Abstract
Photosystem II (PSII) is subject to light-induced damage and continuously undergoes repair to restore the damaged photosystems. During repair of PSII, the Psb27 protein interacts with the CP43 subunit of PSII. Two Psb27-like proteins (Psb27-H1 and Psb27-H2) are involved in the repair of PSII in the higher plant Arabidopsis thaliana. Here, we present evidence that duplication and divergence of Psb27 occurred in the green algal lineage and that the Psb27-H2 protein shares c. 30% sequence identity with Psb27-H1. Structural modelling of Arabidopsis thaliana Psb27-H1 and Psb27-H2 indicate that the two proteins have different distributions of surface charge. We suggest that the green algal lineage Psb27-H2 protein occupies a different binding niche than does Psb27-H1.
Introduction
Photosystem II (PSII) is the enzyme responsible for light-driven water oxidation and plastoquinone reduction. The PSII complexes within green algae and higher plants (hereafter viridiplantae, Leliaert et al. Citation2012) and red algae are of cyanobacterial origin (Larkum et al. Citation2007; Keeling Citation2013). As such, the core components of PSII including the reaction centre proteins D1 and D2 and the chlorophyll a-binding proteins CP43 and CP47 are highly conserved between cyanobacteria and photosynthetic eukaryotes (Eaton-Rye & Putnam-Evans Citation2005; Nixon et al. Citation2005).
Photosynthetic water oxidation is catalysed by the manganese-calcium (Mn4CaO5) cluster of the oxygen-evolving complex (OEC). Both the D1 and the CP43 proteins provide ligands to the Mn4CaO5 cluster and are essential for water oxidation (Umena et al. Citation2011). The OEC is located on the lumenal side of PSII and several proteins that bind to the lumenal side of PSII act to stabilize the Mn4CaO5 cluster. The most highly conserved of these is the PsbO protein; PsbO is found in cyanobacteria and in all algal lineages (Roose et al. Citation2007). The stoichiometry of PsbO to PSII in cyanobacteria and red algae is 1:1 (Umena et al. Citation2011; Krupnik et al. Citation2013), whereas in higher plants there is evidence to suggest that two PsbO proteins bind per reaction centre (Xu & Bricker Citation1992; Popelkova et al. Citation2002a,Citationb). A key difference between viridiplantae PsbO proteins and cyanobacterial PsbO proteins is the presence of an N-terminal extension on viridiplantae PsbO proteins (Eaton-Rye & Murata Citation1989; Popelkova et al. Citation2002a,Citationb). In cyanobacteria and red algae, a ‘PsbQ-like’ protein, PsbU and the PsbV protein join PsbO on the lumenal side of PSII (Bricker et al. Citation2012) but in viridiplantae, PsbU and PsbV are absent and PsbQ and PsbP, together with the putative second copy of PsbO, appear to fill the role of these proteins. A fourth polypeptide, PsbR, also joins PsbO, PsbQ and PsbP in viridiplantae PSII. At present the localization and stoichiometry of PsbR is not well understood (Bricker et al. Citation2012).
PSII is subject to light-induced damage and damaged photosystems are repaired (Vass Citation2011; Tyystjärvi Citation2013). Both de novo biogenesis and the repair cycle of PSII following photodamage involve a large number of proteins (Nixon et al. Citation2010; Komenda et al. Citation2012b; Shi et al. Citation2012; Nickelsen & Rengstl Citation2013). One of the proteins that interacts specifically with the lumenal side of inactive PSII complexes is Psb27 (Roose & Pakrasi Citation2004; Nowaczyk et al. Citation2006). The complexes with which Psb27 interacts are depleted of the Mn4CaO5 cluster and lack the PsbO, PsbQ-like, PsbU and PsbV proteins (Nowaczyk et al. Citation2006; Mamedov et al. Citation2007; Liu et al. Citation2011a). In cyanobacteria, the binding site of Psb27 has been located on the CP43 protein using both chemical cross-linking and two-dimensional blue native SDS–PAGE (Liu et al. Citation2011b; Komenda et al. Citation2012a). The Psb27 protein is a four-helical bundle with the helices arranged in a right-handed up–down up–down topology (Cormann et al. Citation2009; Mabbitt et al. Citation2009; Michoux et al. Citation2012). Cross-linking and solvent-protection data suggest that the loop between helices 2 and 3, and the fourth helix of Psb27 interact with CP43 (Liu et al. Citation2011b, Citation2013).
Two paralogues of Psb27 have been identified in Arabidopsis thaliana (Chen et al. Citation2006; Wei et al. Citation2010). The first protein identified as a Psb27 orthologue (encoded by At1g03600) is more similar in sequence to Synechocystis sp. PCC 6803 Psb27 (33% identity) than is the second protein LPA19 (encoded by At1g05385; 26% identity) (Wei et al. Citation2010; Fagerlund & Eaton-Rye Citation2011). The A. thaliana Psb27 and LPA19 proteins are hereafter referred to as Psb27-H1 (inactivated in psb27 mutant plants) and Psb27-H2 (inactivated in lpa19 mutants), respectively. A. thaliana psb27 and lpa19 mutants were phenotypically different. Although psb27 mutants were susceptible to photodamage, their rate of growth was unimpaired (Chen et al. Citation2006). By comparison, lpa19 mutants have a greatly reduced rate of growth even under low-light conditions. In the lpa19 mutant, the rate of D1 processing from its precursor (pD1) was reduced compared with the rate in wild type (Wei et al. Citation2010). Furthermore, there is some evidence that the binding site of A. thaliana Psb27-H2 is different to that of cyanobacterial Psb27-H1: in yeast two-hybrid assays, the A. thaliana Psb27-H2 protein interacted with both mature D1 and pD1 (Wei et al. Citation2010).
Whereas the Psb27-H1 protein appears to have originated in cyanobacteria and has been retained in viridiplantae (Chen et al. Citation2006), the origin and phylogenetic distribution of the Psb27-H2 protein have not been thoroughly examined. To address this question, we have constructed multiple sequence alignments and phylogenetic trees of Psb27-H1 and Psb27-H2 protein sequences from cyanobacteria, brown algae, red algae, green algae, diatoms and higher plants.
Materials and methods
Multiple sequence alignment
Amino acid sequences for Psb27-H1 and Psb27-H2 were found by a BLAST (Altschul et al. Citation1990) search of the National Center for Biotechnological Information (NCBI) database using the Synechocystis sp. PCC 6803 Psb27 sequence as the query, followed by a second search using A. thaliana Psb27-H2 (Wei et al. Citation2010). Sequences were aligned using the multiple sequence comparison by the log-expectation (MUSCLE) algorithm (Edgar Citation2004). Chloroplast import pathways and signal sequences differ greatly from the thylakoid lumen import pathway in cyanobacteria (Gutensohn et al. Citation2006; Shi & Theg Citation2013). All Psb27 sequences were trimmed so that Tyr11 was the first residue, to ensure that only informative regions of sequence were included in the alignment. Multiple sequence alignment figures were prepared using TeXshade (Beitz Citation2000).
Species and sequences examined
The Psb27 sequences available in the NCBI database from cyanobacteria and higher plants greatly outnumber those from other organisms. For cyanobacteria, representative Psb27 sequences were chosen from phylogenetically diverse species (Gupta & Mathews Citation2010). For viridiplantae land plants, representative Psb27-H1 and Psb27-H2 proteins from an angiosperm, bryophyte and gymnosperm were chosen. For organisms other than cyanobacteria and land plants, all sequences with an expect value (E) of < 1 × 10−5 were included.
Amino acid sequences for Psb27 were obtained for the following species (accession codes in brackets): Aureococcus anophagefferens (EGB06728.1), Acaryochloris marina MBIC 11017 (ABW29403.1), Arabidopsis thaliana (Q9LR64 and NM_100418.2), Chlamydomonas reinhardtii (XP_001700736.1 and XP_001701619.1), Chlorella variabilis (EFN58701.1 and EFN58701.1), Coccomyxa subellipsoidea C−169 (EIE25666.1), Cyanidioschyzon merolae strain 10D (BAM80544.1), Ectocarpus siliculosus (CBN76956.1), Emiliania huxleyi CCMP 1516 (EOD15678.1), Galdieria sulphuraria (EME228982.1), Guillardia theta CCMP 2712 (EKX52014.1), Micromonas pusilla CCMP 1545 (XP_003058671.1 and XP_003062962.1), Micromonas RCC 299 (XP_002508067.1 and XP_002508382.1), Nostoc sp. PCC 7120 (NP_485301.1), Ostreococcus lucimarinus CCE 9901 (XP_001419197.1), Ostreococcus tauri (XP_003078942.1), Paulinella chromatophora (YP_002049501.1), Phaeodactylum tricornutum CCAP 1055/1 (XP_002177207.1), Physcomitrella patens (XP_001770919.1 and XP_001776869.1), Picea sitchensis (ABK23991.1 and ABK23129.1), Prochlorococcus marinus CCMP 1986 (NP_892625.1), Prochlorococcus marinus CCMP 1375 (NP_874900.1), Synechocystis sp. PCC 6803 (NP_441782.1), Thermosynechococcus elongatus BP−1 (NP_683253.1), Thalassiosira oceanica CCMP 1005 (EJK47273.1), Thalassiosira pseudonana CCMP 1335 (XP_002288234.1), Trichodesmium erythraeum IMS101(YP_721473.1) and Volvox carteri (EFJ42752.1 and XP_002948422.1).
Phylogenetic tree
Unrooted maximum-likelihood phylograms were estimated using the Phylogeny Inference Package (PHYLIP version 3.695; Felsenstein Citation2013). Analyses were run allowing global rearrangements using the Jones-Taylor-Thornton probability model of amino acid change (Jones et al. Citation1992).
Modelling of Arabidopsis thaliana Psb27 proteins
Models of A. thaliana Psb27-H1 and Psb27-H2 were generated using the SWISS-MODEL server (Schwede et al. Citation2003; Arnold et al. Citation2006). The solution structure of Synechocystis sp. PCC 6803 Psb27 (Mabbitt et al. Citation2009) was used as the template structure. The A. thaliana Psb27-H1 and Psb27-H2 protein sequences submitted to the SWISS-MODEL server were trimmed so that Tyr11 was the first residue.
Results and discussion
An unrooted maximum-likelihood phylogram was constructed from a multiple sequence alignment of Psb27-H1 and Psb27-H2 sequences (). The Psb27 sequences present in red algae are all more similar to Psb27-H1 than to Psb27-H2. Viridiplantae genomes encode both Psb27-H1 and Psb27-H2 proteins. The absence of Psb27-H2-like sequences in cyanobacteria and red algae suggests that Psb27-H2 is specific to the green algal lineage ().
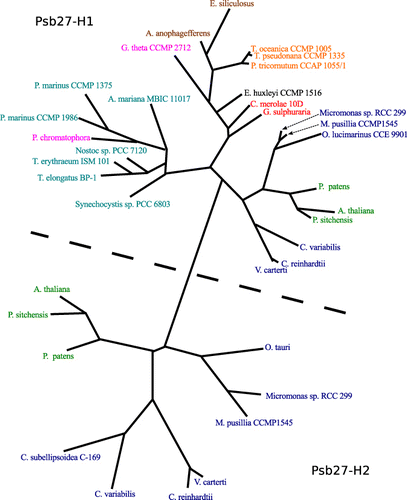
The structures of Synechocystis sp. PCC 6803 and Thermosynechococcus elongatus Psb27-H1 proteins have been solved and the structures are highly similar (Mabbitt et al. Citation2009; Michoux et al. Citation2012). A number of aromatic residues are present in the hydrophobic core of Psb27-H1, these residues appear to be important for the overall fold of Psb27-H1 (Mabbitt et al. Citation2009; Michoux et al. Citation2012). Several of these aromatic residues are highly conserved in both Psb27-H1 and Psb27-H2. These conserved residues are located at positions 11, 53, 65, 78 and 79 (–). An aspartic acid residue located at position 14 of Synechocystis sp. PCC 6803 Psb27-H1 acts to stabilize the structure of Psb27-H1 by hydrogen bonding with the N-terminal tail of Psb27 (Mabbitt et al. Citation2013). This aspartic acid residue is highly conserved amongst Psb27-H1 proteins, but absent from Psb27-H2 proteins (–). A Pro–Φ–Pro motif (where Φ is Ile, Leu or Val) has been identified in Synechocystis sp. PCC 6803 Psb27-H1 (Mabbitt et al. Citation2009). There is evidence to suggest that the Pro–Φ–Pro motif stabilizes the tertiary structure of Psb27-H1 (Mabbitt et al. Citation2013). The Pro–Φ–Pro motif is present in Psb27-H1 polypeptides from phylogenetically diverse organisms (positions 86–88, ), whereas only the central Φ residue of this motif remains highly conserved in the Psb27-H2 proteins (position 88, ). Interestingly, the conservation of the aromatic residues in the hydrophobic core of Psb27-H1 and Psb27-H2 suggests that the proteins retain similar folds; however, the loss of Asp14 and the Pro–Φ–Pro motif suggests that the amino terminus and third loop of Psb27-H2 may be structurally different from those of Psb27-H1.
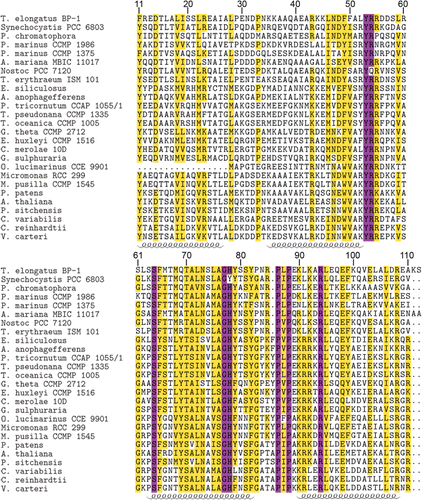
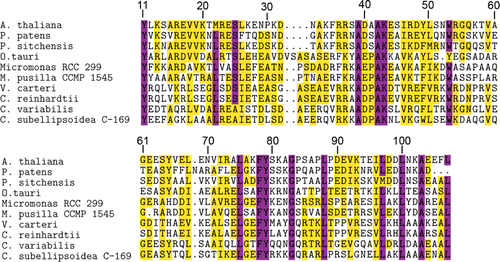
Because structurally important residues are conserved between Synechocystis sp. PCC 6803 Psb27 and both A. thaliana Psb27-H1 and Psb27-H2, we modelled the tertiary structures of the A. thaliana proteins using Synechocystis sp. PCC 6803 Psb27 as the template. The quality of the modelled structures was assessed via the QMEAN Z-score. This score gives an indication of how native-like the modelled protein was compared to a database of high-resolution protein structures (Benkert et al. Citation2011). The QMEAN Z-score for both the Psb27-H1 and Psb27-H2 models was within 1 standard deviation of that observed for the reference dataset (Table S1). Similarly, the QMEAN Z-score for the 2KMF solution NMR structure of Synechocystis sp. PCC 6803 Psb27 was within 1 standard deviation of that observed for the reference dataset (Table S1). The most well modelled regions of both Psb27-H1 and Psb27-H2 were the four helices. The loop between helices 2 and 3 was the least reliable region of both models (Fig. S1).
The A. thaliana Psb27-H1 and Psb27-H2 models retain the right-handed up-down up-down fold of Synechocystis sp. PCC 6803 Psb27. The conserved aromatic residues at positions 11, 53, 65, 78 and 79 occupy equivalent positions in all three structures (). The distribution of surface charge on helices 3 and 4 is markedly different between the Psb27-H1 proteins and the Psb27-H2 protein (). Much of this difference is due to the presence of acidic residues on helix 4 of Psb27-H2 (Glu90, Glu94, Asp97, Asp98, Glu103, Glu104) (–).
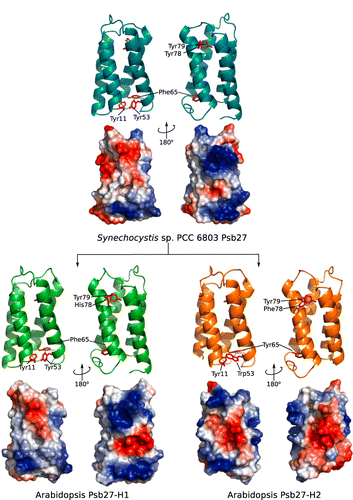
It has been noted that helix 3 of Synechocystis sp. PCC 6803 Psb27-H1 is devoid of acidic or basic residues (Mabbitt et al. Citation2009). This is also the case for Psb27-H1 proteins from photosynthetic eukaryotes (). The highly conserved residues on helix 3 of Psb27-H1 are: Tyr69, Thr70, Ala71, Asn73, Ala76 and Gly77. The equivalent positions on Psb27-H2 do not share the same pattern as Psb27-H1; in addition, both acidic and basic residues are found in this region of the Psb27-H2 sequence (). Several highly conserved acidic and basic residues are found on helix 4 of Psb27-H1: Lys90, Lys92, Arg94, Glu98, Glu103 and Arg108. The equivalent positions on Psb27-H2 are either poorly conserved, retained their charge (position 92) or have reversed their charge (position 90) (–). Given the poor sequence conservation of helix 4 between Psb27-H1 and Psb27-H2 it is unlikely that Psb27-H1 and Psb27-H2 occupy the same binding niche.
Conclusions
The complement of extrinsic proteins in viridiplantae is different from that in cyanobacteria and red algae (Roose et al. Citation2007; Bricker et al. Citation2012). The appearance of the Psb27-H2 protein in the viridiplantae lineage coincides with the loss of the PsbU and PsbV proteins, and changes in the PsbO protein. At present, the function of Psb27-H1 and Psb27-H2 in green algae is unknown. In the higher plant A. thaliana the Psb27-H2 protein had an effect on the rate of D1 processing and PSII accumulation and it was suggested that Psb27-H2 binds to the D1 protein (Wei et al. Citation2010). In cyanobacteria, Psb27-H1 binds to the large lumenal loop of CP43 (Liu et al. Citation2011b, Citation2013; Komenda et al. Citation2012a). We suggest that in viridiplantae, Psb27-H1 retains its association with CP43 and that the Psb27-H2 binds at the interface between the D1 protein and CP43.
Supplementary file
Supplementary file 1: Duplication and divergence of the Psb27 subunit of Photosystem II in the green algal lineage.
Duplication and divergence of the Psb27 subunit of Photosystem II in the green algal lineage.
Download MS Word (417.1 KB)Acknowledgements
PDM was supported by an Otago University postgraduate scholarship. This research was partially supported by a Marsden grant 08-UOO-043 to JJE-R.
References
- Altschul SF, Gish W, Miller W, Myers EW, Lipman DJ 1990. Basic local alignment search tool. Journal of Molecular Biology 215: 403–410.
- Arnold K, Bordoli L, Kopp J, Schwede T 2006. The SWISS-MODEL workspace: a web-based environment for protein structure homology modelling. Bioinformatics 22: 195–201. 10.1093/bioinformatics/bti770
- Beitz E 2000. TeXshade: shading and labeling of multiple sequence alignments using LaTeX2e. Bioinformatics 16: 135–139. 10.1093/bioinformatics/16.2.135
- Benkert P, Biasini M, Schwede T 2011. Towards the estimation of the absolute quality of individual protein structure models. Bioinformatics 27: 343–350. 10.1093/bioinformatics/btq662
- Bricker TM, Roose JL, Fagerlund RD, Frankel LK, Eaton-Rye JJ 2012. The extrinsic proteins of Photosystem II. Biochimica et Biophysica Acta 1817: 121–142. 10.1016/j.bbabio.2011.07.006
- Chen H, Zhang D, Guo J, Wu H, Jin M, Lu Q et al. 2006. A Psb27 homologue in Arabidopsis thaliana is required for efficient repair of photodamaged Photosystem II. Plant Molecular Biology 61: 567–575. 10.1007/s11103-006-0031-x
- Cormann KU, Bangert J-A, Ikeuchi M, Rögner M, Stoll R, Nowaczyk MM 2009. Structure of Psb27 in solution: implications for transient binding during biogenesis and repair. Biochemistry 48: 8768–8770. 10.1021/bi9012726
- Curtis BA, Tanifuji G, Burki F, Gruber A, Irimia M, Maruyama S et al. 2012. Algal genomes reveal evolutionary mosaicism and the fate of nucleomorphs. Nature 492: 59–65. 10.1038/nature11681
- DeLano WL 2002. The PyMol Molecular Graphics System. Palo Alto, CA, Delano Scientific.
- Eaton-Rye JJ, Murata N 1989. Evidence that the amino-terminus of the 33 kDa extrinsic protein is required for binding to the Photosystem II complex. Biochimica et Biophysica Acta 977: 219–226. 10.1016/S0005-2728(89)80075-1
- Eaton-Rye JJ, Putnam-Evans C 2005. The CP47 and CP43 core antenna components. In: Wydrzynski TJ, Satoh K eds. Photosystem II: the light-driven water:plastoquinone oxidoreductase. Advances in photosynthesis and respiration, volume 22. Dordrecht, Springer. Pp. 45–70.
- Edgar RC 2004. MUSCLE: multiple sequence alignment with high accuracy and high throughput. Nucleic Acids Research 32: 1792–1797. 10.1093/nar/gkh340
- Fagerlund RD, Eaton-Rye JJ 2011. The lipoproteins of cyanobacterial Photosystem II. Journal of Photochemistry and Photobiology B 104: 191–203. 10.1016/j.jphotobiol.2011.01.022
- Felsentein J 2013. PHYLIP (Phylogeny Inference Package) version 3.695. Distributed by the author. Department of Genomic Sciences, Seattle, University of Washington.
- Gupta RS, Mathews DW 2010. Signature proteins for the major clades of cyanobacteria. BioMed Central Evolutionary Biology 10: 24. 10.1186/1471-2148-10-24
- Gutensohn M, Fan E, Frielingsdorf S, Hanner P, Hou B, Hust B et al. 2006. Toc, Tic, Tat et al.: structure and function of protein transport machineries in chloroplasts. Journal of Plant Physiology 163: 333–347. 10.1016/j.jplph.2005.11.009
- Jones DT, Taylor WR, Thornton JM 1992. The rapid generation of mutation data matrices from protein sequences. Bioinformatics 8: 275–282. 10.1093/bioinformatics/8.3.275
- Keeling PJ 2013. The number, speed, and impact of plastid endosymbioses in eukaryotic evolution. Annual Review of Plant Biology 64: 583–607. 10.1146/annurev-arplant-050312-120144
- Komenda J, Knoppová J, Kopecná J, Sobotka R, Halada P, Yu J et al. 2012a. The Psb27 assembly factor binds to the CP43 complex of Photosystem II in the cyanobacterium Synechocystis sp. PCC 6803. Plant Physiology 158: 476–486. 10.1104/pp.111.184184
- Komenda J, Sobotka R, Nixon PJ 2012b. Assembling and maintaining the Photosystem II complex in chloroplasts and cyanobacteria. Current Opinion in Plant Biology 15: 245–251. 10.1016/j.pbi.2012.01.017
- Krupnik T, Kotabová E, van Bezouwen LS, Mazur R, Garstka M, Nixon PJ et al. 2013. A reaction centre-dependent photoprotection mechanism in a highly robust Photosystem II from an extremophilic red alga Cyanidioschyzon merolae. Journal of Biological Chemistry 288: 23529–23542.
- Larkum AWD, Lockhart PJ, Howe CJ 2007. Shopping for plastids. Trends in Plant Science 12: 189–195. 10.1016/j.tplants.2007.03.011
- Leliaert F, Smith DR, Moreau H, Herron MD, Verbruggen H, Delwiche CF et al. 2012. Phylogeny and molecular evolution of the green Algae. Critical Reviews in Plant Sciences 31: 1–46. 10.1080/07352689.2011.615705
- Liu H, Chen J, Huang RYC, Weisz D, Gross ML, Pakrasi HB 2013. Mass spectrometry-based footprinting reveals structural dynamics of Loop E of the chlorophyll-binding protein CP43 during Photosystem II assembly in the cyanobacterium Synechocystis 6803. Journal of Biological Chemistry 288: 14212–14220. 10.1074/jbc.M113.467613
- Liu H, Huang RY-C, Chen J, Gross ML, Pakrasi HB 2011b. Psb27, a transiently associated protein, binds to the chlorophyll binding protein CP43 in Photosystem II assembly intermediates. Proceedings of the National Academy of Sciences of the United States of America 108: 18536–18541. 10.1073/pnas.1111597108
- Liu H, Roose JL, Cameron JC, Pakrasi HB 2011a. A genetically tagged Psb27 protein allows purification of two consecutive Photosystem II (PSII) assembly intermediates in Synechocystis 6803, a cyanobacterium. Journal of Biological Chemistry 286: 24865–24871. 10.1074/jbc.M111.246231
- Mabbitt PD, Eaton-Rye JJ, Wilbanks SM 2013. Mutational analysis of the stability of Psb27 from Synechocystis sp. PCC 6803: Implications for models of Psb27 structure and binding to CP43. European Biophysics Journal 42: 787–793.
- Mabbitt PD, Rautureau GJP, Day CL, Wilbanks SM, Eaton-Rye JJ, Hinds MG 2009. Solution structure of Psb27 from cyanobacterial Photosystem II. Biochemistry 48: 8771–8773. 10.1021/bi901309c
- Mamedov F, Nowaczyk MM, Thapper A, Rögner M, Styring S 2007. Functional characterization of monomeric Photosystem II core preparations from Thermosynechococcus elongatus with or without the Psb27 protein. Biochemistry 46: 5542–5551. 10.1021/bi7000399
- Michoux F, Takasaka K, Boehm M, Komenda J, Nixon PJ, Murray JW 2012. Crystal structure of the Psb27 assembly factor at 1.6 Å: implications for binding to Photosystem II. Photosynthesis Research 110: 169–175. 10.1007/s11120-011-9712-7
- Nickelsen J, Rengstl B 2013. Photosystem II assembly: From cyanobacteria to plants. Annual Review of Plant Biology 64: 609–635. 10.1146/annurev-arplant-050312-120124
- Nixon PJ, Michoux F, Yu J, Boehm M, Komenda J 2010. Recent advances in understanding the assembly and repair of Photosystem II. Annals of Botany 106: 1–16. 10.1093/aob/mcq059
- Nixon PJ, Sarcina M, Diner BA 2005. The D1 and D2 core proteins. In: Wydrzynski TJ, Satoh K eds. Photosystem II: the light-driven water:plastoqunone oxidoreductase. Advances in photosynthesis and respiration, volume 22. Dordrecht, Springer. Pp. 71–94.
- Nowack ECM, Melkonian M, Glöckner G 2008. Chromatophore genome sequence of Paulinella sheds light on acquisition of photosynthesis by eukaryotes. Current Biology 18: 410–418. 10.1016/j.cub.2008.02.051
- Nowaczyk MM, Hebeler R, Schlodder E, Meyer HE, Warscheid B, Rögner M 2006. Psb27, a cyanobacterial lipoprotein, is involved in the repair cycle of Photosystem II. The Plant Cell 18: 3121–3131. 10.1105/tpc.106.042671
- Popelkova H, Im MM, D'Auria J, Betts SD, Lydakis-Simantiris N, Yocum CF 2002a. N-terminus of the Photosystem II manganese stabilizing protein: effects of sequence elongation and truncation. Biochemistry 41: 2702–2711. 10.1021/bi0118761
- Popelkova H, Im MM, Yocum CF 2002b. N-terminal truncations of manganese stabilizing protein identify two amino acid sequences required for binding of the eukaryotic protein to Photosystem II and reveal the absence of one binding-related sequence in cyanobacteria. Biochemistry 41: 10038–10045. 10.1021/bi020228u
- Roose JL, Pakrasi HB 2004. Evidence that D1 processing is required for manganese binding and extrinsic protein assembly into Photosystem II. Journal of Biological Chemistry 279: 45417–45422. 10.1074/jbc.M408458200
- Roose JL, Wegener KM, Pakrasi HB 2007. The extrinsic proteins of Photosystem II. Photosynthesis Research 92: 369–387. 10.1007/s11120-006-9117-1
- Schwede T, Kopp J, Guex N, Peitsch MC 2003. SWISS-MODEL: an automated protein homology-modeling server. Nucleic Acids Research 31: 3381–3385. 10.1093/nar/gkg520
- Shi L-X, Hall M, Funk C, Schröder WP 2012. Photosystem II, a growing complex: updates on newly discovered components and low molecular mass proteins Biochimica et Biophysica Acta 1817: 13–25.
- Shi L-X, Theg SM 2013. The chloroplast protein import system: from algae to trees. Biochimica et Biophysica Acta 1833: 314–331. 10.1016/j.bbamcr.2012.10.002
- Tyystjärvi E 2013. Photoinhibition of Photosystem II. In: Jeon KW ed. International review of cell and molecular biology, volume 300. Amsterdam, Elsevier. Pp. 243–303.
- Umena Y, Kawakami K, Shen J-R, Kamiya N 2011. Crystal structure of oxygen-evolving Photosystem II at a resolution of 1.9 Å. Nature 473: 55–60. 10.1038/nature09913
- Vass I 2011. Role of charge recombination processes in photodamage and photoprotection of the Photosystem II complex. Physiologia Plantarum 142: 6–16. 10.1111/j.1399-3054.2011.01454.x
- Wei L, Guo J, Ouyang M, Sun X, Ma J, Chi W et al. 2010. LPA19, a Psb27 homolog in Arabidopsis thaliana, facilitates D1 protein precursor processing during PS II biogenesis. Journal of Biological Chemistry 285: 21391–21398. 10.1074/jbc.M110.105064
- Xu Q, Bricker TM 1992. Structural organization of proteins on the oxidizing side of Photosystem II. Two molecules of the 33-kDa manganese-stabilizing proteins per reaction center. Journal of Biological Chemistry 267: 25816–25821.