts of trees in silvopastoral systems are difficult to predict, because these impacts emerge from the system as the net effect of numerous interdependent mechanisms and complicated feedback patterns (Salt Citation1979; O’Sullivan Citation2004). Understanding these emergent properties of silvopastoral systems requires a holistic approach, using a systems ecology framing to integrate the known ecological and social interactions in the system (Odum Citation1977; Salt Citation1979; Jørgensen Citation2012). Nevertheless, the majority of silvopastoral research does not take this holistic, systems approach (Plieninger et al. Citation2021). For example, in a recent review of silvopastoral research in the European dehesa and montado systems, Plieninger et al. (Citation2021, p. 9) concluded that the majority of past research ‘treated the dehesa system in an unintegrated way […] when in fact they are highly interconnected and interdependent’. Furthermore, applied guidance for silvopastoral managers frequently focuses on design to maximise individual benefits, rather than multifunctionality (Wilkinson Citation1999; Charlton et al. Citation2007; Mackay-Smith et al. Citation2021).
Land managers are faced with numerous options when designing silvopastoral systems. For example, determining (1) which tree species are suited to the soil and microclimate conditions of their land; (2) which tree species provide the key benefits to address a particular aim; (3) where in the landscape trees should be planted to gain the maximum benefit; (4) which type of livestock and at what stocking density is most appropriate; (5) and which pasture species are present or needed. Selecting the right combination of tree, livestock, pasture, and management options is challenging due to the many interdependencies and difficult to predict feedbacks between these separate decisions (Jose Citation2009; Jose et al. Citation2019; Plieninger et al. Citation2021).
In this study, we propose an integrative framework for silvopastoral system design that aims to be holistic in delivering multifunctional silvopastoral landscapes. This requires an understanding of key processes that govern the interactions between trees, pasture, and livestock, and a systems ecology lens that considers the multiple ecological and social interactions of these systems. To achieve this overall aim, the paper has three major objectives:
Review the key processes through which silvopastoral trees impact a range of outcomes using a systematic review of studies that have compared the impact of different tree species on silvopastoral outcomes at the same study site. By comparing different tree species at the same study site, this systematic review aims to identify how contrasting tree attributes impact silvopastoral outcomes, and evaluate the relative importance of different silvopastoral processes with respect to the outcome that they impact. The conclusions of this objective will provide advice to land managers as to which tree attributes may be important for impacting different outcomes in silvopastoral systems.
Use the New Zealand (NZ) silvopastoral context as a case study to characterise the system complexity and demonstrate how future silvopastoral systems can be designed to maximise their positive impacts for improved land management. Since silvopastoral systems are social-ecological systems (Plieninger and Huntsinger Citation2018), local perspectives should be incorporated into the design process so that new systems are both valued and adopted.
Drawing on the systematic review of silvopastoral tree processes, and the in-depth case study of NZ’s silvopastoral systems, we develop 5 key principles for successful future silvopastoral design.
Review of key agroecological processes for silvopastoral system design
A review of silvopastoral system agroecological processes was undertaken to determine the importance of (i) specific tree attributes and (ii) biophysical processes within the system that promote particular outcomes (). Studies were systematically selected that compare the impact of different tree species on a range of silvopastoral outcomes for a given site. By comparing two species at the same site, it could be ascertained how different tree attributes have differing impacts on the silvopasture environment, which can be used to provide recommendations as to which tree attributes are important for positively impacting the silvopastoral agro-ecological environment.
It was important to compare different tree species at the same site because varying climate, topography, aspect, livestock type, grazing management, fertility management, livestock behaviour and tree spatial design makes it very difficult to compare the impact of tree attributes on the agro-ecological outcomes from presence/absence studies of single tree species on different farms. We think the most appropriate way of comparing the impact of different tree attributes on agro-ecological outcomes is to review studies that compare different tree species, with differing tree attributes, at the same site with consistent underlying farm parameters where compounding farm and climatic variables have been controlled for.
Review methods
The literature search was undertaken on the Web of Science Core Collection database on 12th September 2022. The following keywords were searched in titles, keywords and abstracts: ‘silvopastoral’, ‘silvopasture’, ‘silvopastoralism’, ‘scattered tree* AND pasture’, ‘scattered tree* AND grass’, ‘wood pasture’, ‘wooded pasture’, ‘space-planted tree*’ and ‘tree pasture’. The search was limited to English articles and no date limit was used. This resulted in 1629 articles. Titles and abstracts were read and any studies that compared multiple tree species at the same site on any silvopastoral outcome were recorded in a database. The studies did not have to compare silvopasture treatments with treeless/open pasture, but had to compare the impact of different tree species at the same site. The impact of differing tree attributes on silvopasture outcomes could then compared, resulting in tree attribute recommendations for silvopasture design. This resulted in 165 articles, which were categorised based on the silvopastoral outcomes they measured.
The second screening step focused on the method section of these papers and removed articles that did not qualify for the following criteria:
Studies were required to be designed at the field/paddock scale since it is the scale at which the agroecological processes function ().
Studies that reported results from stands that had a density lower than 10 × 10 m or a row spacing of less than 10 m were removed because tree species with different densities can impact the agroecological environment differently (Rozados-Lorenzo et al. Citation2007). As we were interested in learning how different tree attributes impact different silvopasture processes, by only selecting systems that had a density higher than 10 × 10 m or row spacing higher than 10 m, we could limit density as a confounding factor in the review.
Studies that measured isolated trees were assumed to have a density greater than 10 × 10 m, and so were included in the study.
Studies that had a between-row spacing of greater than 10 m but a within-row spacing of less than 10 m were and compared the impact of trees on silvopasture outcomes between the rows were included in the study.
Studies that reported results from stands that were less than 5 years old were removed because these silvopastoral trees would have likely had minimal impact on the agroecological environment.
Any studies that only measured tree establishment (tree growth or survival) or fodder production were removed because this review was specifically interested in the agroecological processes within the system, and not outcomes that are related to tree management.
Studies that did not study silvopasture processes or outcomes in-situ were removed.
Full papers had to be available, or else they were removed.
Finally, cited references were screened in the original list of papers and included if they met the selection criteria. This yielded 42 articles (see Appendix 1 for the full list of studies).
Despite not technically being silvopastoral systems, several studies yielded in this search were of tree comparisons in savanna grasslands. These were retained in the review because of their relevancy to the research question. ‘Savanna’ was not included in the original search because this addition yielded 17,396 articles, too many to screen with the available resources.
A qualitative and quantitative approach were used to review the studies. The studies were first quantitatively grouped into studies of ‘processes’ and ‘outcomes’ (). The main findings of the articles were then discussed qualitatively because of the variety of outcomes and processes that were included in the review. A quantitative comparison of silvopastoral systems in different regions is challenging because of the multitude of factors which influence silvopasture outcomes, such as climate, land use history, pasture and livestock management, tree age, tree density and tree management.
Silvopastoral system process review
Processes and outcomes
Twenty-five papers measured soil (soil chemical properties, soil moisture or soil physical properties) outcomes in the reviewed studies, which was the most researched outcome, followed by pasture (24) and greenhouse gas emissions (21) (). The silvopasture processes that were researched the most were N2-fixing (14) and microclimate (10) ().
N2-fixing trees
Of the reviewed systems, 8 studied systems compared the impact of N2-fixing and non N2-fixing trees on soil N (Belsky et al. Citation1989, Citation1993a, Citation1993b; Rhoades et al. Citation1998; Menezes and Salcedo Citation1999; Menezes et al. Citation2002; Tripathi et al. Citation2005; Treydt et al. Citation2007; Casals et al. Citation2014; Dubeux et al. Citation2014; Cá et al. Citation2022). Three of these systems found increases of soil N or greater soil N mineralisation under N2-fixing trees compared to non N2-fixing tree systems (Rhoades et al. Citation1998; Menezes and Salcedo Citation1999; Menezes et al. Citation2002; Cá et al. Citation2022). However, 5 of these system comparisons did not report greater soil N under the N2-fixing trees (Belsky et al. Citation1989, Citation1993a, Citation1993b; Treydt et al. Citation2007; Casals et al. Citation2014; Dubeux et al. Citation2014). Some of the studies hypothesised other processes had overriding impacts on the agroecological environment over whether trees were N2-fixing or not, such as livestock activity (Belsky et al. Citation1989, Citation1993b; Dubeux et al. Citation2014), litter quantity (Casals et al. Citation2014) and impacts on soil moisture (Menezes et al. Citation2002).
Although not included in the systematic search, a previous meta-analysis has measured the impact of different functional groups (deciduous trees, Eucalyptus, N2-fixing trees and evergreen oaks) on pasture production (Rivest et al. Citation2013) and so provides an important comparison for this study. This study reported that N2-fixing trees were the only functional group to significantly increase pasture production compared to open pasture controls, deciduous trees and evergreen oaks had an overall neutral impact on pasture production, and Eucalyptus trees negatively impacting pasture production. Nevertheless, the N2-fixing results by Rivest et al. (Citation2013) are misleading. For example, three of the N2-fixing studies reviewed by Rivest et al. (Citation2013) compared N2-fixing trees and non N2-fixing trees. Treydt et al. (Citation2007) found no difference between the impact of N2-fixing trees and non N2-fixing trees on pasture production. Belsky et al. (Citation1989) measured a similar grass response between of N2-fixing trees and non N2-fixing trees. Moreover, despite Belsky et al. (Citation1993a) reporting significantly more grass under acacia (Acacia tortilis (Forssk.) Hayne) trees compared to under baobab (Adansonia digitata L.) trees, this was suggested to be because of greater elephant utilisation under this species and not because the acacia trees were N2-fixers.
There were two other English studies reviewed by Rivest et al. (Citation2013) in the N2-fixing group that were not a comparison of different species at the same site and so were not included in the systematic search. Ludwig et al. (Citation2001) found no difference between pasture production under acacia (Aacia tortilis) trees and open pasture, and although Durr and Rangel (Citation2002) did find 132% more grass growth under N2-Fixing Samanea saman (Jacq.) Merr. trees compared to open pasture, the authors could not confirm why the trees increased pasture production, and suggested nutrient transfer by cattle could have confounded this result. These examples indicate that it is not clear that the N2-fixing attribute was responsible for the positive impacts of the N2-fixing group on pasture production reported by Rivest et al. (Citation2013).
The results of this review and a look into the papers reviewed by Rivest et al. (Citation2013) suggest that N2-fixing should not be relied upon as a key tree attribute when selecting silvopastoral trees to improve soil condition, and that other factors can have an overriding influence on the agroecological environment compared to whether the tree fixes N or not.
Litterfall dynamics
Litterfall dynamics were frequently hypothesised to be an important driver of soil fertility outcomes in the reviewed studies (Casals et al. Citation2014; Martínez et al. Citation2014; Lima et al. Citation2020; Cá et al. Citation2022). Nevertheless, only two of the reviewed studies compared litterfall dynamics and silvopastoral outcomes (Menezes et al. Citation2002; Cá et al. Citation2022). Cá et al. (Citation2022) measured higher total organic carbon (TOC) sequestration in the top 10 cm of soil in a high tree diversity silvopastoral system with higher litterfall rates, however, Menezes et al. (Citation2002) provided evidence that impacts to soil moisture had much greater effects on the system than differing litterfall dynamics between species.
Although the authors did not directly measure litterfall, Casals et al. (Citation2014) hypothesised that litter quantity had much more impact on soil fertility and carbon than litter quality. This was because the impact of different tree species on these soil variables was not related to whether the trees were N2-fixers or not, nor to whether the trees were evergreen or deciduous. Moreover, measured livestock activity preferences were similar between tree species.
Litterfall dynamics have also been studied in the gliricidia (Gliricidia sepium (Jacq.) Kunth) and mimosa (Mimosa caesalpiniifolia Benth.) silvopastoral systems in Brazil, with litterfall rates being slightly greater in the mimosa system (10,790 kg ha−1 compared to 10,420 kg ha−1) (Apolinário et al. Citation2016a). However, N concentration of the leaves was greater in the gliricidia system (21.5 kg ha−1 compared to 18.8 kg ha−1). Moreover, the gliricidia system litterfall had a lower C/N ratio and lignin concentration, so it was suggested by the authors that the leaves of this species will decompose faster. This has been confirmed by other studies not included in the systematic review (Apolinário et al. Citation2016b; Herrera et al. Citation2020).
It was also reported that pasture production (Gomes da Silva et al. Citation2021b) and livestock productivity (Gomes da Silva et al. Citation2021a) were greater in the gliricidia system compared to the mimosa system, however, the authors suggested that this was because of significantly higher soil moisture in this treatment (mimosa: 16.2%; gliricidia: 17.2%) impacting pasture production, and not differences in litterfall decomposition rates (Gomes da Silva et al. Citation2021b).
Livestock activity
Despite not measuring livestock behaviour, two of the reviewed systems suggested that livestock activity was the likely reason for different soil and pasture outcomes in silvopastoral systems. Belsky et al. (Citation1993a) suggested elephant damage to be the main reason for the disparity between pasture production results under acacia and baobab trees at their higher rainfall site. Furthermore, Dubeux et al. (Citation2014) hypothesised that livestock activity had overriding influences on soil organic matter because they found 64% greater organic matter levels under two non N2-fixing compared to two N2-fixing trees at a 50% canopy site in the Itambé Experimental Station in Brazil.
Nevertheless, only two of the reviewed studies quantified livestock preferences (where livestock spend preferential time in the paddock) under different tree species (Wilson Citation2002; Casals et al. Citation2014) and have contrasting results. Casals et al. (Citation2014) reported no differences in livestock activity between species, whereas Wilson (Citation2002) reported nearly 20 times more livestock dung on transects around Eucalyptus melliodora A.Cunn. ex S.Schauer trees compared to E. nova-anglica H.Deane & Maiden trees. Clearly livestock preferences vary between different silvopastoral systems, and even between different tree species.
Microclimate
Seven of the reviewed studies measured processes in black walnut (Juglans nigra L.) silvopastures compared to honey locust (Gleditsia triacanthos L.) systems in Virginia (Buergler et al. Citation2005, Citation2006; DeBruyne et al. Citation2011; Fannon et al. Citation2017; Pent et al. Citation2020a; Pent and Fike Citation2019; Poudel et al. Citation2022). Black walnut silvopastures have tended to reduce annual pasture production compared to honey locust systems (Fannon et al. Citation2017; Pent and Fike Citation2019; Pent et al. Citation2020a; Poudel et al. Citation2022). Lack of photosynthetically active radiation (PAR) is suggested as the primary cause of pasture production differences, being attributed to the large canopies, leaf shape and canopy density of the black walnut system. This was quantified by Poudel et al. (Citation2022) who reported PAR and temperature were on average 49% and 1.1°C less in the black walnut silvopastures.
Reduced temperatures and increased PAR interception have also been related to improved livestock welfare in the black walnut system, in terms of animal thermal comfort indexes (Pezzopane et al. Citation2019) and hair cortisol levels (Poudel et al. Citation2022), and greater grazing time (Pent et al. Citation2020a). Moreover, despite the black walnut system having reduced pasture growth and a lower stock rate compared to the honey locust and open pasture systems (which had similar stock rates), these positive impacts to grazing time meant that the black walnut system had equivalent total lamb productivity to the honey locust and open pasture systems (Pent et al. Citation2020a, Citation2020b).
Microclimate has also been shown to enhance arthropod populations under specific trees, and different arthropod species are adapted to microclimate conditions under different trees (Tripathi et al. Citation2005).
Finally, three of the reviewed studies found evidence that deciduous trees had improved outcomes compared to evergreen trees (Frost and McDougald Citation1989; Ratliff et al. Citation1991; Rusch et al. Citation2014; Plevich et al. Citation2019). For instance, Rusch et al. (Citation2014) measured significantly lower pasture production from July to August under an evergreen tree compared to two other deciduous trees, and the evergreen tree also had the greatest crown density in the dry and rainy season. There was no evidence that soil moisture was a function of canopy density, and so the authors suggested that the differing impacts on pasture productivity were because of the evergreen crown intercepting more PAR. A similar conclusion was found by Plevich et al. (Citation2019) as pasture production was 23% and 70% higher under Quercus robur L. (deciduous) between April and October compared to Pinus elliottii Engelm. (evergreen) and Eucalyptus viminalis Labill. (evergreen) trees, respectively. However, this finding was not universal, and other studies found evergreen trees to have similar or improved outcomes compared to deciduous trees (Belsky et al. Citation1989, Citation1993a, Citation1993b; Casals et al. Citation2014; Castillo et al. Citation2020). This indicates that in these studies there are other factors that had a greater impact on pasture and soil outcomes compared to competition for PAR in winter by evergreen trees, such as the impact of evergreen/deciduous trees on livestock activity (livestock camping) and litterfall activity as described above.
Tree water competition
Studies in two systems attributed pasture and livestock production reductions to impacts on soil moisture (Menezes et al. Citation2002; Gomes da Silva et al. Citation2021a, Citation2021b). Menezes et al. (Citation2002) found lower soil moisture under isolated Prosopis juliflora (Sw.) DC. trees (14 years old) earlier in the season compared to a grass control, but no difference was found between the grass control and soil moisture under isolated Ziziphus joazeiro Mart. trees (~50 years old). This could have been because of greater competition for water under P. juliflora trees. Alternatively, it could have been because of evapotranspiration reductions under Z.joazeiro because Z. joazeiro and P. juliflora tree canopies intercepted 65–70% and 20–30% solar radiation, respectively, and soil temperature was statistically similar between open pasture soil and soil under P. juliflora, but was significantly less under Z. joazeiro trees.
Other tree groups
Native silvopastoral trees were shown to increase the diversity of dung beetle species and functional groups compared to exotic Pinus taeda silvopastoral systems (Gómez-Cifuentes et al. Citation2019, Citation2020).
Two studies in Californian silvopastures have found digger pine (Pinus sabiniana Douglas) trees to almost always negatively impact pasture production compared to pasture under blue oak (Quercus douglasii Hook. & Arn.) and interior live oak (Quercus wislizeni A.DC.) trees in rangelands with varying productivity levels (Frost and McDougald Citation1989; Ratliff et al. Citation1991).
Summary of findings and recommendations
Published evidence found the following attributes were beneficial for improving the silvopastoral environment:
Livestock activity can have overriding influences on the agroecological environment, and it is important that the tree species and the spatial design of the system facilitates livestock grazing under tree canopies, but is not conducive to overgrazing.
Higher litter quality increases litter decomposition rates, but slower decomposing litter may also be important in forming stable soil organic matter in the tropics.
Maximising litter quantity can improve soil outcomes – although this must be balanced against leaf smother (Eason Citation1991).
Minimising PAR interception by the tree canopy is important for maximising pasture production, however, this must be balanced with the potential of increased PAR interception resulting in improvements to livestock performance.
Deciduous trees can have improved pasture production outcomes, however, this effect is not universal.
In the reviewed studies, there was a distinctive lack of studies measuring processes () within silvopasture, with most studies focused on outcomes (). Moreover, there were no studies that measured a full range of processes in a silvopastoral system or related these to silvopasture outcomes. Moreover, none of the reviewed studies that directly compared tree water use between tree species. It will be important for future research to cover a broader range of processes and compare trees with different functional traits, to provide more compelling evidence as to which tree functional traits are important for improving given combinations of outcomes.
An important finding is the evidence that livestock can have overriding influences on the silvopastoral environment, and the review provides evidence that livestock activity is an essential consideration when comparing outcomes between systems. More work is required to measure livestock preferences for different tree species, in what situations livestock preferences exist, and how the impact of livestock activity as a process compares to direct tree processes like litter decomposition or competition for water.
N2-fixation is generally seen as an important tree attribute in silvopastoral tree selection (Nair Citation1993; Rivest et al. Citation2013). However, this review indicates that N2-fixing trees do not necessarily result in improved soil outcomes and other processes, such as livestock activity and litter quantity, can have overriding impacts on the agroecological environment.
There were two other potentially important processes that received no research in past comparison studies: allelopathy, which is when one species produces biochemical that impacts the survival or growth of another organism (Rice Citation1974), and leaf smother (Eason Citation1991). Both these processes can negatively impact pasture growth or seed germination (Eason Citation1991; Ahmed et al. Citation2008; Halvorson et al. Citation2017), so more research is required to quantify the importance of these processes with the processes covered in this review ().
Finally, only three studies provided evidence that deciduous trees result in improved outcomes compared to evergreen trees. Moreover, in their meta-analysis, Rivest et al. (Citation2013) reported that both deciduous trees (the majority of which were oaks) and evergreen oaks had an overall neutral impact on pasture production. As with N2-fixing trees, this suggests that there are likely other attributes that can have more influence on the agroecological environment, which may include the size of the trees, the crown architecture or allopathy effects. Furthermore, there are likely other important factors impacting the system besides tree attributes, such as livestock management and behaviour, or how the spatial design of the trees as well as tree architecture impacts livestock activity. It is important that future research spans a greater variety of tree functional traits, and to research how these traits interact with management factors and livestock activity.
Silvopasture in New Zealand: towards integrative design
To provide an example case study of how more integrative design of silvopasture could be developed, we discuss the design of silvopastoral systems in NZ. Silvopastoralism has already been adopted widely in NZ, with poplar (Populus spp.) and willow (Salix spp.) principally being planted in the context of soil erosion (Kemp et al. Citation2018; Spiekermann et al. Citation2021; McIvor et al. Citation2023). However, there remains much potentials for other tree species to provide a range of additional benefits to NZ farms (Mackay-Smith et al. Citation2021). New Zealand therefore represents an excellent case study to showcase the important of taking a holistic and integrative perspective to silvopasture design.
The New Zealand silvopasture context
Following contact with European traders and settlers in the 1800s, extensive deforestation for pastoral farming resulted in high erosion and sedimentation rates (Goff Citation1997; Glade Citation2003; Green Citation2013; Phillips et al. Citation2018). Erosion processes in NZ remain very active compared to elsewhere (Jansson Citation1988), due to a predisposed natural environment with steep slopes, weak sedimentary rocks, and a climate featuring high annual rainfall and relatively frequent high magnitude rainfall events (Hicks et al. Citation2011, Citation1996; Basher Citation2013). High sediment yields can have detrimental outcomes on riverine and estuarine habitats by reducing the diversity, types, and abundance of fauna (Fuller and Death Citation2018).
Scattered or widely spaced silvopastoral trees have been used as a soil conservation and sediment mitigation tool on erosion-prone sloped pastures in NZ since the 1960s (Wilkinson Citation1999; Kemp et al. Citation2018; Spiekermann et al. Citation2021). The primary silvopastoral species that have been planted in NZ are poplar (Populus spp.) and willow (Salix spp.) (Wilkinson Citation1999; Kemp et al. Citation2018; Spiekermann et al. Citation2021). These trees have been chosen and actively promoted because they can be easily planted as sharpened, coppiced poles in the presence of grazing livestock (Wilkinson Citation1999; Phillips et al. Citation2014), and because they perform well in terms of soil erosion (Wilkinson Citation1999; Douglas et al. Citation2013; McIvor et al. Citation2015; Mackay-Smith et al. Citation2021; Spiekermann et al. Citation2021).
Nevertheless, there are other tree attributes that will be important for different silvopastoral functions on NZ farms (Mackay-Smith et al. Citation2021), and silvopastoral trees will likely provide additional value to less erosion-prone pastures in NZ, either in more rolling pastureland or flatter more productive pastures. In short, these systems are not designed considering the complexity of their functions, interactions or potentials, which limits the value silvopastoral systems add to NZ farms.
Stakeholder perspectives on current and future NZ silvopastoral systems
Methods
A broad range of individuals including farmers, landowners, land management advisors, policy advisors, and advocates were openly invited to a workshop to explore current silvopastoral systems used in NZ, and potentials for the future (). The range in occupations of participants enabled perspectives to be collected, spanning from those active in tree planting to those that advised or advocated the use of silvopastoral systems. By having focus groups dedicated to specific occupations, opinions could be compared between groups, with the intention to identify gaps between those using these systems and those advocating or providing advice.
Table 2. Occupation and participant numbers for the workshop participants.
Focus groups were held on 11th October 2022 on a farm in the Wairarapa region of NZ. Individuals were grouped in focus groups based on occupation and there were 34 participants in total ().
There were two focus group sessions during the day. The first session focused on silvopastoral systems that are currently used in NZ. The questions for the landowner group explored (i) whether they planted silvopastoral trees on their farms, (ii) the reasons they did or did not plant, and (iii) challenges related to the use of these systems. The land management advisors (LMAs), policy advisors and advocacy groups were asked (i) whether they advise farmers to plant silvopastoral trees, (ii) the reasons they do or do not advise farmers to plant, and (iii) challenges related to advising the use of silvopastoral trees.
The second session explored the potential of silvopastoral systems in the future for positively impacting NZ farms. For the landowners, the following questions were asked: (1) What potential benefits of these systems are important to you? (2) What potential barriers are there to planting in the future. For the LMAs, policy advisors and advocacy groups, the following questions were asked: (1) Where do you see the potential for silvopastoral systems to respond to future challengers? (2) Do you think there will be any barriers to adoption of future silvopastoral systems.
Responses were recorded and compiled on flipcharts. Participants were fully anonymised and not recorded. Full consent for this work to be published was provided by all participants at the beginning of the workshop.
Workshop findings – current silvopastoral systems that are used, the reasons for their use and challenges of their use
Only one landowner participant did not plant or manage silvopastoral trees on their farm. Poplar (Populus spp.), followed by willow (Salix spp.) and eucalyptus (Eucalyptus spp.) trees, were the most common silvopastoral trees planted. Other trees that were planted included tagasaste (Cytisus proliferus L.f.), cork oak (Q. suber L.), acacia (Acacia spp.), chestnut (Castanea spp.), Tasmanian blackwood (A. melanoxylon R.Br.), maple (Acer spp.), radiata pine, ginkgo trees (Ginkgo biloba L.) and mānuka (Leptospermum scoparium J.R.Forst. & G.Forst.). Native remnant tree species were also managed as silvopastoral trees, such as totara (Podocarpus totara G.Benn. ex D.Don), kānuka (Kunzea spp.) and southern beech (Nothofagus spp.).
The landowner participants had substantial knowledge of the potential benefits of silvopastoral systems, with soil erosion, shade, shelter and carbon credits being the most commonly stated benefits (). The most common limitations associated with these silvopastoral systems included protection against pests, tree survival, and cost of planting, protection and maintenance ().
Many of the benefits and limitations of silvopastoral systems stated by the non-landowner groups () were similar to those stated by landowners (). Additional benefits stated by the policy group included iwi partnership/Te Ao Māori benefits, tourism and contributing to NZ’s clean green image, and ecosystem health. The central challenges for LMAs when advising landowners included difficulties with regulation (competing incentives and regulations), managing farmer expectations and farmer resistance, timescale issues (in terms of a time lag until effectiveness and total costs during a tree’s life), and the cost of providing advice. The advocacy group stated they did not provide much advice on silvopastoral systems as they were not often consulted. A reason suggested for this was that the focus in NZ has been more on pastoralism, as opposed to silvopastoralism.
Table 3. Limitations given by non-landowner groups for the potential of silvopastoral systems to be realised in the future.
Future silvopastoral systems and their potential
The landowners already had considerable knowledge of the future potential of silvopastoral systems, indicated by the wide variety of benefits this group already stated in . The potential barriers to adoption were also very similar to the limitations reported in . The use of silvopastoral systems by landowners, and knowledge of them, was greater than what was anticipated when planning the workshop.
In addition to barriers presented in , landowner participants raised more intangible barriers to the future adoption of silvopastoral systems in this section, such as narratives and perceptions. For example, one group discussed that the current paradigm is focused on fixing what is broken rather than creating future possibilities. Other comments focused on the importance of managing the tension between the economic and environmental impacts of these systems, and past landowner attitudes that have typically been negative towards trees.
Another important barrier that was discussed was the regulatory environment for NZ’s Emissions Trading Scheme (ETS). There was frustration that credits could not be collected from individual trees that had been planted if they were not in a group of trees greater than 1 ha in size that had a canopy cover greater than 30% (Ministry for Primary Industries Citation2020). There was also frustration that the regulatory environment was not recognising the environmental work farmers were already doing on their farms.
One of the most important barriers brought up by both the landowners and non-landowner groups was the cost of these systems. A lack and cost of labour was emphasised as an important barrier for these systems, as well as the cost of protection. An important barrier to future adoption discussed in the landowner groups but not in the non-landowner groups (policy group, LMA, advocacy) was the impact of pests on trees ().
Some landowners and the LMA groups expanded on future steps that may be required to increase the use of silvopastoral systems in NZ. There was an emphasis on scientific research, specifically research that informs tree selection and improves tree cultivar options. Funding was also deemed important to help with planting, pest control and tree management. Improving pest control methods was also suggested, in addition to various answers related to capacity building, both in terms of support for farmers, and capacity building that improves relationships between researchers and farmers.
Limitations
It is likely that the participants who attended the workshop had more knowledge of silvopastoral systems than if participants were randomly sampled in the region because those that attended likely had an interest in trees. Therefore, the number of landowners who used silvopastoralism was most likely an overestimation. Moreover, it is expected that the typical landowner in the region would not have planted the diverse set of trees mentioned, but instead planted just poplars and willows, or no silvopastoral trees. Nevertheless, because the landowners likely had more experience using silvopastoral trees compared to a randomly sampled landowner in the region, these experiences with trees were important for informing on the challenges and potentials of these systems in NZ.
Integrative silvopasture design in New Zealand
Outcomes
While silvopastoral systems in NZ have primarily been implemented for erosion mitigation, there is potential for future design to target a broader range of outcomes. presents a broad range of silvopastoral benefits that have been identified globally. Here, we summarise previous silvopasture research into some outcomes that may be particularly relevant for NZ.
The impact of silvopastoral systems on soil erosion and pasture production has been particularly well studied in NZ. Most empirical studies aimed at quantifying the effectiveness of biological erosion control under silvopastoral systems have been carried out in NZ (Douglas et al. Citation2013). A paired comparison study following a storm event in April 2011 in the Hawke’s Bay region, selected 86 sites with trees (defined as stands of 1–14 trees) and 25 control sites with pasture only (McIvor et al. Citation2015). Landslide erosion was 78% less on sites with trees compared to the pasture control sites. A similar method was used across 65 sites in the Manawatu and Wairarapa following storms in 2004 and 2006, respectively (Douglas et al. Citation2013). Trees reduced landslide occurrence by 95% compared to paired pasture control sites (0.4% vs. 7.9% scar area, respectively), and scars occurred on fewer sites with trees than pasture (10 vs. 45).
Since slope instability is caused by multiple interacting factors, Spiekermann et al. (Citation2022a) developed a data-driven approach to improve targeting of erosion control from slope to landscape scales to improve upon the empirical observations of previous research (e.g. Douglas et al. Citation2013). By modelling future shallow landslide occurrence based on the actual tree cover versus a treeless (pasture only) baseline scenario, the performance of silvopastoralism in terms of reducing landslide erosion was assessed for two farms. The difference in outcomes across the two selected farms (17% versus 43% reductions) demonstrated the importance of targeting tree planting to slopes that are more likely to be sites of future landsliding. Furthermore, Spiekermann et al. (Citation2022b) undertook similar modelling to quantify the effect of silvopastoralism on landslide-derived sediment yields, contrasting the cost-effectiveness of targeted and non-targeted approaches to tree planting, which will be explored below.
The majority of research measuring the impact of silvopastures of pasture production has studied poplar (Populus spp.) and radiata pine (Pinus radiata D.Don) trees, and without exception, every study has found reduced pasture production under low-density systems using these species (< 200 trees ha−1), with reductions ranging from 12% to 94% (Percival and Knowles Citation1988; Hawke Citation1991; Gilchrist et al. Citation1993; Cossens and Cossens Citation1995; Devkota et al. Citation1997; Cossens and Hawke Citation2000; Douglas et al. Citation2001; Wall Citation2006; Guevara-Escobar et al. Citation2007; Benavides et al. Citation2009). However, a recent study reported that individually spaced, mature kānuka (Kunzea robusta de Lange & Toelken) trees increased pasture production by 108% compared to open pasture at two sites, which was associated with significantly greater Olsen-P, organic matter, Total-N and porosity under the trees. This increase was hypothesised to be because the trees facilitated livestock activity under the canopies, in addition to the potential addition of organic matter by the trees to the soil (Mackay-Smith et al. Citation2022b). Mackay-Smith et al. (Citation2022b) is the only published study to report increased pasture production under mature silvopastoral trees compared to open pasture in NZ. Further research is required to explain the disparity between the increased pasture production under kānuka trees reported by Mackay-Smith et al. (Citation2022b) and the past poplar research (Benavides et al. Citation2009; Kemp et al. Citation2018).
There has only been one study that has compared the impact of fully grown isolated silvopastoral trees on surface runoff and associated nutrient and sediment loss in NZ (Mackay-Smith et al. Citation2022a). The authors reported 54.0 mm of annual surface runoff under kānuka (K. robusta) trees and 7.5 mm of annual surface runoff in open pasture. Sediment and nutrient losses were 10–100 times greater under the kānuka trees, however, sediment and nitrogen (N) losses were small compared to past NZ studies, although phosphorus (P) losses were relatively high compared to past studies (McDowell and Wilcock Citation2008; Mackay-Smith et al. Citation2022a). There was evidence of selective grazing of pasture under the kānuka trees in this study, which likely increased surface runoff and associated sediment and nutrient losses in this treatment (Mackay-Smith et al. Citation2022a).
A few studies have been conducted in NZ on greenhouse gas (GHG) emissions or soil carbon sequestration in silvopastoral systems. Several NZ studies measured elevated soil carbon concentrations under radiata pine (Saggar et al. Citation2001; Chang et al. Citation2002) and kānuka silvopastoral systems (Mackay-Smith et al. Citation2022b) compared to bare soil or open pasture. These studies, however, were not designed to compare the effect of long-term silvopasture to open pasture on soil carbon stocks at depth and dedicated research focussed on measuring carbon sequestration under silvopastoral systems is needed. Douglas et al. (Citation2020) compared soil carbon under 14-year-old poplars, 16-year-old alders and open pasture. Soil C mass was reported to be 11–18% lower in the pasture-poplar system compared to open pasture, and 6% greater in the pasture-alder compared to open pasture. Because the poplar and alder trees in this study were planted on separate sites, they were not included in the systematic review as part of this paper.
Moreover, animal welfare and productivity has rarely been studied in NZ silvopastoral systems, with previous research focusing on artificial shade (Kendall et al. Citation2006; Fisher et al. Citation2008) and widely spaced willow (Salix spp.) trees (Betteridge et al. Citation2012). The benefits of shelter (tree lines, sheds, rocky out crops etc.) to newborn lamb survival in NZ are clearly established in Pollard (Citation2006), yet none of these studies examine the effect of silvopastoral systems on cold stress in animals.
Earthworm populations have been studied under poplar trees, which were found to reduce or maintain earthworm populations compared to open pasture (Guevara-Escobar et al. Citation2002), however, no published studies comparing bird or insect biodiversity in silvopastoral systems in NZ, despite there being potential for these systems to harbour greater abundance compared to treeless pastures (Torralba et al. Citation2016; Gómez-Cifuentes et al. Citation2020, Citation2019).
Historically, planting of poplars and willows in NZ was primarily for the purpose of erosion control, but the co-benefit of forage provision (leaves, twigs, bark) has long been appreciated and researched (Kemp et al. Citation2001). More recent research has highlighted the potential of a broader range of species, including 9 exotic and 10 indigenous tree species, although almost no research has been conducted on indigenous species (Tozer et al. Citation2021). More research is required on other income opportunities in these systems, especially native high-value timber species and the viability of trees to produce additional tree by-products.
There has been much research and development on the current pole planting method that is used to plant poplar and willow trees in NZ (Wilkinson Citation1999; Charlton et al. Citation2007; Phillips et al. Citation2014). There has been limited research into the development of protection strategies for planting alternative tree seedlings in the presence of livestock.
Finally, there has been little formalised research on the cultural values of silvopastoral systems in New Zealand. Moreover, given that NZ recognises two underpinning worldviews, Te Ao Pākehā (the British Monarchy) and Te Ao Māori, the indigenous Māori worldview (Harcourt et al. Citation2022), it is important to create a space for Māori to explore how silvopastoralism may fit with their own values and aspirations. We advocate for a kaupapa Māori approach (research conducted by, with and for Māori) so that Māori communities can assess the potential benefits of silvopastoralism.
Despite great potential for silvopasture to positively impact a range of outcomes if designed correctly, the paucity of research into the impacts of silvopastoral systems on cultural values, surface runoff and sediment and nutrient losses, biodiversity conservation, GHG emissions, livestock welfare and diversification options highlights many opportunities for silvopastures to provide a range of additional benefits to NZ farms. Moreover, if research is expanded to a greater breadth of outcomes, this will enable different NZ silvopastoral trees to be more fairly compared and assessed over their full range of known functions (Mackay-Smith et al. Citation2021), and likely show how a more diverse range of trees species could be used in silvopastoral systems within NZ.
Tree attributes and processes
As was discussed in Section Integrative silvopasture design in New Zealand: Outcomes, a function that has not been utilised in New Zealand is the potential for silvopastoral trees to increase pasture production compared to open pasture. As was explored in the processes review, there are likely more nuanced tree functional traits than whether trees are evergreen or deciduous, or are N2-fixers or not, that are important for improved pasture production in silvopastoral systems. This becomes clear when comparing various studies of a single tree system comparison between tree pasture and open pasture positions.
For example, Durr and Rangel (Citation2002) reported 132% more pasture production under N2-fixing deciduous Samanea saman trees in north-east Queensland, Australia. Mackay-Smith et al. (Citation2022b) also found over 100% more pasture production under kānuka trees in NZ compared to open pasture, although kānuka trees are evergreen and non N2-fixing. Evidently, pasture production can be greater under deciduous or evergreen trees, and N2-fixing or non N2-fixing trees.
Pasture production can even vary under the same tree species in similar climates and systems. Gea-Izquierdo et al. (Citation2009) measured ∼3500 kg dry matter (DM) ha−1 under the canopy of holm oak (Quercus ilex L.) trees, and ∼2000kg DM ha−1 outside the canopy in a Spanish site with rainfall of 573 mm. On the contrary, Cubera et al. (Citation2009) reported significantly less pasture production under the canopy of holm oak trees in unfertilised (open areas: 2540 kg DM ha−1; under tree canopy areas: 1390 kg DM ha−1) and fertilised (open areas: 5230 kg DM ha−1; under tree canopy areas: 2080 kg DM ha−1) treatments in a site with 665 mm of rainfall. Clearly, there are likely other tree attributes that are important for ensuring silvopastoral trees maximise pasture production on farms.
A factor that was not measured in these studies was livestock activity (Durr and Rangel Citation2002; Cubera et al. Citation2009; Gea-Izquierdo et al. Citation2009; Mackay-Smith et al. Citation2022b). Livestock activity was an important process in the studies reviewed in the process review, and both Mackay-Smith et al. (Citation2022b) and Durr and Rangel (Citation2002) suggest that livestock spending preferential time under the measured silvopastoral trees could be having important impacts in the measured positions. The impact of livestock activity could be an integral piece in the puzzle that explains much of the variation seen within broader-scale functional groups generally considered in pasture silvopastoral comparisons (Rivest et al. Citation2013).
It is unknown whether the livestock camping effects measured by Mackay-Smith et al. (Citation2022b) and Durr and Rangel (Citation2002) provided a net improvement to pasture production at the paddock scale. For example, the livestock effects could have been nutrient re-distribution from open areas of the paddock to tree-pasture areas of the paddock. Alternatively, as the trees studied by Mackay-Smith et al. (Citation2022b) were on 20–25 degree slopes, the re-distribution could have been from flatter areas of the paddock where nutrients were likely in excess, to the tree-pasture areas, resulting in a net increase in pasture production at the paddock scale. Further work is required to understand the relationship between pasture production, tree spatial design and livestock activity at the paddock scale in silvopastoral systems.
Another important factor could be the size of silvopastoral trees. Rivest et al. (Citation2013) reported that eucalyptus trees were the only functional group to significantly reduce pasture production compared to open pasture, and all past work on poplar trees greater than 15 years old in NZ has reported reduced pasture production under their canopies compared to open pasture (Benavides et al. Citation2009). Both these genera (eucalyptus and poplar) are typically large trees and greater than 30 m when fully mature. This contrasts with the principle silvopastoral trees used in Southern Europe (holm oak and cork oak) and Patagonia, Argentina (Nothofagus antarctica (G.Forst.) Oerst.), that are all between 4 and 15 m (Pulido et al. Citation2001; Sánchez-González et al. Citation2005; Gouveia and Freitas Citation2008; Peri et al. Citation2016), and past studies in all these systems have reported increased pasture production under these silvopastoral trees in certain situations (Peri Citation2005; Moreno Citation2008; Gea-Izquierdo et al. Citation2009).
These examples show that there are still many unknowns in terms of tree attribute-pasture production relationships, and more research is required so silvopastoral systems can be designed to maximise the productive benefits they add to farms. Furthermore, it is likely that the impact of tree attributes on silvopastoral outcomes will vary depending on regions and farm types, so this must be considered in future research.
Local perspectives
The perspectives presented highlight that silvopastoral systems are already used in NZ, albeit with many limitations. This indicates that if new systems can be designed which overcome some of the limitations presented in , they should be adopted. Nevertheless, a key limitation raised in the workshop was the practical realities of planting and managing silvopastoral trees in general. Tree survival is integral, in addition to the cost of establishing and managing the system, and protection against livestock and pest damage (). This is likely one reason why poplar and willow have been so successful in NZ, because they can be established as sharpened, coppiced poles in the presence of livestock. If other trees are to be used in NZ, planting methods and survival rates must rival that of poplar and willow pole planting.
If more research can show whether trees with different attributes to poplar consistently increase pasture production compared to open pasture (Mackay-Smith et al. Citation2022b, Citation2021), then this could potentially offset the costs of planting and managing silvopastoral trees. Moreover, it will also be important to select silvopastoral trees that require minimal management. One key constraint of poplar plantings is current land management advice to fell and replant the trees after 40 or 50 years (Charlton et al. Citation2007). More recent varieties (e.g. Populus maximowiczii × nigra) are expected to grow for longer than this, which would reduce the overall management required for each of the trees. Additionally, if other silvopastoral tree species can be found that grow for over 200 years, as it common in the dehesa system in Spain (Plieninger et al. Citation2021), this may substantially increase the attractiveness of these systems.
Targeting
illustrates the importance of carefully planned silvopastoral design in the context of soil erosion, based on the integration of landslide susceptibility and connectivity modelling (Spiekermann et al. Citation2022a, Citation2022b). A large number of widely spaced trees are shown in the aerial photo, which are mainly poplar and willow trees, as well as a labelled eucalyptus grove (shown in the photo). These trees were planted on slopes to reduce landslide erosion following the severe rainfall event of 1977. The two maps identify areas where future landslide-derived sediment is likely to be sourced from, with approximately two-thirds estimated to be sourced from the ‘high’ zone. The maps show the difference with and without trees present. The Eucalyptus grove has been planted primarily in the red zone, which is where landslides would be expected to occur in future – and deliver sediment to adjacent streams – if no trees were present. The second map shows the same landslide susceptibility classification, now including the influence of the trees depicted in the orthophoto. The slope with the eucalyptus grove has mostly changed to green, which indicates it has successfully been stabilised. It provides a contrast to the poplars and willows to the west, which are primarily located on lower slopes or valley bottom where landslides are unlikely to occur with or without trees. Therefore, there is little change to the extent of the red zone in this part of the map and demonstrates the difference between a targeted and non-targeted approach to silvopastoral design. Soil conservation trees not fulfilling their primary function have additional implications due to the possible reduction in pastoral production beneath their canopy, ultimately resulting in an undesirable net negative outcome.
Figure 4. Potential landslide-derived sediment delivery to streams based on modelling landslide susceptibility (Spiekermann et al. Citation2022a) and landslide connectivity (Spiekermann et al. Citation2022b) modelling for a small pastoral area in the Wairarapa, NZ. The comparison of a treeless baseline scenario with the actual tree cover (shown in the orthophoto from 2013) demonstrates that the eucalyptus grove (photo insert) has led to a much greater reduction in future sediment delivery compared with the poplars and willows to the west, which were largely planted in areas where landslides are unlikely to occur with or without trees present.
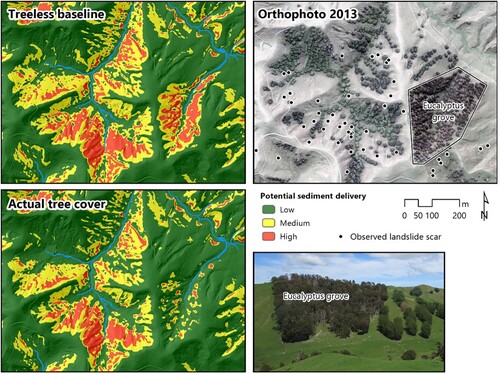
Decision-support tools can be used to develop well designed silvopastoral systems. For example, Spiekermann et al. (Citation2022b) undertook a cost-effectiveness assessment by quantifying the reduction in sediment delivery across fifty farms in the Wairarapa region of NZ, based on contrasting planting options: (1) targeting critical source areas of sediment and (2) a non-targeted approach to tree planting. Results of the cost-effectiveness assessment suggest there is a ten-fold difference between a targeted and non-targeted approach in terms of the ratio of investment to reduction in sediment delivery achieved. In monetary terms, the average cost for a 500 ha farm to achieve a 10% reduction in landslide-derived sediment was estimated at $14,100 for targeted mitigation and $131,000 for non-targeted tree planting.
An additional key finding in integrating landslide susceptibility and connectivity modelling is that, with a shift in focus from soil conservation to freshwater management, the area requiring tree planting halved since many landslide deposits would not reach streams. In total, only 6.5% (2370 ha) of the 36,000 ha of farmland assessed were deemed to be the potential source of approximately two-thirds of landslide-derived sediment across the 50 farms. Due to existing tree cover, this area requiring tree planting has already been reduced to 4.7% (1720 ha). This example illustrates how targeting trees in the pursuit of a desired outcome can be achieved with appropriate decision-support tools.
Key principles for an integrative approach to designing silvopastoral systems
Silvopastoral landscapes are complex systems that require holistic management across all dimensions
In reviewing the processes silvopastoral system literature globally, it is evident that there is a lack of studies assessing the biophysical processes holistically. When discussing why silvopastoral trees may facilitate or compete with pasture production, many studies focus on just tree effects and miss the impacts livestock activity has in the system (Frost and McDougald Citation1989; Gea-Izquierdo et al. Citation2009; Rivest et al. Citation2013; Seddaiu et al. Citation2018). Nevertheless, considering all known ecological interactions is integral to more fully understand the processes within the system, and how different tree attributes impact these processes and the impact this has on different outcomes.
Moreover, there is a bias in terms of which outcomes have been studied in silvopasture comparison studies (), and in past NZ silvopasture research. Researching and considering the impact of silvopastures on a more diverse range of outcomes is integral for these systems to fulfil their potential, and viewing them holistically should facilitate this process.
The views, values and experiences of local people are deeply connected to silvopastoral system design and must be incorporated
Considering local and indigenous values, experiences and perspectives is essential if ecologically sound silvopastures are to be adopted. Every region will have slightly different challenges and desires. Understanding these and considering them when selecting silvopastoral trees should be the cornerstone to integrative silvopasture design. The workshop findings also demonstrate the importance of practical constraints when designing silvopastoral systems, and these must be considered in any proposed system. These can only be understood by speaking to practitioners.
Spatial heterogeneity in environmental and social conditions requires locally specific decisions
The summary of possible silvopastoral outcomes () identifies a multitude of possible objectives when designing silvopastoral systems. However, it is likely that a general hierarchy in the prioritisation of outcomes exists and that the order of priorities will vary spatially as a function of limiting environmental factors (e.g. climate and soils), risks (e.g. erosion, heat stress in livestock), and individual preferences (e.g. cultural). Since in general silvopastoral systems are foremost aimed at animal production, economic sustainability is a fundamental priority. Thus, the central desired outcome in a silvopastoral system is generally animal production. However, for a given farm, the priorities in outcomes may vary spatially, giving rise to silvopastoral designs bespoke to the differing limiting factors, risks, and preferences.
Determining the central function prior to planting decisions, and selecting trees that have attributes that are beneficial to this function, are an integral part of designing value-maximising silvopastoral systems. shows a schematic for a pastoral landscape with multiple land-use classifications. This schematic highlights how different tree planting will be appropriate for different areas on the farm, and will vary spatially depending on the function of the trees. For example, to conserve soils and reduce sediment delivery to adjacent streams, steep slopes prone to landslide erosion will require different tree species and planting densities compared to rolling pastures. While there is substantial evidence that poplar, the most commonly planted silvopastoral tree in NZ, negatively impact pasture production (Benavides et al. Citation2009; Mackay-Smith et al. Citation2021), the main intended function of poplar tree planting is soil conservation. While a reduction in pastoral production may be acceptable in low producing areas of the farm, to achieve a balance between production and environmental outcomes in medium producing pastoral land will require a change in focus with respect to tree attributes and spatial layout of trees ().
Figure 5. Schematic diagram illustrates how silvopastoral design could vary spatially within a farm and how the design relates to desired outcomes. A typical farm will be more spatially diverse than this diagram indicates, and this figure is purely a representation of how there is a relationship between silvopasture outcome, topography and tree attribute/vegetation type on the farm.
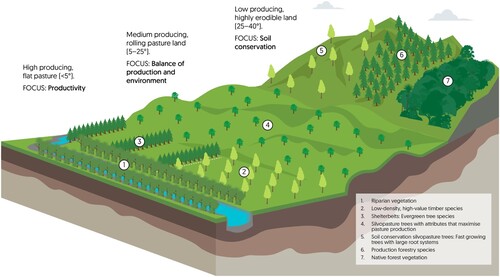
Understanding of ecological processes must underpin all management decision
There are many different ecological considerations in silvopastoral system design (Jose et al. Citation2019). When attempting to positively impact a specific outcome, it is important to understand how different tree attributes impact the agroecological processes within silvopastoral systems (). Silvopastoral can then be optimised based on tree attributes so they increase facilitation whilst minimising competition (Jose et al. Citation2019).
The processes reviewed () did not focus on one specific outcome (). This is because if silvopastoral trees can improve the agroecological environment, this will positively impact most outcomes. For instance, independent of the process, if silvopastoral trees can improve soil fertility, soil organic matter and soil physical capacity, this will likely have positive impacts on pasture production (Durr and Rangel Citation2002; Mackay-Smith et al. Citation2022b), discharge rates and sediment losses (Zhu et al. Citation2020). Moreover, the addition of a tree will sequester carbon (Guevara-Escobar et al. Citation2002), improve slope stability (Spiekermann et al. Citation2022a, Citation2021), have important livestock health benefits (Blackshaw and Blackshaw Citation1994), and could benefit biodiversity (Torralba et al. Citation2016; Gómez-Cifuentes et al. Citation2020; Mupepele et al. Citation2021). Taking a processes understanding of the system should help in determining which tree attributes are important for improving the agroecological environment, resulting in integrative improvements to silvopastoral outcomes and the pastoral environment.
The complexity and spatial heterogeneity present in silvopastoral systems requires high-resolution data and tools
Section Targeting illustrated the use of decision support tools in the form of spatial predictions of future landslide-derived sediment delivery to determine where to focus silvopastoral tree planting to mitigate erosion and sediment loss. However, since priorities of outcomes will differ spatially – also due to individual preferences, spatial tools that can cater for these preferences are required to aid land managers in making informed decisions. There is significant further field-based research required before outcomes such as dry matter production can be modelled at the scale of individual trees while accounting for agroecological interactions in the silvopastoral system (). Notwithstanding current knowledge gaps, decision support tools (e.g. multi-criteria decision analysis) need to be designed to better integrate technical information and stakeholder values.
Moreover, additional information would result in more informed and possible different decision making (Spiekermann et al. Citation2015). Decision-support tools can help minimise limitations related to subjectivity in decision-making and help overcome the complexity involved in considering trade-offs between environmental, economic, political and cultural impacts (Huang et al. Citation2011).
Conclusion
This paper took an integrative approach to silvopastoralism design to account for the ecological and social complexity of these systems. Ecological processes within silvopastoral systems were systematically reviewed. This was followed by an integrative design process using a NZ case study, which generated 5 key principles that should be considered in future silvopastoral system designs globally.
The silvopastoral system process review demonstrated that livestock activity can have substantially more impact than more typically researched processes, such as microclimate influences and N2 fixation.
More research is required on under-researched areas such as livestock activity, leaf smother and allelopathy effects, and there is a need for studies that measure a greater range of processes in a silvopastoral system which are then related to silvopasture outcomes. Furthermore, it is important that future research spans a greater variety of tree functional traits and silvopasture processes, such as tree size, tree architecture, livestock preferences, tree water use, leaf smother and allelopathy effects.
The NZ case study explored integrative design in four themes: outcomes, processes, local perspectives, and targeting. A NZ case study workshop generated information of the practical constraints of using silvopastoral systems, such as the impact of pests, survival challenges and costs, and any evaluation of new systems must account for these limitations. Furthermore, silvopastoral systems have not fulfilled their potential over a broad range of outcomes in NZ, and more research is required to learn which key functional traits are required for maximising pasture production in these systems. Finally, an example was shown how targeting soil conservation trees is fundamental for improving the cost-effectiveness of these systems and the positive impacts of them to farms.
Following the NZ case study, the authors propose 5 key principles that should be considered in future silvopasture design:
Silvopastoral systems are complex and require holistic management.
The views, values and experiences of local people are deeply connected to silvopastoral system design.
Spatial heterogeneity in environmental and social conditions requires locally specific decisions.
Understanding of ecological processes must underpin all management decisions.
The complexity and spatial heterogeneity present in silvopastoral systems requires high-resolution data and tools.
Considering these principles will be integral for maximising the positive benefits novel silvopastoral systems provide to pastoral farms.
Acknowledgements
Our thanks to all participants of the silvopastoralism workshop, especially Elizabeth McGruddy, Esther Dijkstra, David Boone, to Richard Tosswill for letting us use his farm for the venue of the workshop, and to Nicolette Faville for designing and .
Disclosure statement
No potential conflict of interest was reported by the author(s ).
Additional information
Funding
References
- Ahmed R, Hoque ATMR, Hossain MK. 2008. Allelopathic effects of leaf litters of Eucalyptus camaldulensis on some forest and agricultural crops. J For Res. 19:19–24. doi:10.1007/s11676-008-0003-x.
- Álvarez F, Casanoves F, Suárez JC. 2021. Influence of scattered trees in grazing areas on soil properties in the Piedmont region of the Colombian Amazon. PLoS One. 16:e0261612. doi:10.1371/journal.pone.0261612.
- Apolinário VXO, Dubeux JCB, Andrade Lira M, Sampaio EVSB, Amorim SO, Miranda e Silva NG, Muir JP. 2016a. Arboreal legume litter nutrient contribution to a tropical silvopasture. Agron J. 108:2478–2484. doi:10.2134/agronj2016.02.0120.
- Apolinário VXO, Dubeux JCB, Lira MA, Ferreira RLC, Mello ACL, Coelho DL, Muir JP, Sampaio EVSB. 2016b. Decomposition of arboreal legume fractions in a silvopastoral system. Crop Sci. 56:1356–1363. doi:10.2135/cropsci2015.09.0588.
- Ares A, Reid W, Brauer D. 2006. Production and economics of native pecan silvopastures in central United States. Agrofor Syst. 66:205–215. doi:10.1007/s10457-005-8302-0.
- Aryal DR, Gómez-González RR, Hernández-Nuriasmú R, Morales-Ruiz DE. 2019. Carbon stocks and tree diversity in scattered tree silvopastoral systems in Chiapas, Mexico. Agrofor Syst. 93:213–227. doi:10.1007/s10457-018-0310-y.
- Avendaño-Yáñez MDLL, Quiroz-Martínez S, Pérez-Elizalde S, López-Ortiz S. 2020. Litterfall from tropical dry forest trees scattered in pastures. Revista Chapingo serie ciencias forestales y del ambiente. 26:409–418. doi:10.5154/r.rchscfa.2019.12.092.
- Baah-Acheamfour M, Carlyle CN, Bork EW, Chang SX. 2020. Forest and perennial herbland cover reduce microbial respiration but increase root respiration in agroforestry systems. Agric For Meteorol. 280:107790. doi:10.1016/j.agrformet.2019.107790.
- Baah-Acheamfour M, Carlyle CN, Lim S-S, Bork EW, Chang SX. 2016. Forest and grassland cover types reduce net greenhouse gas emissions from agricultural soils. Sci Total Environ. 571:1115–1127. doi:10.1016/j.scitotenv.2016.07.106.
- Bahamonde HA, Peri PL, Martínez Pastur G, Lecinas MV. 2009. Variaciones microclimaticas en bosques primarios y bajo uso silvopastoril de Nothofagus antarctica en dos Clases de Sitio en Patagonia Sur. Proceedings of the 1st National Congress of Silvopastoral Systems. Misiones: INTA Editions; p. 14–16.
- Bambo SK, Nowak J, Blount AR, Long AJ, Osiecka A. 2009. Soil nitrate leaching in silvopastures compared with open pasture and pine plantation. J Environ Qual. 38:1870–1877. doi:10.2134/jeq2007.0634.
- Basher LR. 2013. Erosion processes and their control in New Zealand. In: Dymond J, editor. Ecosystem services in New Zealand – conditions and trends. Lincoln: Manaaki Whenua Press; p. 363–374.
- Belsky AJ, Amundson RG, Duxbury JM, Riha SJ, Ali AR, Mwonga SM. 1989. The effects of trees on their physical, chemical and biological environments in a semi-arid savanna in Kenya. J Appl Ecol. 26:1005. doi:10.2307/2403708.
- Belsky AJ, Mwonga SM, Amundson RG, Duxbury JM, Ali AR. 1993a. Comparative effects of isolated trees on their undercanopy environments in high- and low-rainfall savannas. J Appl Ecol. 30:143. doi:10.2307/2404278.
- Belsky AJ, Mwonga SM, Duxbury JM. 1993b. Effects of widely spaced trees and livestock grazing on understory environments in tropical savannas. Agrofor Syst. 24:1–20. doi:10.1007/BF00705265.
- Benavides R, Douglas GB, Osoro K. 2009. Silvopastoralism in New Zealand: review of effects of evergreen and deciduous trees on pasture dynamics. Agrofor Syst. 76:327–350. doi:10.1007/s10457-008-9186-6.
- Betteridge K, Costall D, Martin S, Reidy B, Stead A, Millner I. 2012. Impact of shade trees on Angus cow behaviour and physiology in summer dry hill country: grazing activity, skin temperature and nutrient transfer issues. In: Currie LD, Christensen CL, editors. Advanced nutrient management: gains from the past – goals for the future. Palmerston North, New Zealand: Fertiliser and Lime Research Centre, Massey University; p. 1–12.
- Blackshaw JK, Blackshaw AW. 1994. Heat stress in cattle and the effect of shade on production and behaviour: a review. Aust J Exp Agric. 34:285–295. doi:10.1071/EA9940285.
- Borremans L, Reubens B, Van Gils B, Baeyens D, Vandevelde C, Wauters E. 2016. A sociopsychological analysis of agroforestry adoption in Flanders: understanding the discrepancy between conceptual opportunities and actual implementation. Agroecol Sustain Food Syst. 40:1008–1036. doi:10.1080/21683565.2016.1204643.
- Brown P, Mortimer C, Meurk C. 2012. Visual landscape preferences in the Canterbury region. Lincoln: Landcare Research.
- Bruck SR, Bishaw B, Cushing TL, Cubbage FW. 2019. Modeling the financial potential of silvopasture agroforestry in eastern North Carolina and Northeastern Oregon. J For. 117:13–20. doi:10.1093/jofore/fvy065.
- Bryant JR, Snow VO. 2010. Modelling pastoral farm agro-ecosystems: a review. N Z J Agric Res. 51:349–363. doi:10.1080/00288230809510466.
- Buergler AL, Fike JH, Burger JA, Feldhake CM, McKenna JR, Teutsch CD. 2006. Forage nutritive value in an emulated silvopasture. Agron J. 98:1265–1273. doi:10.2134/agronj2005.0199.
- Buergler AL, Fike JH, Burger JA, Feldhake CR, McKenna JA, Teutsch CD. 2005. Botanical composition and forage production in an emulated silvopasture. Agron J. 97:1141–1147. doi:10.2134/agronj2004.0308.
- Cá J, Lustosa Filho JF, da Silva NR, de Castro CRT, de Oliveira TS. 2022. C and N stocks in silvopastoral systems with high and low tree diversity: evidence from a twenty-two year old field study. Sci Total Environ. 833:155298. doi:10.1016/j.scitotenv.2022.155298.
- Callaway RM, Nadkarni NM, Mahall BE. 1991. Facilitation and interference of Quercus douglasii on understory productivity in central California. Ecology. 72:1484–1499. doi:10.2307/1941122.
- Cardoso ADS, Alari FDO, de Andrade N, Nascimento TDS, van Cleef FDOS, Alves BJR, Ruggieri AC, Malheiros EB. 2022. Nitrous oxide emissions in silvopastoral systems: key driving variables and seasonality. Agric For Meteorol. 316:108851. doi:10.1016/j.agrformet.2022.108851.
- Casals P, Romero J, Rusch GM, Ibrahim M. 2014. Soil organic C and nutrient contents under trees with different functional characteristics in seasonally dry tropical silvopastures. Plant Soil. 374:643–659. doi:10.1007/s11104-013-1884-9.
- Castillo MS, Tiezzi F, Franzluebbers AJ. 2020. Tree species effects on understory forage productivity and microclimate in a silvopasture of the Southeastern USA. Agric Ecosyst Environ. 295:106917. doi:10.1016/j.agee.2020.106917.
- Chang X, Amatya G, Beare MH, Mead DJ. 2002. Soil properties under a Pinus radiata – ryegrass silvopastoral system in New Zealand. Part I. Soil N and moisture availability, soil C, and tree growth. Agrofor Syst. 54:137–147.
- Charlton D, McIvor IR, Gawith P, Douglas G. 2007. Growing poplar and willow trees on farms – guidelines for establishing and managing poplar and willow trees on farms. Compiled and Prepared by the National Poplar and Willow Users Group as part of the Sustainable Farming Fund’s Poplar & Willow Project (Grant No. 04/089), New Zealand.
- Chen H, Zhang X, Abla M, Lü D, Yan R, Ren Q, Ren Z, Yang Y, Zhao W, Lin P, et al. 2018. Effects of vegetation and rainfall types on surface runoff and soil erosion on steep slopes on the Loess Plateau, China. Catena. 170:141–149. doi:10.1016/j.catena.2018.06.006.
- Cloete SWP, Muller A, Steyn S, van der Merwe DA, Nel CL, Cloete S, Kruger ACM, Brand TS. 2021. The effect of tree shade on ambient conditions and heat stress indicator traits of new-born South African Mutton Merino and Dormer lambs: preliminary results. J Therm Biol. 99:103024. doi:10.1016/j.jtherbio.2021.103024.
- Cossens GG, Cossens GS. 1995. Akatore agroforestry trail results from 1974–1995 (No. Report No. 26). Agroforestry Research Collaborative.
- Cossens GG, Hawke MF. 2000. Agroforestry in Eastern Otago: results from two long-term experiments. Proc N Z Grassl Assoc. 62:93–98. doi:10.33584/jnzg.2000.62.2398.
- Crawford TW, Messina JP, Manson SM, O’Sullivan D. 2005. Complexity science, complex systems, and land-use research. Environ Plan B Plan Des. 32:792–798. doi:10.1068/b3206ed.
- Cubera E, Manuel Nunes J, Madeira M, Gazarini L. 2009. Influence of Quercus ilex trees on herbaceous production and nutrient concentrations in southern Portugal. J Plant Nutr Soil Sci. 172:565–571. doi:10.1002/jpln.200800191.
- Dada JMV, Santos MLPD, Muniz PC, Nunes-Zotti MLA, Barros FROD, Vieira FMC. 2021. Postpartum behavioural response of Santa inês x dorper ewes and lambs in a silvopastoral system. Small Rumin Res. 203:106495. doi:10.1016/j.smallrumres.2021.106495.
- De K, Sharma S, Kumawat PK, Kumar D, Mohapatra A, Sahoo A. 2020. Tree shade improves the comfort of sheep during extreme summer. J Vet Behav. 40:103–107. doi:10.1016/j.jveb.2020.10.003.
- de Aguiar MI, Maia SMF, Xavier FADS, de Sá Mendonça E, Filho JAA, de Oliveira TS. 2010. Sediment, nutrient and water losses by water erosion under agroforestry systems in the semi-arid region in northeastern Brazil. Agrofor Syst. 79:277–289. doi:10.1007/s10457-010-9310-2.
- Dean G, Rivera-ferre MG, Rosas-casals M, Lopez-i-gelats F. 2021. Nature’s contribution to people as a framework for examining socioecological systems: the case of pastoral systems. Ecosyst Serv. 49:101265. doi:10.1016/j.ecoser.2021.101265.
- DeBruyne SA, Feldhake CM, Burger JA, Fike JH. 2011. Tree effects on forage growth and soil water in an Appalachian silvopasture. Agrofor Syst. 83:189–200. doi:10.1007/s10457-011-9376-5.
- Devkota NR, Wall AJ, Kemp PD, Hodgson J. 1997. Relationship between canopy closure and pasture production in deciduous tree based temperate silvopastoral systems. Proceedings of the XVIII International Grasslands Conference. Winnipeg and Saskatoo, Canada; p. 652–653.
- Dold C, Thomas AL, Ashworth AJ, Philipp D, Brauer DK, Sauer TJ. 2019. Carbon sequestration and nitrogen uptake in a temperate silvopasture system. Nutr Cycl Agroecosystems. 114:85–98. doi:10.1007/s10705-019-09987-y.
- Douglas G, Mackay A, Vibart R, Dodd M, McIvor I, McKenzie C. 2020. Soil carbon stocks under grazed pasture and pasture-tree systems. Sci Total Environ. 715:136910. doi:10.1016/j.scitotenv.2020.136910.
- Douglas GB, Mcivor IR, Manderson AK, Koolaard JP, Todd M, Braaksma S, Gray RAJ. 2013. Reducing shallow landslide occurrence in pastoral hill country using wide-spaced trees. Land Degrad Dev. 24:103–114. doi:10.1002/ldr.1106.
- Douglas GB, Walcroft AS, Wills BJ, Hurst SE, Foote AG, Trainor KD, Fung LE. 2001. Resident pasture growth and the micro-environment beneath young, widespaced poplars in New Zealand. Proc N Z Grassl Assoc. 63:131–138. doi:10.33584/jnzg.2001.63.2441.
- Dubeux JC, Lira MDA, dos Santos MVF, Muir J, da Silva MA, Teixeira VI, de Mello ACL. 2014. Soil characteristics under legume and non-legume tree canopies in signalgrass (Brachiaria decumbens) pastures. Afr J Range Forage Sci. 31:37–42. doi:10.2989/10220119.2014.880746.
- Durr PA, Rangel J. 2002. Enhanced forage production under Samanea saman in a subhumid tropical grassland. Agrofor Syst. 54:99–102. doi:10.1023/A:1015070203455.
- Eason WR. 1991. The effect of tree leaf litter on sward botanical composition and growth. For Ecol Manag. 45:165–172. doi:10.1016/0378-1127(91)90214-G.
- Fannon AG, Fike JH, Greiner SP, Feldhake CM, Wahlberg MA. 2017. Hair sheep performance in a mid-stage deciduous Appalachian silvopasture. Agrofor Syst. 93:81–93. doi:10.1007/s10457-017-0154-x.
- Fernández-Moya J, San Miguel-Ayanz A, Cañellas I, Gea-Izquierdo G. 2011. Variability in Mediterranean annual grassland diversity driven by small-scale changes in fertility and radiation. Plant Ecol. 212:865–877. doi:10.1007/s11258-010-9869-8.
- Fischer J, Zerger A, Gibbons P, Stott J, Law BS. 2010. Tree decline and the future of Australian farmland biodiversity. Proc Natl Acad Sci. 107:19597–19602. doi:10.1073/pnas.1008476107.
- Fisher AD, Roberts N, Bluett SJ, Verkerk GA, Matthews LR. 2008. Effects of shade provision on the behaviour, body temperature and milk production of grazing dairy cows during a New Zealand summer. N Z J Agric Res. 51:99–105. doi:10.1080/00288230809510439.
- Franzel S, Carsan S, Lukuyu B, Sinja J, Wambugu C. 2014. Fodder trees for improving livestock productivity and smallholder livelihoods in Africa. Curr Opin Environ Sustain. 6:98–103. doi:10.1016/j.cosust.2013.11.008.
- Frey GE, Fassola HE, Pachas AN, Colcombet L, Lacorte SM, Pérez O, Renkow M, Warren ST, Cubbage FW. 2012. Perceptions of silvopasture systems among adopters in northeast Argentina. Agric Syst. 105:21–32. doi:10.1016/j.agsy.2011.09.001.
- Frost WE, McDougald NK. 1989. Tree canopy effects on herbaceous production of annual rangeland during drought. J Range Manag. 42:281–283. doi:10.2307/3899494.
- Fuller IC, Death RG. 2018. The science of connected ecosystems: what is the role of catchment-scale connectivity for healthy river ecology? Land Degrad Dev. 29:1413–1426. doi:10.1002/ldr.2903.
- Galicia L, Garcı́a-Oliva F. 2004. The effects of C, N and P additions on soil microbial activity under two remnant tree species in a tropical seasonal pasture. Applied Soil Ecology. 26:31–39. doi:10.1016/j.apsoil.2003.10.006.
- Garrido P, Elbakidze M, Angelstam P, Plieninger T, Pulido F, Moreno G. 2017. Stakeholder perspectives of wood-pasture ecosystem services: a case study from Iberian dehesas. Land Use Policy. 60:324–333. doi:10.1016/j.landusepol.2016.10.022.
- Gea-Izquierdo G, Montero G, Cañellas I. 2009. Changes in limiting resources determine spatio-temporal variability in tree–grass interactions. Agrofor Syst. 76:375–387. doi:10.1007/s10457-009-9211-4.
- Gilchrist AN, Hall JRD, Foote AG, Bulloch BT. 1993. Pasture growth around trees planted for grassland stability. Proceedings of the XVII Grassland Congress. Rockhampton, Queensland, Australia; p. 2062–2063.
- Glade T. 2003. Landslide occurrence as a response to land use change: a review of evidence from New Zealand. Catena. 51:297–314. doi:10.1016/S0341-8162(02)00170-4.
- Goff JR. 1997. A chronology of natural and anthropogenic influences on coastal sedimentation, New Zealand. Mar Geol. 138:105–117. doi:10.1016/S0025-3227(97)00018-2.
- Gomes da Silva IA, Dubeux JCB, Melo ACL, da Cunha MV, dos Santos MVF, Apolinário VXO, Freitas EV. 2021a. Tree legume enhances livestock performance in a silvopasture system. Agron J. 113:358–369. doi:10.1002/agj2.20491.
- Gomes da Silva IA, Dubeux JCB, Santos MVF, de Mello ACL, Cunha MV, Apolinário VXO, de Freitas EV. 2021b. Tree canopy management affects dynamics of herbaceous vegetation and soil moisture in silvopasture systems using arboreal legumes. Agronomy. 11:1509. doi:10.3390/agronomy11081509.
- Gómez-Cifuentes A, Giménez Gómez VC, Moreno CE, Zurita GA. 2019. Tree retention in cattle ranching systems partially preserves dung beetle diversity and functional groups in the semideciduous Atlantic forest: the role of microclimate and soil conditions. Basic Appl Ecol. 34:64–74. doi:10.1016/j.baae.2018.10.002.
- Gómez-Cifuentes A, Vespa N, Semmartín M, Zurita G. 2020. Canopy cover is a key factor to preserve the ecological functions of dung beetles in the southern Atlantic forest. Appl Soil Ecol. 154:103652. doi:10.1016/j.apsoil.2020.103652.
- Gouveia AC, Freitas H. 2008. Intraspecific competition and water use efficiency in Quercus suber: evidence of an optimum tree density? Trees. 22:521–530. doi:10.1007/s00468-008-0212-0.
- Green MO. 2013. Catchment sediment load limits to achieve estuary sedimentation targets. N Z J Mar Freshw Res. 47:153–180. doi:10.1080/00288330.2012.757241.
- Grewal SS, Juneja ML, Singh K, Singh S. 1994. A comparison of two agroforestry systems for soil, water and nutrient conservation on degraded land. Soil Technol. 7:145–153. doi:10.1016/0933-3630(94)90016-7.
- Guevara S, Meave J, Moreno-Casasola P, Laborde J. 1992. Floristic composition and structure of vegetation under isolated trees in neotropical pastures. Journal of Vegetation Science. 3:655–664. doi:10.2307/3235833.
- Guevara-Escobar A, Kemp PD, Mackay A, Hodgson J. 2002. Soil properties of a widely spaced, planted poplar (Populus deltoides)-pasture system in a hill environment. Aust J Soil Res. 40:873–886. doi:10.1071/SR01080.
- Guevara-Escobar A, Kemp PD, Mackay AD, Hodgson J. 2007. Pasture production and composition under poplar in a hill environment in New Zealand. Agrofor Syst. 69:199–213. doi:10.1007/s10457-007-9038-9.
- Haile SG, Nair VD, Nair PKR. 2010. Contribution of trees to carbon storage in soils of silvopastoral systems in Florida, USA: contribution of trees to carbon storage in soils. Glob Change Biol. 16:427–438. doi:10.1111/j.1365-2486.2009.01981.x.
- Halvorson JJ, Belesky DP, West MS. 2017. Inhibition of forage seed germination by leaf litter extracts of overstory hardwoods used in silvopastoral systems. Agrofor Syst. 91:69–83. doi:10.1007/s10457-016-9908-0.
- Harcourt N, Robson-Williams M, Tamepo R. 2022. Supporting the design of useful and relevant holistic frameworks for land use opportunity assessment for indigenous people. Australas J Water Resour. 26:116–130. doi:10.1080/13241583.2022.2031571.
- Hawke MF. 1991. Pasture production and animal performance under pine agroforestry in New Zealand. For Ecol Manag. 45:109–118. doi:10.1016/0378-1127(91)90210-M.
- He X-H, Critchley C, Bledsoe C. 2003. Nitrogen transfer within and between plants through common mycorrhizal networks (CMNs). Crit Rev Plant Sci. 22:531–567. doi:10.1080/713608315.
- Herrera AM, de Mello ACL, Apolinário VXO, Dubeux Júnior JCB, da Silva VJ, dos Santos MVF, da Cunha MV. 2020. Decomposition of senescent leaves of signalgrass (Urochloa decumbens Stapf. R. Webster) and arboreal legumes in silvopastoral systems. Agrofor Syst. 94:2213–2224. doi:10.1007/s10457-020-00542-1.
- Hicks DM, Hill J, Shankar U. 1996. Variation of suspended sediment yields around New Zealand: the relative importance of rainfall and geology. IAHS-AISH Publ. 236:149–156.
- Hicks DM, Shankar U, Mckerchar AI, Basher L, Lynn I, Page M, Jessen M. 2011. Suspended sediment yields from New Zealand rivers. J Hydrol N Z. 50:81–142.
- Holderieath J, Valdivia C, Godsey L, Barbieri C. 2012. The potential for carbon offset trading to provide added incentive to adopt silvopasture and alley cropping in Missouri. Agrofor Syst. 86:345–353. doi:10.1007/s10457-012-9543-3.
- Hoosbeek MR, Remme RP, Rusch GM. 2018. Trees enhance soil carbon sequestration and nutrient cycling in a silvopastoral system in south-western Nicaragua. Agrofor Syst. 92:263–273. doi:10.1007/s10457-016-0049-2.
- Howlett DS, Moreno G, Mosquera Losada MR, Nair PKR, Nair VD. 2011. Soil carbon storage as influenced by tree cover in the Dehesa cork oak silvopasture of central-western Spain. J Environ Monit. 13:1897. doi:10.1039/c1em10059a.
- Huang IB, Keisler J, Linkov I. 2011. Multi-criteria decision analysis in environmental sciences: ten years of applications and trends. Sci Total Environ. 409:3578–3594. doi:10.1016/j.scitotenv.2011.06.022.
- Jackson RA, Townsend KG, Hawke MF. 1986. The availability of ovine infective trichostrongyle larvae on forested paddocks. New Zeal Vet J. 34(12):205–209. http://dx.doi.org/10.1080/00480169.1986.35351.
- Jakobsson S, Plue J, Cousins SAO, Lindborg R. 2019. Exploring the effects of pasture trees on plant community patterns. J Veg Sci. 30:809–820. doi:10.1111/jvs.12771.
- Jansson MB. 1988. A global survey of sediment yield. Geogr Ann Ser Phys Geogr. 70:81–98. doi:10.1080/04353676.1988.11880241.
- Jørgensen SE. 2012. Introduction to systems ecology. 1st ed. Boca Raton, FL: CRC Press/Taylor & Francis.
- Jose S. 2009. Agroforestry for ecosystem services and environmental benefits: an overview. Agrofor Syst. 76:1–10. doi:10.1007/s10457-009-9229-7.
- Jose S, Dollinger J. 2019. Silvopasture: a sustainable livestock production system. Agrofor Syst. 93:1–9. doi:10.1007/s10457-019-00366-8.
- Jose S, Walter D, Mohan Kumar B. 2019. Ecological considerations in sustainable silvopasture design and management. Agrofor Syst. 93:317–331. doi:10.1007/s10457-016-0065-2.
- Kemp PD, Hawke M, Knowles R. 2018. Temperate agroforestry systems in New Zealand. In: Gordon AW, Newman SM, Coleman BRW, editors. Temperate agroforestry systems. Oxfordshire: CAB International; p. 224–236.
- Kemp PD, Mackay AD, Matheson LA, Timmins ME. 2001. The forage value of poplars and willows. Proc N Z Grassl Assoc. 63:115–119. doi:10.33584/jnzg.2001.63.2444.
- Kendall PE, Nielsen PP, Webster JR, Verkerk GA, Littlejohn RP, Matthews LR. 2006. The effects of providing shade to lactating dairy cows in a temperate climate. Livest Sci. 103:148–157. doi:10.1016/j.livsci.2006.02.004.
- Klopfenstein NB, Rietveld WJ, Carman RC, Clason TR, Sharrow SH, Garrett G, Anderson B. 1997. Silvopasture: an agroforestry practice. Agrofor Notes. 8:1–4.
- Lana ÂMQ, Lana RMQ, Lemes EM, Reis GL, Moreira GHFA. 2016. Influence of native or exotic trees on soil fertility in decades of silvopastoral system at the Brazilian savannah biome. Agroforest Syst. 92:415–424. doi:10.1007/s10457-016-9998-8.
- Lencinas MV, Kreps G, Soler R, Peri PL, Porta A, Ramírez M, Pastur GM. 2015. Neochelanops michaelseni (Pseudoscorpiones: Chernetidae) as a potential bioindicator in managed and unmanaged Nothofagus forests of Tierra del Fuego. J Arachnol. 43:406–412. doi:10.1636/0161-8202-43.3.406.
- Lencinas MV, Martínez Pastur G, Rivero P, Busso C. 2008. Conservation value of timber quality versus associated non-timber quality stands for understory diversity in Nothofagus forests. Biodivers Conserv. 17:2579–2597. doi:10.1007/s10531-008-9323-6.
- Levia DF, Hudson SA, Llorens P, Nanko K. 2017. Throughfall drop size distributions: a review and prospectus for future research. WIREs Water. 4:e1225. doi:10.1002/wat2.1225.
- Li G, Wan L, Cui M, Wu B, Zhou J. 2019. Influence of canopy interception and rainfall kinetic energy on soil erosion under forests. Forests. 10:509. doi:10.3390/f10060509.
- Lima HNB, Dubeux JCB Jr, Santos MVF, Mello ACL, Lira MA, Cunha MV, De Freitas EV, Apolinário VXO. 2020. Herbage responses of signalgrass under full sun or shade in a silvopasture system using tree legumes. Agron J. 112:1839–1848. doi:10.1002/agj2.20137.
- López-Díaz ML, Rolo V, Moreno G. 2011. Trees’ role in nitrogen leaching after organic, mineral fertilization: a greenhouse experiment. J Environ Qual. 40:853–859. doi:10.2134/jeq2010.0165.
- López-Santiago JG, Casanova-Lugo F, Villanueva-López G, Díaz-Echeverría VF, Solorio-Sánchez FJ, Martínez-Zurimendi P, Aryal DR, Chay-Canul AJ. 2019. Carbon storage in a silvopastoral system compared to that in a deciduous dry forest in Michoacán, Mexico. Agrofor Syst. 93:199–211. doi:10.1007/s10457-018-0259-x.
- Lorenz K, Lal R. 2014. Soil organic carbon sequestration in agroforestry systems. A review. Agron Sustain Dev. 34:443–454. doi:10.1007/s13593-014-0212-y.
- Ludwig F, Kroon H, Prins HHT, Berendse F. 2001. Effects of nutrients and shade on tree-grass interactions in an East African savanna. J Veg Sci. 12:579–588. doi:10.2307/3237009.
- Mackay-Smith TH, Burkitt L, Reid J, López IF, Phillips C. 2021. A framework for reviewing silvopastoralism: a New Zealand hill country case study. Land. 10:1386. doi:10.3390/land10121386.
- Mackay-Smith TH, Burkitt LL, López IF, Reid JI. 2022a. The impact of a kānuka silvopastoral system on surface runoff and sediment and nutrient losses in New Zealand hill country. Catena. 213:106215. doi:10.1016/j.catena.2022.106215.
- Mackay-Smith TH, López IF, Burkitt LL, Reid JI. 2022b. Kānuka trees facilitate pasture production increases in New Zealand hill country. Agronomy. 12:1701. doi:10.3390/agronomy12071701.
- Mackay‐Smith TH, López IF, Burkitt LL, Reid JI. 2023. Pasture production–diversity relationships in a kānuka silvopastoral system. Ecol Solut Evid. 4(2). http://dx.doi.org/10.1002/eso3.v4.2.
- Mancera KF, Zarza H, Buen LLD, Adolfo A, García C, Palacios FM, Galindo F. 2018. Integrating links between tree coverage and cattle welfare in silvopastoral systems evaluation. Agron Sustain Dev. 38:1–9. doi:10.1007/s13593-017-0478-y.
- Martínez J, Cajas YS, León JD, Osorio NW. 2014. Silvopastoral systems enhance soil quality in grasslands of Colombia. Appl Environ Soil Sci. 2014:1–8. doi:10.1155/2014/359736.
- McDowell RW, Wilcock RJ. 2008. Water quality and the effects of different pastoral animals. N Z Vet J. 56:289–296. doi:10.1080/00480169.2008.36849.
- McIvor I, Mackay-Smith TH, Spiekermann RI. 2023. Drivers and New Opportunities for Woody Vegetation Use in Erosion Management in Pastoral Hill Country in New Zealand. In: Mahmood S, editor. Soil Erosion - Risk Modeling and Management. London: IntechOpen.
- McIvor IR, Clarke K, Douglas G. 2015. Effectiveness of conservation trees in reducing erosion following a storm event. In: Currie LD, Burkitt LL, editors. Proceedings, 28th Annual Fertiliser and Lime Research Centre Workshop ‘Moving Farm Systems to Improved Attenuation’. Occasional Report 28. Palmerston North: Massey University; p. 1–12.
- Menezes RSC, Salcedo IH. 1999. Influence of tree species on the herbaceous understory and soil chemical characteristics in a silvopastoral system in semi-arid northeastern Brazil. Rev Bras Ciênc Solo. 23:817–826. doi:10.1590/S0100-06831999000400008.
- Menezes RSC, Salcedo IH, Elliott ET. 2002. Microclimate and nutrient dynamics in a silvopastoral system of semiarid northeastern Brazil. Agrofor Syst. 56:27–38. doi:10.1023/A:1021172530939.
- Michel G-A, Nair VD, Nair PKR. 2007. Silvopasture for reducing phosphorus loss from subtropical sandy soils. Plant Soil. 297:267–276. doi:10.1007/s11104-007-9352-z.
- Ministry for Primary Industries. 2020. Forest land in the ETS [WWW document]. [accessed 2021 April 25]. https://www.mpi.govt.nz/forestry/forestry-in-the-emissions-trading-scheme/forest-land-in-the-ets.
- Montagnini F, Nair P. 2016. Carbon sequestration: an underexploited environmental benefit of agroforestry systems. Agrofor Syst. 61:281–295. doi:10.1023/B:AGFO.0000029005.92691.79.
- Moreno G. 2008. Response of understorey forage to multiple tree effects in Iberian dehesas. Agric Ecosyst Environ. 123:239–244. doi:10.1016/j.agee.2007.04.006.
- Moreno G, Aviron S, Berg S, Crous-Duran J, Franca A, de Jalón SG, Hartel T, Mirck J, Pantera A, Palma JHN, et al. 2018. Agroforestry systems of high nature and cultural value in Europe: provision of commercial goods and other ecosystem services. Agrofor Syst. 92:877–891. doi:10.1007/s10457-017-0126-1.
- Moreno G, Obrador J, García E, Cubera E, Montero M, Pulido FJ. 2005. Consequences of dehesa management on the tree-understory interactions. Proceedings of the Silvopastoralism and Sustainable Land Management. Lugo: CAB International.
- Mupepele A-C, Keller M, Dormann CF. 2021. European agroforestry has no unequivocal effect on biodiversity: a time-cumulative meta-analysis. BMC Ecol Evol. 21:193. doi:10.1186/s12862-021-01911-9.
- Nair PKR. 1993. An introduction to agroforestry. 1st ed. Dordrecht: Kluwer Academic Publishers in Cooperation with International Centre for Research in Agroforestry.
- Nair PKR. 2012. Climate change mitigation and adaptation: a low hanging fruit of agroforestry. In: Nair P, Garrity D, editors. Agroforestry: the future of global land use. Dordrecht: Springer; p. 31–36.
- Nair PKR, Kumar BM, Nair VD. 2022. An introduction to agroforestry: four decades of scientific developments. 2nd ed. Cham: Springer International Publishing. doi:10.1007/978-3-030-75358-0.
- Nair VD, Nair PKR, Kalmbacher RS, Ezenwa IV. 2007. Reducing nutrient loss from farms through silvopastoral practices in coarse-textured soils of Florida, USA. Ecol Eng. 29:192–199. doi:10.1016/j.ecoleng.2006.07.003.
- Nanko K, Hotta N, Suzuki M. 2006. Evaluating the influence of canopy species and meteorological factors on throughfall drop size distribution. J Hydrol. 329:422–431. doi:10.1016/j.jhydrol.2006.02.036.
- Odum EP. 1977. The emergence of ecology as a new integrative discipline: ecology must combine holism with reductionism if applications are to benefit society. Science. 195:1289–1293. doi:10.1126/science.195.4284.1289.
- Orefice J, Carroll J, Conroy D, Ketner L. 2017. Silvopasture practices and perspectives in the Northeastern United States. Agrofor Syst. 91:149–160. doi:10.1007/s10457-016-9916-0.
- O’Sullivan D. 2004. Complexity science and human geography. Trans Inst Br Geogr. 29:282–295. doi:10.1111/j.0020-2754.2004.00321.x.
- Pent GJ. 2020. Over-yielding in temperate silvopastures: a meta-analysis. Agrofor Syst. 94:1741–1758. doi:10.1007/s10457-020-00494-6.
- Pent GJ, Fike JH. 2019. Lamb productivity on stockpiled fescue in honeylocust and black walnut silvopastures. Agrofor Syst. 93:113–121. doi:10.1007/s10457-018-0264-0.
- Pent GJ, Fike JH, Kim I. 2021. Ewe lamb vaginal temperatures in hardwood silvopastures. Agrofor Syst. 95:21–32. doi:10.1007/s10457-018-0221-y.
- Pent GJ, Greiner SP, Munsell JF, Tracy BF, Fike JH. 2020a. Lamb performance in hardwood silvopastures, II: animal behavior in summer. Transl Anim Sci. 4:363–375. doi:10.1093/tas/txz177.
- Pent GJ, Greiner SP, Munsell JF, Tracy BF, Fike JH. 2020b. Lamb performance in hardwood silvopastures, I: animal gains and forage measures in summer. Transl Anim Sci. 4:385–399. doi:10.1093/tas/txz154.
- Percival NS, Knowles RL. 1988. Relationship between radiata pine and understorey pasture production. Agroforestry Symposium Proceedings. Rotorua: Forest Research Institute and New Zealand Ministry of Forestry; p. 153–164.
- Peri PL. 2005. Patagonia Sur. Sistemas Silvopastoriles en Ñirantales. IDIA XXI For. 5:245–249.
- Peri PL, Bahamonde HA, Lencinas MV, Gargaglione V, Soler R, Ormaechea S, Pastur GM. 2016. A review of silvopastoral systems in native forests of Nothofagus antarctica in southern Patagonia, Argentina. Agrofor Syst. 90:933–960. doi:10.1007/s10457-016-9890-6.
- Peri PL, Ormaechea S. 2013. Relevamiento de los bosques nativos de n ̃ire (Nothofagus antarctica) en Santa Cruz: base para su conservacio ́n y manejo. Buenos Aires: Ediciones INTA.
- Pezzopane JRM, Nicodemo MLF, Bosi C, Garcia AR, Lulu J. 2019. Animal thermal comfort indexes in silvopastoral systems with different tree arrangements. J Therm Biol. 79:103–111. doi:10.1016/j.jtherbio.2018.12.015.
- Phillips C, Marden M. 2005. Reforestation schemes to manage regional landslide risk. In: Glade T, Anderson MG, Crozier MJ, editors. Landslide hazard and risk. New Jersey, USA: John Wiley & Sons; p. 517–547.
- Phillips C, Marden M, Basher LR. 2018. Geomorphology and forest management in New Zealand’s erodible steeplands: an overview. Geomorphology. 307:107–121. doi:10.1016/j.geomorph.2017.07.031.
- Phillips C, Marden M, Suzanne SM. 2014. Observations of root growth of young poplar and willow planting types. N Z J For Sci. 44:15. doi:10.1186/s40490-014-0015-6.
- Pizarro D, Vásquez H, Bernal W, Fuentes E, Alegre J, Castillo MS, Gómez C. 2020. Assessment of silvopasture systems in the northern Peruvian Amazon. Agrofor Syst. 94:173–183. doi:10.1007/s10457-019-00381-9.
- Plevich JO, Gyenge J, Delgado AS, Tarico JC, Fiandino S, Utello MJ. 2019. Production of fodder in a treeless system and in silvopastoral system in central Argentina. Floresta E Ambiente. 26:e20160517. doi:10.1590/2179-8087.051716.
- Plieninger T, Flinzberger L, Hetman M, Horstmannshoff I, Reinhard-Kolempas M, Topp E, Moreno G, Huntsinger L. 2021. Dehesas as high nature value farming systems: a social-ecological synthesis of drivers, pressures, state, impacts, and responses. Ecol Soc. 26:art23. doi:10.5751/ES-12647-260323.
- Plieninger T, Huntsinger L. 2018. Complex rangeland systems: integrated social-ecological approaches to silvopastoralism. Rangel Ecol Manag. 71:519–525. doi:10.1016/j.rama.2018.05.002.
- Pollard JC. 2006. Shelter for lambing sheep in New Zealand: a review. N Z J Agric Res. 49:395–404. doi:10.1080/00288233.2006.9513730.
- Poudel S, Fike JH, Pent GJ. 2022. Hair cortisol as a measure of chronic stress in ewes grazing either hardwood silvopastures or open pastures. Agronomy. 12:1566. doi:10.3390/agronomy12071566.
- Pulido FJ, Dı́az M, Hidalgo de Trucios SJ. 2001. Size structure and regeneration of Spanish holm oak Quercus ilex forests and dehesas: effects of agroforestry use on their long-term sustainability. For Ecol Manag. 146:1–13. doi:10.1016/S0378-1127(00)00443-6.
- Ratliff RD, Duncan DA, Westfall SE. 1991. California oak-woodland overstory species affect herbage understory: management implications. J Range Manag. 44:306. doi:10.2307/4002388.
- Rhoades CC. 1996. Single-tree influences on soil properties in agroforestry: lessons from natural forest and savanna ecosystems. Agrofor Syst. 35:71–94. doi:10.1007/BF02345330.
- Rhoades CC, Eckert GE, Coleman DC. 1998. Effect of pasture trees on soil nitrogen and organic matter: implications for tropical montane forest restoration. Restor Ecol. 6:262–270. doi:10.1046/j.1526-100X.1998.00639.x.
- Rice EL. 1974. Allelopathy. 1st ed. New York: Academic Press.
- Rivest D, Paquette A, Moreno G, Messier C. 2013. A meta-analysis reveals mostly neutral influence of scattered trees on pasture yield along with some contrasted effects depending on functional groups and rainfall conditions. Agric Ecosyst Environ. 165:74–79. doi:10.1016/j.agee.2012.12.010.
- Robinson LW. 2009. A complex-systems approach to pastoral commons. Hum Ecol. 37:441–451. doi:10.1007/s10745-009-9253-2.
- Rodriguez-Rigueiro FJ, Santiago-Freijanes JJ, Mosquera-Losada MR, Castro M, Silva-Losada P, Pisanelli A, Pantera A, Rigueiro-Rodriguez A, Ferreiro-Dominguez N. 2021. Silvopasture policy promotion in European Mediterranean areas. PLoS One. 16:1–16. doi:10.1371/journal.pone.0245846.
- Roos PC, Allsopp N. 1997. Soil nutrient ecology associated with Acacia sieberana at different tree densities in a South African savanna. Afr J Range Forage Sci. 14:39–44. doi:10.1080/10220119.1997.9647918.
- Rossetti I, Bagella S, Cappai C, Caria MC, Lai R, Roggero PP, Martins da Silva P, Sousa JP, Querner P, Seddaiu G. 2015. Isolated cork oak trees affect soil properties and biodiversity in a Mediterranean wooded grassland. Agric Ecosyst Environ. 202:203–216. doi:10.1016/j.agee.2015.01.008.
- Rozados-Lorenzo MJ, González-Hernández MP, Silva-Pando FJ. 2007. Pasture production under different tree species and densities in an Atlantic silvopastoral system. Agrofor Syst. 70:53–62. doi:10.1007/s10457-007-9032-2.
- Rusch GM, Zapata PC, Casanoves F, Casals P, Ibrahim M, DeClerck F. 2014. Determinants of grassland primary production in seasonally-dry silvopastoral systems in Central America. Agrofor Syst. 88:517–526. doi:10.1007/s10457-014-9711-8.
- Saggar S, Hedley CB, Salt GJ. 2001. Soil microbial biomass, metabolic quotient, and carbon and nitrogen mineralisation in 25-year-old Pinus radiata agroforestry regimes. Soil Res. 39:491. doi:10.1071/SR00012.
- Salt GW. 1979. A comment on the use of the term emergent properties. Am Nat. 113:145–148. doi:10.1086/283370.
- Sánchez-González M, Tomé M, Montero G. 2005. Modelling height and diameter growth of dominant cork oak trees in Spain. Ann For Sci. 62:633–643. doi:10.1051/forest:2005065.
- Santos-Reis M, Correia AI. 1999. Caracterizaçao da flora e fauna do montado da Heredade da Ribeira Abaixo (Grândola, Baixo Alentejo). Lisbon: CBA.
- Sarto MVM, Borges WLB, Sarto JRW, Pires CAB, Rice CW, Rosolem CA. 2020. Soil microbial community and activity in a tropical integrated crop-livestock system. Applied Soil Ecology. 145:103350. doi:10.1016/j.apsoil.2019.08.012.
- Schmidt KM, Roering JJ, Stock JD, Dietrich WE, Montgomery DR, Schaub T. 2001. The variability of root cohesion as an influence on shallow landslide susceptibility in the Oregon coast range. Can Geotech J. 38:995–1024. doi:10.1139/cgj-38-5-995.
- Seddaiu G, Bagella S, Pulina A, Cappai C, Salis L, Rossetti I, Lai R, Roggero PP. 2018. Mediterranean cork oak wooded grasslands: synergies and trade-offs between plant diversity, pasture production and soil carbon. Agrofor Syst. 92:893–908. doi:10.1007/s10457-018-0225-7.
- Sidle RC, Ochiai H. 2006. Natural factors influencing landslides. In: Sidle RC, Ochiai H, editors. Landslides: processes, prediction, and land use. American Geophysical Union; p. 41–119. doi:10.1029/18WM04.
- Skonieski FR, Souza ERD, Gregolin LCB, Fluck AC, Costa OAD, Destri J, Neto AP. 2021. Physiological response to heat stress and ingestive behavior of lactating Jersey cows in silvopasture and conventional pasture grazing systems in a Brazilian subtropical climate zone. Trop Anim Health Prod. 53:213. doi:10.1007/s11250-021-02648-9.
- Smith MM, Bentrup G, Kellerman T, MacFarland K, Straight R, Ameyaw L, Stein S. 2022. Silvopasture in the USA: a systematic review of natural resource professional and producer-reported benefits, challenges, and management activities. Agric Ecosyst Environ. 326:107818. doi:10.1016/j.agee.2021.107818.
- Somarriba E. 1992. Revisiting the past: an essay on agroforestry definition. Agrofor Syst. 19:233–240. doi:10.1007/BF00118781.
- Spiekermann R, Kienberger S, Norton J, Briones F, Weichselgartner J. 2015. The disaster-knowledge matrix – reframing and evaluating the knowledge challenges in disaster risk reduction. Int J Disaster Risk Reduct. 13:96–108. doi:10.1016/j.ijdrr.2015.05.002.
- Spiekermann RI, McColl S, Fuller I, Dymond J, Burkitt L, Smith HG. 2021. Quantifying the influence of individual trees on slope stability at landscape scale. J Environ Manage. 286:112194. doi:10.1016/j.jenvman.2021.112194.
- Spiekermann RI, Smith HG, McColl S, Burkitt L, Fuller IC. 2022a. Quantifying effectiveness of trees for landslide erosion control. Geomorphology. 396:107993. doi:10.1016/j.geomorph.2021.107993.
- Spiekermann RI, Smith HG, McColl S, Burkitt L, Fuller IC. 2022b. Development of a morphometric connectivity model to mitigate sediment derived from storm-driven shallow landslides. Ecol Eng. 180:106676. doi:10.1016/j.ecoleng.2022.106676.
- Thorrold BS, Knowles RL, Nicholas ID, Power IL, Carter JL. 1997. Evaluation of agroforestry options for three tree species. Proc N Z Grassl Assoc. 59:187–190. doi:10.33584/jnzg.1997.59.2240.
- Tölgyesi C, Bátori Z, Gallé R, Urák I, Hartel T. 2018. Shrub encroachment under the trees diversifies the herb layer in a Romanian silvopastoral system. Rangel Ecol Manag. 71:571–577. doi:10.1016/j.rama.2017.09.004.
- Torralba M, Fagerholm N, Burgess PJ, Moreno G, Plieninger T. 2016. Do European agroforestry systems enhance biodiversity and ecosystem services? A meta-analysis. Agric Ecosyst Environ. 230:150–161. doi:10.1016/j.agee.2016.06.002.
- Tozer K, Douglas G, Dodd M, Müller K. 2021. Vegetation options for increasing resilience in pastoral hill country. Front Sustain Food Syst. 5:550334. doi:10.3389/fsufs.2021.550334.
- Treydt AC, Heitkonig IMA, Prins HHT, Ludwig F. 2007. Trees improve grass quality for herbivores in African savannas. Perspect Plant Ecol Evol Syst. 8:197–205. doi:10.1016/j.ppees.2007.03.001.
- Tripathi G, Ram S, Sharma BM, Singh G. 2005. Soil faunal biodiversity and nutrient status in silvopastoral systems of Indian desert. Environ Conserv. 32:178–188. doi:10.1017/S0376892905002109.
- Vandermeulen S, Ramírez-Restrepo CA, Beckers Y, Claessens H, Bindelle J. 2018. Agroforestry for ruminants: a review of trees and shrubs as fodder in silvopastoral temperate and tropical production systems. Anim Prod Sci. 58:767. doi:10.1071/AN16434.
- van Mil HGJ, Foegeding EA, Windhab EJ, Perrot N, van der Linden E. 2014. A complex system approach to address world challenges in food and agriculture. Trends Food Sci Technol. 40:20–32. doi:10.1016/j.tifs.2014.07.005.
- Venturi M, Piras F, Corrieri F, Aguilar E, Santoro A. 2021. The multifunctional role of linear features in traditional silvopastoral systems: the sabana de morro in Dolores (El Salvador) and the pastures with carob trees in Ragusa (Italy). Biodivers Conserv. 31(10):2315–2327. doi:10.1007/s10531-021-02220-9.
- Vieira FMC, Deniz M, Vismara ES, Herbut P, Pilatti JA, Sponchiado MZ, de Oliveira Puretz B. 2020. Thermoregulatory and behaviour responses of dairy heifers raised on a silvopastoral system in a subtropical climate. Ann Anim Sci. 20:613–627. doi:10.2478/aoas-2019-0074.
- Wall AJ. 2006. The effect of poplar stand density on hill country pastures [PhD]. Palmerston North: Institute of Natural Resources, Massey University.
- Wick B, Tiessen H, Menezes RSC. 2000. Land quality changes following the conversion of the natural vegetation into silvo-pastoral systems in semi-arid NE Brazil. Plant and Soil. 222:59–70. doi:10.1023/A:1004756416281.
- Wiersum KF. 1985. Effects of various vegetation layers in an Acacia auriculiformis forest plantation on surface erosion in Java, Indonesia. In: El-Swaify SA, Moldenhauer WC, Lo A, editors. Soil erosion and conservation. Ankeny, IA: Soil Conservation Society of North America; p. 79–89.
- Wilkens P, Munsell JF, Fike JH, Pent GJ, Frey GE. 2022. Is livestock producers’ interest in silvopasture related to their operational perspectives or characteristics? Agrofor Syst. 96:541–551. doi:10.1007/s10457-021-00664-0.
- Wilkinson AG. 1999. Poplars and willows for soil erosion control in New Zealand. Biomass Bioenergy. 16:263–274. doi:10.1016/S0961-9534(99)00007-0.
- Wilson B. 2002. Influence of scattered paddock trees on surface soil properties: a study of the Northern Tablelands of NSW. Ecol Manag Restor. 3:211–219. doi:10.1046/j.1442-8903.2002.00115.x.
- Wossink A, Swinton SM. 2007. Jointness in production and farmers’ willingness to supply non-marketed ecosystem services. Ecol Econ. 64:297–304. doi:10.1016/j.ecolecon.2007.07.003.
- Yamamoto W, Dewi IA, Ibrahim M. 2007. Effects of silvopastoral areas on milk production at dual-purpose cattle farms at the semi-humid old agricultural frontier in central Nicaragua. Agric Syst. 94:368–375. doi:10.1016/j.agsy.2006.10.011.
- Zhou J, Fu B, Gao G, Lü Y, Liu Y, Lü N, Wang S. 2016. Effects of precipitation and restoration vegetation on soil erosion in a semi-arid environment in the Loess Plateau, China. Catena. 137:1–11. doi:10.1016/j.catena.2015.08.015.
- Zhu X, Liu W, Chen J, Bruijnzeel LA, Mao Z, Yang X, Cardinael R, Meng F-R, Sidle RC, Seitz S, et al. 2020. Reductions in water, soil and nutrient losses and pesticide pollution in agroforestry practices: a review of evidence and processes. Plant Soil. 453:45–86. doi:10.1007/s11104-019-04377-3.