Abstract
Numerous studies have examined how leaf temperature affects photosynthesis, but few have investigated photosynthetic temperature sensitivity during leaf expansion. Here, we show that immature leaves (~18% of final leaf area) of the common New Zealand tree, Weinmannia racemosa L. f. (Cunoniaceae), are less sensitive than mature leaves to rapid increases in leaf temperature (from 25 to 35 °C) as measured by chlorophyll a fluorescence. Relative differences in the tolerance of photosystem II to heat were supported by solute leakage experiments, in which disks from immature leaves incubated at 47 °C lost significantly fewer solutes than did disks from mature leaves. In addition, levels of trienoic (18:3) fatty acids were far lower in immature than in mature leaves (low levels are known to be associated with photosynthetic thermotolerance). The developmental changes in photosynthetic tolerance to heat stress likely correspond to compositional differences of the thylakoid membranes during leaf expansion. This result may be useful in understanding photosynthetic responses to temperature in young leaves which lack functioning stomates, or under extreme conditions when leaf temperatures are high or fluctuate rapidly.
Introduction
Although the effects of leaf temperature on photosynthesis have been extensively studied, most investigations have focused on the responses of fully expanded leaves (Sharkey Citation2005), with only few reports showing how the stage of leaf development affects this interaction (Jiang et al. Citation2006; Karim et al. Citation1999). This is a particularly important omission because young leaves can have distinctively different photosynthetic characteristics from those of mature leaves. In particular, the more immature leaf laminae exhibit far lower rates of photosynthesis as measured by gas exchange (Hughes et al. Citation2007; Snider et al. Citation2009; Woodall et al. Citation1998) and chlorophyll a fluorescence (Choinski et al. Citation2003; Dodd et al. Citation1998). Young leaves also generally have lower chlorophyll contents, higher levels of carotenoids and higher dark respiration rates (Choinski et al. Citation2003; Hughes et al. Citation2007; Snider et al. Citation2009; Woodall et al. Citation1998). They often show a transient flush of anthocyanin pigments (see studies cited in Chalker-Scott Citation1999; Gould Citation2004), reflect more light between 630 and 690 nm (Woodall et al. Citation1998) and typically exhibit chronic photoinhibition (Choinski et al. Citation2003; Dodd et al. Citation1998).
It has recently been shown that immature leaves of Rhus glabra were up to 6 °C warmer than fully expanded leaves from the same plants during periods of high irradiance (Snider et al. Citation2009). This difference was attributable to lower transpiration rates in the younger leaves, which, in the absence of functional stomata, cannot dissipate excess heat via transpirational cooling. Thus, the developmental age of a leaf needs to be considered alongside other factors that influence leaf temperature, including: leaf angle and intensity of incident radiation (Boa & Nilsen Citation1988), stomatal conductance (Radin et al. Citation1994; Matsumoto et al. Citation2000), degree of pubescence (Ehleringer & Mooney Citation1978), exposure/orientation to the wind (Telewski Citation1995) and convective heat exchange (Jordan & Smith Citation1994). Under natural conditions, temperatures of mature leaves often climb substantially higher than that of the surrounding air (Singsaas et al. Citation1999); under high irradiance or extreme environmental conditions, immature leaf temperatures could be even greater than those of mature leaves. Species that show extended periods of leaf expansion or multiple flushes during the growing season may be particularly impacted (Green & Jane Citation1983; Hughes et al. Citation2007; Woodall et al. Citation1998). However, because they maintain the capacity for photosynthetic activity, and do not normally exhibit symptoms of thermal injury under such conditions, there is evidently an effective mechanism by which the chloroplasts in immature leaves tolerate excess heat loads. Indeed, relative to mature leaves, the immature leaves of Rhus glabra have a higher photosynthetic thermal tolerance, as measured by chlorophyll a fluorescence (Snider et al. Citation2010). Enhanced thermal tolerance of immature leaves is likely to be commonplace. However, mechanisms for thermotolerance in developing leaves have not been studied previously.
Here, we compare the thermotolerance of photosystem II (PSII) in expanding and mature leaves of Weinmannia racemosa (Cunoniaceae), one of the most abundant trees in the temperate forests of New Zealand. Weinmannia racemosa was particularly useful for our purpose because it is a broadleaf evergreen that continually produces new leaves throughout the growing season, with the duration of leaf lamina expansion exceeding one month (Green & Jane Citation1983). Recent research on temperature effects on photosynthesis has attempted to mimic field conditions, with experiments involving rapid changes in leaf temperature to induce moderate heat stress (35–38 °C), and observing the effects on gas exchange and/or chlorophyll a fluorescence compared with 25 °C controls (Haldimann & Feller Citation2004 ,Citation2005). Accordingly, we investigated the effect of temperature on chlorophyll a fluorescence parameters in developing leaves using a similar method to test the hypothesis that thermotolerance is greater in developing than in mature leaves at higher temperatures (35–40 °C). In addition, possible mechanisms for thermotolerance in immature leaves were explored by examining solute leakage from heat-treated leaf disks, and the fatty acid composition of membranes.
Materials and methods
Plant material
Young trees of W. racemosa (‘kamahi’) used in this study grew naturally in Otari-Wilton's Bush, a nature reserve in Wellington, New Zealand (41°15′47.68′′S, 174°45′46.32′′E). For one experiment examining the impact of growth conditions on lipid fatty acid content, leaves were also collected from a mountainous (561 m) site ~50 km from Wellington in the Tararua Forest Park (41°06′48.62′′S, 175°13′56.10′′E). Both populations of trees were similar in overall appearance and 1–1.5 m tall. Long-term averages of mean annual precipitation and temperature data for collection sites in Otari-Wilton's Bush and the Tararua Forest Park were 1271 mm year−1 and 12.5 °C and 1966 mm year−1 and 9.5 °C, respectively. Temperature was obtained from interpolation of data from nearby climactic stations using the LENZ (Land Environments of New Zealand) database (www.landcareresearch.co.nz/database/lenz) and rainfall data from www.worldclim.org.
Weinmannia racemosa is a broadleaved evergreen species that exhibits pairs of simple, opposite leaves with leaf material in three distinctive stages: immature expanding (IME), immature expanded and thickening (IM) and mature (M) (). Although the specific time required for leaves to progress from the IME to the M stage was not measured, all stages were continually observed in the leaf canopy during recurrent sampling on the following dates: 6, 12, 17, 22, 26, 28 November 2008; 1, 3, 12, 14 December 2008; 16, 20, 29 January 2009; and 9 February 2009. IME leaves were harvested from the first easily observable node from the apical meristem of the primary shoot and/or lateral branches, were light green in color and <2 cm2 in area, whereas IM leaves were found on the next (second) node, bright green and of similar area (>10 cm2) to mature leaves. M leaves (produced during the current or previous flush) were dark green and thicker than either IME or IM leaves and found at numerous nodes along the stem. Care was taken when selecting leaves for study to choose only those that were minimally shaded either by other leaves or nearby plants. There was no difference in leaf orientation between the leaf stages, all being (more or less) horizontal to incoming sunlight. Leaves were harvested in the field at ~0630 h (before direct sunlight irradiated the leaf surfaces) to minimize the impact of potential photoinhibition, transferred to plastic bags containing moist filter paper and held in a dark container at 20 °C.
Fig. 1 Morphology and anatomy of Weinmannia racemosa leaf stages. Representative leaves from greenhouse-grown plants show in transverse section the anatomical changes occurring during leaf expansion and thickening. Note that mature leaves are approximately twice as thick as immature expanding and/or immature expanded leaves with a more developed cuticular layer and palisade mesophyll tissue.
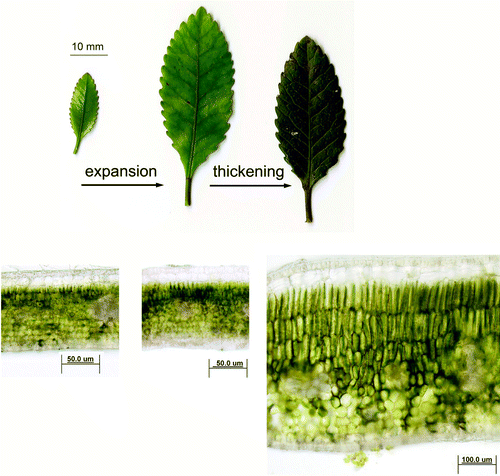
Pigment analyses
Two disks per leaf stage were excised using a 5 mm diameter cork borer, and then extracted in 5 ml of 100% ethanol in the dark for 6 days (Dillenburg et al. Citation1995). Previous studies have shown that chlorophylls isolated from leaf tissue using the same extraction method degraded ~3% after 6 weeks of storage (Knudson et al. Citation1977). Chlorophyll and carotenoid content was determined spectrophotometrically using the absorbances and equations of Lichtenthaler (Citation1987). Leaf areas were estimated by using ImageJ software (http://rsb.info.nih.gov/ij/) on scanned images of harvested leaves.
Leaf spectral measurements
Transmittance (T) of leaf stages over the 450–700 nm waveband was measured using a CI-700 LP Leaf Probe (CID Ltd, Camas, WA, USA) connected to an Ocean Optics portable spectrometer (USB4000, Ocean Optics, Dunedin, FL, USA) fitted with a cosine-corrected receptor. Diffuse reflectance (R) was determined under same conditions from an angle of incidence at 45° to the adaxial surface. A polynomial smoothing function (provided by Sigma Plot 11.0 software) was applied to all raw transmittance and reflectance data; smoothed data was then used to calculate leaf absorbance (A) using the formula:
The fractional absorption (a) of visible light for leaves of each developmental stage was estimated by integrating the area under the appropriate absorption curve.
Microscopy
Fresh transverse sections were cut through the central portions of leaf laminae from each of the three stages. The sections were examined in an Olympus AX70 compound microscope, and photographed using an Olympus DP70 digital camera.
Chlorophyll a fluorescence
Fluorescence measurements were taken using a pulse amplitude modulation fluorometer (Imaging PAM, Maxi-version; Heinz Walz, Effeltrich, Germany) on leaves harvested from four trees growing in the nature reserve. Excitation, actinic and saturating pulses were provided by an array of blue (450 nm) LEDs; fluorescence was imaged using a CCD-camera and quantification of fluorescence parameters was accomplished on the pixels found within a representative area of interest on the leaf image using Imaging Win 2.30 software. Prior to the determination of any fluorescence parameter, leaf segments (~1 cm2 in area) were cut under dim laboratory light, placed on a thermoelectric cooler/heater (described below), adjusted to the desired temperature (usually 25 °C) and then given an additional 10 min of darkness before being subjected to a saturating pulse (2700 µmol m−2 s−1 for 0.8 s) to determine F v/F m [= (F m−F 0)/F m], the maximum quantum yield of PSII. Actinic light at 281 µmol m−2 s−1 supplied by the Imaging PAM was applied for another 20 min (preliminary experiments showed that quantum yield became constant after 15 min of similar illumination at constant temperature), after which F m′ and F were determined, and the following fluorescence parameters calculated using Imaging PAM software: ΦPSII [(F m′ − F)/F m′], actual quantum yield (Genty et al. Citation1989); F v′/F m′, the efficiency of excitation capture by open PSII reaction centers; NPQ [(F m−F m′)/F m′], non-photochemical quenching; q N [(F m−F m′)/(F m−F 0′)], coefficient of non-photochemical quenching and q P [(F m′ − F)/(F m′ − F 0′)], coefficient of photochemical quenching. The imaging PAM lacks a far-red light to re-oxidize the PSII acceptor and so F 0′ was estimated using the method [F 0′ = F 0 (F v/F m+F 0/F m′)] of Oxborough & Baker (Citation1997). Apparent electron transport rates (ETR) were calculated using the equation:
with a (the fractional light absorption of each leaf stage) determined as described above, and f (the fraction of absorbed energy sent to PSII) assumed to be 0.5. The leaf segments were then rapidly heated (as explained below) to 30 °C and held at that temperature for 6 min while saturating pulses were applied at 2-min intervals to monitor the effect of temperature on fluorescence parameters. This procedure was repeated as the segments were heated to 35 and 40 °C. The effect of temperature on ΦPSII was measured as the linear rate of change occurring between the second and sixth minute (allowing 2 min for temperature equilibration); fluorescence parameters reached at the sixth minute at each temperature were used for statistical analyses.
Determination of T c (the critical temperature for PSII thermal stability) was carried out using a modification of the method of Froux et al. (Citation2004). Briefly, leaf segments treated as described above were gradually heated (1.0–1.5 °C min−1) from 25 to 55 °C and F 0 (ground fluorescence yield) was monitored using the measuring light of the Imaging PAM. F 0 and temperature data were plotted and T c estimated as the temperature when F 0 showed a rapid upward inflexion.
Leaf segment temperatures were changed using a 40×44×3.3 mm thermoelectric heater/cooler (All Electronics Corporation, Van Nuys, CA, USA) connected to either a power supply delivering +/− 6 V and 3 A or a 12-V rechargeable battery. The top side of the thermoelectric element rapidly changed temperature (within a range of 10–60°C) with the bottom side of the device held at ambient temperature by an off-the-shelf CPU fan/heat sink combination. The temperature of the abaxial surface of leaf segments were monitored by a fine-wire thermocouple and a hand-held infrared thermometer was used to determine the time required for the adaxial surface to reach the same temperature. There was, depending on leaf thickness, an approximately 1-min delay between the temperature recorded at the abaxis with the surface temperature measured by the infrared thermometer; thus, a 5 °C change in leaf surface temperature occurred (giving time to adjust the voltage to the appropriate value) in ~1.2 min.
Determination of relative injury to membranes
The effect of temperature on membrane stability was estimated using a modification of the method of Blum & Ebercon (Citation1981). Briefly, single leaf disks (5 mm in diameter) were cut from the centers of the appropriate leaf stages, randomized, washed three times with distilled water and then incubated at either 22 or 47 °C for 6 h in 15 ml of distilled water in a capped tube (two disks/tube). Changes in conductivity caused by the leakage of solutes from the leaf disks were periodically measured using a conductivity meter (Model 340; IQ Scientific Instruments, Carlsbad, CA, USA). Relative injury (RI) to the membranes was calculated thus:
where S and C refer to the conductivities of solutions from leaf disks heated to 47 and 22 °C, respectively. Initial conductivities (i) were obtained after a given time of heat treatment (up to 6 h); final conductivities (f) (a measure of total solute leakage potential) were determined after the disks (and solutions) had been frozen overnight and incubated for an additional 10 min at 50°C.
Fatty acid composition
Lipids were extracted from pooled leaf samples (1.6 to 5.0 g fresh weight depending on leaf stage) using the method of Bligh & Dyer (Citation1959). Methylation of fatty acids was conducted using the method of Carreau & Dubacq (Citation1978). Lipid samples (5–16 mg) were dissolved in 0.5 ml toluene containing tricosanoic acid as an internal standard (1.97 mg ml−1). After heating and partitioning into petroleum ether, fatty acid analysis was conducted using a gas chromatograph (HP 5980, Hewlett Packard, Palo Alto, CA, USA) fitted with a BP-20 column (30 m, 0.25 mm I.D., 0.25 µm film). Detection was by flame ionization and peak identification was conducted using ECL values and by comparison with lipid standards. Individual fatty acid classes were expressed as % (w/w) of total fatty acid.
Statistical analyses
As appropriate, one-way and two-way analysis of variance (ANOVA) was performed using Sigma Plot 11.0 software. Two-way ANOVA with difference between means were determined using (as appropriate) the Holm–Sidak or Dunn's methods. Unless otherwise stated, the level of statistical significance was p < 0.05.
Results
Leaf characteristics
Mature (M) leaves from W. racemosa were glabrous, hypostomatic and dorsiventral (). They had characteristic C-3 anatomy, with a thick cuticle, one or two layers of hypodermal collenchyma, up to three layers of palisade mesophyll and eight layers of spongy mesophyll. Substantial development of palisade mesophyll and the cuticular layer occurred only after the leaves had fully expanded and thickened (). The immature expanding (IME) leaves used for comparisons of pigment content and chlorophyll fluorescence measurements were 18% of their final leaf lamina area. Immature leaves (IM) that had completed their lateral expansion of leaf laminae continued to increase in thickness approximately twofold until maturity (; ). Chlorophyll a, b, total chlorophyll and carotenoid contents significantly increased as the leaves both expanded and thickened. The rates of increase, however, were different, with chlorophyll a increasing approximately three times as fast during expansion as it did during thickening, whereas chlorophyll b increased four times faster during expansion compared with thickening. These differences in rates parallel the observation that chlorophyll a/b ratios showed a transient decline during the expansion phase only (). The carotenoid/chlorophyll ratio was 50% higher in IME leaves when compared with either IM or M leaf stages. Predawn F v/F m was highest in M leaves and similarly lower in IM and IME stages ().
Table 1 Characteristics of Weinmannia racemosa leaf stages harvested from trees growing at Otari-Wilton's Bush. Data are mean±SE for 10 replicates (12 replicates for predawn F v/F m). Differences in leaf stage characteristics determined using the Holm–Sidak or Dunn's method as appropriate; means followed by different letters horizontally are statistically different (p<0.05)
Effect of temperature on chlorophyll a fluorescence parameters
The three leaf developmental stages differed significantly in their responses to leaf temperature as measured by various chlorophyll a fluorescence parameters. In general, IME leaves were least affected by changes in leaf temperature, followed by M and IM leaf stages (). Photochemical quantum yield (ΦPSII) of M leaves did not alter significantly when they were warmed from 25 to 35 °C, but was 43% lower at 40 °C when compared with 25 °C (A). Values of ΦPSII for IM leaves decreased significantly at 35 °C (30% lower) and 40 °C (68% lower) when compared with 25 °C. By contrast, IME leaves did not show any decreases in ΦPSII at any temperature examined, and ΦPSII values were observed to be significantly higher at 40 °C when compared with M or IM leaves; M and IM leaves were also significantly different (A). Electron transport rates (ETR) similarly varied with temperature except that at 40 °C IME leaves were not significantly different from M leaves (B). Decreases in ΦPSII or ETR for M and IM leaf stages were associated with a reduction in the efficiency of excitation energy capture by PSII reaction centers (F v′/F m′) (D) and a lowered q P value (the fraction of open PSII reaction centers) (E). Nonphotochemical quenching (NPQ and q N) also increased concurrently, indicating that less electron capture by PSII reaction centers was accompanied by increased thermal dissipation of excess excitation energy (C,F). By contrast, IME leaves did not show any significant temperature-dependent increases in NPQ (C) or q N (F) or decrease in qP (E).
Fig. 2 Effects of rapid changes in leaf temperature on: (A) quantum yield of photosystem II (PSII) electron transport (FPSII); (B) electron transport rate (ETR); (C) nonphotochemical quenching (NPQ); (D) efficiency of electron capture by open PSII reaction centers (F v′/F m′); (E) fraction of open PSII reaction centers (q P) and (F) fraction of closed PSII reaction centers (q N). Chlorophyll fluorescence of leaf segments was monitored after 6 min at a given temperature. Data are means±SE (at least four replicates). Differences were determined using the Holm–Sidak method; means followed by different letters horizontally within a temperature treatment or different numbers of asterisks vertically within a parameter are statistically different at p<0.05.
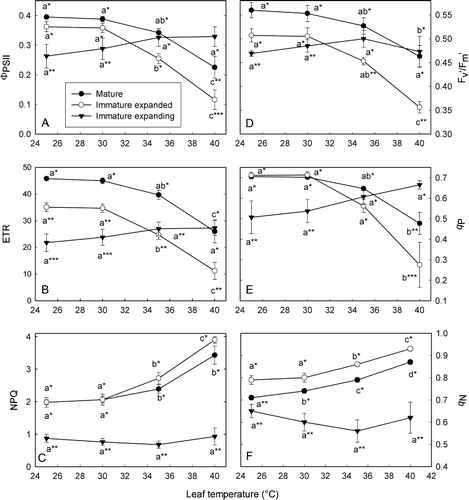
Effect of sampling date on leaf temperature effects on quantum yield
Leaves harvested on 12 December 2008 (A) and 29 January 2009 (B) showed similar responses of ΦPSII to changes in leaf temperature, although the relative ranking of leaf stage sensitivity to temperature stress changed. For example, IM and M leaves harvested in the cooler weather on 12 December were 68 and 43% lower, respectively, at 40 °C when compared to 30 °C, whereas IME leaves did not show reductions in ΦPSII at any temperature tested (A). The IM leaves harvested on 12 December were more sensitive to changes in temperature than M leaves (i.e. the temperature curves do not overlap; A). By contrast, leaves collected on 29 January 2009 showed that IM and M stages declined somewhat faster than on 12 December, losing 72 and 61%, respectively, of their initial activity at 40 °C without any significant difference at 40 °C (B). The overall responses of M and IM leaves to temperature treatments, however, were not significantly different (i.e. the temperature response curves overlapped). IME leaves measured on 29 January 2009 showed 20% less activity at 40 °C when compared with 30 °C, but this difference was not statistically significant (p=0.06; B).
Fig. 3 Effect of rapid alterations in leaf temperature on proportionate changes in quantum yield (FPSII ) for leaf segments sampled on (A) 12 December 2008 and (B) 29 January 2009. Data are means±SE (at least four replicates), expressed as percentage difference from values at 30 °C. Differences were determined using the Holm–Sidak method; means followed by different letters horizontally within a temperature treatment or different numbers of asterisks vertically within a parameter are statistically different at p<0.05.
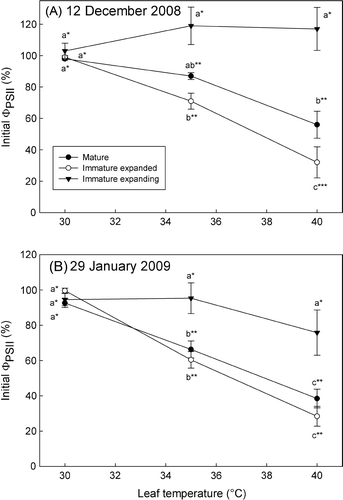
Thermostability of the photosynthetic apparatus
Significant differences were observed in the thermostability of the photosynthetic apparatus as measured by the critical temperature (T c) at which F o rises. IME leaves had a higher T c value than either IM or M stage leaves (). For example, T c of IME was ~7 °C higher than that of IM leaves, and 6 °C higher than that of M leaves. The T c of IM leaves was on average ~1 °C lower than that of M leaves, although this difference was not statistically significant.
Fig. 4 Thermostability of the photosynthetic apparatus at different leaf stages as measured by the critical temperature for ground fluorescence breakup (T c). Data are means±SE for eight replicates. Differences in T c were determined using the Holm–Sidak method; means followed by different letters are statistically different at p<0.05. T c=critical temperature at which F 0 shows an abrupt increase.
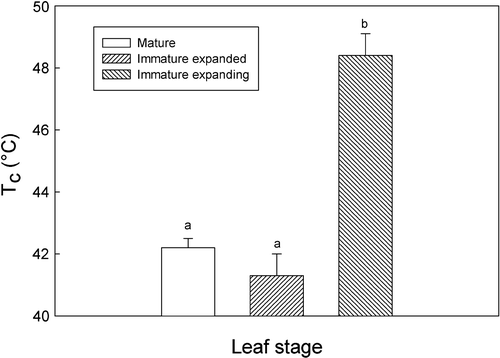
Heat treatment and solute leakage
Continuous heat treatment at 47 °C (compared with 22 °C controls) caused solutes to leak proportionately faster from leaf disks harvested from M or IM leaves compared with disks obtained from IME leaf stages (). Differences in leakage expressed as percent relative injury (RI) were seen after the disks had been incubated for between 1 and 2 h with M, IM and IME showing 52, 44 and 29% RI, respectively. After 6 h of incubation, these values increased to 94, 81 and 55%, respectively.
Fig. 5 Effect of heat treatment on cell membrane stability at different leaf stages. Membrane stability was expressed as relative injury (%) calculated by comparing solute leakage in leaf discs incubated at 22 and 47 °C. Data are means±SE for four replicates. All differences between leaf stages beyond the 60-min treatment were statistically significant at p<0.05. Closed circle, mature; open circle, immature expanded and thickening; triangle, immature expanding.
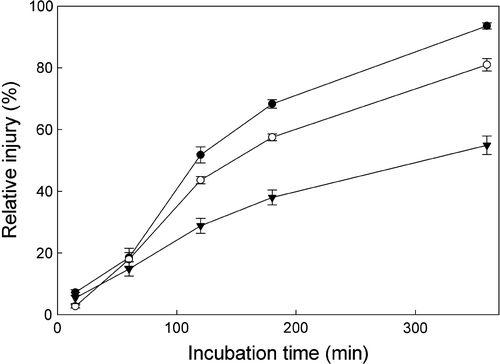
Fatty acid composition
An analysis of fatty acid composition of extracted lipids showed differences between leaf stage and collection site. The main fatty acid classes identified were 16:0, 18:2 and 18:3 with the trienoic fatty acids the most common (with the exception of IME leaves harvested from the Tararua Forest Park). Trienoics (the major fatty acid in chloroplast membrane glycerolipids) were always lowest in IME leaf lipids, followed by IM and M leaves, although the magnitude of the difference varied with collection site. At the warmer, drier Otari-Wilton's Bush site, trienoics in M leaves were 45% higher than in IME leaves, whereas at the cooler, wetter Tararua Forest Park site, the difference was 140% (). All other lipid classes were higher in IM or IME leaves when compared with M ().
Table 2 Fatty acid composition of total lipid extracts of leaf stages harvested from contrasting environments. Each leaf stage extract represents a bulked sample harvested from Otari-Wilton's Bush (OWB) or the Tararua Forest Park (TFP)
Discussion
Immature expanding leaves (IME) of W. racemosa had lower photosynthetic quantum efficiencies as measured by chlorophyll a fluorescence, but greater tolerance to heat stress, than expanded (IM and M) leaves ( and ). This is consistent with preliminary work we did with two other New Zealand tree species, Griselinia littoralis and Nothofagus fusca, and with work done on Rhus glabra, for which immature leaves had higher photosynthetic temperature tolerance than mature leaves when heated above 35°C (Snider et al. Citation2010). Similarly, the immature leaves of maize have been reported to be more resistant to a 90-min 42 °C heat treatment than were nearly developed or fully developed leaves, as measured by F v/F m or FPSII (Karim et al. Citation1999). By contrast, leaves of elm were found to be more sensitive to heat stress when young than when mature, with the developing leaves showing an abrupt increase in F 0 at only 30 °C (Jiang et al. Citation2006). Elm appears to be unusually sensitive to heat stress, compared, for example, with the mature leaves of oak and pea, which showed an appreciable decline in quantum efficiency only when heated to 45 °C (Haldimann & Feller Citation2004 ,Citation2005). In W. racemosa, F 0 did not increase until IME, IM and M leaf temperatures exceeded 48, 42 and 41 °C, respectively (), similar to reports from various species showing a T c>42 °C (Daas et al. Citation2008; Froux et al. Citation2004).
It is not clear why photosynthesis in developing (both IM and IME) leaves showed less sensitivity to moderate heat stress when compared with mature leaves, although membrane thermal stability appeared to play an important role. Consistent with this, our solute leakage data indicated that less membrane damage occurred at high temperatures in IME than in IM or M leaves (). Moreover, IME membranes had lower levels of trienoic fatty acids when compared with either IM or M leaves, particularly when harvested from a colder, wetter climate (). However, our measurements of lipid composition were obtained from a blend of chloroplast, mitochondrial and other cellular membranes, the relative abundances of which would likely change over the course of leaf development. Thus, the observed changes in thermotolerance cannot be attributed exclusively to alterations in plastid lipid composition. Nonetheless, the data were consistent with solute leakage experiments carried out in developing maize leaves (Karim et al. Citation1999) and with reports showing that both tobacco (Murakami et al. Citation2000) and rice (Tang et al. Citation2007) mutants with increased resistance to photosynthetic thermal stress had lower levels of trienoic fatty acids. By contrast, a study of Arabidopsis mutants with a similarly altered lipid composition did not show the same result (Kim & Portis Citation2005). Thus, the thermal resistance of IME W. racemosa leaves may be related to other factors in addition to membrane stability. The observation that the 18:2 lipid class (the precursor to 18:3) was higher in IM or IME leaf stages compared with M leaves () may indicate that the expression of omega-3 fatty acid desaturase, the enzyme responsible for the 18:2 to 18:3 conversion (Murakami et al. Citation2000) was limited during leaf expansion. Another possibility is that IME leaves have higher photorespiration and associated oxygen radical scavenging rates, as has been observed in other young leaves (Polle et al. Citation2001). For example, young leaves of hybrid poplar (Bagard et al. Citation2008) and a heat-resistant Acer rubrum genotype (Weston & Bauerle Citation2007) had higher photorespiration rates and more resistance to heat stress when compared with mature leaves. Other possibilities include the accumulation of heat shock proteins (Heckathorn et al. Citation2002), protective solutes (Park et al. Citation2003) or the short-term synthesis of isoprene (Sharkey Citation2005). The isoprene hypothesis is less likely to explain our results, however, because isoprene production has been shown to be very low during the early stages of leaf expansion (Sharkey et al. Citation2008; Wiberley et al. Citation2005).
Weinmannia racemosa is similar to many other tree species in showing increased pigment content per unit area during leaf expansion and thickening. For example, total chlorophyll content of IME leaves (at ~18% of their final area) increased approximately fivefold when fully expanded and thickened (), similar to green young leaves of Syzygium corynanthum (Woodall et al. Citation1998) and anthocyanic leaves of Corymbia gummifera (Choinski et al. Citation2003). The increases in pigment content observed when comparing IM and M leaves are partly associated with leaf thickening () and development of palisade mesophyll () as well as new chlorophyll synthesis (Hughes et al. Citation2007). The decrease in chlorophyll a/b ratios during leaf expansion (IME compared with IM) has been observed in other species exhibiting green developing leaves (Hughes et al. Citation2007, Manetas et al. Citation2003) and was probably because the chloroplasts in young leaves have underdeveloped thylakoids (Choinski & Wise Citation1999) with higher amounts of PSII relative to PSI (Pettigrew & Vaughn Citation1998). The carotenoid/chlorophyll ratio also decreased during leaf expansion (), consistent with most (Barker et al. Citation1997; Hughes et al. Citation2007; Krause et al. Citation1995), although not all previous studies (Manetas et al. Citation2002). The role of carotenoids in photoprotection through the xanthophyll cycle has been well documented (Demmig-Adams & Adams Citation1998) and so the decreases accompanying leaf expansion are typically associated with increased reflection of photoinhibitory light (Hughes et al. Citation2007) or the development of some structural photoprotective feature (e.g. a thick cuticular layer) (Choinski & Wise Citation1999). Weinmannia racemosa is likely to be in the latter category because M leaves reflected less visible light than either IM or IME leaves (data not shown), but had thicker cuticles and more developed epidermal layers (). The higher carotenoid/chlorophyll ratio in IME leaves indicated that young leaves also had a greater capacity for the harmless dissipation of light energy through the xanthophyll cycle when compared with IM or M leaves (Demmig-Adams & Adams Citation1998; Dodd et al. Citation1998; Havaux & Tardy Citation1996).
Finally, enhanced thermotolerance of young leaves may have ecological importance, particularly in species with extended periods of leaf expansion and/or those that undergo continuous or multiple flushes during the growing season. The literature does not contain extensive information about these points, although for a fast-growing species of Syzygium, full leaf expansion was reached within 7–10 days after bud break (Woodall et al. Citation1998), whereas other species (including W. racemosa) required 20–35 days to fully expand (Choinski & Wise Citation1999; Green & Jane Citation1983; Woodall et al. Citation1998). Many woody species from temperate climates also show multiple flushes during the growing season, including: Syzygium sp. (Woodall et al. Citation1998), Acer rubrum, Cercis canadensis, Liquidambar styraciflua (Hughes et al. Citation2007) and Sarcopoterium spinosum (Orshan Citation1964). These periods of leaf expansion are likely sufficient to affect photosynthetic thermal optima as it has been reported that only short exposures (as little as five days) were required to alter chlorophyll a fluorescence parameters (e.g. T c) when saplings were transferred to increasingly higher growth temperatures (Daas et al. Citation2008; Froux et al. Citation2004). We also observed that the sensitivity of ΦPSII in W. racemosa leaf stages to short-term heat stress was different depending on when in the growing season it was measured (), suggesting that variations in air temperature (or some other environmental variable) on the sampling dates was a factor. For example, juvenile leaves of Rhus glabra (a fast-growing tree with very high stomatal conductance) had significantly higher temperatures than mature leaves (as much as 6 °C) during periods of high irradiance, and this difference was greater earlier in the growing season when air temperatures were cooler and became much less later on when air temperatures were higher (Snider et al. Citation2009). In summary, we conclude that the elevated temperatures experienced by immature leaves as a consequence of reduced transpiration rates (Snider et al. Citation2009) may lead (through temperature-induced plasticity) to developmental differences in photosynthetic thermal optima and possibly enhanced survival during periods of heat stress.
Acknowledgements
We thank Dr Lesley Milicich, Neville Higgison, Cameron Jack and Craig Doney for technical help, Dr William Slaton for designing and constructing the leaf heater/cooler apparatus, Dr Stephen Hartley for providing climactic data for the leaf collection sites and Dr Andrew MacKenzie for lipid analyses. This project was supported by the National Research Initiative Competitive Grant no. 2008-35100-19221 from the USDA National Institute of Food and Agriculture, and the University of Central Arkansas Sabbatical leave fund.
References
- Bagard , M , Le Thiec , D , Delacote , E , Hasenfratz-Sauder , M-P , Bonvoy , J , Gérard , J , Dizengremel , P and Jolivet , Y . 2008 . Ozone-induced changes in photosynthesis and photorespiration of hybrid poplar in relation to the developmental stages of the leaves . Physiologia Plantarum , 134 : 559 – 574 .
- Barker , DH , Seaton , GGR and Robinson , SA . 1997 . Internal and external photoprotection of developing leaves of the CAM plant Cotyledon orbiculata . Plant, Cell & Environment , 20 : 617 – 624 .
- Bligh , EG and Dyer , WJ . 1959 . A rapid method of total lipid extraction and purification . Canadian Journal of Biochemistry and Physiology , 37 : 911 – 917 .
- Blum , A and Ebercon , A . 1981 . Cell membrane stability as a measure of drought and heat tolerance in wheat . Crop Science , 21 : 43 – 47 .
- Boa , Y and Nilsen , ET . 1988 . The ecophysiological significance of leaf movements in Rhododendron maximum . Ecology , 69 : 1578 – 1587 .
- Carreau , JP and Dubacq , JP . 1978 . Adaptation of macro-scale method to the micro-scale for the fatty acid methyl transesterification of biological lipid extracts . Journal of Chromatography , 151 : 384 – 390 .
- Chalker-Scott , L . 1999 . Environmental significance of anthocyanins in plant stress responses . Photochemistry and Photobiology , 70 : 1 – 9 .
- Choinski , JS Jr , Ralph , P and Eamus , D . 2003 . Changes in photosynthesis during leaf expansion in Corymbia gummifera . Australian Journal of Botany , 51 : 111 – 118 .
- Choinski , JS Jr and Wise , RR . 1999 . Leaf growth and development in relation to gas exchange in Quercus marilandica . Journal of Plant Physiology , 154 : 302 – 309 .
- Daas , C , Montpied , P , Hauchi , B and Dryer , E . 2008 . Responses of photosynthesis to high temperatures in oak saplings assessed by chlorophyll-a fluorescence: inter-specific diversity and temperature-induced plasticity . Annals of Forest Science , 65 : 305
- Demmig-Adams , B and Adams , WW . 1998 . Survey of thermal energy dissipation and pigment composition in sun and shade leaves . Plant and Cell Physiology , 39 : 474 – 482 .
- Dillenburg , LR , Sullivan , JH and Teramura , AH . 1995 . Leaf expansion and development of photosynthetic capacity and pigments in Liquidambar styraciflua (Hammamelidaceae) . American Journal of Botany , 82 : 433 – 440 .
- Dodd , IC , Critchley , C , Woodall , GS and Stewart , GR . 1998 . Photoinhibition in different coloured juvenile leaves of Syzygium species . Journal of Experimental Botany , 49 : 1437 – 1445 .
- Ehleringer , JR and Mooney , HA . 1978 . Leaf hairs: effects on physiological activity and adaptive value to a desert shrub . Oecologia , 37 : 183 – 201 .
- Froux , F , Ducrey , M , Epron , D and Dreyer , E . 2004 . Seasonal variations and acclimation potential of the thermostability of photochemistry in four Mediterranean conifers . Annals of Forest Science , 61 : 235 – 241 .
- Genty , B , Briantais , JM and Baker , NR . 1989 . The relationship between the quantum yield of photosynthetic electron transport and quenching of chlorophyll fluorescence . Biochimica et Biophysica Acta , 990 : 87 – 92 .
- Gould , K . 2004 . Nature's Swiss army knife: the diverse protective roles of anthocyanins in leaves . Journal of Biomedicine and Biotechnology , 5 : 314 – 320 .
- Green , TGA and Jane , GT . 1983 . Changes in osmotic potential during bud break and leaf development of Nothofagus menziesii, Weinmannia racemosa, Quintinia acutifolia, and Ixerba brexioides . New Zealand Journal of Botany , 21 : 391 – 395 .
- Haldimann , P and Feller , U . 2004 . Inhibition of photosynthesis by high leaf temperature in oak (Quercus pubescens L.) leaves grown under natural conditions closely correlates with a reversible heat-dependent reduction of the activation state of ribulose-1,5-bisphosphate carboxylase/oxygenase . Plant, Cell & Environment , 27 : 1169 – 1183 .
- Haldimann , P and Feller , U . 2005 . Growth at moderately elevated temperature alters the physiological response of the photosynthetic apparatus to heat stress in pea (Pisum sativum L.) leaves . Plant, Cell & Environment , 28 : 302 – 317 .
- Havaux , M and Tardy , F . 1996 . Temperature-dependent adjustment of the thermal stability of photosystem II in vivo: possible involvement of xanthophyll-cycle pigments . Planta , 186 : 324 – 333 .
- Heckathorn , SA , Ryan , SL , Baylis , JA , Wang , D , Hamilton , EW , Cundiff , L and Luthe , DS . 2002 . In vivo evidence from an Agrostis stolonifera selection genotype that chloroplast small heat-shock proteins can protect photosystem II during heat stress . Functional Plant Biology , 29 : 933 – 944 .
- Hughes , NM , Morley , CB and Smith , WK . 2007 . Coordination of anthocyanin decline and photosynthetic maturation in juvenile leaves of three deciduous tree species . New Phytologist , 175 : 675 – 685 .
- Jiang , C-D , Jiang , G-M , Wang , X , Li , L-H , Biswas , DK and Li , Y-G . 2006 . Increased photosynthetic activities and thermostability of photosystem II with leaf development of elm seedlings (Ulmus pumila) probed by the fast fluorescence rise OJIP . Environmental and Experimental Botany , 58 : 261 – 268 .
- Jordan , DN and Smith , WK . 1994 . Energy balance analysis of nighttime leaf temperatures and frost formation in a subalpine environment . Agricultural and Forest Meteorology , 71 : 359 – 372 .
- Karim , MA , Fracheboud , Y and Stamp , P . 1999 . Photosynthetic activity of developing leaves of Zea mays is less affected by heat stress than that of developed leaves . Physiologia Plantarum , 105 : 685 – 693 .
- Kim , K and Portis , AR Jr . 2005 . Temperature dependence of photosynthesis in Arabidopsis plants with modifications in rubisco activase and membrane fluidity . Plant and Cell Physiology , 46 : 522 – 530 .
- Knudson , LL , Tibbits , TW and Edwards , GE . 1977 . Measurement of ozone injury by determination of leaf chlorophyll concentration . Plant Physiology , 60 : 606 – 608 .
- Krause , GH , Virgo , A and Winter , K . 1995 . High susceptibility to photoinhibition of young leaves of tropical forest trees . Planta , 197 : 583 – 591 .
- Lichtenthaler , HK . 1987 . Chlorophylls and carotenoids: pigments of photosynthetic biomembranes . Methods in Enzymology , 148 : 350 – 382 .
- Manetas , Y , Drinia , A and Petropoulou , Y . 2002 . High contents of anthocyanins in young leaves are correlated with low pools of xanthophyll cycle components and low risk of photoinhibition . Photosynthetica , 40 : 349 – 354 .
- Manetas , Y , Petropoulou , Y , Psaras , GK and Drinia , A . 2003 . Exposed red (anthocyanic) leaves of Quercus coccifera display shade characteristics . Functional Plant Biology , 30 : 265 – 270 .
- Matsumoto , J , Muraoka , H and Washitani , I . 2000 . Ecophysiological mechanisms used by Aster kantoensis, an endangered species, to high light and heat stresses of its gravelly floodplain habitat . Annals of Botany , 86 : 777 – 785 .
- Murakami , Y , Tsuyama , M , Kobayashi , Y , Kodama , H and Iba , K . 2000 . Trienoic fatty acids and plant tolerance of high temperature . Science , 287 : 476 – 477 .
- Orshan , G . 1964 . “ Seasonal dimorphism of desert and Mediterranean chamaephytes and its significance as a factor in their water economy ” . In The water relations of plants , Edited by: Rutter , AJ and Whitehead , FH . 206 – 222 . Edinburgh, Blackwell .
- Oxborough , K and Baker , NR . 1997 . Resolving chlorophyll a fluorescence efficiency into photochemical and non-photochemical components – calculation of q P and Fv′/Fm′ without measuring F 0 . Photosynthesis Research , 54 : 135 – 142 .
- Park , SH , Jun , SS , An , GH , Hong , YN and Park , MC . 2003 . A comparative study on the protective role of trehalose and LEA proteins against abiotic stresses in transgenic Chinese cabbage (Brassica campestris) overexpressing CaLEA or otsA . Journal of Plant Biology , 46 : 277 – 286 .
- Pettigrew , WT and Vaughn , KC . 1998 . Physiological, structural and immunological characterization of leaf and chloroplast development in cotton . Protoplasma , 202 : 23 – 37 .
- Polle , A , Schwanz , P and Rudolf , C . 2001 . Developmental and seasonal changes of stress responsiveness in beech leaves (Fagus sylvatica L.) . Plant, Cell & Environment , 24 : 821 – 829 .
- Radin , JW , Lu , Z , Percy , RG and Zeiger , E . 1994 . Genetic variability for stomatal conductance in Pima cotton and its relation to improvements of heat adaptation . Proceedings of the National Acadamy of Science (USA) , 91 : 7217 – 7221 .
- Sharkey , TD . 2005 . Effects of moderate heat stress on photosynthesis: importance of thylakoid reactions, rubisco deactivation, reactive oxygen species, and thermotolerance provided by isoprene . Plant, Cell & Environment , 28 : 269 – 277 .
- Sharkey , TD , Wiberley , AE and Donohue , AR . 2008 . Isoprene emission from plants: why and how . Annals of Botany , 101 : 5 – 18 .
- Singsaas , EL , LaPorte , MM , Shi , J-Z , Monson , RK , Bowling , DR , Johnson , K , Lerdau , M , Jasentuliytana , A and Sharkey , TD . 1999 . Kinetics of leaf temperature fluctuation affect isoprene emission from red oak (Quercus rubra) leaves . Tree Physiology , 19 : 917 – 924 .
- Snider JS , Choinski JS Jr , Slaton W 2010 . Juvenile leaves of Rhus glabra have higher photosynthetic thermal tolerance than mature leaves . Botany 88 : 286 289 .
- Snider JS , Choinski JS Jr , Wise RR 2009 . Juvenile Rhus glabra leaves have higher temperatures and lower gas exchange rates than mature leaves in the field when compared during periods of high irradiance . Journal of Plant Physiology 166 : 686 696 .
- Tang , Y , Chen , M , Xu , Y and Kuang , T . 2007 . Changes in thermostability of photosystem II and leaf lipid composition of a rice mutant with deficiency of light-harvesting chlorophyll a/b protein complexes . Journal of Integrative Plant Biology , 49 : 515 – 522 .
- Telewski , FW . 1995 . “ Wind-induced physiological and developmental responses in trees ” . In Wind and trees , Edited by: Coutts , MP and Grace , J . 237 – 263 . Cambridge : Cambridge University Press .
- Weston , DJ and Bauerle , WL . 2007 . Inhibition and acclimation of C-3 photosynthesis to moderate heat: a perspective from thermally contrasting genotypes of Acer rubrum (red maple) . Tree Physiology , 27 : 1083 – 1092 .
- Wiberley , AE , Linskey , AR , Falbel , TG and Sharkey , TD . 2005 . Development of the capacity for isoprene emission in kudzu . Plant, Cell & Environment , 28 : 898 – 905 .
- Woodall , GS , Dodd , IC and Stewart , GR . 1998 . Contrasting leaf development within the genus Syzygium . Journal of Experimental Botany , 49 : 79 – 87 .