ABSTRACT
Isolepis R.Br. (Cyperaceae) is a genus of aquatic, wetland, or ephemeral sedges that comprises 76 species, mostly in the southern hemisphere, especially Africa and Australasia. Isolepis crassiuscula Hook.f., Isolepis lenticularis R.Br. and Isolepis producta (C.B. Clarke) K.L.Wilson are among the Australasian members of a morphologically well-defined subgenus Fluitantes. Specimens belonging to this subgenus, collected from Mount Tongariro, New Zealand, showed ambiguous yet somewhat intermediate morphology compared with these three species. We sequenced directly, or cloned and sequenced, nuclear DNA (nrITS) and chloroplast DNA (rbcL, rps16 and trnL) regions of these collections and samples of the three aforementioned species of subgenus Fluitantes. Ribotypes and chloroplast haplotypes were compared to resolve if the Tongariro collection was of hybrid origin and, if so, to address which of the three species may have been involved in the hybridisation event. The Tongariro plants had the species-specific ribotypes of I. crassiuscula and I. lenticularis and the chloroplast haplotype of I. lenticularis, strongly suggesting hybrid origin between them. No data support the inclusion of I. producta as a parent. The morphologically uniform population from which the Tongariro plants were collected is likely to be derived from a single hybridisation event, although this hypothesis merits further investigation.
Introduction
Hybridisation is recognised as a widespread and important mode of evolution in plants (e.g. Rieseberg Citation1995; Yakimowski & Rieseberg Citation2014). However, spontaneous hybridisation is not distributed evenly across plant groups, but seems to be more common in taxa that have a perennial life cycle, outcrossing breeding systems, and the possibility of clonal reproduction (Ellstrand et al. Citation1996). Cases of interspecific hybridisation are reported in the sedge family (Cyperaceae), especially in the genus Carex L. (e.g. Smith & Waterway Citation2008; Volkova et al. Citation2008; Gizaw et al. Citation2016), yet the frequency and extent of hybridisation in the other genera of the family has been only partially explored (Košnar et al. Citation2010; Yano et al. Citation2010).
Isolepis R.Br. (Cyperaceae) is a genus of aquatic, wetland or ephemeral sedges that comprises 76 species mostly in the southern hemisphere, especially Africa and Australasia (Australia and New Zealand) (Muasya & Simpson Citation2002; Govaerts et al. Citation2011). This perennial, clonal genus (with unknown reproductive system) includes putative hybrids in New Zealand: Isolepis aucklandica Hook.f. × Isolepis cernua (Vahl) Roem & Schult., Isolepis distigmatosa (C.B.Clarke) Edgar × Isolepis prolifera (Rottb.) R.Br. and Isolepis inundata R.Br. × I. prolifera (Edgar Citation1970). In Africa, Gordon-Gray (Citation1995) hypothesised that ‘Isolepis angelica B.L.Burtt may have arisen by … interspecific hybridisation’ because of its morphology that combines the characters of Isolepis fluitans (L.) R.Br. and Isolepis setacea (L.) R.Br., without discussing whether I. angelica is a homoploid hybrid, allotetraploid, or a hybrid species (Gordon-Gray Citation1995). No data suggesting that allopolyploid have occurred in Isolepis.
Subgenus Fluitantes is one of the four subgenera of Isolepis and is a group disjunctively distributed in Africa and Australasia that includes, among other species, Isolepis crassiuscula Hook.f., Isolepis lenticularis R.Br. (formerly I. fluitans var. lenticularis (R.Br.) Muasya) and Isolepis producta (C.B.Clarke) K.L.Wilson (Muasya & Simpson Citation2002; Ito et al. Citation2016). These three species are characterised by the morphological characters ‘involucral bract shorter than spikelet’, ‘spikelet always terminal, bract shorter or as long as spikelet’ and ‘style always bifid’, and are distinguishable from one another by the morphological features of the spikelets, anthers and fruit (Ito et al. Citation2016; ). The habitat preferences of these species are similar in Australasia, though no evidence is available that they occur synpatrically, as, for instance, Wilson (Citation1994) mentions that ‘ … I. crassiuscula occurs at higher altitudes than either of those species (I. producta and I. lenticularis (I. fluitans in Wilson Citation1994))’: I. crassiuscula grows in high-altitude bogs, at water margins or submerged, at 700–3700 m altitude; I. lenticularis in shallow pools and seepages at c. 800 m altitude (I. fluitans var. lenticularis in Muasya & Simpson Citation2002); I. producta in ponds at 800–1400 m in altitude (Muasya & Simpson Citation2002). We recently made collections of Isolepis subgenus Fluitantes from a single population of c. 2 m × 30 cm clumped in a small stream at c. 1100 m in altitude in Mount Tongariro in New Zealand that show ambiguous yet somewhat intermediate morphology among these three species, although I. producta does not occur in New Zealand (, ). Because natural hybrids often display intermediate morphology between putative parental taxa (Rieseberg et al. Citation2000), and this also applies to Cyperaceae hybrids (Košnar et al. Citation2010; Yano et al. Citation2010), this could be a product of inter-specific hybridisation among the morphologically and ecologically closely related species. This hypothesis does not disagree with the fact that our Mt Tongariro Isolepis collections at least do not set mature fruits but only immature and apparently malformed ones. Molecular markers have been proven useful in detecting hybrids and determining the parents (Soltis et al. Citation1992). For this purpose, the internal transcribed spacer (ITS) region of nuclear ribosomal (nr) DNA (hereinafter called nrITS) is of particular merit because this can provide evidence of reticulate evolution when the hybrid retains multiple sequence copies contributed by each parent (Baldwin et al. Citation1995; Soltis et al. Citation2008), and numerous studies have confirmed hybrid origin using nrITS (e.g. Sang et al. Citation1995; Campbell et al. Citation1997; Fuertes et al. Citation1999; Moody & Les Citation2002; Saito et al. Citation2006; Pan et al. Citation2008; Lee et al. Citation2012). Additionally, assuming maternal inheritance of the chloroplast genome in Cyperaceae, as in the majority of plant groups (Birky Citation1995), the simultaneous usage of nrITS and chloroplast DNA (hereinafter called cpDNA) markers will clarify the direction of pollen flow in hybridisation.
Table 1. A morphological comparison of three Isolepis species and a hybrid among them in New Zealand.
The aim of this study was to test the morphology-based hypothesis that this population of Isolepis from Mt Tongariro in New Zealand is of hybrid origin. To do so, we employed DNA sequence comparison of nuclear DNA (nrITS) and cpDNA (rbcL, rps16 and trnL) and sought evidence of hybridisation, i.e. polymorphism pattern of nrITS. We further determined which of the three aforementioned species from subgenus Fluitantes were involved in the hybridisation event.
Materials and methods
Taxon sampling
The present study included two representative samples from the Isolepis population on Mt Tongariro in New Zealand inferred to be of hybrid origin (; ). Data from seven, two and two samples respectively of I. crassiuscula, I. lenticularis and I. producta from Australia, Japan and New Zealand were obtained from herbarium specimens or previously published data sets (Muasya et al. Citation2001; Hirahara et al. Citation2007a, Citation2007b; Ito et al. Citation2016). Details of these samples and voucher specimens are given in .
DNA extraction, polymerase chain reaction amplification, sequencing and molecular cloning
Total genomic DNA was extracted from either silica gel-dried leaf tissue or herbarium specimens using the CTAB method described in Ito et al. (Citation2010). The targeted DNA regions, three from cpDNA (rbcL, rps16 and trnL) and one from nuclear ribosomal DNA (nrITS), were amplified using the following primers: rbcL-1F (Fay et al. Citation1997) and rbcL-729R (5′-CTTCGCATGTACCTGCAGTAGC-3′; modified from Fay et al. Citation1997) plus rbcL-636F (Asmussen & Chase Citation2001) and rbcL-1379R (Little & Barrington Citation2003) for rbcL, ‘c’ and ‘d’ for the trnL intron (Taberlet et al. Citation1991), rpsF and rpsR2 for rps16 (Oxelman et al. Citation1997) and ITS-4 and ITS-5 for nrITS (Baldwin Citation1992). Polymerase chain reaction amplification was performed following the procedure of Ito et al. (Citation2010).
Direct sequencings of ITS showed polymorphisms in the putative Isolepis hybrid, for which, apparent multiple nrITS sequences were cloned and sequenced. Molecular cloning was performed following the procedure of Ito et al. (Citation2010). Note that no cloning was performed with I. lenticularis (AK-289724) and I. crassiuscula (AK-289487) because apparent heterogeneous sequences overlapped at a single position, which we isolated manually.
DNA sequence alignments
Sequences were aligned using CLUSTAL W (Thompson et al. Citation1994) and edited manually in BioEdit v. 7.0.9 (Hall Citation1999). The simple indel coding of Simmons & Ochoterena (Citation2000) was used to code gaps found in rps16, trnL and nrITS.
Haplotype network estimation
Unrooted haplotype networks were constructed using the TCS method (Clement et al. Citation2000) in PopArt (Leigh & Bryant Citation2015). DNA sequences were separately analysed following Ito et al. (Citation2016) who reconstructed phylogenies based on concatenated cpDNA and nrITS data sets. The cpDNA data set includes rps16 and trnL because rbcL showed no sequence variation (data not shown). Gaps were coded and used as a fifth state.
Results
Nuclear ITS comparison and ribotype network
Aligned nrITS sequences of Isolepis crassiuscula, I. lenticularis and I. producta were 656 bp in length. A total of 22 nucleotide substitutions and two indels were obtained from the 11 samples of three Isolepis species, based on which, seven species-specific nrITS ribotypes were distinguished: Ribotype A was found in I. crassiuscula from Australia and Japan; ribotypes B and C in I. crassiuscula from New Zealand; ribotypes D and E in I. lenticularis from New Zealand; ribotypes F and G in I. producta (). The three species’ ribotypes were significantly separated from one another in a network (A). Cloned heterogeneous nrITS sequences derived from the putative hybrid of Isolepis were identical to those of I. crassiuscula from New Zealand (ribotype-C) and I. lenticularis (ribotype-D), respectively (A, ).
Figure 2. Haplotype networks of three species of Isolepis sugbenus Fluitantes in Australasia and a hybrid among them. A, Nuclear internal transcribed spacer ribotype network. B, Chloroplast DNA haplotype network. Two ribotypes and a haplotype recovered from the hybrid are shown with dotted lines.
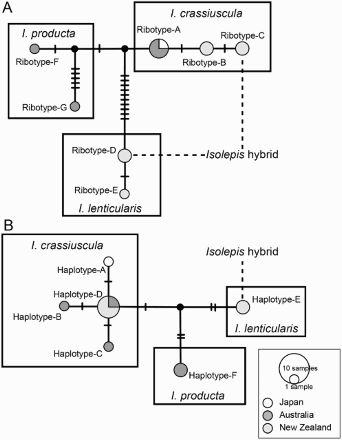
Table 2. A list of nuclear ITS ribotypes of three Isolepis species of subgenus Fluitantes and a hybrid among them. Positions of nucleotide substitution among accessions are summarised.
Chloroplast DNA comparison and haplotype network
Aligned cpDNA sequences in Isolepis crassiuscula, I. lenticularis and I. producta were 822 bp for rps16 and 665 bp for trnL in length. A total of 15 nucleotide substitutions (eight for rps16 and seven for trnL) and four indels (one for rps16 and three for trnL) were obtained from 11 samples of three Isolepis species, though rps16 was missing in four of them, and based on which, six species-specific cpDNA haplotypes were distinguished: Haplotype A was found in I. crassiuscula from Japan; haplotypes B and C in I. crassiuscula from Australia (AB823597 and LC102350/LC102354, respectively); haplotype D in I. crassiuscula from Australia (LC102349/LC102353) and New Zealand; haplotype E in I. lenticularis from New Zealand; haplotype F in I. producta (). The three species’ haplotypes were significantly separated from one another in a network (B). The rps16 and trnL sequences of the putative hybrid of Isolepis were identical to those of I. lenticularis (haplotype-E) (B, ).
Table 3. A list of chloroplast DNA haplotypes of three Isolepis species of subgenus Fluitantes in Australasia and a putative hybrid among them. Positions of nucleotide substitutions among accessions are summarised.
Discussion
The present study tested the morphology-based hypothesis that an Isolepis population we collected from Mt Tongariro in New Zealand is of hybrid origin. Three species of Isolepis subgenus Fluitantes were hypothesised to be candidates for parents of this putative hybrid. Our DNA sequence comparison and network analyses showed that two heterogeneous nrITS sequences recovered from the putative hybrid of Isolepis were identical to those which were otherwise unique to I. crassiuscula and I. lenticularis, respectively (A, ). In addition, the voucher specimens of the putative hybrid show ambiguous yet somewhat intermediate morphology (), bear no mature fruits, and have only a small number of pollen grains that look somewhat abnormal. These two lines of evidence clearly indicate that the putative hybrid of Isolepis is indeed a product of hybridisation between I. crassiuscula and I. lenticularis. Similar evidence has led to the inference of other Cyperaceae hybrids: Carex caroliniana Schwein. × Carex hirsutella Mack. (Smith & Waterway Citation2008), Eleocharis cellulosa Torr. × Eleocharis interstincta (Vahl.) Roem. et Schult. (Košnar et al. Citation2010), Schoenoplectus gemmifer C.Sato, T.Maeda & Uchino × Schoenoplectus hotarui (Ohwi) Holub (Yano et al. Citation2010), and Carex monostachya A.Rich. × Carex runssoroensis K.Schum. (Gizaw et al. Citation2016).
Chloroplast DNA is, in general, maternally inherited in flowering plants (Corriveau & Coleman Citation1988; Birky Citation1995). Our cpDNA comparison, which aimed to elucidate the direction of pollen flow in hybridisation, found a haplotype in the hybrid of Isolepis that was otherwise specific to I. lenticularis (B, ). We therefore conclude that a natural hybridisation event between paternal I. crassiuscula and maternal I. lenticularis best explains the origin of this morphologically intermediate population of Isolepis in Mt Tongariro, New Zealand. There is no indication from our data for the involvement of the other candidate species, I. producta. Molecular studies based on more detailed sampling of the population of the Isolepis hybrid will further assess whether hybridisation occurred once or twice, bidirectionally or not. However, we expect that this morphologically uniform population is most probably of a single origin.
Morgan-Richards et al. (Citation2009) reviewed cases of hybridisation in New Zealand and placed the studies into categories. The present study found no morphological evidence of fertility in Isolepis crassiuscula × I. lenticularis, as is the case with another sedge hybrid (C. caroliniana × C. hirsutella (Smith & Waterway Citation2008)). Therefore, without any evidence of the presence of a hybrid zone with the parental species, the hybridisation of Isolepis most likely represents an example of the ‘infertile’ with ‘no gene flow’ category in New Zealand (Morgan-Richards et al. Citation2009). This has also been shown for the Great Barrier Is kanuka (Kunzea Rchb.) and Manuka (Leptospermum J.R. Forst. & G. Forst.) trees (Harris et al. Citation1992). However, it could also be considered ‘infertile’ under ‘vegetative or clonal reproduction’ (Morgan-Richards et al. Citation2009) as is the case with the fern Asplenium × lucrosum Perrie & Brownsey (Perrie et al. Citation2005).
Acknowledgements
The authors thank the curators of the following herbaria for arranging loans from their institutions and/or for hospitality during our recent visits: E Cameron and D Ranatunga (AK), E Kapinos, L Csiba, T Fulcher and C Drinkell (K); C Ishii (Japan) for help with DNA sequencing. We would also like to thank MF Watson (E) for his help in checking the English of the manuscript, and AM Muasya (BOL), A Gizaw (Ethiopia), P de Lange (New Zealand), PB Pelser (CANU), KL Wilson (NSW), J Li (China) and T Hoshino (OKAY) for their continuous encouragement and support. OY and YI received a plant collecting permit for Manawatu-Wanganui, New Zealand from Department of Conservation Te Papa Atawhai (35911-FLO).
Associate Editor: Dr Leon Perrie.
Disclosure statement
No potential conflict of interest was reported by the authors.
Additional information
Funding
References
- Asmussen CB, Chase MW. 2001. Coding and noncoding plastid DNA in palm systematics. Am J Bot. 88:1103–1117. doi: 10.2307/2657094
- Baldwin BG. 1992. Phylogenetic utility of the internal transcribed spacers of nuclear ribosomal DNA in plants: an example from the compositae. Mol Phylogenet Evol. 1:3–16. doi:10.1016/1055-7903(92)90030-K
- Baldwin BG, Campbell CS, Porter JM, Sanderson MJ, Wojciechowski MF, Donoghue MJ. 1995. Utility of nuclear ribosomal DNA internal transcribed spacer sequences in phylogenetic analysis of angiosperms. Ann Mo Bot Gard. 82:247–277. doi: 10.2307/2399880
- Birky CW Jr. 1995. Uniparental inheritance of mitochondrial and chloroplast genes: mechanisms and evolution. Proc Natl Acad Sci USA. 92:11331–11338. doi: 10.1073/pnas.92.25.11331
- Campbell CS, Wojciechowski MF, Baldwin BG, Alice LA, Donoghue MJ. 1997. Persistent nuclear ribosomal DNA sequence polymorphism in the Amelanchier agamic complex (Rosaceae). Mol Biol Evol. 14:81–90. doi: 10.1093/oxfordjournals.molbev.a025705
- Clement M, Posada D, Crandall KA. 2000. TCS: a computer program to estimate gene genealogies. Mol Ecol. 9:1657–1659. doi: 10.1046/j.1365-294x.2000.01020.x
- Corriveau JL, Coleman AW. 1988. Rapid screening method to detect potential biparental inheritance of plastid DNA and results for over 200 angiosperm species. Am J Bot. 75:1443–1458. doi: 10.2307/2444695
- Edgar E. 1970. Cyperaceae. In: Moore LB, Edgar E, editor. Flora of New Zealand. Vol. II. Indigenous trachyophyta. monocotyledones except Gramineae. Wellington, ShearerAR: Government Printer. p. 167–285.
- Ellstrand NC, Whitkus RW, Rieseberg LH. 1996. Distribution of spontaneous plant hybrids. Proc Natl Acad Sci USA. 93:5090–5093. doi: 10.1073/pnas.93.10.5090
- Fay MF, Swensen SM, Chase MW. 1997. Taxonomic affinities of Medusagyne oppositifolia (Medusagynaceae). Kew Bulletin. 52:111–120. doi:10.2307/4117844
- Fuertes J, Rosselló JA, Nieto G. 1999. Nuclear ribosomal DNA (nrDNA) concerted evolution in natural and artificial hybrids of Armeria (Plumbaginaceae). Mol Ecol. 8:1341–1346. doi: 10.1046/j.1365-294X.1999.00690.x
- Gizaw A, Wondimu T, Mugizi TF, Masao CA, Abdi AA, Popp M, Ehrich D, Nemomissa S, Brochmann C. 2016. Vicariance, dispersal, and hybridization in a naturally fragmented system: the afro-alpine endemics Carex monostachya and C. runssoroensis (Cyperaceae). Alpine Bot. 126:59–71. doi:10.1007/s00035-015-0162-2
- Gordon-Gray KD. 1995. Cyperaceae in Natal. Pretoria: National Botanical Institute.
- Govaerts R, Koopman J, Simpson DA, Goetghebeur P, Wilson KL, Egorova T, Bruhl JJ. 2011. World checklist of Cyperaceae. Kew, UK: Royal Botanic Gardens. [cited 2014 Aug 2]. Available from: http://apps.kew.org/wcsp/.
- Hall TA. 1999. BioEdit: a user-friendly biological sequence alignment editor and analysis program for Windows 95/98/NT. Nucleic Acids Symposium Series. 41:95–98.
- Harris W, Porter NG, Dawson MI. 1992. Observations on biosystematic relationships of Kunzea sinclairii and on an intergeneric hybrid Kunzea sinclairii × Leptospermum scoparium. New Zeal J Bot. 30:213–230. doi: 10.1080/0028825X.1992.10412903
- Hirahara T, Katsuyama T, Hoshino T. 2007a. Suprageneric phylogeny of Japanese Cyperaceae based on DNA sequences from chloroplast ndhF and 5.8 S nuclear ribosomal DNA. Acta Phytotax Geobot. 58:57–68.
- Hirahara T, Yano O, Hoshino T. 2007b. Chromosome observations and chloroplast DNA sequences of a Japanese endangered species, Isolepis crassiuscula Hook. f. (Cyperaceae). Bunrui. 7:23–30. (in Japanese).
- Ito Y, Ohi-Toma T, Murata J, Tanaka N. 2010. Hybridization and polyploidy of an aquatic plant, Ruppia (Ruppiaceae), inferred from plastid and nuclear DNA phylogenies. Am J Bot. 97:1156–1167. doi:10.3732/ajb.0900168
- Ito Y, Viljoen J, Tanaka Nr, Yano O, Muasya AM. 2016. Phylogeny of Isolepis (Cyperaceae) revisited: Non-monophyletic nature of I. fluitans sense lato and resurrection of I. lenticularis. Plant Syst Evol. 302:231–238. doi:10.1007/s00606-015-1253-7
- Košnar J, Košnar J, Herbstová M, Macek P, Rejmánková E, Štech M. 2010. Natural hybridization in tropical spikerushes of Eleocharis subgenus Limnochloa (Cyperaceae): Evidence from morphology and DNA markers. Am J Bot. 97:1229–1240. doi:10.3732/ajb.1000029
- Lee CS, Kim C, Yeau SH, Lee NS. 2012. Nuclear and cpDNA sequences demonstrate spontaneous hybridization between Goodyera schlechtendaliana Rchb. f. and G. velutina Maxim. (Orchidaceae) in Jeju Island, Korea. Syst Bot. 37:356–364. doi:10.1600/036364412X635421
- Leigh JW, Bryant D. 2015. PopART: full-feature software for haplotype network construction. Methods Ecol Evol. 6:1110–1116. doi:10.1111/2041-210X.12410
- Little DP, Barrington DS. 2003. Major evolutionary events in the origin and diversification of the fern genus Polystichum (Dryopteridaceae). Am J Bot. 90:508–514. doi:10.3732/ajb.90.3.508
- Moody ML, Les DH. 2002. Evidence of hybridity in invasive watermilfoil (Myriophyllum) populations. Proc Natl Acad Sci USA. 99:14867–14871. doi:10.1073/pnas.172391499
- Morgan-Richards M, Smissen RD, Shepherd LD, Wallis GP, Hayward JJ, Chan C, Chambers GK, Chapman HM. 2009. A review of genetic analyses of hybridisation in New Zealand. J Roy Soc New Zeal. 39:15–34. doi: 10.1080/03014220909510561
- Muasya AM, Simpson DA. 2002. A monograph of the genus Isolepis R. Br. (Cyperaceae). Kew Bulletin. 57:257–362. doi:10.2307/4111111
- Muasya AM, Simpson DA, Chase MW. 2001. A phylogeny of Isolepis (Cyperaceae) inferred using plastid rbcL and trnL-F sequence data. Syst Bot. 26:343–353. doi:10.1043/0363-6445-26.2.342
- Oxelman B, Lidén M, Berglund D. 1997. Chloroplast rps16 intron phylogeny of the tribe Sileneae (Caryophyllaceae). Plant Syst Evol. 206:393–410. doi: 10.1007/BF00987959
- Pan YZ, Shi SH, Gong X, Kuroda C. 2008. A natural hybrid between Ligularia paradoxa and L. duciformis (Asteraceae, Senecioneae) from Yunnan, China. Ann Mo Bot Gard. 95:487–494. doi:10.3417/2006034
- Perrie LR, Shepherd LD, Brownsey PJ. 2005. Asplenium xlucrosum nothosp nov.: a sterile hybrid widely and erroneously cultivated as “Asplenium bulbiferum”. Plant Syst Evol. 250:243–257. doi: 10.1007/s00606-004-0239-7
- Rieseberg LH. 1995. The role of hybridization in evolution: old wine in new skins. Am J Bot. 82:944–953. doi: 10.2307/2445981
- Rieseberg LH, Baird SJE, Gardner KA. 2000. Hybridization, introgression, and linkage evolution. Plant Mol Biol. 42:205–224. doi: 10.1023/A:1006340407546
- Saito Y, Möller M, Kokubugata G, Katsuyama T, Marubashi W, Iwashina T. 2006. Molecular evidence for repeated hybridization events involved in the origin of the genus × Crepidiastrixeris (Asteraceae) using RAPDs and ITS data. Bot J Linn Soc. 151:333–343. doi:10.1111/j.1095-8339.2006.00513.x
- Sang T, Crawford DJ, Stuessy TF. 1995. Documentation of reticulate evolution in peonies (Paeonia) using internal transcribed spacer sequences of nuclear ribosomal DNA: implications for biogeography and concerted evolution. Proc Natl Acad Sci USA. 92:6813–6817. doi: 10.1073/pnas.92.15.6813
- Simmons MP, Ochoterena H. 2000. Gaps as characters in sequence-based phylogenetic analyses. Syst Biol. 49:369–381. doi:10.1093/sysbio/49.2.369
- Smith TW, Waterway MJ. 2008. Evaluating species limits and hybridization in the Carex complanata complex using morphology, amplified fragment length polymorphisms, and restriction fragment analysisThis paper is one of a selection of papers published in the Special Issue on Systematics Research. Bot. 86:809–826. doi:10.1139/B08-031
- Soltis PS, Doyle JJ, Soltis DE. 1992. Molecular data and polyploid evolution in plants. In: Soltis PS, Soltis DE, Doyle JJ, editors. Molecular systematics of plants. New York, Chapman & Hall. p. 177–201.
- Soltis DE, Mavrodiev EV, Doyle JJ, Rauscher J, Soltis P. 2008. ITS and ETS sequence data and phylogeny reconstruction in allopolyploids and hybrids. Syst Bot. 33:7–20. doi:10.1600/036364408783887401
- Taberlet P, Ludovic G, Pautou G, Bouvet J. 1991. Universal primers for amplification of three non-coding regions of chloroplast DNA. Plant Mol Biol. 17:1105–1109. doi: 10.1007/BF00037152
- Thompson JD, Higgins DG, Gibson TJ. 1994. CLUSTAL W: improving the sensitivity of progressive multiple sequence alignment through sequence weighting, position-specific gap penalties and weight matrix choice. Nucleic Acids Res. 22:4673–4680. doi: 10.1093/nar/22.22.4673
- Volkova PA, Shipunov AB, Elven R, Brochmann C. 2008. The seashore sedges of the Russian Kola Peninsula: how many species?. Flora. 203:523–533. doi: 10.1016/j.flora.2007.09.004
- Wilson KL. 1994. Cyperaceae. In: Walsh NG, Entwistle TJ, editor. Flora of victoria 2, ferns and allied plants, conifers and monocotyledons. Melbourne: Inkata Press. p. 306–321.
- Yakimowski SB, Rieseberg LH. 2014. The role of homoploid hybridization in evolution: a century of studies synthesizing genetics and ecology. Am J Bot. 101:1247–1258. doi:10.3732/ajb.1400201
- Yano O, Ikeda H, Hoshino T. 2010. Molecular and cytological studies of an interspecific hybrid in the genus Schoenoplectus (Cyperaceae). Acta Phytotax Geobot. 60:141–149.