ABSTRACT
Ecological disturbances triggered by earthquakes and volcanic eruptions are of fundamental importance in structuring the temperate forests of southwestern South America and New Zealand. We review studies of the ecological effects of these tectonic phenomena and how they have been central to progress in the modern development of forest ecology in both regions. Studies of tectonic influences on the dynamics of southern temperate rainforests of Chile and New Zealand published in the 1970s and early 1980s contributed prominently to the shift away from the equilibrium paradigms dominant globally in the 1960s and towards modern non-equilibrium frameworks of forest dynamics. Empirical studies of tectonic ecology in these temperate forests in combination with critical evaluations of earlier successional theory have significantly advanced understanding of the roles of coarse-scale disturbance in the dynamics of forests in southwestern South America and New Zealand. Recognition that cohort forest structures triggered by exogenous disturbances such as wind storms and tectonic events are the norm rather than all-aged structures has been of fundamental importance to understanding the dynamics of these forests. The non-equilibrium patch dynamics framework for interpreting forest structure and dynamics bolstered by tectonic ecology studies in southern South America and New Zealand was of key importance in refining older views of these forests as being out of equilibrium with contemporary climate, revising understanding of the effects of introduced browsing animals on forest structure, and guiding the development of appropriate forest management practices.
Introduction
The locations of southwestern South America (SSA) and New Zealand along boundaries of colliding tectonic plates mean that earthquakes and volcanic eruptions are major sources of ecological disturbances affecting the structure and function of the southern temperate forest ecosystems of these landmasses. Although the ecological importance of the destruction of vegetation on the flanks of erupting volcanoes has long been apparent, it was not until the late twentieth century that ecologists began to appreciate the effects of regionally widespread but diffuse earthquake-triggered mass movements on the structures of southern temperate forests in SSA and New Zealand (Veblen & Ashton Citation1978; Stewart & Veblen Citation1982a). Strong parallels exist between SSA and New Zealand as tectonically active zones with rapidly uplifting mountains and rugged topography. Coarse-scale disturbances directly or indirectly related to tectonism play a fundamental role in shaping the structure and function of these forests. Studies of the ecological effects of these tectonic phenomena have been central to progress in modern forest ecology research in both regions and have contributed significantly to the conceptual development of modern non-equilibrium frameworks in ecology globally. We argue that the study of the ecological effects of tectonism in SSA and in New Zealand played a role equivalent to the importance of studies of fire ecology in the development of modern forest ecology in many other parts of the world.
This synthesis focuses on the role of tectonic phenomena as triggers of ecological disturbance affecting the structure and dynamics of temperate forests in SSA and New Zealand. An over-arching theme of this synthesis is the fruitful interplay between ecological theory and the empirical field evidence of vegetation dynamics (e.g. Clarkson Citation1990). Modern ecological theory was developed primarily from early twentieth century studies conducted in northern temperate ecosystems of North America and Europe (Glenn-Lewin et al. Citation1992), which naturally raises questions about how observations in southern temperate forests may or may not be consistent with some of the generalities of ecological theory (e.g. González et al. Citation2014; Lara et al. Citation2014a). While fundamental ecological processes are expected to have universal applicability, differences in biota and environments between northern and southern temperate forests potentially may result in differences in the relative roles played by various types of disturbance, successional mechanisms, and plant traits shaped by those disturbances (e.g. Lusk et al. Citation2015). Hence, to provide context for the synthesis of tectonic ecology in SSA and New Zealand temperate forest ecosystems we first briefly describe how shifting paradigms have influenced interpretations of the dynamics of these forests.
Shifting paradigms in vegetation dynamics
During the last several decades of the twentieth century, appreciation of the roles of natural disturbances in vegetation dynamics shifted from viewing coarse-scale disturbances as relatively rare events interrupting otherwise stable vegetation configurations to a modern framework in which repeated disturbance and continuing change are emphasized (White Citation1979; Sousa Citation1984). This recognition of the role of natural disturbance in vegetation dynamics was the key driver of the shift from equilibrium to non-equilibrium paradigms in ecology that matured in the 1980s (Sousa Citation1984; Glenn-Lewin et al. Citation1992). This paradigmatic shift emphasized the volatility of environmental change and challenged the traditional views of natural systems trending toward stability or some sort of stasis. Research conducted largely during the 1970s and early 1980s in the southern temperate forests of SSA and New Zealand contributed significantly to this paradigm shift (Veblen & Stewart Citation1982a; Ogden Citation1985; Burrows Citation1990; Enright et al. Citation1995).
Clements’ (Citation1916) classic successional theory emphasized predictable changes in species composition culminating in self-perpetuating climax vegetation, the characteristics of which were largely controlled by a stable regional climate (Glenn-Lewin et al. Citation1992). The prevailing views in ecology through the 1960s stressed the predictability of vegetation change and the self-regulation of undisturbed ecosystems (White Citation1979; Sousa Citation1984). Conceptual frameworks of vegetation change in the 1960s and 1970s were termed developmental models of vegetation change by Drury and Nisbet (Citation1971) because they stressed the development of a predetermined, relatively stable plant community in a stable physical habitat. As an alternative to developmental models, Drury and Nisbet (Citation1971) proposed a kinetic model that does not assume a stable end point for succession and instead emphasizes continuous change. Hence, a kinetic framework accepts the fundamental instability of the physical site, in contrast to the assumption of long-term site and climatic stability in developmental models of vegetation change.
Although the term ‘kinetic’ did not persist in the ecological literature (but see Burrows Citation1990), emphasis on repeated disturbance is a key component of the modern non-equilibrium framework for studying vegetation dynamics (White Citation1979; Sousa Citation1984). In a non-equilibrium framework, repeated disturbance as a driver of change is given equal weight to plant-controlled changes of the micro-environment typically emphasized in traditional developmental models. Modern ecologists continue to examine the types and relative importance of plant-centred mechanisms of succession stressed by early twentieth century ecologists, but today equal attention is devoted to understanding plant population responses to disturbances and to quantifying disturbance regimens (Pickett et al. Citation1987; White & Jentsch Citation2001).
Disturbances influence plant communities through their direct effects on plant biomass by killing entire plants or removing parts of plants, which in turn affects competition, environmental conditions, and the availability of substrate and resources to other plants (White Citation1979; Pickett et al. Citation1987). Disturbance is a primary cause of fine-scale as well as coarse-scale spatial heterogeneity in ecosystems (White & Jentsch Citation2001). Tectonic disturbances are often sources of coarse-scale patchiness (lava flows, ash falls) but also can result in moderate- to fine-scale patchiness (landslides, tree-falls).
A disturbance regimen is a description of all the disturbances affecting an ecosystem, landscape, or even a larger region, and must be explicitly linked to a real place in the landscape (i.e. not generalized to an abstract biome type) and to a defined time period. The key descriptors of a disturbance regimen are the type of disturbance, spatial attributes and temporal characteristics (Sousa Citation1984). In the context of tectonic ecology, examples of spatial characteristics include extent and depth of volcanic ash fall (Swanson & Major Citation2005) or extent and location of earthquake-triggered mass movements relative to distance to epicentres, slope steepness and substrate (Wright & Mella Citation1963). Important temporal characteristics include not only frequency of events but also duration (e.g. of ash falls) and timing (e.g. coincidence of earthquakes with drought or high precipitation). Linked disturbances (sensu Simard et al. Citation2011) are disturbances that interact by altering the extent, severity, or probability of occurrence of a subsequent disturbance. Examples relevant to tectonic ecology include the effects of ash fall on susceptibility to wind-caused tree-falls or to foliar herbivory by insects. Compound disturbances (sensu Paine et al. Citation1998) refer to the occurrence of two disturbances at relatively short intervals that have a synergistic effect greater than the effect predicted from the sum of their individual impacts. Examples of compound disturbances relevant to tectonic ecology include ash fall on a post-fire stand too young to have reproductively mature trees, occurrence of high winds in a forest severely shaken by an earthquake, or torrential rainfall immediately following earthquake-triggered landslides.
Earthquake-related disturbances and forest dynamics in southwestern South America
A conspicuous feature of old stands of the Valdivian rainforests of south-central Chile is the scarcity of understorey trees of the same species that dominate the canopy of old forests, implying a lack of continuous tree regeneration of the regionally extensive dominant species. Specifically, for the Andean forests in the Province of Valdivia in unlogged old forests there is a scarcity of small diameters of Nothofagus dombeyi, Nothofagus alpina, and Nothofagus obliqua in mixed-species rainforests dominated by large trees of these species (Brun Citation1975). In contrast, the associated shade-tolerant tree species such as Laureliopsis philippiana, Aextoxicon punctatum and Saxegothaea conspicua are abundantly represented in all size classes from juveniles in the understorey through subcanopy and main canopy trees (Brun Citation1975). Hence, the size structure of these forests is indicative of a successional trend from shade-intolerant Nothofagus spp. towards dominance by the shade-tolerant species. The dominance of Nothofagus spp. throughout the Valdivian Andes raises the question of why there are apparently no extensive stands representing a compositional equilibrium (or climax) dominated exclusively or primarily by the shade-tolerant tree species. Observations in the 1970s of the effects of mass movements and other disturbances triggered by the giant 1960 Chile earthquake (at magnitude 9.5 Mw the most powerful recorded; Cisternas et al. Citation2005) were pivotal in unravelling this apparent paradox (Veblen & Ashton Citation1978).
A series of studies conducted in the late 1970s developed and tested the hypothesis that the importance of Nothofagus spp. in the old-growth forests of the mid-montane Valdivian Andes depends on their periodic regeneration after severe coarse-scale disturbances (Veblen & Ashton Citation1978). The evidence in support of this hypothesis included: (i) the abundance and vigorous growth of Nothofagus seedlings on surfaces recently disturbed by mass movements; (ii) the absence or scarcity of Nothofagus regeneration in the understoreys of the mixed-species old-growth stands; and (iii) the historical frequency of coarse-scale earthquake-triggered mass movements and other associated disturbances such as flooding (Veblen et al. Citation1980, Citation1981). Although the hypothesis was developed specifically for the Valdivian Andes (c. 40°S), it provided a conceptual framework for subsequent investigation of the roles of disturbance in the dynamics of temperate forests generally in Chile and Argentina (Gutiérrez et al. Citation2004; Lara et al. Citation2014a).
On the wet western side of the Andes, strong earthquakes periodically trigger thousands of mass movements (e.g. landslides, rock falls, debris flows and mudflows; Wright & Mella Citation1963). Thick volcanic ash layers on glacially over-steepened slopes are highly prone to mass movements triggered by earthquakes or by intense rain storms (Wright & Mella Citation1963). Bare surfaces exposed by mass movements are rapidly colonized (often within 1 to 5 yr) by tree species including Nothofagus spp., Aristotelia chilensis, Caldcluvia paniculata, Drimys winteri, Embothrium coccineum, Eucryphia cordifolia, Lomatia ferruginea and Weinmannia trichosperma (Veblen & Ashton Citation1978; Veblen et al. Citation1989; Rosas Citation1991; Larraín Citation1997). Successful establishment and growth of these trees on bare surfaces is probably facilitated by the ectomycorrhizae of the Nothofagus spp. and by the presence of nitrogen fixers such as the cyanobacterial genus Nostoc in symbiotic association with the giant herb Gunnera chilensis () (Veblen et al. Citation1996b).
Figure 1. Photographs of landslides at Lago Todos Los Santos, Chile triggered by the 1960 giant earthquake. A, January 1966 showing the site completed denuded by the sliding of soil, vegetation and volcanic ash. B, The site dominated by the giant perennial herb Gunnera chilensis and shrubs in March 1979. C, December 1993, showing that trees (primarily Nothofagus dombeyi) that were present as seedlings beneath the herb and shrub cover in 1979 have grown into a closed canopy forest. Photographs: A, Carlos Vargas; B and C, Thomas Veblen.
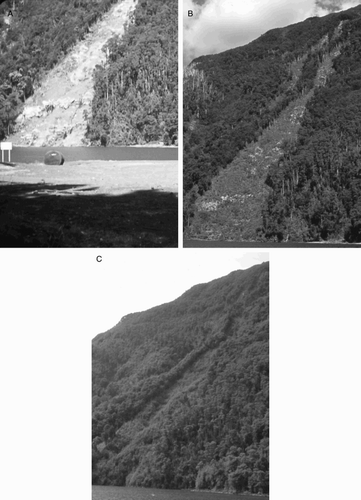
Over extensive areas in the Valdivian rainforest district, relatively old (> 300 yr old) stands are dominated by emergent trees of shade-intolerant species such as Nothofagus spp. and Eucryphia cordifolia (Veblen & Ashton Citation1978; Veblen et al. Citation1980, Citation1981; Donoso et al. Citation1984; Donoso et al. Citation1985; Veblen Citation1985; Armesto & Figueroa Citation1987). In undisturbed stands, young or small trees of these species are absent or scarce. In contrast, shade-tolerant tree species (such as Laurelia sempervirens, Laureliopsis philippiana, Saxegothaea conspicua, Persea lingue, Aextoxicon punctatum and numerous myrtaceous trees) are typically abundant and occur as all-aged populations (Donoso et al. Citation1984, Citation1985; Veblen Citation1985; Armesto & Figueroa Citation1987; Lusk Citation1996b; Pollmann & Veblen Citation2004). In the absence of disturbance, there is a gradual successional trend towards dominance by these shade-tolerant species. In landscapes with a high frequency of coarse-scale disturbances, this successional trend is not completed and the shade-intolerant trees (especially Nothofagus) remain dominant in the oldest stands (Veblen & Ashton Citation1978, Veblen et al. Citation1981).
Written records and tree-ring reconstructions document high-magnitude earthquakes affecting south-central Chile and the adjacent eastern slopes of the Andes, which triggered widespread mass movements and floods in 1575, 1737, 1751, 1837 and 1960 (Veblen & Ashton Citation1978; Kitzberger et al. Citation1995). Along the coast of Chile these earthquakes caused extensive forest mortality due to subsidence, tsunamis and flooding as revealed by stratigraphy of soils buried by flood deposits (Cisternas et al. Citation2005). On the islands and the coast south of Chiloé Island, stands of dead-standing trees lacking evidence of fire were attributed to effects of tidal waves and tectonic subsidence associated with the 1837 earthquake (Rothkugel Citation1916). Dead stands of inundated coastal forests occur throughout the southern Chilean Archipelago from just south of Chiloé Island to Tierra del Fuego and have been linked to earthquake-triggered subsidence (Goodall Citation1979).
Studies of the effects of large-scale mass movements and associated flooding whether triggered by earthquakes or by torrential rain storms (Lara Citation1991), supported development of a general model of forest dynamics in the Andean region of the mid-latitudes of South America (Veblen et al. Citation1996a, Citation1996b; Pollmann & Veblen Citation2004). The focus of the model originally was on Nothofagus spp. but with modifications to account for differences in distribution and habitats, it also applies to other long-lived shade-intolerant pioneer species for which regeneration is dependent to a large degree on canopy opening by disturbances (e.g. Eucryphia cordifolia, Weinmannia trichosperma) (Veblen et al. Citation1981; Veblen Citation1985; Donoso et al. Citation1984, Citation1985; Lusk Citation1999). The ubiquitous distribution of Nothofagus in the Andes at these latitudes can be explained by two processes : (i) At climatically and edaphically favourable sites where there is intense competition from shade-tolerant rainforest species, coarse-scale disturbances create establishment opportunities for Nothofagus and other shade-intolerant long-lived pioneer species. (ii) At climatically unfavourable sites (i.e. cooler or drier sites) and on edaphically suboptimal sites, the rainforest species are absent or rare, and consequently Nothofagus is able to regenerate even in the absence of coarse-scale disturbance. The interpretation that disturbance favours Nothofagus persistence is also supported by life history traits expected for long-lived pioneer species, including rapid tree growth rates under open conditions, longevity of at least several centuries, and effective seed dispersal into canopy openings (Veblen et al. Citation1996b; Lusk & del Pozo Citation2002; Pollmann Citation2004). In species-rich lowland forests intense competition from abundant shade-tolerant species precludes regeneration of Nothofagus beneath tree-fall gaps (Veblen et al. Citation1979; Veblen Citation1985), but at higher elevations or drier sites with fewer tree species the same size gap may result in successful regeneration of Nothofagus (Veblen Citation1989a, Citation1989b; Pollmann & Veblen Citation2004).
The general model of Nothofagus forest dynamics described above pertains primarily to the Andes where volcanism and earthquake-caused mass movements are common but not to the non-volcanic coastal mountains of southern Chile where these tectonic disturbances are relatively unimportant. In the coastal mountains the relative dominance of shade-tolerant tree species is greater; shade-intolerant pioneer species are less abundant and even locally absent (Veblen et al. Citation1996b). For example, Nothofagus spp. are largely absent at elevations below 400 m between 41°S and 43°S in the coastal mountains (Veblen et al. Citation1981; Armesto & Figueroa Citation1987). In the absence of large-scale tectonic disturbances, fine-scale gap processes predominate in the low- to mid-elevation coastal ranges (Veblen et al. Citation1981; Armesto & Fuentes Citation1988; Lusk Citation1996b). In addition, coarse-scale disturbance by stand-scale blowdowns and pre-Hispanic fires have also been documented in the coastal forests (Lusk Citation1996a, Citation1996b).
Recognition of the importance of coarse-scale natural disturbances, triggered by tectonic events as well as others such as severe storms, has informed the development of science-based forest management in SSA. Management prescriptions for forests dominated by long-lived pioneer species (primarily Nothofagus spp.) are based on a sound understanding of their responses to coarse-scale disturbances (Donoso et al. Citation2014). As recently as the 1970s the most valuable timber trees, such as N. alpina, were selectively logged from these forests, resulting in no or little regeneration of the valuable timber species and a degradation of the resource. Recognition of the role of infrequent coarse-scale disturbance in creating regeneration opportunities for threatened species such as Fitzroya cupressoides, formerly regarded as a relict not adapted to the current climate, similarly has been important in restoration and species preservation projects (Lara et al. Citation2014b).
Earthquake-related disturbance and forest dynamics in New Zealand
New Zealand is prone to earthquakes because it sits on the boundary between the Australian and Pacific crustal plates, a boundary marked in part by the Alpine Fault, which stretches along the entire length of the South Island (Wells et al. Citation2001). Explorers in the late nineteenth century in Westland noted that much of the forest appeared to be dominated by similarly sized trees and suggested that widespread forest destruction had occurred in the past, possibly caused by earthquakes (Charles Douglas in Holloway Citation1957). A team of governmental scientists investigating potential causes of forest mortality in the 1950s in Westland also noted what appeared to be even-aged patches of forest and also ascribed their origin to major earthquakes (Chavasse Citation1955). These patterns were later described by Wardle (Citation1966, Citation1971) who further suggested that a major earthquake occurred between AD 1730 and 1740 (Wardle Citation1980). The first in-depth studies of the dynamics of these forests documented that indeed many of the forests were dominated by even-aged stands of trees as a consequence of earthquake-triggered mass movements (Stewart & Veblen Citation1982a; Veblen & Stewart Citation1982a; Stewart & Rose Citation1989).
Regional compilation of forest structure data for Westland indicated episodes of widespread forest regeneration around 250–350 and 550–600 years before present (yr BP) that appeared to have been initiated by catastrophic disturbances (Wells et al. Citation1998). Tree-ring reconstruction of forest disturbances in south Westland in conifer–hardwood forests documented catchment-wide episodes of cohort-initiating disturbances around AD 1820–30, 1710–1720, 1610–1620 and 1460 (Wells et al. Citation2001). These episodes coincide with the three most recent Alpine Fault earthquakes (c. AD 1717, 1630 and 1460) and with earthquakes recorded to the south of the study area in AD 1826. Eighty-six per cent of the study area had experienced erosion and sedimentation events over the last 650 yr (Wells et al. Citation2001).
The impacts of earthquakes on forest structure and composition vary widely with topographic setting, magnitude of the earthquake, and time since the previous strong earthquake. In Westland, even-aged cohorts initiated by the AD 1717 earthquake are located near the Alpine Fault line and in the frontal ranges of the Southern Alps, whereas even-aged stands triggered by the AD 1630 earthquake occur on floodplains all the way to the coast (Cullen et al. Citation2003). The AD 1630 earthquake followed a relatively long period since the previous strong earthquake in AD 1460, allowing for more sediment build-up in the headwaters of streams for release and deposition downstream in floodplains (Cullen et al. Citation2003). A combination of fault trenching, 14C dating of landslides and terraces, and tree-ring dating demonstrate that infrequent, strong earthquakes, through triggering erosion and flooding events, are the dominant forest disturbance agents in Westland (Adams Citation1980; Wells et al. Citation1999). Tree regeneration opportunities created by earthquakes for different groups of tree species depend on topography and edaphic conditions. The 1968 Inangahua earthquake (magnitude 7.1 Mw) triggered landslides in the Buller Gorge exposing bare surfaces that have recovered to regenerating stands of Nothofagus fusca and Nothofagus menziesii (). In the mixed conifer/beech/broadleaved forests of north Westland on a post-glacial terrace in the Grey Valley after an earthquake around 365 yr ago, N. fusca, N. menziesii and Weinmannia racemosa regenerated on well-drained sites while Dacrycarpus dacrydioides and Dacrydium cupressinum along with N. menziesii regenerated on poorly drained soils (Urlich et al. Citation2005). These differential patterns of regeneration can be further complicated by the availability of suitable establishment sites for seedlings as in these wet forests many tree species preferentially establish on raised surfaces such as upturned trees and logs (Stewart & Veblen Citation1982a; Stewart Citation2002).
Figure 2. Photographs showing landslides triggered in the Buller Gorge (Westland, New Zealand) by the 1968 Inangahua earthquake (magnitude 7.1 Mw). A, Mostly bare surfaces and a narrow band of tree regeneration along the forest edge in 1980. B, 2011 showing recovery to young stands of Nothofagus fusca and Nothofagus menziesii. Photographs: Thomas Veblen.
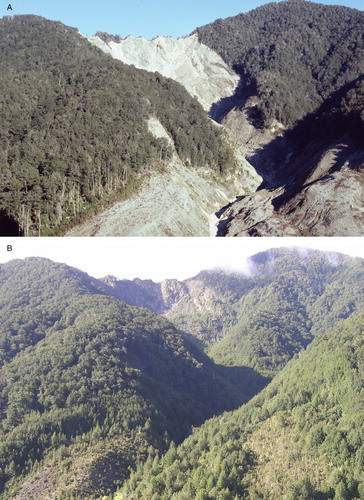
Although less frequently studied than for the Alpine Fault zone of Westland, earthquake impacts are significant in forests elsewhere on the South Island. In the Matiri Valley in the northwest of the South Island the Murchison earthquake (7.7 Mw) in 1929 resulted in pulses of regeneration of N. fusca in large gaps created by landslides whereas smaller gaps with little or no landslide damage were rapidly closed by crown expansion of N. fusca and/or N. menziesii (Vittoz et al. Citation2001). Further south and east, in inland Canterbury, permanent plots established around 1970 in Nothofagus solandri var. cliffortioides forests recorded a 23% decline in stand biomass following a 6.7 Mw earthquake in 1994 (Allen et al. Citation1999). On average, 74% of the total stem biomass mortality resulted from landslides (Allen et al. Citation1999).
A little-studied but important influence on coastal forests of the South Island has been from forest disturbance caused by earthquake-triggered tsunami inundation. Sedimentological, geochemical and micro-palaeontological data from the Ōkārito Lagoon in south Westland indicate that tsunami inundation occurred in 1826, a known date of earthquake activity reported by early sealers in the area (Goff et al. Citation2004). Similarly, episodic formation of shore-parallel dune systems at Haast further south has been linked to major ruptures of the Alpine Fault, and the dunes support tree cohorts established soon after major regional earthquakes in c. AD 1460, 1615, 1717 and 1826 (Wells & Goff Citation2006).
A conspicuous feature of central Westland forests are the extensive, even-sized Metrosideros umbellata/Weinmannia racemosa stands that are prominent along the steep front ranges and middle valley reaches over at least 250 km (Stewart & Veblen Citation1982a; Rose et al. Citation1992). It has been suggested that many of these stands originated following a large Alpine Fault earthquake (Holloway Citation1957; Wardle Citation1980; Stewart & Veblen Citation1982b). The M. umbellata and W. racemosa sampled in these stands all originated after the last major Alpine Fault earthquake in AD 1717 (Wardle Citation1980; Wells et al. Citation2001), and it is likely that stands of this age are widespread. This could help to explain the widespread dieback of these stands at mid-elevations in Westland (Chavasse Citation1955, Stewart & Veblen Citation1982b, Citation1983). Stand dieback has commonly been attributed primarily to browsing by the introduced brushtail possum (Trichosurus vulpecula) (e.g. Bachelor Citation1983). However, trees growing in even-aged cohorts that arose after a region-wide disturbance event would senesce at about the same time, making them more susceptible to widespread synchronous dieback from a trigger factor such as possum browse (Veblen & Stewart Citation1982b). Location and extent of these even-aged stands helps to explain the variation in the magnitude of canopy dieback observed throughout Westland (Stewart & Veblen Citation1982b; Stewart & Rose Citation1988; Rose et al. Citation1992).
Volcanism and its influence on the forests of the southern Chile and Argentina
Effects on the rainforests of southern Chile
The principal types of disturbances associated with eruptions of the many volcanoes located in the mid-latitude Andean region of temperate rainforests are lava flows, pyroclastic flows, air-borne deposits (tephra or ash falls), mudflows (lahars) and debris avalanches (Swanson & Major Citation2005). Vast areas in southern Chile are covered in tephra deposits from numerous eruptions throughout the Holocene indicating widespread and severe volcanic disturbances (Casertano Citation1963; Jara & Moreno Citation2012). Analogous to the situation described for earthquake-triggered mass movements above, bare or partially bare surfaces created by volcanic disturbances can, under particular circumstances, be colonized by some of the dominant tree species of the region including Nothofagus spp. as well as the conifers Araucaria araucana and Fitzroya cupressoides (Veblen et al. Citation1977; Veblen & Ashton Citation1978; Veblen Citation1982; Lara Citation1991; González et al. Citation2014). And, again this is a pattern typical of the Andes but not found in the non-volcanic coastal mountain ranges of south-central Chile and Chiloé Island where non-tectonic disturbances such as fine-scale gap processes are more prevalent (Veblen et al. Citation1981; Armesto & Fuentes Citation1988; Lusk Citation1996b).
Only a few recent volcanic eruptions have been studied in detail to reveal the complexity of their ecological impacts. These include the eruptions of the Hudson, Chaitén and Cordón Caulle volcanoes in 1991, 2008 and 2011, respectively (Swanson et al. Citation2013; González et al. Citation2014). In the 1991 eruption of the Hudson Volcano at c. 46°S, extensive forests of Nothofagus pumilio, N. dombeyi and Nothofagus antarctica were killed by ash fall and by the lahars and floods that affected the Rio Ibañez. The Chaitén eruption affected primarily Valdivian rainforest at c. 43°S, whereas the eruption of Cordón Caulle (c. 40°35′S) deposited ash not only in adjacent areas of Valdivian rainforest but, due to the prevailing westerly winds ash, also affected large areas of drier Nothofagus and Austrocedrus forests in Argentina east of the Andes (). Many woody and rhizoid understorey species survived ash burial of depths of c. 10 cm or more (Vogel et al. Citation1994; González et al. Citation2014). The understorey shrubs Drimys andina and Maytenus disticha survived several months of intermittent ash deposition during Cordón Caulle eruption (A; González et al. Citation2014). Resprouting and production of adventitious roots and shoots facilitated the survival and recovery of the bamboo Chusquea quila, the large fern Lophosoria quadripinnata, shrubs such as Ribes magellanicum, Ovidia andina, Berberis spp. and Fuchsia magellanica and the perennial herb Gunnera magellanica (B; Vogel et al. Citation1994; González et al. Citation2014). Gunnera magellanica is abundant at wet sites and due to its symbiotic association with the nitrogen-fixing cyanobacterial genus Nostoc is a key species promoting accumulation of C and N in the soil (Fernández-Martinez et al. Citation2013). Two to three years after the eruption of Cordón Caulle and Hudson volcanoes there was a massive recruitment of N. pumilio (B), but the long-term survival of these seedling populations is likely to depend on fortuitous establishment in the limited areas of suitable light and soil nutrient conditions (Vogel et al. Citation1994). Although the presence of mature trees of species such as Nothofagus spp., Araucaria araucana, Fitzroya cupressoides, Weinmannia trichospherma and Austrocedrus chilensis on scoria deposited within the last one to a few centuries is commonly observed (Veblen & Ashton Citation1978; Veblen Citation1982; González et al. Citation2014), to date there are no published studies of the site conditions necessary for such successful tree regeneration on volcanic deposits.
Figure 3. Schematic representation of the typical ash fall patterns and effects on vegetation dynamics along a west-to-east gradient in northwestern Patagonia from eruptions of the Puyehue–Cordón Caulle volcanic complex.
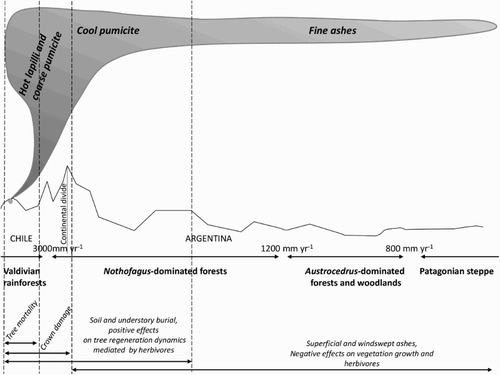
Figure 4. Photographs of the Cordón Caulle ash fall in a Nothofagus pumilio forest. A, Drimys andina survived despite being buried by ash for 6 months. B, A fallen tree provided a safe microsite for establishment of the shrub Ribes magellanicum, the herbaceous creeper Acaena ovalifolia, and the perennial herb Gunnera magellanica. Photographs: A, Thomas Veblen; B, Mauro González.
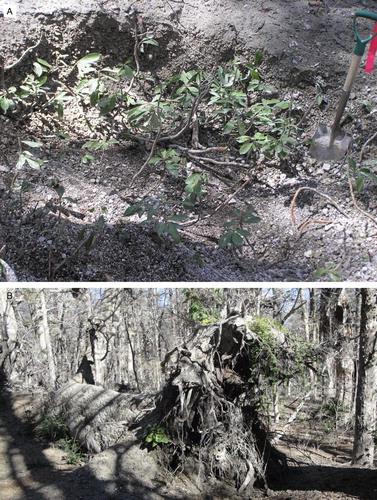
Figure 5. Photographs of volcanic ash from Cordón Caulle. A, Cordón Caulle erupting in January 2012 with Nothofagus pumilio in the foreground that mostly died; the explosive phase of the eruption lasted from June 2011 to April 2012. B, Abundant establishment of Nothofagus pumilio seedlings in 2014 c. 40 km west of Cordón Caulle at western Lago Traful on a c. 5–6 cm thick ash layer. Photographs: A, Thomas Veblen; B, Joaquin Brunet.
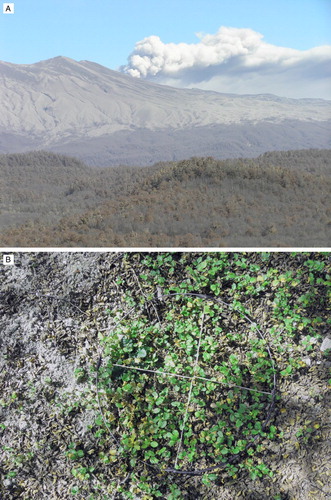
The eruption of the Chaitén Volcano in 2008 sent ash 20 km into the atmosphere, which was transported by the predominantly westerly winds eastwards over an area larger than a 1000 km2 (Swanson et al. Citation2013). During the initial few days of activity, ash up to 10 cm deep was deposited at distances of 30 km from the source. Coarse tephra was deposited on the flanks of the volcano and transported into rivers and forested floodplains. The volcanic and associated hydrological processes resulted in a broad range of mechanisms and severities of ecological disturbance affecting trees, including tree snapping from impact force, defoliation, abrasion, heat damage, canopy loading by ash capture, and stem burial by mudflows and ash. Coarse tephra (gravel size) abraded tree foliage over an area of c. 50 km2, and crown weighting due to fine tephra capture broke branches and bent young, flexible trees over an area of 480 km2. In the toppled-tree zone, species observed to have sprouted from epicormic buds on trunks and branches included Weinmannia trichosperma, Eucryphia cordifolia, Amomyrtus luma and Amomyrtus meli, but in some cases the sprouts subsequently died (Swanson et al. Citation2013). Flood deposition of > 1 m of re-mobilized tephra buried floodplain forests over many kilometres of the adjacent river valleys (Swanson et al. Citation2013).
At sites outside the total destruction zone of the Chaitén eruption, the weight of fine-grain volcanic ash stripped branches off large canopy trees and toppled smaller trees (Swanson et al. Citation2013). The fact that most of the trees in the rainforests of southern Chile are broadleaved evergreen angiosperms makes them particularly vulnerable to damage resulting from the weight of leaf capture of volcanic ash. Litter fall traps installed in a forest 25 km south of the Villarrica Volcano collected 13.6 tons/ha of sand-size ash over a few days of volcanic activity in 1971 (Veblen et al. Citation1980). This type of relatively infrequent but spatially extensive canopy disturbance may provide regeneration opportunities for the dominant shade-intolerant species (Nothofagus spp., Eucyrphia and Weinmannia), which otherwise would slowly be replaced by more shade-tolerant rainforest species (Veblen et al. Citation1981). In fact, multi-millennial fossil pollen records from rainforest sites indicate abrupt increases in Eucryphia and Weinmannia pollen following tephra deposition, even at sites many kilometres distant from the volcanic vents (Jara & Moreno Citation2012). Both Eucryphia and Weinmannia are long-lived pioneer species dependent on canopy openings for regeneration (Veblen Citation1985; Lusk Citation1999; Gutiérrez et al. Citation2004), leading to the conclusion that eruptions can enhance regional abundance of early to mid-successional species (Jara & Moreno Citation2012). In addition to regeneration opportunities resulting from canopy openings due to ash fall, fires ignited by volcanism create regeneration opportunities for the shade-intolerant tree species (Veblen & Ashton Citation1978; Jara & Moreno Citation2012).
Tephra fall is the most widespread volcanic disturbance and the repeated re-mobilization of ash as mudflows is particularly important in the alpine treeline zone where vegetation recovery is inherently slow (Veblen et al. Citation1977). In the treeline zone, N. antarctica resprouts adventitiously from its branches, giving it the capacity to withstand at least 30 cm of burial by ash mudflows (Veblen et al. Citation1977). Similarly, N. pumilio can develop a new lateral root system at the new soil surface following burial by mudflows of volcanic ash (Veblen et al. Citation1977). However, repeated burial of trees by volcanic ash in combination with the limited water-holding capacity of the coarsely textured tephra results in treelines depressed below the elevations they could potentially reach under climatic limitations alone (Daniels & Veblen Citation2003; Daniels & Veblen Citation2004).
Ash fall effects on drier forests east of the Andes in northwestern Patagonia
Given the predominantly westerly winds at mid-latitudes in SSA, eruptions of Andean volcanoes on the western side of the continent deposit large quantities of ash eastwards into Argentina affecting a gradient of vegetation from mesic through dry forests and the Patagonian steppe. Thickness of tephra deposits attenuates sharply from west to east and particle size declines from gravel size within a few kilometres of the source to fine tephra (sand and silt) eastwards. Thus, the 2011 eruption of Cordón Caulle deposited thick layers of gravel- and sand-sized tephra in mesic Nothofagus forests on the eastern slopes of the Andes and thinner layers of silt-sized ash in the drier Austrocedrus forests and woodlands in the foothills near the ecotone with the steppe ()
During the June 2011 eruption of Cordón Caulle the westernmost N. pumilio forests in Argentina were affected by incandescent volcanic ejecta, which damaged foliage and growth buds on trees in permanent plots at a distance of 22 km from the vent (Chaneton et al. Citation2014). The result was partial crown mortality but rarely death of the entire tree at that distance from the source. Hot ash damaged winter foliar buds, which reduced leaf area production by nearly 60% during the following season (Chaneton et al. Citation2014). This is in contrast to more westerly N. pumilio stands located within a few kilometres of the volcano, which under hotter ash fall, suffered complete bud mortality and tree death (A).
Further east in sub-mesic pure Nothofagus forests, the most prominent effect of the eruption of Cordón Caulle is the burial of soils and understorey plants by sandy pumicite layers that create a bare mineral substrate beneath intact forest canopies. Seedling establishment of both N. dombeyi and N. pumilio is directly and indirectly enhanced by ash deposition (J. Brunet et al. unpubl. data). Dense carpets of new Nothofagus seedlings established on the ash (B) indicate that germination and early growth are favoured by the shift from a forest litter substrate to an ash substrate from which the pre-existing understorey has been mostly removed. Seedlings of N. dombeyi that endured the ash burial form adventitious roots that rapidly proliferate and grow laterally through the new ash layer. Due to the dramatic reduction in available forage (both beneath forest canopies and in open areas), cattle populations declined by c. 50% so that browsing and trampling damage on tree regeneration was sharply reduced. Cattle numbers declined partly due to mortality, but mostly due to the decision of cattle owners to remove animals because of the lack of forage (J. Brunet et al. unpubl. data). Overall, despite some subsequent seedling mortality, burial of soils and understorey had the net effect of increasing Nothofagus regeneration; this was particularly the case for stands where large cattle populations had previously impeded tree regeneration (Veblen et al. Citation1992). Several prominent cohorts of Nothofagus (particularly N. pumilio) in the Lago Traful area appear to have developed from the release of advance regeneration coincident with the 1920 and 1960 eruptions of the Puyehue–Cordón Caulle complex (J. Brunet et al. unpubl. data).
Further east at the ecotone with the steppe, ash deposition in 2011 grass growth declined by 36–50% due to reduced interception of solar radiation (Oyarzabal et al. Citation2011; Irisarri et al. Citation2012). In addition, in the open interspaces among the shrubs and bunchgrasses, ash deposition favoured the recruitment of perennial rhizomatous grasses or geophytes over annuals (Ghermandi & González Citation2012). The event had a large impact on livestock dependent on highly productive mallines (wetland meadows) composed of short grasses that became buried by ashes resulting in stock losses or relocation to distant pastures.
In addition to direct effects on vegetation, volcanic eruptions have important indirect effects on forest ecosystem functioning mediated by impacts on insect herbivores. Effects of the ash deposition from the 2011 Cordón Caulle eruption on arthropods are highly variable according to feeding habits and habitats of arthropods (Ruggiero & Kitzberger Citation2014). Ants and ground beetles remained relatively undisturbed by the event. In contrast, arthropod pollinators and canopy foliovores exhibited significant impacts. Ash deposition resulted in a sustained disruption of pollination services by a key bumble bee species (Morales et al. Citation2014). For insect foliovores, the ash fall acted as a natural insecticide at a regional scale, drastically reducing insect-caused leaf damage in N. pumilio forests (Chaneton et al. Citation2014).
Volcanism and its influence on the forests of New Zealand
Volcanism has strongly shaped the contemporary forest pattern in the North Island of New Zealand. In particular, in the central North Island numerous eruptions of the Taupo supervolcano have occurred over the last 300,000 yr, and its most recent massive pumice eruption in 1718 ± 5 cal yr BP covered 30,000 km2 with tephra deposits and c. 20,000 km2 with ignimbrite (Hogg et al. Citation2012). Near Lake Taupo, conifer-broadleaved hardwood forests similar in structure and composition to the contemporary forests were buried by more than 100 m of pyroclastic flows (Clarkson et al. Citation1992, Citation1998). Stands of bracken (Pteridium esculentum) flourished immediately after the eruption, and fires occurred during and for several decades following the eruption (Wilmshurst & McGlone Citation1996). Most sites available for plant establishment after an extensive rhyolitic eruption are deficient in nutrients, especially nitrogen and phosphorus, so that often the first establishing plants are species that harbour mycorrhizal associations (Uhe Citation1972). Several tall forest species (Weinmannia and Metrosideros) produce abundant, light wind-dispersed seed and also sprout after damage (Wardle Citation1966, Citation1971). However, even several centuries following deposition of up to 40 cm of ash over a 150 km2 area around Mount Taranaki, the composition of the treeline vegetation reflects this disturbance event and treeline elevation is suppressed below the elevation of unaffected areas (A; Efford et al. Citation2014). Light-demanding, cold-tolerant taxa were able to take advantage of the newly created open sites resulting in establishment of new cohorts of taxa such as Libocedrus bidwilli (B; Efford et al. Citation2014).
Figure 6. Photographs of treeline vegetation on the North Island of New Zealand. A, Tephra deposit (35 cm thick) near Dawson falls. B, Treeline vegetation near North Mount Taranaki/Egmont with emergent Libocedrus bidwillii. Photographs: Jackson Efford.
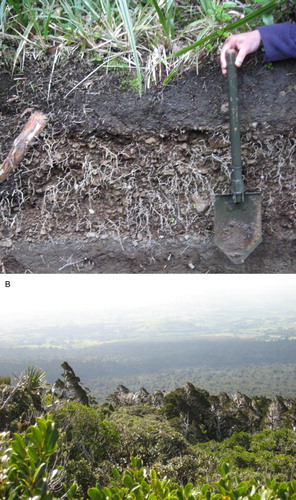
The relative roles of volcanic disturbance and climatic gradients in determining the spatial pattern of species composition of the current conifer/broadleaved hardwood forests of the Taupo region have been widely debated. McKelvey (Citation1963, Citation1973) ascribed a dominant role to the eruption, documenting a pattern where conifers were dominant close to the eruption centre, and broadleaved hardwood trees dominated further away. He concluded that the dense conifer stands were a pioneering stage in a somewhat slow centripetal colonization away from the eruption centre. Clarkson et al. (Citation1992) examined macrofossil evidence to evaluate McKelvey’s hypothesis. They found that broadleaved forest, notably Beilschmiedia tawa, is not the dominant endpoint of the forest succession on flat to undulating sites. They also showed differences in the species composition of forests buried by the Taupo eruption that were probably due to pre-eruption differences in local climate and soils. Overall, the fossil record indicates little or no convergence towards broadleaved dominance predicted by McKelvey (Citation1963). Furthermore, it suggests that site differences as well as fertility gradients over time scales of centuries to millennia are likely to be important determinants of forest composition at sites affected by the Taupo eruption. Clarkson et al. (Citation1986) showed that forests can rapidly re-form, especially where forest destruction has been only fragmentary or partial. At least some of the dense conifer stands are of uneven age, suggesting that they may have arisen after a more complex set of circumstances (Herbert Citation1980). Conifer population age structures in Tongariro National Park exhibit discontinuous age distributions, indicating that establishment had occurred underneath a progressively dying overstorey rather than after a single massive exogenous disturbance (Lusk & Ogden Citation1992). Leathwick and Mitchell (Citation1992) showed that forest patterns in the volcanically disturbed central North Island are also strongly correlated with spatial patterns of climate, topography and drainage. Although the massive eruption of Taupo in 1718 ± 5 cal yr BP triggered abrupt changes in the vegetation patterns, over time the effects of climate and topographic patterns also have affected post-disturbance vegetation patterns (Leathwick & Mitchell Citation1992).
Clarkson (Citation1990) systematically evaluated the field evidence of vegetation changes following volcanic disturbance in the context of traditional (Clements Citation1916) and modern (e.g. Pickett et al. Citation1987) models of succession. He found that there was no exclusive ‘volcanic succession flora’. In fact, the flora of the volcanically disturbed sites has many of the attributes of plants that ensure success after other disturbances, including fire, as can be seen in the greater abundance and range of the same species during periods of Polynesian (and later, European) burning (McGlone Citation1983). He stressed the importance of variability related to scale and intensity of disturbance, and the type and heterogeneity of the substrate emplaced. Primary succession on large denuded sites exhibited elements of facilitation, autogenic change and relay floristics consistent with classic models of succession. Examples, revealed by subsequent detailed research, include initial establishment of lichens and mosses followed by flowering plants and facilitation by nitrogen-fixers such as Coriaria spp. (Clarkson & Clarkson Citation1995; Walker et al. Citation2003). An experiment representing post-volcanic succession showed that establishment of Coriaria arborea greatly accelerated an underlying trend of gradually increasing numbers and diversity of seeds in the soil (Clarkson et al. Citation2002). Overall, changes in species compositions involved a range of successional mechanisms including facilitation and inhibition, patterns explained by time gradients of resource availability, and disturbance regimens producing shifting-mosaic steady-states. Clarkson (Citation1990) stressed the need for long-term studies of processes of vegetation change and the importance of chance events that make predictions probabilistic rather than deterministic.
Conclusion
Studies conducted largely in the 1970s and early 1980s of the effects of tectonic disturbances, specifically disturbances associated with strong earthquakes, revealed that in extensive areas of southern Chile and New Zealand these relatively infrequent coarse-scale disturbances are key determinants of forest structure and composition (Veblen & Ashton Citation1978; Stewart & Veblen Citation1982a). These studies of the long-lasting effects of tectonic events pioneered systematic investigation of coarse-scale disturbance processes in the context of the non-equilibrium frameworks that became increasingly accepted during the 1980s and 1990s in both regions. This conceptual framework emphasizing repeated coarse-scale disturbances, including disturbances triggered by tectonism, has strongly influenced subsequent development of ecological research on southern temperate forests (Ogden Citation1985; Read & Hill Citation1988; Enright et al. Citation1995; Ogden et al. Citation1996; Adie & Lawes Citation2009; Lara et al. Citation2014a; Lusk et al. Citation2015).
Studies of the dynamics of temperate rainforests in the 1970s and1980s in widely separated areas in temperate forests of SSA and the southwest Pacific yielded a general model of how long-lived pioneer tree species that are shade-intolerant can dominate extensive areas of forest following coarse-scale tectonically related disturbances (Veblen & Ashton Citation1978; Veblen et al. Citation1980, Citation1981; Read & Hill Citation1988; Read et al. Citation1990; Veblen et al. Citation1996a). During long periods lacking coarse-scale disturbance by tectonism or wind storms, these long-lived pioneer species are gradually replaced by more shade-tolerant species but in some regions, coarse-scale disturbances are frequent enough to maintain dominance by the shade-intolerant trees. The role of tectonic disturbances in shaping forest structures and selecting disturbance-related traits in rainforests of southern Chile and New Zealand may be comparable to the ecological and evolutionary influences of fire in some other temperate forest biomes.
Studies of tectonic influences on the dynamics of southern temperate rainforests of Chile and New Zealand published in the 1970s and early 1980s contributed prominently to the shift away from Clements’ climax theory and towards modern non-equilibrium frameworks of forest dynamics (Sousa Citation1984; Attiwill Citation1994). The recognition that in temperate forests in SSA and New Zealand cohort structures, triggered by exogenous disturbances such as wind storms and tectonic events, are the norm rather than all-aged structures has been of fundamental importance to understanding the dynamics of these forests (Veblen & Ashton Citation1978; Ogden Citation1985; Ogden et al. Citation1996, Ogden et al. Citation2005; Wells et al. Citation2001; Lara et al. Citation2014a). This recognition promoted new interpretations of forest structures that previously had been ascribed to climate-caused regeneration failures, ‘relict’ status of some tree species, or to impacts of introduced browsing animals (Veblen & Stewart Citation1982a, Citation1982b; Ogden Citation1985; Wilson & Lee Citation2012). Adoption and refinement of a non-equilibrium patch dynamics framework for interpreting forest structure and dynamics in the 1980s was a fundamental advancement in refining older equilibrium-infused views of southern temperate forest dynamics.
Acknowledgements
We thank CH Lusk, BD Clarkson, and one anonymous reviewer for helpful comments on the draft.
Associate Editor: Dr Chris Lusk.
Disclosure statement
No potential conflict of interest was reported by the authors.
Additional information
Funding
References
- Adams J. 1980. Palaeseismicity of the Alpine fault seismic gap, New Zealand. Geology. 8:72–76. doi: 10.1130/0091-7613(1980)8<72:POTAFS>2.0.CO;2
- Adie H, Lawes MJ. 2009. Explaining conifer dominance in Afrotemperate forests: shade tolerance favours Podocarpus latifolius over angiosperm species. Forest Ecol Manag. 259:176–186. doi: 10.1016/j.foreco.2009.10.006
- Allen RB, Bellingham PJ, Wiser SK. 1999. Immediate damage by an earthquake to a temperate montane forest. Ecology. 80:708–714. doi: 10.1890/0012-9658(1999)080[0708:IDBAET]2.0.CO;2
- Armesto JJ, Figueroa J. 1987. Stand structure and dynamics in the temperate rain forests of Chiloé Archipelago, Chile. J Biogeogr. 14:367–376. doi: 10.2307/2844944
- Armesto JJ, Fuentes ER. 1988. Tree species regeneration in a mid-elevation, temperate rain forest in Isla de Chiloé, Chile. Vegetatio. 74:151–159. doi: 10.1007/BF00044740
- Attiwill PM. 1994. The disturbance of forest ecosystems: the ecological basis for conservation management. Forest Ecol Manag. 63:247–300. doi: 10.1016/0378-1127(94)90114-7
- Bachelor CL. 1983. The possum and rata-kamahi dieback in New Zealand: a review. Pac Sci. 37:415–426.
- Brun R. 1975. Estructura y potencialidad de distintos tipos de bosque nativo en el sur de Chile. Bosque. 1:6–17. doi: 10.4206/bosque.1975.v1n1-03
- Burrows CJ. 1990. Processes of vegetation change. London: Unwin Wyman.
- Casertano L. 1963. General characteristics of active Andean volcanoes and summary of their activities during recent centuries. B Seismol Soc Am. 53:1415–1433.
- Chaneton EJ, Mazía N, Garibaldi LA, Chaij J, Kitzberger T. 2014. Impact of volcanic ash deposition on foliar productivity and insect herbivory in northern Patagonia deciduous forests. Ecología Austral. 24:51–63.
- Chavasse CGR. 1955. Mortality in rata/kamahi protection forests, Westland [Unpublished report]. Wellington: New Zealand Forest Service.
- Cisternas M, Atwater BF, Torrejón F, Sawai Y, Machuca G, Lagos M, Eipert A, Youlton C, Salgado I, Kamataki T, Shishikura M, Rajendran M, Malik CP, Rizal Y, Husni M. 2005. Predecessors of the giant 1960 Chile earthquake. Nature. 437:404–407. doi: 10.1038/nature03943
- Clarkson BD. 1990. A review of vegetation development following recent (<450 years) volcanic disturbance in North Island, New Zealand. New Zeal J Ecol. 14:59–71.
- Clarkson BR, Clarkson BD, Patel RN. 1992. The pre-Taupo eruption (c. AD 130) forest of the Bennydale-Pureora district, central North Island, New Zealand. J Roy Soc New Zeal. 22:61–76. doi: 10.1080/03036758.1992.10420806
- Clarkson BR, Clarkson BD. 1995. Recent vegetation changes on Mount Tarawera, Rotorua, New Zealand. New Zeal J Bot. 33:339–354. doi: 10.1080/0028825X.1995.10412961
- Clarkson BD, Clarkson BR, McGlone MS. 1986. Vegetation history of some West Taupo mires. In: Veale B, Innes J compilers editors. Ecological research in the central North Island volcanic plateau region. Proceedings of a New Zealand Forest Service Workshop. Rotorua: Forest Research Institute; p. 34–37.
- Clarkson BR, Patel RN, Clarkson BD. 1988. Composition and structure of forest overwhelmed at Pureora, central North Island, New Zealand, during the Taupo eruption (c. AD 130). J Roy Soc New Zeal. 18:417–436. doi: 10.1080/03036758.1988.10426466
- Clarkson BR, Walker LR, Clarkson BD, Silvester WB. 2002. Impact of Coriaria arborea on seed banks during primary succession on Mt Tarawera, New Zealand. New Zeal J Bot. 40:629–638. doi: 10.1080/0028825X.2002.9512819
- Clements FE. 1916. Plant succession: an analysis of the development of vegetation. Washington, DC: Carnegie Institution.
- Cullen LE, Duncan RP, Wells A, Stewart GH. 2003. Floodplain and regional scale variation in earthquake effects on forests, Westland, New Zealand. J Roy Soc New Zeal. 33:693–701. doi: 10.1080/03014223.2003.9517753
- Daniels LD, Veblen TT. 2003. Regional and local effects of disturbance and climate on altitudinal treelines in northern Patagonia. J Veg Sci. 14:733–742. doi: 10.1111/j.1654-1103.2003.tb02205.x
- Daniels LD, Veblen TT. 2004. Spatio–temporal influences of climate on altitudinal treeline in Northern Patagonia. Ecology. 85:1284–1296. doi: 10.1890/03-0092
- Donoso C, Escobar B, Urrutia J. 1985. Estructura y estrategias regenerativas de un bosque virgen de Ulmo (Eucryphia cordifolia Cav.) - Tepa (Laurelia philippiana Phil.) Looser en Chiloé, Chile. Revist Chilena de Historia Natural. 58:171–186.
- Donoso C, Grez R, Escobar B, Real P. 1984. Estructura y dinámica de bosques del tipo forestal siempreverde en un sector de Chiloé Insular. Bosque. 5:82–104. doi: 10.4206/bosque.1984.v5n2-04
- Donoso C, Sandoval V, Grez R, Rodríguez J. 1993. Dynamics of Fitzroya cupressoides forests in southern Chile. J Veg Sci. 4:303–312. doi: 10.2307/3235588
- Donoso PJ, Donoso C, Navarro C. 2014. Manejo de Ecosistemas Forestales. In: Donoso C, González ME, Lara A, editors. Ecología Forestal. Bases para el Manejo Sustentable y Conservación de los Bosques Nativos de Chile. Valdivia: Ediciones Universidad Austral de Chile; p. 505–525.
- Drury WH, Nisbet CT. 1971. Interrelations between developmental models in geomorphology, plant ecology, and animal ecology. Gen Syst. 16:57–68.
- Efford JT, Clarkson BD, Bylsma RJ. 2014. Persistent effects of a tephra eruption (AD 1655) on treeline composition and structure, Mt Taranaki, New Zealand. New Zeal J Bot. 52:245–261. doi: 10.1080/0028825X.2014.886599
- Enright NJ, Hill RS, Veblen TT. 1995. The southern conifers – an introduction. In: Enright NJ, Hill RS editors. Ecology of the southern conifers. Melbourne: Melbourne University Press; p. 1–9.
- Fernández-Martinez MA, de los Ríos A, Sancho LG, Perez-Ortega S. 2013. Diversity of endosymbiotic Nostoc in Gunnera magellanica (L) from Tierra del Fuego, Chile. Microb Ecol. 66:335–350. doi: 10.1007/s00248-013-0223-2
- Ghermandi L, González S. 2012. Observaciones tempranas de la deposición de ceniza por la erupción volcánica del Cordón Caulle y sus consecuencias sobre la vegetación de la estepa del noroeste de la Patagonia. Ecología Austral. 22:144–149.
- Glenn-Lewin DC, Peet RK, Veblen TT, editors. 1992. Plant succession: theory and prediction. London, UK: Chapman and Hall.
- Goff J, Wells A, Chague-Goff C, Nichol SL, Devoy RJN. 2004. The elusive 1826 tsunami, south Westland, New Zealand. New Zeal Geogr. 60:28–39. doi: 10.1111/j.1745-7939.2004.tb01710.x
- González ME, Amoroso M, Lara A, et al. 2014. Ecología de disturbios y su influencia en los bosques templados de Chile y Argentina. In: Donoso C, González ME, Lara A, editors. Ecología Forestal. Bases para el Manejo Sustentable y Conservación de los Bosques Nativos de Chile. Valdivia: Ediciones Universidad Austral de Chile; p. 411–502.
- Goodall RNC. 1979. Tierra del Fuego. Buenos Aires: Ediciones Shanamaiim.
- Gutiérrez A, Armesto JJ, Aravena JC. 2004. Disturbance and regeneration dynamics of an old-growth north Patagonian rain forest in Chiloé Island, Chile. J Ecol. 92:598–608. doi: 10.1111/j.0022-0477.2004.00891.x
- Herbert JW. 1980. Structure and growth of dense podocarp forest at Tihoi, central North Island, and the impact of selective logging. New Zeal J Forest. 25:44–57.
- Hogg A, Lowe DJ, Palmer J, Boswijk G, Ramsey CB. 2012. Revised calendar date for the Taupo eruption derived by 14C wiggle-matching using a New Zealand kauri 14C calibration data set. The Holocene. 22:439–449. doi: 10.1177/0959683611425551
- Holloway JT. 1957. Charles Douglas—observer extraordinary. New Zeal J Forest. 4:35–40.
- Irisarri JG, Oesterheld M, Paruelo J, Baldassini P, Arocena D, Oyarzabal M. 2012. Impacto de la erupción del volcán Puyehue y el déficit de precipitaciones sobre la producción de materia seca del Suroeste de Río Negro. LART—Laboratorio de Análisis Regional y Teledetección, IFEVA-FAUBA-CONICET Report Buenos Aires; Universidad de Buenos Aires.
- Jara IA, Moreno PI. 2012. Temperate rainforest response to climate change and disturbance agents in northwestern Patagonia (41°S) over the last 2600 years. Quat Res. 77:235–244. doi: 10.1016/j.yqres.2011.11.011
- Kitzberger T, Veblen TT, Villalba R. 1995. Tectonic influences on tree growth in northern Patagonia, Argentina: the roles of substrate stability and climatic variation. Can J For Res. 25:1684–1696. doi: 10.1139/x95-182
- Lara A. 1991. The dynamics and disturbance regimes of Fitzroya cupressoides forest in south-central Andes of Chile [unpublished PhD thesis]. Boulder, CO: University of Colorado.
- Lara A, Amoroso M, Bannister J, et al. 2014a. Sucesión y dinámica de bosques templados en Chile. In: Donoso C, González ME, Lara A, editors. Ecología Forestal. Bases para el Manejo Sustentable y Conservación de los Bosques Nativos de Chile. Valdivia: Ediciones Universidad Austral de Chile; p. 323–408.
- Lara A, Little C, Cortés M, et al. 2014b. Restauración de ecosistemas forestales. In: Donoso C, González ME, Lara A, editors. Ecología Forestal. Bases para el Manejo Sustentable y Conservación de los Bosques Nativos de Chile. Valdivia: Ediciones Universidad Austral de Chile; p. 605–672.
- Larraín OE. 1997. Establecimiento de coigüe [Nothofagus dombeyi [Mirb]. Oerst] en deslizamientos de tierra en el sector de Pilmaiquén, X Región. Valdivia, Chile: Facultad de Ciencias Forestales, Universidad Austral de Chile.
- Leathwick JR, Mitchell ND. 1992. Forest pattern, climate and vulcanism in central North Island, New Zealand. J Veg Sci. 3:603–616. doi: 10.2307/3235827
- Lusk C, Ogden J. 1992. Age structure and dynamics of a podocarp-broadleaf forest in Tongariro National Park, New Zealand. J Ecol. 80:379–393. doi: 10.2307/2260684
- Lusk CH. 1996a. Gradient analysis and disturbance history of temperate rain forests of the coast range summit plateau. Rev Chil Hist Nat. 69:401–411.
- Lusk CH. 1996b. Stand dynamics of shade-tolerant conifers Podocarpus nubigena and Saxegothaea conspicua in Chilean temperate rain forest. J Veg Sci. 7:549–558. doi: 10.2307/3236304
- Lusk CH. 1999. Long-lived light-demanding emergent in southern temperate forests: the case of Weinmannia trichosperma (Cunoniaceae) in Chile. Plant Ecol. 140:111–115. doi: 10.1023/A:1009764705942
- Lusk CH, Jorgensen MA, Bellingham PJ. 2015. A conifer–angiosperm divergence in the growth vs. shade tolerance trade-off underlies the dynamics of a New Zealand warm-temperate rain forest. J Ecol. 120:479–488. doi: 10.1111/1365-2745.12368
- Lusk CH, del Pozo A. 2002. Survival and growth of seedlings of 12 Chilean rainforest trees in two light environments: gas exchange and biomass distribution correlates. Austral Ecol. 27:173–182. doi: 10.1046/j.1442-9993.2002.01168.x
- McGlone MS. 1983. Polynesian deforestation of New Zealand: a preliminary synthesis. Archaeol Ocean. 18:11–25.
- McKelvey PJ. 1963. The synecology of the West Taupo indigenous forest. New Zealand Forest Service Bulletin No. 14. Wellington: RE Owen, Government Printer.
- McKelvey PJ. 1973. The pattern of the Urewera forests. New Zealand Forest Research Institute Technical Paper No. 59. Rotorua: Forest Research Institute, New Zealand Forest Service.
- Morales CI, Saez A, Arbetman MP, Cavallero L, Aizen MA. 2014. Detrimental effects of volcanic ash deposition on bee fauna and plant-pollinator interactions. Ecología Austral. 24:42–50.
- Ogden J. 1985. An introduction to plant demography with special reference to New Zealand trees. New Zeal J Bot. 23:751–772. doi: 10.1080/0028825X.1985.10434241
- Ogden J, Fordham RA, Horrocks M, Pilkington S, Serra RG. 2005. Long-term dynamics of the long-lived conifer Libocedrus bidwillii after a volcanic eruption 2000 years ago. J Veg Sci. 16:321–330.
- Ogden J, Stewart GH, Allen RB. 1996. The ecology of New Zealand forests. In: Veblen TT, Hill RS, Read J, editors. The ecology and biogeography of Nothofagus forests. New Haven, CT: Yale University Press; p. 25–82.
- Oyarzabal M, Paruelo J, Baldassini P, Aguiar S. 2011. Impacto de la erupción del volcán Puyehue sobre la productividad forrajera de Chubut. LART - Laboratorio de Análisis Regional y Teledetección. IFEVA-FAUBA-CONICET, Universidad de Buenos Aires, Buenos Aires.
- Paine RT, Tegner MJ, Johnson EA. 1998. Compounded perturbations yield ecological surprises. Ecosystems. 1:535–545. doi: 10.1007/s100219900049
- Pickett STA, Collins SL, Armesto JJ. 1987. Models, mechanisms and pathways of succession. Bot Rev. 53:335–371. doi: 10.1007/BF02858321
- Pollmann W. 2004. Regeneration dynamics and life history differences in southern Chilean Nothofagus forests: a synthesis. Plant Ecol. 174:355–371. doi: 10.1023/B:VEGE.0000049114.58663.6d
- Pollmann W, Veblen TT. 2004. Nothofagus regeneration dynamics in south-central Chile: a test of a general model. Ecol Monogr. 74:615–634. doi: 10.1890/04-0004
- Read J, Hill RS. 1988. The dynamics of some rainforest associations in Tasmania. J Ecol. 76:558–584. doi: 10.2307/2260613
- Read J, Hope G, Hill R. 1990. The dynamics of some Nothofagus-dominated rain forests in Papua New Guinea. J Biogeogr. 17:185–204. doi: 10.2307/2845326
- Rosas G. 1991. Estudio de la vegetación leñosa en áreas afectadas por deslizamientos en el Parque Nacional Puyehue, Osorno, Décima Región. Tesis de Ingeniería Forestal. Valdivia, Chile: Facultad de Ciencias Forestales, Universidad Austral de Chile; p. 82.
- Rose AB, Pekelharing CJ, Platt KH. 1992. Magnitude of canopy dieback and implications for conservation of southern rata-kamahi (Metrosideros umbellata-Weinmannia racemosa) forests, central Westland, New Zealand. New Zeal J Ecol. 16:23–32.
- Rothkugel M. 1916. Los Bosques Patagónicos. Buenos Aires, Argentina: Ministerio de Agricultura; p. 207.
- Ruggiero A, Kitzberger T. 2014. Special section – Ecological responses of arthropods to volcanism. Ecología Austral. 24:1–2.
- Simard M, Romme WH, Griffin JM, Turner MG. 2011. Do mountain pine beetle outbreaks change the probability of active crown fire in lodgepole pine forests? Ecol Monogr. 81:3–24. doi: 10.1890/10-1176.1
- Sousa WP. 1984. The role of disturbance in natural communities. Ann Rev Ecol Syst. 15:353–391. doi: 10.1146/annurev.es.15.110184.002033
- Stewart GH. 2002. Structure and canopy tree species regeneration requirements in indigenous forests, Westland, New Zealand. Wellington: DOC Science Internal Series 66, Department of Conservation; 37p.
- Stewart GH, Rose AB. 1988. Factors predisposing rata-kamahi (Metrosideros umbellata-Weinmannia racemosa) forests to canopy dieback, Westland, New Zealand. Geojournal. 17:217–223. doi: 10.1007/BF02432925
- Stewart GH, Rose AB. 1989. Conifer regeneration failure in New Zealand: dynamics of montane Libocedrus bidwillii stands. Vegetatio. 79:41–49. doi: 10.1007/BF00044847
- Stewart GH, Veblen TT. 1982a. Regeneration patterns in southern rata (Metrosideros umbellata)/kamahi (Weinmannia racemosa) forest in central Westland, New Zealand. New Zeal J Bot. 20:55–72. doi: 10.1080/0028825X.1982.10426404
- Stewart GH, Veblen TT. 1982b. A commentary on canopy tree mortality in Westland rata-kamahi protection forests. New Zeal J Forest. 27:168–188.
- Stewart GH, Veblen TT. 1983. Forest instability and canopy tree mortality in Westland, New Zealand. Pac Sci. 37:427–431.
- Swanson FJ, Jones JA, Crisafulli CM, Lara A. 2013. Effects of volcanic and hydrologic processes on forest vegetation: Chaitén volcano, Chile. Andean Geol. 40:359–391.
- Swanson FJ, Major JJ. 2005. Physical environments, events, and geological-ecological interactions at Mount St. Helens–March 1980–2004. In: Dale VH, Swanson FJ, Crisafulli CM, editors. Ecological responses to the 1980 eruption of Mount St. Helens. New York, NY: Springer-Verlag; p. 27–44.
- Uhe G. 1972. A contribution to the ecology of vegetation on recent volcanic deposits [unpublished PhD thesis]. Auckland: University of Auckland.
- Urlich S, Stewart GH, Duncan RP, Almond PC. 2005. Tree regeneration in a New Zealand rain forest influenced by disturbances and drainage interactions. J Veg Sci. 16:423–432. doi: 10.1111/j.1654-1103.2005.tb02382.x
- Veblen TT. 1982. Regeneration patterns in Araucaria araucana forests in Chile. J Biogeogr. 9:11–28. doi: 10.2307/2844727
- Veblen TT. 1985. Forest development in treefall gaps in the temperate rain forests of Chile. National Geogr Res. 1:162–183.
- Veblen TT. 1989a. Nothofagus regeneration in treefall gaps in northern Patagonia. Can J Forest Res. 19:365–371. doi: 10.1139/x89-055
- Veblen TT. 1989b. Tree regeneration responses to gaps along a transandean gradient. Ecology. 70:541–545. doi: 10.2307/1940197
- Veblen TT, Ashton DH. 1978. Catastrophic influences on the vegetation of the Valdivian Andes, Chile. Vegetatio. 36:149–167. doi: 10.1007/BF02342598
- Veblen TT, Ashton DH, Rubulis S, Lorenz DC, Cortes M. 1989. Nothofagus stand development on in-transit moraines, Casa Pangue Glacier, Chile. Arct Alp Res. 21:144–155. doi: 10.2307/1551626
- Veblen TT, Ashton DH, Schlegel FM, Veblen AT. 1977. Plant succession in a timberline depressed by vulcanism in south-central Chile. J Biogeogr. 4:275–294. doi: 10.2307/3038061
- Veblen TT, Ashton DH, Schlegel ME. 1979. Tree regeneration strategies in a lowland Nothofagus dominated forest in south–central Chile. J Biogeogr. 6:329–340. doi: 10.2307/3038085
- Veblen TT, Donoso C, Kitzberger T, Rebertus AJ. 1996b. Ecology of southern Chilean and Argentinean Nothofagus forests. In: Veblen TT, Hill RS, Read J, editors. The ecology and biogeography of Nothofagus forests. New Haven, CT: Yale University Press; p. 293–353.
- Veblen TT, Donoso C, Schlegel FM, Escobar B. 1981. Forest dynamics in south-central Chile. J Biogeogr. 8:211–247. doi: 10.2307/2844678
- Veblen TT, Hill RS, Read J. 1996a. Introduction: themes and concepts in the study of Nothofagus forests. In: Veblen TT, Hill RS, Read J, editors. The ecology and biogeography of Nothofagus forests. New Haven, CT: Yale University Press; p. 1–10.
- Veblen TT, Kitzberger T, Lara A. 1992. Disturbance and forest dynamics along a transect from Andean rain forest to Patagonian shrublands. J Veg Sci. 3:507–520. doi: 10.2307/3235807
- Veblen TT, Schlegel FM, Escobar B. 1980. Structure and dynamics of old-growth Nothofagus forest in the Valdivian Andes, Chile. J Ecol. 68:1–31. doi: 10.2307/2259240
- Veblen TT, Stewart GH. 1982a. On the conifer regeneration gap in New Zealand: the dynamics of Libocedrus bidwillii stands on South Island. J Ecol. 70:413–436. doi: 10.2307/2259912
- Veblen TT, Stewart GH. 1982b. The effects of introduced wild animals on New Zealand forests. Ann Assoc Am Geogr. 72:372–397. doi: 10.1111/j.1467-8306.1982.tb01832.x
- Vittoz P, Stewart GH, Duncan RP. 2001. Earthquake impacts in old-growth Nothofagus forests, New Zealand. J Veg Sci. 12:417–426. doi: 10.2307/3236856
- Vogel A, Hildebrand-Vogel R, Godoy R. 1994. Auswirkungen eines Aschefalls auf die Vegetation eines Südbuchenwaldes in Westpatagonien. Verhandlungen der Gesellschaft für Ökologie. 23:177–186.
- Walker LR, Clarkson BD, Silvester WB, Clarkson BR. 2003. Colonization dynamics and facilitative impacts of a nitrogen-fixing shrub in primary succession. J Veg Sci. 14:277–290. doi: 10.1111/j.1654-1103.2003.tb02153.x
- Wardle P. 1966. Biological flora of New Zealand.1. Weinmannia racemosa Linn.f. (Cunoniaceae). Kamahi. New Zeal J Bot. 4:114–131. doi: 10.1080/0028825X.1966.10443958
- Wardle P. 1971. Biological flora of New Zealand. 6. Metrosideros umbellata Cav. [Syn. M. lucida (Forst. F.) A. Rich.] (Myrtaceae). Southern rata. New Zeal J Bot. 9:645–671. doi: 10.1080/0028825X.1971.10430227
- Wardle P. 1980. Primary succession in Westland National Park and its vicinity New Zealand. New Zeal J Bot. 18:221–232. doi: 10.1080/0028825X.1980.10426921
- Wells A, Duncan RP, Stewart GH. 2001. Forest dynamics in Westland, New Zealand: the importance of large, infrequent earthquake-induced disturbance. J Ecol. 89:1006–1018. doi: 10.1111/j.1365-2745.2001.00594.x
- Wells A, Goff J. 2006. Coastal dune ridge systems as chronological markers of palaeoseismic activity: a 650 year record from southwest New Zealand. The Holocene. 16:543–550. doi: 10.1191/0959683606hl949rp
- Wells A, Stewart GH, Duncan RP. 1998. Evidence of widespread, synchronous, disturbance-initiated forest establishment in Westland, New Zealand. J Roy Soc New Zeal. 28:333–345. doi: 10.1080/03014223.1998.9517569
- Wells A, Yetton MD, Duncan RP, Stewart GH. 1999. Prehistoric dates of the most recent Alpine Fault earthquakes, New Zealand. Geology. 27:995–998. doi: 10.1130/0091-7613(1999)027<0995:PDOTMR>2.3.CO;2
- White PS. 1979. Pattern, process and natural disturbance in vegetation. Bot Rev. 45:229–299. doi: 10.1007/BF02860857
- White PS, Jentsch A. 2001. The search for generality in studies of disturbance and ecosystem dynamics. Progr Bot. 62:3994–50.
- Wilmshurst JM, McGlone MS. 1996. Forest disturbance in the central North Island, New Zealand, following the 1850 BP Taupo eruption. The Holocene. 6:399–411. doi: 10.1177/095968369600600402
- Wilson JB, Lee WG. 2012. Is New Zealand vegetation really ‘problematic’? Dansereau’s puzzles revisited. Biol Rev. 87:367–389. doi: 10.1111/j.1469-185X.2011.00202.x
- Wright C, Mella A. 1963. Modifications to the soil pattern of South-Central Chile resulting from seismic and associated phenomena during the period May to August 1960. B Seismol Soc Am. 53:1367–1402.