ABSTRACT
The endemic bull kelp Durvillaea willana Lindauer grows on South and Stewart/Rakiura Islands, with a small North Island population on the northern Wairarapa coast. Drawing on field observations, the distribution of this species is mapped. Within its biogeographic range, there are three lengthy tracts of rocky shore where the kelp is conspicuously absent. These distributional gaps are: Wellington’s south coast (despite proximity to populations on the South Island side of Cook Strait), the southern and central Wairarapa Coast, and in South Westland. Theorising that these gaps have been caused by earthquake-driven coastal uplift, this paper examines the effects on Durvillaea species of a 7.8 Mw earthquake in 2016, which raised around 100 km of seashore along the Kaikōura coast, plus the historical evidence of uplift along the Akatore Fault, south of Dunedin. This evidence suggests that the requirements for such local extinctions are that the uplift is consistently higher than the kelp’s vertical range, and that it occurs along an entire rocky coastal tract flanked by barriers to recolonisation such as long beaches or deep ocean–major obstacles to a non-buoyant kelp with poor dispersive ability.
Introduction
Barriers to New Zealand seaweed dispersal have previously been hypothesised as: long sand and shingle beaches, wide river mouths, inlet entrances, ocean straits and the effect of currents on sea-surface temperatures (Laing Citation1894). When I first mapped the distribution of the shallow subtidal New Zealand bull kelp, Durvillaea willana, many of the discontinuities–distributional ‘gaps’–could be attributed to the factors described by Laing, with long expanses of shingle beach being particularly obvious (Hay Citation1977).
Other factors affecting kelp distributions are heatwaves and regional changes in ‘wave climate’. At Tautuku Peninsula, southeast Otago (site 43, ), intertidal Durvillaea antarctica (Chamisso) Hariot and the higher-zoned D. poha Fraser Spencer and Waters are periodically sun-burned. This causes sheets of surface cortical cells (4 cells deep) to peel off the blades, although new surface cells soon form (Hay Citation1977). Further north, however, there have been recent reports of large-scale mortality of these species during unusually hot, dry conditions, on Banks Peninsula for example (Thomsen et al. Citation2019). Such widespread mortality would result in at least temporary gaps in their distribution. Heat stress is, however, unlikely to affect D. willana, because even the shallowest plants, although briefly and partly exposed to air on low spring tides, are wetted by waves.
Figure 1. The New Zealand distribution of D. willana and places mentioned in text. Sites where D. willana has been recorded are numbered (and arrowed) 1–77. For detail, see Appendix. Areas of recent coastal uplift (Wellington’s south coast, and Kaikōura) are cross-hatched. Other areas presumed to have been uplifted (Wairarapa coast, South Westland and Akatore) are depicted with solid, oval symbols.
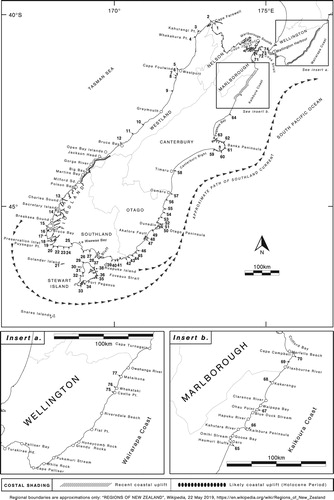
On a small spatial scale, wave climate clearly influences the horizontal distribution of species, which is why, in southern New Zealand, both D. antarctica and D. willana extend only a short distance inside harbours and bays before being replaced by other fucalean algae, particularly Xiphophora gladiata (Labill.) Mont. ex Kjellm. (pers. obs.). In a large-scale study of the community structure, diversity and biogeographic differences in dominant species around the New Zealand coast (73 sites monitored over 17 years), Schiel (Citation2011) reported that changes in wave climate (most likely in concert with the Southern Oscillation Index), rather than changing sea surface temperatures (SSTs), probably caused disturbances to fucoid canopy cover and the loss of understorey species. This significantly changed community structure, the effects lasting 2–6 years. Intervals of more severe wave climate during episodes of the El Niño Southern Oscillation pattern (ENSO) are unlikely to cause long-term major discontinuities in the distribution of D. willana, however. Although powerful storms periodically detach huge quantities of D. willana, where this happens, as on the southern Kaikōura coast, the kelp persists (pers. obs. 1972-76).
There are, however, three major gaps in the distribution of D. willana that cannot be explained by any of the aforementioned phenomena. These are: (1) the unusual absence of the kelp from apparently suitable rocky reefs and shoreline on Wellington’s south coast, west of Turakirae Head; (2) its absence from the southern and central Wairarapa coast for tens of kilometres northeast of Cape Palliser/Matakitakiakupe; and (3) from abundant rocky shoreline, for at least 100 km, in South Westland, south of Jacksons Bay. The reason for the kelp’s absence in these three areas may relate to the fact that all are prone to periodic episodes of shoreline uplift caused by earthquakes, sufficient to elevate the entire sublittoral fringe and shallow subtidal zone well above the reach of the tide (Graham Citation2015). After first describing the biology, habit and distribution of this kelp, this perspectives paper examines evidence for gaps in its distribution caused by earthquake-driven uplift.
The effect of instant uplift on species of Durvillaea, including D. willana became apparent when a 7.8 Mw earthquake on 14 November 2016 uplifted the shoreline of most of the Kaikōura coast. This highly variable uplift created new, emergent offshore reefs in places (Schiel et al. Citation2018; Alestra et al. Citation2019). It obliterated the intertidal Durvillaea species in many areas, the scorched, dying plants being used as indicators of uplift on aerial surveys in the days following the earthquake (Clark Citation2017). The damage to D. willana was generally less than that of the two intertidal Durvillaea species, but where the uplift was 2–3 m, then all D. willana, elevated into a new intertidal zone, withered and died. Where the uplift was less, e.g. 0.5-1.0 m, the (previously) deepest attached plants usually survived (Alestra et al. Citation2019). The variable effect of this earthquake on D. willana reinforces the theory that only uniform uplift of an entire rocky shoreline, sufficient to elevate the sublittoral zone of D. willana, would explain the three distributional gaps mentioned above.
Elsewhere, e.g. in Chile and Japan, the instant uplift or subsidence of shorelines after earthquakes, which are often followed by tsunamis, has devastated intertidal and shallow subtidal marine life, changing community composition and patterns of zonation (Alestra et al. Citation2019). For example, in 2010, an earthquake (8.8 Mw) in Chile uplifted the rocky shoreline 0.2–3.1 m across two degrees of latitude. This resulted in mass mortality of large brown seaweeds such as Macrocystis pyrifera (L.) C. Ag. and species of Lessonia and Durvillaea (Castilla et al. Citation2010). Given the frequency of powerful earthquakes in New Zealand, it is not unreasonable, therefore, to presume that at least some discontinuities in marine distributions are the result of uplifts, e.g. in the late Pleistocene and Holocene periods.
Methods
Published distributional records of D. willana are sparse. Some early zonation studies at Taylors Mistake, Banks Peninsula (Knox Citation1953) and Little Papanui, Otago Peninsula (Batham Citation1958) clearly depict D. willana; but there are few such studies within the kelp’s geographic range. Regional algal lists contained a few locations, although most were known before 1977 viz: Rakiura/Stewart Island 4 records; Kaikōura Coast 2; Marlborough 3; Westland 8; Fiordland 3 (Adams et al. Citation1974; South and Adams Citation1976; Nelson et al. Citation1992; Neale and Nelson Citation1998; Nelson et al. Citation2002).
Sightings from the shore were made about an hour either side of low tide (typically spring tides) either by venturing out into the kelp zone, or by identifying the plant with binoculars from vantage points when conditions were hazardous; the key identifier being the presence of stipitate lateral blades on the stipe and, if present, along the lower margins of the primary blade. (Where the primary blade has broken off, the stipe sometimes heals over, reducing the plant to a stump with side branches). The level at which the plant grows on the shore is also an obvious indicator and at low tide it is often briefly sighted between wave surges.
Where there is road access, sea state and tides chiefly determined the timing of field observations. Remote areas such as the southern shore of Fiordland National Park between Preservation Inlet and Te Waewae Bay; most of Stewart Island/Rakiura and the Southwestland shore south of Jacksons Bay have necessitated walking those coasts when tides and sea-sate favoured observations–trips taking a week or longer.
Relatively few sightings were made using scuba; divers tending to avoid the strong wave action and shallow depth of the D. willana zone. As a result, on scuba surveys commonly using tiered sampling methods (e.g. Shears and Babcock Citation2007) down line transects deployed (usually from a small boat) at right angles to the shoreline, D. willana is often ‘under-sampled’ (e.g. Shears Citation2007 working in southern Westland and Kettles et al. Citation2017 working in greater Foveaux Strait), or unrecorded (e.g. Atalah and Sneddon Citation2016 working on Banks Peninsula). Yet often the kelp grows nearby. Whereas Shears (Citation2007) noted that the ‘Durvillaea fringe’ was important habitat, albeit under-sampled (occurring in less than 1% of 517 square metre quadrats), Kettles et al. (Citation2017) erroneously concluded that a similar low frequency meant D. willana was rare or absent throughout the greater Foveaux Strait region suggesting ‘water temperature and hydrodynamics’ as causes. In fact, the kelp occurs commonly throughout the region and is an ecologically dominant species in some places surveyed by Kettles et al. (Citation2017), e.g. Green Islets.
Reliable sightings are still needed for some coastal tracts, especially the outer coast of northern Fiordland between Milford and Doubtful Sounds (c.100 km) along which there is a clearly defined intertidal band of D. antarctica; but scant detail about D. willana. Shorter tracts of shore, for which information is still lacking are: the Westland coast between Bruce Bay and Moeraki River (30 km); Ruapuke and several Muttonbird (Titi) Islands in eastern Foveaux Strait; between Lords and Robertson Rivers on the southeastern shore of Rakiura/Stewart Island (30 km); and the east-facing, plunging, 12 km–long cliff coast of Arapawa Island (south of Cape Koamaru) in Cook Strait.
The 77 numbered sightings of D. willana in (and the appendix) are a compilation of my personal observations since 1972, and those of others who searched for the kelp on my behalf: lighthouse keepers, fishermen, government field officers (notably Sandra King of DOC), colleagues whose research was on the outer rocky coast (notably Kerry-Jayne Wilson studying the southern fur seal) and residents in some remote areas, e.g. Robert Long and family at Gorge River, Southwestland (Long Citation2010). Many sightings are supported by photographs that I hold.
Sea Surface Temperatures (SSTs) are a distillation of satellite-derived temperatures from 1989, available online (Global Sea Temperatures Citationn.d.; Surf-forecast.com Citationn.d.). The surfing website is useful for SSTs in remote areas. SSTs (as °C) are expressed as monthly maxima, minima and mean (in brackets) for the warmest (usually February) and (where applicable) for the coldest (July or August) months.
D. willana: biology and dispersive ability
D. willana, an endemic species, is one of three Durvillaea species growing on mainland New Zealand shores, the other two being D. antarctica, a polyphletic species with a circum-Southern Ocean distribution (Fraser et al. Citation2009), and endemic D. poha (Fraser et al. Citation2012). At all the sites where I have recorded D. willana, it grows in close proximity to either or both of these intertidal species. On some broad, tilted shore platforms, e.g. Tautuku Peninsula, the three species form distinct, contiguous, zones with D. poha, the highest dwelling species, growing at about mid-tide level.
Durvillaea willana is New Zealand’s largest fucalean seaweed, 5–6 m long, and weighing 40 kg +. Unlike the blades of D. antarctica and D. poha, which are internally honeycombed and buoyant, the blades of D. willana are solid, leathery and sink. Its stiff, erect stipe 1–2 m long, bearing side branches (), arises from a solid, woody, domed-to-pyramidal holdfast up to 0.5 m wide (). Holdfasts coalesce to form a composite holdfast supporting several plants. In a sample of 310 D. willana holdfasts from the southern Kaikōura coast, 63.5% of the holdfasts had a single stipe, 29.6% two or three stipes and the remainder (c. 7%) between four and nine stipes (Hay Citation1977).
Figure 2. Stipe and blade (both with secondary branches and blades) of D. willana. Moses Nugget, Ringaringa, Stewart Island/Rakiura 1988.
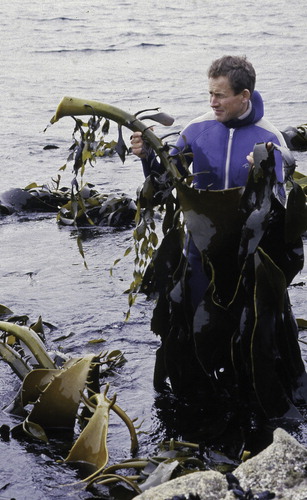
Figure 3. Stipes and holdfasts of D. willana. Moses Nugget, Ringaringa, Stewart Island/Rakiura 1988. Stipes entangled with Macrocystis pyrifera (R).
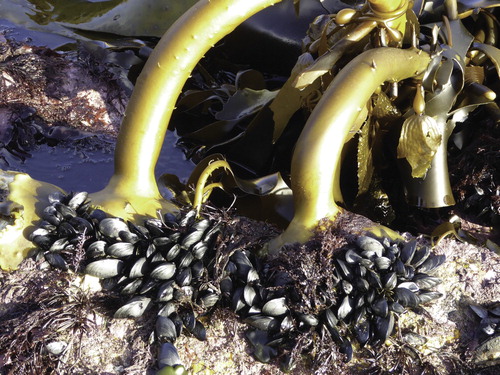
Unlike the extensively burrowed and hollowed-out holdfasts of D. antarctica and D. poha, the unborrowed holdfasts of D. willana adhere much more firmly to the rock. During storms the stipes often snap at the juncture with the holdfast rather than the holdfasts being ripped loose (Hay Citation1977). This is why Garden and Smith (Citation2011) found less than a third of beach-cast D. willana plants had holdfasts, compared with more than three quarters of beach-cast D. antarctica.
Where D. willana forms thick beds, it attains very high biomass values, much higher than for D. antarctica (whether measured in terms of per square metre or length of shore). Areas cleared on the southern Kaikōura coast in January 1973 (at a site now buried by 2016 earthquake debris) and another at Tautuku on the south-east Otago coast in March 1975 yielded values of 23 and 98 wet kg.m² respectively (holdfasts excluded). Comparative values for D. antarctica from six areas distributed between Bethels Beach near Auckland and Ringaringa, Stewart Island /Rakiura ranged from 10–33 kg.m² (holdfasts included) (Hay Citation1977). D. willana is, therefore, an important source of dissolved and particulate organic matter in coastal waters; it provides shelter for a wide range of understorey species and has an important buffering effect on wave action, benefiting more fragile species further inshore and reducing coastal erosion (Hay Citation1977).
Like all Durvillaea species, D. willana is dioecious. Fertilised ova attach rapidly, and in culture studies at least, small diploid plantlets become visible to the naked eye in 7–10 days. Four years monitoring of tagged plants, some of which initially had three or four annual conceptacle layers submerged in their cortical tissue, plus knowing it took at least two years for plants to become fertile, indicated some specimens live for 8–10 years (Hay Citation1977).
When detached, the two buoyant Durvillaea species drift vast distances (e.g. Garden et al. Citation2011), whereas non-buoyant D. willana plants do not move very far. Although wave action and longshore current can ‘bounce’ plants–sometimes with their holdfasts still adhering to large chunks of rock or small boulders–along the shallow seabed of beaches for hundreds of metres, on rocky shores the plants accumulate and decay in subtidal channels, gutters and hollows unless cast ashore (pers. obs.).
D. willana: habitat
Growing predominantly on wave-exposed rocky shores with its upper limit between neap and spring low water levels, D. willana extends down into the sublittoral fringe and shallow sublittoral zone sometimes to c. 4 m below mean low water level (MLW) in clear waters, but more commonly 0.5–1.5 m below MLW where turbid waters prevail. D. willana plants at the top of the kelp zone are often battered and reduced to a stump with side branches. D. willana is stiffer with a more upright habit than D. antarctica. On cliff coasts D. willana favours ledges, small shelves and gutters. Elsewhere, it forms a conspicuous zone on shallow offshore reefs, stable boulder shores and broad wave-cut platforms. Whereas ocean swells elevate the band of D. antarctica holdfasts on cliff faces many metres above the nominal highwater mark, the band of D. willana tends to remain semi-submerged in the surge of the sublittoral fringe and shallow sub-littoral. It tolerates some abrasion by sand and gravel, often growing right at the interface between emergent bed rock and sandy seabed, e.g. along the Akatore shoreline south of Dunedin. Dense beds of D. willana near the mouths of major rivers suggests tolerance of low salinities.
Distribution of D. willana
Confined to shores of Stewart Island/Rakiura and South Island and a small North Island population near Castle Point on the northern Wairarapa coast, D. willana spans a latitudinal range of 6°47´–much narrower than that of D. antarctica which, in the greater New Zealand region, straddles about 23° of latitude between subtropical Norfolk and (probably) Kermadec Islands (Nelson and Adams Citation1984; Millar Citation1999; pers. comm. 2003) and subantarctic Macquarie Island. The northern limit of D. willana, like the upper temperature limits of many kelps (Setchell Citation1893), is presumably determined by temperature. Its present northern limit on west and east shores correlates with a maximum mean monthly sea surface temperature (SST) isotherm of 19–20°C. Temperatures above 20°C for several consecutive days in the warmest month are likely to be detrimental to the plant. In this regard, D. willana’s upper temperature limit (and indeed its northern distribution on the east coast) is the same as that of Macrocystis pyrifera in New Zealand (Hay Citation1990).
In the west, near its northern limit, at Westport and Cape Farewell, where there is local upwelling (Shirtcliffe et al. Citation1990), summer SSTs are 17.5–20.3(18.9)°C and 17.0–20.2(18.0)°C. In the east, at its northern limit near Castle Point, highest temperatures are 17–20(19)°C. This is why D. willana does not grow in the greater western Cook Strait region off the Marlborough Sounds, Nelson and along Wellington’s western shore. And it is why it extends no further north than the Castle Point area on the northern Wairarapa coast–an area coincident with the northern extent of the Southland Current where there are steep temperature gradients. Near Cape Turnagain, north of Castle Point, autumnal SSTs have been recorded as increasing from 16 to 22°C in just 10 nautical miles (Ridgway Citation1970).
The lowest temperature limit for D. willana is not known. It may be similar to laminarian kelps. On Sabre Reef off Robertson River, near the southern limit of D. willana, lowest winter SSTs (August) range from 7–11(10)°C. Moreover, D. willana grows in some winter-shaded localities on the southern Kaikōura coast where ice occasionally forms at the sea’s edge (pers. obs.). Although absent from the southern shelf islands of The Snares Islands/Tini Heke, and Auckland and Campbell Islands, this does not mean that it would not grow there if it could spread that far.
South Island and Stewart Island/Rakiura
Excluding the 100 km + distributional gap south of Open Bay Islands in Southwestland (discussed below) and the outer rocky coast of the northern fiords, for which information is lacking, the large gaps between the sightings of D. willana around the shores of South Island and Stewart Island/Rakiura () are either long sandy beaches (e.g. Te Waewae Bay and Pegasus Bay) or lengthy tracts of shingle shore (e.g. Westland south of Greymouth, the Canterbury Bight and bays north and south of Kaikōura Peninsula).
South from near Cape Farewell (sites 1 and 2) D. willana grows on most wave-exposed headlands and reefs of northern Westland, abundantly so at several locations south of Westport (sites 5–8). Further south between Greymouth and Bruce Bay (site 12) the kelp is more sparsely distributed partly because frequent changes in beach levels and profiles in this region temporarily buries low intertidal and shallow subtidal rocky substrata. Common again in southern Fiordland, south of Dusky Sound, D. willana extends east of Puysegur Point (site 20) along Southland’s southern coast (and the northern shore of Foveaux Strait) () to Waipapa Point (site 39).
Around Stewart Island/Rakiura, in the path of the cool Southland Current, the kelp occurs on most exposed rocky shores, forming dense beds in places such as Ringaringa (site 37). North of the eastern approaches of Foveaux Strait, along South Island’s southeastern coast to Otago Peninsula, D. willana is exceedingly abundant, extending to depths of 3–4 m below mlw where clear water prevails (pers. obs.). North of Otago Peninsula it grows on all headlands and offshore reefs until Timaru (site 58) where rocky shore ends for 150 km. D. willana grows commonly around volcanic Banks Peninsula (a rocky ‘island’ flanked by long beaches) reappearing again on most exposed rocky shoreline beginning north of Pegasus Bay. Still in the path of the Southland Current, the kelp is particularly abundant along the southern Kaikoura Coast. It grows (or grew) on Kaikōura Peninsula (another rocky ‘island’) and then sporadically north of Hapuku River until Cape Campbell (site 70). North of Cape Campbell, in western Cook Strait, the kelp extends northwards to The Brothers (site 74).
North Island
Apart from a localised population near Castle Point on the northern Wairarapa Coast (site 75) there are no records of D. willana growing on North Island shores. At Castle Point, D. willana grows along the exposed and near-perpendicular seaward edge of the rocky promontory on which the lighthouse stands–an erosion-resistant outcrop of shelly limestone and calcareous sandstone of Late Pliocene age in near horizontal stratified layers. The plants are sporadic, often battered, and do not form a conspicuous zone (pers. obs. March 1993). Northeast of Castle Point, for about 14 km, there are low lying rocky reefs to about the mouth of the Mataikona River. Adams (Citation1972) recorded the kelp from Whakataki and Mataikona (at the southern and northern ends of this tract of shore) and noted that the kelp is abundant in the drift.
The 2016 Kaikōura Earthquake: effects on D. willana
Uplift along different coastal sectors
This earthquake straddled two distinct seismotectonic domains, involved at least 13 separate faults, breaking many, and was one of the most complex earthquakes ever recorded. The earthquake impacted about 105 km of coast (measured in a straight line) from near Oaro River on the southern Kaikōura coast to Cape Campbell on Marlborough’s north-eastern shore. About a quarter of this coast is rocky and three-quarters is beaches varying in length from pocket-size to tracts tens of kilometres long. Most of the shoreline was uplifted in the 2016 Earthquake, although the extent of uplift (−0.2 to just over 6 m) was highly variable, even at a small spatial scale. Uplift was generally greatest north of Kaikōura Peninsula, particularly just south of the Clarence River mouth (e.g. Waipapa Bay) (Clark et al. Citation2017; Hamling et al. Citation2017; Kaiser et al. Citation2017).
Proceeding northwards from Haumuri Bluffs (site 65) there are four rocky coastal tracts, flanked by beaches, each with D. willana, and each variably uplifted. First, most, but not all of a 15 km section of mainly rocky shore between Haumuri Bluffs and the Kahutara River, where D. willana is (or was) very abundant–much more so here than north of Kaikōura Peninsula (Hay Citation1977). The uplifted length of this sector lies between a point just north of Oaro River (where the Hundalee Fault crosses the coastline to emerge at the head of the Kaikōura Submarine Canyon) and the Kahutara River. Uplift near Omahi was 1.5–2.5 m and at Goose Bay (slightly further north) 1.6 m, while other parts were variably uplifted 0.5–1.0 m (Clark Citation2017; Alestra et al. Citation2019). There was, however, negligible uplift along about 6 km rocky shore between a point just south of Haumuri Bluffs and limestone platform reefs immediately south of Oaro River.
Second, around Kaikōura Peninsula, where the uplift was 1.01 m (Stephenson et al. Citation2017), there are wide wave-cut mudstone and Amuri limestone shore platforms that support three species of Durvillaea. D. willana is the least abundant, being confined to the outermost points of the various promontories.
Third, from just north of Hapuku River at Blue Duck Stream to Waipapa Bay (c. 12 km), there is rocky shore (mainly greywacke) where small promontories and outcrops (e.g. Ohau Point, uplifted 2–3 m) are interspersed with small pocket beaches. This area experienced the greatest uplift: 4–6 m at Waipapa Bay where dying D. antarctica at a level normally occupied by coastal shrubs and small trees was extensively photographed and reported in the media.
Fourth, north of the Flaxbourne River as far as Cape Campbell (47 km) there is intermittent sedimentary rocky shore with small offshore reefs, and (approaching the Cape) wide intertidal platforms separated by beaches 0.5–3 km long. This sector is flanked by beaches to the south and west (Clifford Bay). Some of this shore was uplifted 1.5–2.5 m although the uplift diminished to about 1 m towards at Cape Campbell and Marfells Beach, just west of the Cape inside Clifford Bay. Nevertheless, huge areas of new intertidal reef emerged near the Cape Campbell lighthouse.
Predicted long-term effect on D. willana distribution
The maximum tidal range at Kaikōura Peninsula (site of the tide gauge) is 2.18 m, with Spring tidal ranges commonly between 1.8 and 2.0 m (Tide Predictions Citationn.d.). In many areas (especially north of the Peninsula), the uplift therefore equalled or exceeded the average Spring tidal range–near Waipapa Bay up to three times the tidal range. No D. willana survived the uplift. Even where the uplift was less (1.5–2.0 m), intertidal life was elevated above supralittoral zone, while species like D. willana growing just below the sublittoral fringe were suddenly elevated to mid-tide level or higher, where there was little chance of them surviving ().
Figure 4. D. willana on southern coast of Fiordland National Park near Green Islets, 2 km southwest of Andrew Burn (, site 22). Photo taken between surges at low tide, 6 February 2012, Plants in the lower right corner are D. antarctica.
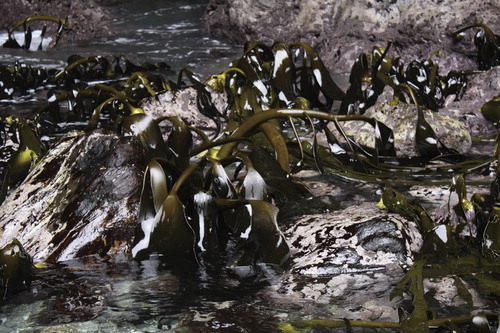
South of Kaikōura Peninsula, in areas where the uplift was a metre or less, any D. willana previously growing near the bottom of the kelp’s normal vertical range, in the shallow subtidal zone, were likely to have survived (Alestra et al. Citation2019). My pre-earthquake observations of D. willana along this shore, particularly south of Kaikōura Peninsula, indicated that the usual lowest level of the kelp in the shallow sublittoral was about 1.5–2.0 m below MLW. The earthquake would, therefore, have raised the lowest-attached plants into what suddenly became the sublittoral fringe, where they would still be semi-submerged and wetted by waves at low tide. At that level, however, the impact force of waves is much stronger, so that the elevated plants became battered, commonly losing their primary blade and sometimes their secondaries (Alestra et al. Citation2019; Figure 10) (). Also, the abrasive effect of shattered rock and mobilised gravels in the sublittoral fringe after the earthquake damaged and buried holdfasts. Therefore, south of Kaikōura Peninsula, although patches of D. willana were eliminated, the species survived (albeit battered) where the uplift was less than the vertical range of the plant, while a sizable ‘reservoir’ population nearby (south from Oaro River) was little affected.
Figure 5. D. willana, battered but still surviving after the 14 November 2016 Kaikōura Earthquake. Photo taken (February 2017) at low tide about 12 weeks after the earthquake. Prior to the earthquake the plants in the foreground were totally submerged at low tide; those in the background would have been partly emergent. Higher zoned plants originally growing in the sublittoral fringe (near the mean low water Spring tide level) have died, although a few holdfasts remain. The areas covered with sea lettuce (Ulva sp.) were all uplifted. Other uplifted brown seaweeds are Marginariella boryana (A. Rich.) Tandy and Lessonia variegata J. Agardh. Location: near Flaxbourne River mouth, northern Kaikōura coast. Photo: Elizabeth Lochhead.
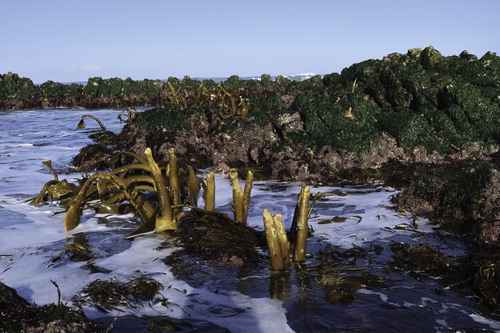
Time will tell if uplift eliminated D. willana from the Kaikōura Peninsula. The uplift of 1.01 m, while obliterating all intertidal seaweeds (e.g. Alestra et al. Citation2019; Figure 21), may have been small enough for the deeper-dwelling D. willana plants to survive. If none survived, then the long flanking beaches will likely impede recolonisation.
North of the Kaikōura Peninsula, in the Blue Duck Stream to Waipapa Bay sector, where in some areas the uplift exceeded 4 m, it is possible that entire populations on some offshore reefs, and along sectors of shore bounded by beaches, were wiped out. If so, re-colonisation may be very slow. Because the uplift was so variable, however, some of the more deeply attached plants may have survived especially if elevated to places where their fronds were wetted in surge channels at low tide.
Because the Kaikōura earthquake was in early summer after the period of gamete release, there were no gametes available to recolonise the newly uplifted rock for about six months. As clearing experiments of D. antarctica and D. poha at Tautuku, Southeast Otago showed, areas cleared in spring and summer were quickly covered by turf-forming seaweeds; especially articulated corallines. Forming a dense, carpet-like sward, these prevented any recolonisation by Durvillaea the following winter and the sites remained kelp-free for at least four years. In complete contrast, winter-cleared areas were promptly covered by a lawn-like growth of small Durvillaea sporelings which grew rapidly, some becoming fertile in 2 years (Hay Citation1977; Hay and South Citation1979). At Kaikōura, therefore, recolonisation may be impeded to some extent by fast-growing opportunistic species covering the rock in the months before D. willana released gametes in the winter of 2017.
There are likely to be similarities between regrowth of D. willana on the southern Kaikōura coast and that of the laminarian kelp Lessonia nigrescens Bory de Saint-Vincent in central Chile. Like D. willana, its upper limit is also the intertidal-subtidal interface and its lower limit c. 1.5 m below low tide. On 3 March 1982, an earthquake (7.8 Mw) uplifted shoreline 44–59 cm on which the coastal marine station Estación Costera de Investigaciones Marinas, La Cruces, is located. A before-and-after earthquake comparison of belt transects (divided into upper, mid and low plots) made through the 1.55 m wide L. nigrescens zone revealed that all the plants in the upper and middle plots died (although those in the middle plot took over a year to succumb) and that there was no recolonisation in those plots. The plants in the lower plots survived, however, their biomass more than doubled and there was significant recolonisation even in the low plots where previously there had been no Lessonia (Castilla Citation1988).
Where uplift on the southern Kaikōura coast was about 0.5 m, similar recovery for D willana is likely. If, however, the uplift had been uniformly 2–3 m (or greater) at Kaikōura Peninsula or along the southern Kaikōura coast, and if it had also extended southwards beyond Oaro River, then, because of long flanking beaches, recolonisation of both areas would be very slow, perhaps taking hundreds of years. This is probably what happened on Wellington’s south coast after the 1855 earthquake described in the next section.
Gaps in the distribution of D. willana
Absence from the Wellington south coast west of Palliser Bay
Given the narrowness of Cook Strait, and the proximity of D. willana on Marlborough’s northeastern shore, the absence of D. willana on this southern-most North Island shoreline is unusual, particularly so when D. antarctica and many other large brown algae typically associated with D. willana grow there (pers. obs.). The absence of D. willana is unlikely to be due to sea surface temperatures. Highest summer SSTs at Lyall Bay, Breaker Bay and Island Bay, on Wellington’s south coast are all 16–19(17)°C–little different from highest summer temperatures on the southern side of the Strait near Cape Campbell, viz: 16–19(17)°C at Whites Bay; 15–18(17)°C at Kekerengu; 17–20(19)°C at Castle Point–and slightly cooler than the SSTs off northern Westland and northwest Nelson. The kelp could, however, have been eliminated by earthquake uplift, with Cook Strait and long beaches preventing recolonisation from the nearest unaffected populations on South Island and in the northern Wairarapa.
This tract of shore, west of Palliser Bay (spring tidal range 1.36 m) has been uplifted by several major earthquakes as evidenced by the well-known sequence of five, of which three are prominent, raised shorelines above Turakirae Head. These dates back about 7000 years, viz: 110–430 BC (uplift 9.1 m); 2164–3468 BC (6.8 m); 4660–4970 BC (7.3 m); and an earlier uplift of unknown magnitude at c. 7000 BC (McSaveney et al. Citation2006).
The last major uplift, the M8+ Wairarapa Earthquake on 23 January 1855, caused an uplift that decreased westward from 6.4 m at Barney’s Whare (c. 5 km northeast of Turakirae Head) to: 2.7 m at Turakirae Head, 1.5 m at Wellington Harbour entrance, 1.2 m at Island Bay and 0.5 m on Wellington’s west coast (Darby and Beanland Citation1992; Grapes Citation2000; Begg and McSaveney Citation2005; McSaveney et al. Citation2006). This uplift would have certainly obliterated the intertidal band of D. antarctica which, with its buoyant, honeycombed blades and propensity to drift, would have recolonised the shore. In contrast, recolonisation would not have been possible for non-buoyant D. willana (assuming it was present at the time of the uplift, which is unknown).
If eliminated from the Wellington south coast by uplift, then potentially there are three ways D. willana could recolonise the shore: (1) by gametes or fertilised eggs drifting from South Island; (2) by freshly detached plants releasing their gametes which might quickly colonise the newly uplifted shore; or (3) by rafting to Wellington, entangled with buoyant kelps such as Macrocystis pyrifera and Durvillaea antarctica.
Alternative (1) is highly improbable. Gametes or fertilised Durvillaea ova are unlikely to drift very far, certainly not across the eastern approaches of Cook Strait. Culturing Durvillaea sporelings from gametes (e.g. Naylor Citation1953; Delépine Citation1964; Hay Citation1977, Citation1994), and experiments to determine how firmly zygotes adhere to the substrate after settlement (Taylor and Schiel Citation2003), suggest Durvillaea ova are unlikely to become even a short-term component of nearshore plankton. Once fertilised, the zygote sinks quite rapidly, becomes rounded and quickly adheres to the substrate, commonly within 1–2 h. A root-like, primary rhizoid, precursor to a holdfast, develops in 10–12 h. Taylor and Schiel (Citation2003), who subjecting settled zygotes to waves in a wave tank, found that after as little as an hour, 75% of zygotes were firmly glued; after 6 and 12 h, 90 and 100%, respectively, had adhered strongly.
Alternative (2) would have been impossible, at least as for the 1855 earthquake, because the uplift was in mid-summer when D. willana is not reproductive. If D. willana had been present, its gamete release period would have been between July and September–months before the earthquake (Hay and South Citation1979).
Alternative (3) is theoretically possible because, by growing close to raft-forming D. antarctica, D. poha and Macrocystis, D. willana could potentially be entangled in rafts of those species despite its propensity to sink (often with rock attached). In reality, I am unaware of any drift records for D. willana on Wellington’s southern coast where I periodically searched the seaweed drift over 15 years including a vast quantity after an unusually powerful southerly storm between 20–22 May 1983; then the lengthiest since 1960 (New Zealand Weather Summary Citation1983). I found no D. willana plants.
I concluded, therefore, that D. willana is a poor rafting candidate. Were that not so, and rafting was frequent, then, like D. antarctica, the species would be much more widely distributed; it would have reached islands like Snares Islands/Tini Heke and the Chatham Islands (the Southland Current eddies and curves eastwards to the Chatham Islands east of Cook Strait); and the large distributional gaps, discussed here, would not occur.
Distribution gap on the southern and central Wairarapa coast
Although much of the shoreline along the southern and central Wairarapa coast between Cape Palliser/Matakitakiakupe and Castle Point (c. 120 km) is surf beach, there are numerous rocky promontories, tracts of rocky shore (1–3 km long) and some offshore reefs, all apparently suitable habitat for D. willana. It has, however, never been recorded from this coast.
Tectonically a very active region, most of the southern part of North Island is actively uplifting several millimetres per year. Seawards of the Wairarapa coast lies the Hikurangi Trough, part of the boundary between the oceanic crust of the westward-dipping Pacific Plate, which is subducting beneath the continental crust of North Island that forms part of the overriding Australian Plate (Berryman et al. Citation1989; Berryman et al. Citation2015). Between the Trough and the shore, running roughly parallel with the coast, are several active faults (Berryman et al. Citation2011; Figure 1). Berryman et al. (Citation1989; Figure 4) mapped 14 tectonic subregions along the east coast where there have been episodes of coastal uplift as indicated by a series (up to seven) of well-preserved coastal marine terraces formed in the last 6700 years. On the southern Wairarapa Coast, these are particularly well preserved near Pukemuri Stream, 25 km northeast of Cape Palliser/Matakitakiakupe (Berryman et al. Citation2011; Figure 2c; Lichfield and Clark Citation2015; Figure 2a). The intervals between uplifts varied between 400 and 2000 years with the height of the uplifts ranging from 1 to 4 m. A uniform uplift of 3–4 m would have obliterated entirely the population of D. willana along the Wairarapa coast if it were present.
Distribution gap in South Westland
Southwest from Bruce Bay (site 12), past Jackson Head and Open Bay Island, to the entrance of Milford Sound (a straight-line distance of about 130 km), the nature of the shore changes abruptly from predominantly unstable beach to lengthy tracts of much-faulted rocky shore, extending well into the subtidal. A generalised map, together with diagrams of stratified columns of tertiary rocks by Young (Citation1978) depicts areas of rocky shore comprising conglomerate or breccia, siltstone or mudstone and sometimes limestone interspersed with consolidated glacial deposits with sizable boulders. On such a shoreline elsewhere in southern New Zealand, a near-continuous band of D. antarctica and D. willana, would occur. But shore searches by Wilson in August 1972, Hay in March 1975, and Hay and Turnbull in 2009 and 2011 and observations by Richard Long (over 30 years) found no D. willana and very little D. antarctica. Going south from Bruce Bay, D. antarctica first appears (as a bed) at Cascade Point (Wilson pers. comm. August 1972, Long pers. comm. December 2011).
Neale (Citation1992) suggested local oceanography might be explain this gap because this is the region where oligotrophic subtropical water from Australia (the East Australia Current), when meeting the obstacle of South Island, surfaces and, mixing with coastal water, diverges to flow northeastwards (as the intermittent Westland Current) and southwestwards (as part-origin of the Southland Current) (Menge et al. Citation2003). This is, however, an improbable explanation for the kelp’s absence, because in southeastern Australia, the same oligotrophic East Australia Current water supports abundant populations of two Durvillaea species (Weber et al. Citation2017) similar to D. willana morphologically and in terms of its ecological niche (pers. obs.).
In terms of temperature and nutrients, oceanography at Jackson Head would appear to favour the growth of D. willana. There is nothing to indicate that SSTs near Jackson Head are (or have been) significantly warmer than areas further north and south where D. willana grows abundantly. In fact, SSTs in South Westland are significantly cooler than off Northwest Nelson and much cooler than at Castle Point–both localities where D. willana occurs.
While the East Australian Current water is described as oligotrophic, there is no evidence to suggest that the absence of D. willana in this region is caused by localised low concentrations of nutrients, e.g. less than 1.0 µM L−1 for nitrate, a suggested lower threshold for large kelps (Gerard Citation1982). Water upwelling from 75 m depth near Jackson Head had a nitrate concentration of about 5 µM L−1. Mapped sea surface nitrate concentrations in June 1979 showed that levels were higher (1.5–2.0 µM L−1) in South Westland, where Durvillaea spp. does not grow, than further north between Greymouth and Cape Foulwind where the kelp is common (0.5 µM L−1) (Bradford Citation1979; Figures 4 and 11). Upwelling is also significantly more frequent at Jackson Head than at points further northeast (Menge et al. Citation2003). Also, with nitrate concentrations in South Westland rivers ranging from 3.5–14.3 µM L−1 (Bradford Citation1979), nutrient levels in the near-shore waters of Westland are also periodically elevated (as well as being cooled) by river outflows (Moore and Murdoch Citation1993). This is a coast where upwelling is common (Stanton Citation1976). Monthly mean upwelling indices, as measured by Bakum upwelling indices (m³.sec.100 m of coast) have shown that the west coast is upwelling-dominated (twice the downwelling frequency and about every five days), whereas the downwelling-dominated east coast of South Island is relatively nutrient poor (Menge et al. Citation2003). Yet D. willana is much more abundant on South Island’s east coast.
Tectonic activity may provide the answer. Although relative to the Wairarapa coast, there is scant evidence here, and even less evidence further south in northern Fiordland, of raised shorelines, this does not mean that uplifts have not occurred. This coastal region, so close to the Alpine Fault, is highly earthquake-prone. From where it emerges from the sea near Milford Sound, the Alpine Fault runs north-eastwards obliquely across South Island until the Nelson Lakes area, a straight-line distance of about 460 km. Southwest of Milford Sound, the fault is submarine and runs roughly parallel to the Fiordland coast to join, in the southwest, with the Puysegur Subduction Zone.
On land, geologists have divided the fault into three sections: Milford Sound to Jacksons Bay, then to Hokitika, and from Hokitika to Lake Rotoiti in Nelson Lakes. The Milford to Jacksons Bay section correlates with the gap in Durvillaea distribution. Here, where the subducting Australian Plate converges with the eastward-dipping and overlying Pacific Plate, the continental crustal rock on both sides of the plate boundary collides causing considerable horizontal slipping of 29 mm per year (north-eastwards), modest uplift, and many powerful, shallow earthquakes (e.g. 6.7 Mw on 10 August 1993 and 7.2 Mw on 22 August 2003). The 2003 earthquake, 12 km deep and with 6365 aftershocks, caused huge landslides on Secretary Island and a very much wider area. The predicted uplift immediately offshore was 45 cm, while the actual uplift inside Thompson Sound was 20 cm (Downes et al. Citation2005). This southern section ruptures every 291 ± 23 years and there have been 27 ruptures of magnitude 7.8–8.0 Mw, the last powerful one in about 1771 (Downes et al. Citation2005). Further north along the Fault there was a major quake between 1740 and 1840 (Cooper and Norris Citation2015; Gorman Citation2019).
Maōri tradition records powerful earthquakes in this region before European colonisation and during the European sealing era, which began about 1792. There are reports of huge tidal waves (tsunami) sweeping along Southland’s Foveaux Strait in c.1820 drowning many Maōri. The Maōri stronghold of Ruapuke seems to have fared particularly badly (Downes et al. Citation2005). Such waves can only have been caused by massive earthquakes either along the Alpine Fault or further south in the Puysegur Subduction Zone.
Whether these earthquakes created uplifted terraces is not clear. The steepness, coastal erosion, high rainfall (and perhaps glaciation) likely obliterated such signatures. There is, however, strong anecdotal evidence suggesting that a powerful Fiordland earthquake (and many aftershocks) in 1826 caused tsunamis and huge landslips over a wide area, e.g. for about 180 km south of Cascade Point and substantive uplift (Taylor Citation1855). Modelling such a rupture in the Fiordland Subduction Zone (estimated as 7.7 Mw)–assuming rupturing of a large part of the zone but without breaking the surface–has indicated a seafloor uplift of 1.4 m near the epicentre and 0.8–1.0 m along the immediate shoreline (Downes et al. Citation2005).
This is supported by sealer, Edward Meurant, in his account of a convenient little anchorage just inside the entrance of Doubtful Sound, on the southern shore, that he called ‘The Gaol’. He described it as being semi-enclosed by steep rock walls and having sufficiently deep water to tie boats up right alongside and so step directly ashore. As a result of an earthquake in 1826, the entire floor of this little inlet was elevated so high that it could no longer be used as an anchorage (Meurant cited in Taylor Citation1855, p. 235; cited in Downes et al. Citation2005). This has been identified almost certainly as Cascade Cove on the mainland shore just off the seaward end of Bauza Island in Doubtful Sound. When geologists examined the terrain immediately inland, they found a series of five raised platforms, the most extensive of which resembles an elevated wave-cut platform 2–3.2 m above current sea level (Downes et al. Citation2005).
It is plausible, therefore, that this South Westland gap in the distribution of D. willana may have been caused by such instant coastal uplift that left the entire zone of the kelp high and dry and obliterating the species along tens of kilometres of shore. On the outer cliffs, D. antarctica would have persisted given its abilities to grow many metres above the nominal mean high water mark on a cliff shore exposed to huge waves and to recolonise the shore by drifting.
Tectonically this shore (and that of the entire Fiordland further south) is mapped as uplifting (0–1 mm per year). Interestingly, the sector of coast inhabited by D. willana immediately north-east, is relatively stable (Berryman et al. Citation2015, p. 144), adding further support to this hypothesis.
Discussion
The 2016 Kaikōura Earthquake showed that while such uplift obliterated intertidal species of Durvillaea, and other large brown seaweeds, the effect on D. willana growing in the sublittoral fringe and shallow subtidal zone was less dire. Where the height of the uplift was less than the vertical range of this species, where it was spatially variable, and where some areas of contiguous rocky shore were not uplifted, then D. willana survived, despite massive mortality.
Another coastal location uplifted in recent geological time, where D. willana has persisted, lies south of Otago Peninsula along the Akatore Fault (Harrington Citation1958). The 23 km stretch of shore off the active Akatore Fault has a series of four coastal terraces, the lower two separated by a scarp near sea level. These are Holocene coastal platforms 3 and 6 m above the current high water mark. The lowest platform was dated from uplifted mollusc shells as c.1400 years BP (Litchfield and Norris Citation2000). This lowest uplift, as well as those higher, were considered indicative of three Late Holocene earthquakes. But, as often is the case, there is the problem of determining if the uplifts were instant and massive or if they were incrementally caused by a swarm of smaller quakes perhaps decades apart. A recent marine botanical study (Parvici et al. Citation2019) indicates that they were instant. If the shore rose 1.5–2 m, as the scarp at Quoin Point suggests (pers. obs. July 2019). then clearly all intertidal Durvillaea would be obliterated. But buoyant, recolonising intertidal plants from beyond the uplifted zone would eventually drift in and ‘reseed’ the shore.
By comparing the DNA of intertidal Durvillaea currently growing on this presumed uplifted shore with the DNA of (control) populations growing on stable shore nearby, these authors found significant differences for both D. antarctica and D. poha, indicating that uplift rendered the original populations of these species locally extinct, to be replaced by plants with genetically different DNA that rafted in, probably from the northwest (Parvici et al. Citation2019). There is, however, no gap in the distribution of D. willana consistent with this uplifted Akatore shoreline. It grows abundantly on all wave-exposed rocky areas along the Akatore shore, forming a clearly defined kelp zone several metres wide–continuously so for hundreds of metres as at Quoin Point–and contiguous with D. antarctica. This suggests three possible explanations for the lack of any distribution gap: (1) there was no instant uplift (unlikely given the kelp DNA evidence of Parvici et al. Citation2019); (2) the uplift did eliminate D. willana which over several hundred years has returned by being recolonised from the adjacent, stable shores–especially to the north (future genetic studies, this time on D. willana, may determine if this was a likely event); or (3) uplift, while devastating much of the D. willana population, was not big enough to eliminate the deepest attached plants, the same outcome as seen on the southern Kaikōura coast.
The Akatore event does not, therefore, disprove the theory that uplift would cause a gap in the distribution of D. willana. Rather it is partly supportive. Based on the genetic evidence, the Akatore uplift caused the demise of intertidal Durvillaea. Had the uplift been greater and had this tract of shore was not been flanked by un-uplifted rocky shore currently as close as 1–2 km, then D. willana would have been eliminated and slow to recolonise.
On the northern Wairarapa coast, D. willana grows between Castle Point and Mataikona (Figure 1, Inset a) along a shore where four adjacent raised terraces indicate several uplifts. The presence of the kelp here appears contradictory. What, therefore, are its likely origins? It is unlikely to be a northern outlier of South Island populations, that are 200 km distant, especially when there are no intervening populations. A more likely explanation, therefore, is that this population is a northern relic, from before 18,000 y BP, when sea levels were 135 m lower.
At that time the Subtropical Convergence and Subantarctic Front (and the Southland Current) pushed much further north and sea surface temperatures were 5–6(10) °C cooler (McGlone Citation2002). The three main islands were then joined, and the old eastern shore, lying roughly along the edge of the continental shelf, was relatively un-indented except for a strongly tidal, 230 km long, narrow inlet orientated SE to NW approximately in the position of the current Cook Strait Canyon (Proctor and Carter Citation1989). Under a cooler climate, a species like D. willana would have extended further northwards along the eastern shore–later moving inshore as sea level approached present day levels–as did many cold water mollusca for which we have a fossil record (Hornibrook Citation1978). The limestone and shell-sandstone of Castle Point contains over 70 warm and cool-water fossilised species, some still extant, including the deep, cold water southern scallop Zygoclamys deliculata (Hutton). These are arranged in layers as a result of species advancing and retreating in response to paleoclimatic fluctuations since about 2 million y BP (Homer and Moore Citation1989). In keeping with mollusca, the northern limit of D. willana will have advanced and retreated during the numerous (20+) glacial advances and relatively brief warm interstadials of the Pleistocene, and more recently since the coldest period of New Zealand’s Last Glacial Maximum 67–62,000 y BP (Williams et al. Citation2015). At times when the cool Southland Current pushed further north, then so too would D. willana, while in warmer times, as the warm East Cape Current curved around East Cape to flow southwards, the kelp would retreat accordingly.
If, historically, D. willana grew a little further north of its current northern limit near Mataikona, then, significantly, it would have been growing along an 85 km tract of shore that, unlike the rest of the Wairarapa coast was not uplifted (Berryman et al. Citation1989, p. 188, Figure 4). Thus, it could have survived, relatively undisturbed by earthquake uplift further south. That today there are no records of D. willana growing north of Mataikona may be the result of warming SSTs since the Last Glacial Maximum which meant D. willana, along with many cold water mollusca, retreated southwards to colonise the adjacent Castle Point shoreline. In time, and driven by northeast winds, the Castle Point population of D. willana may slowly spread south.
The presence of D. willana on Solander Island (site 21), western Foveaux Strait, situated 45 km from shore, might appear to contradict the presumed poor dispersal of this non-buoyant plant. But when sea levels were lower, Solander Island was much closer to the Holocene shore. In Foveaux Strait, carbon dating of submarine peat (indicative of lower shorelines) has indicated that sea levels started to rise quite rapidly 11–9,000 years BP (Cullen Citation1966). On the Snares Islands/Tini Heke, south of Stewart Island/Rakiura, however, where there is no D. willana, a strait separated the islands from mainland New Zealand, even when sea levels were at their lowest (ca 135 m below present day level). This strait would also explain the small endemic element in the Snares Islands/Tini Heke seaweed flora (Hay Citation1987).
When relating coastal uplift to the kelp’s absence, the largest area likely to provide further examples is the outer coast of northern Fiordland–north of Breaksea Sound. Here eleven fiord entrances, 1–3.5 km wide, separate ten sectors of outer rocky shore with an average length of 12 km (range 4–23 km). Prone to uplift, as the outer shore of Secretary Island (between Thompson and Doubtful Sounds) has shown, it is possible that some of these sectors have been uplifted en masse by powerful earthquakes along the Alpine Fault after the ice sheet melted finally from the Fiordland valleys about 10,000 years ago (McKellar and Soons Citation1991). This may have caused gaps in the distribution of D. willana particularly when the deep Fiord entrances are major barriers to any longshore movement of detached plants. Such gaps are, however, conjecture until the detailed distribution of D. willana along this outer shore is known. Because of the frequently huge seas pounding this shore, this local distribution might best be mapped by operating drones from an offshore vessel.
Conclusion
The three major gaps in D. willana distribution that cannot be attributed to the explanatory features identified by Laing (Citation1894), or to oceanographic or climatic factors, are all on parts of the coast subject to instant, earthquake-driven, coastal uplifts and are likely to be the result of such uplift. The 2016 Kaikōura earthquake demonstrated the devastating effect of uplift on shallow sublittoral species such as D. willana. It also showed that for a gap to form, any such uplift must be consistent along an entire tract of rocky shore (flanked by dispersal barriers) and that it must be of adequate magnitude to elevate the entire kelp zone high enough to eliminate all plants.
A study similar to recent genetical studies of other Durvillaea species in New Zealand and Australia (e.g. Fraser et al. Citation2009, Citation2010, Citation2012, Citation2016; Weber et al. Citation2017; Parvici et al. Citation2019) of some of the geographically isolated D. willana populations (e.g. Banks Peninsula, Solander Island, Castle Point) may provide some clues as to the length of time they have been reproductively isolated. Although Fraser et al. (Citation2010) concluded there was little genetic variation of D. willana, they did detect northern and southern haplotypes by comparing samples from just three localities north and two localities south of the Canterbury Bight. The geographical range of D. willana is, however, very much wider than Fraser et al. (Citation2010) depicted and it would be interesting, therefore, to find out if there is significant genetic variation among populations along South Island’s western, Tasman Sea coast, and if they differ from those along New Zealand’s Pacific seaboard.
Acknowledgements
To the late Dr Lucy B. Moore who sparked my interest in seaweeds on a memorable field trip to Oaro in 1963. To the late Nancy M. Adams, whose infectious enthusiasm for the subject helped maintain my interest and with whom I periodically discussed the possibility that earthquake uplift eliminated D. willana from on Wellington’s coast. To my wife, Jane Turnbull, who organises our coastal ‘expeditions’. To my son, Erik, who drew the map. Finally, to various colleagues and friends who periodically over decades, have searched for D. willana on my behalf.
Disclosure statement
No potential conflict of interest was reported by the author.
References
- Adams NM. 1972. The marine algae of the Wellington area. Records of the Dominion Museum. 8(4):43–98.
- Adams NM, Conway E, Norris RE. 1974. The marine algae of Stewart Island: a list of species. Records of the Dominion Museum. 8(14):185–245.
- Alestra T, Gerrity S, Dunmore R, Marsden I, Pirker J, Schiel D. 2019. Rocky reef impacts of the Kaikōura earthquake: quantification and monitoring of nearshore habitats and communities. Wellington: Ministry of Primary Industries. 120 p. New Zealand Aquatic Environment and Biodiversity Report 212.
- Atalah J, Sneddon R. 2016. Lyttelton Harbour and Banks Peninsula shoreline reef ecology: Field survey data report (February 2016). Prepared for the Lyttelton Port Co. Ltd. Cawthron Institute Report 2854a; 30 p. + appendices.
- Batham EJ. 1958. Ecology of a southern New Zealand rocky shore at Little Papanui, Otago Peninsula. Transactions of the New Zealand Royal Society. 85(4):647–658. Plates 47–49.
- Begg JG, McSaveney MJ. 2005. Wairarapa fault rupture–vertical deformation in 1855 and a history of similar events from Turakirae Head. In: Townend J, Landridge R, Jones A, editors. Proceedings of the 1855 Wairarapa earthquake symposium: 150 years of thinking about 8+ earthquakes and seismic hazard in New Zealand; Sep 8–10; Museum of New Zealand Te Papa Tongarewa. Wellington: Greater Wellington Regional Council; p. 21–30.
- Berryman K, Cochran U, Wallace L. 2015. Cut and thrust. In: Graham IJ, chief editor. A continent on the move: New Zealand geoscience revealed. 2nd ed. Wellington: GSNZ Miscellaneous Publication 124; p. 142–145.
- Berryman KR, Ota Y, Hull AG. 1989. Holocene paleoseismicity in the fold and thrust belt of the Hikurangi subduction zone, eastern north Island, New Zealand. Tectonophysics. 163:185–195. doi: 10.1016/0040-1951(89)90256-4
- Berryman K, Ota Y, Miyauchi T, Hull A, Clark K, Ishibashi K, Iso N, Litchfield N. 2011. Holocene paleoseismic history of upper-plate faults in the southern Hikurangi Subduction Margin, New Zealand, deduced from marine terrace records. Bulletin of the Seismological Society of America. 101(5):2064–2087. doi: 10.1785/0120100282
- Bradford JM. 1979. Physical and chemical oceanographic observations off Westland, New Zealand. New Zealand Journal of Marine and Freshwater Research. 17(1):71–81. doi: 10.1080/00288330.1983.9515988
- Castilla JC. 1988. Earthquake-caused coastal uplift and its effects on rocky intertidal kelp communities. Science. 242(4877):440–443. doi: 10.1126/science.242.4877.440
- Castilla JC, Manriquez PH, Camaño A. 2010. Effects of rocky shore coseismic uplift and the 2010 Chilean mega-earthquake on intertidal biomarker species. Marine Ecology Progress Series. 418:17–23. doi: 10.3354/meps08830
- Clark K. 2017. Coastal uplift along North Canterbury-Marlborough coast: results from coastal survey team. GeoNet. [accessed 10 June 2019]. https://www.geonet.org.nz/news/2bzLb7U5A86YgWCGS62uq2
- Clark KJ, Nissen EK, Howarth JD, Hamling IJ, Mountjoy JJ, Ries WF, Jones KJ, Goldstein S, Cochran UA, Villamor P, et al. 2017. Highly variable coastal deformation in the 2016 MW7.8 Kaikōura earthquake reflects rupture complexity along a transitional plate boundary. Earth and Planetary Science Letters. 474:334–344. doi: 10.1016/j.epsl.2017.06.048
- Cooper A, Norris R. 2015. The great divide. In: Graham IJ, chief editor. A continent on the move: New Zealand geoscience revealed. 2nd ed. Wellington: GSNZ Miscellaneous Publication 124; p. 120–121.
- Cullen DJ. 1966. Submarine evidence from New Zealand of a rapid rise in sea level about 11,000 years B.P. Palaeogeography, Palaeoclimatology, Palaeoecology. 3:289–298. doi: 10.1016/0031-0182(67)90022-3
- Darby DJ, Beanland S. 1992. Possible source models for the 1855 Wairarapa earthquake, New Zealand. Journal of Geophysical Research. 97(B9):12375–12389. doi: 10.1029/92JB00567
- Delépine R. 1964. Le genera Durvillea dans les Îles Australes Françaises. Comité National Français de Recherches Antarctiques. 1(7):141–147.
- Downes G, Cochran U, Wallace L, Reyners M, Berryman K, Walters R, Callaghan F, Barnes P, Bell R. 2005. Understanding local source tsunami: 1820s Southland tsunami. Wellington: Institute of Geological and Nuclear Sciences. Client Report 2005/153; v + 92 p.
- Fraser CI, Hay CH, Spencer HE, Waters JM. 2009. Genetic and morphological analyses of the southern bull-kelp Durvillaea antarctica (Phaeophyceae: Durvillaeales) in southern New Zealand reveal cryptic species. Journal of Phycology. 45:436–443. doi: 10.1111/j.1529-8817.2009.00658.x
- Fraser CI, McGaughran A, Chuah A, Waters JM. 2016. The importance of replicating genomic analyses to verify phylogenetical signal of recently evolved lineages. Molecular Ecology. 25:3683–3695. doi: 10.1111/mec.13708
- Fraser CI, Spencer HG, Waters JM. 2012. Durvillaea poha sp. nov. (Fucales, Phaeophyceae): a buoyant southern bull-kelp genus endemic to New Zealand. Phycologia. 51:151–156. doi: 10.2216/11-47.1
- Fraser CI, Winter DJ, Spencer HG, Waters JM. 2010. Multigene phylogeny of the southern bull-kelp genus Durvillaea (Phaeophycea: Fucales). Molecular Phylogenetics and Evolution. 57:1301–1311. doi: 10.1016/j.ympev.2010.10.011
- Garden CJ, Craw D, Waters J, Smith A. 2011. Rafting rocks reveal marine biological dispersal: a case study using clasts from beach-cast macroalgal holdfasts. Estuarine Coastal and Shelf Science. 95:388–394. doi: 10.1016/j.ecss.2011.10.008
- Garden CJ, Smith AM. 2011. The role of kelp in sediment transport: observations from southeast New Zealand. Marine Geology. 281:35–42. doi: 10.1016/j.margeo.2011.01.006
- Gerard VJ. 1982. In situ rates of nitrate uptake by giant kelp, Macrocystis pyrifera (L.) C. Agardh: tissue differences, environmental effects, and predictions of nitrogen-limited growth. Journal of Experimental Marine Biology and Ecology. 62:211–224. doi: 10.1016/0022-0981(82)90202-7
- Global Sea Temperatures. n.d. New Zealand sea temperatures. [accessed 10 June 2019]. https://www.seatemperature.org/australia-pacific/new-zealand/
- Gorman P. 2019. Scientists discover alpine fault’s last big earthquake more recent than first thought. Wellington: Stuff. [accessed 20 June 2019]. https://www.stuff.co.nz/science/113563015/scientists-discover-alpine-faults-last-big-earthquake-more-recent-than-first-thought
- Graham IJ, chief editor. 2015. A continent on the move: New Zealand geoscience revealed. 2nd ed. Wellington: Geoscience Society of New Zealand in association with GNS Science.
- Grapes R. 2000. The day the earth shifted. New Zealand Geographic. 46:45–56.
- Hamling IJ, Hreinsdóttir S, Clark K, Elliott J, Liang C, Fielding E, Litchfield N, Villamor P, Wallace L, Wright TJ, et al. 2017. Complex multifault rupture during the 2016 Mw 7.8 Kaikōura earthquake, New Zealand. Science. 356(6334):154–164. doi: 10.1126/science.aam7194
- Hare J. 1992. Paterson Inlet marine benthic assemblages: report of coastal investigations. Invercargill: Department of Conservation Technical Series No. 5.
- Harrington HJ. 1958. The geology of the Kaitangata Coalfield. New Zealand Geological Survey Bulletin. 59:131, p.+ map.
- Hay CH. 1977. A biological study of Durvillaea antarctica (Chamisso) Hariot and D. willana Lindauer in New Zealand [Unpublished PhD thesis]. Christchurch: University of Canterbury; 265 p.
- Hay CH. 1987. Lessonia adamsiae sp. nov. (Phaeophyta: Laminariales) from the Snares Islands, New Zealand. New Zealand Journal of Botany. 25:295–308. doi: 10.1080/0028825X.1987.10410076
- Hay CH. 1990. The distribution of Macrocystis (Phaeophyta: Laminariales) as a biological indicator of cool sea surface temperature, with special reference to New Zealand waters. Journal of the Royal Society of New Zealand. 20(4):313–336. doi: 10.1080/03036758.1990.10426716
- Hay CH. 1994. Durvillaea (Bory). In: Akatsuka I, editor. Biology of economic algae. The Hague: SPB Publishing; p. 352–384.
- Hay CH, South GR. 1979. Experimental ecology with particular reference to proposed commercial harvesting of Durvillaea (Phaeophyta: Durvilleales) in New Zealand. Botanica Marina. 22:431–436. doi: 10.1515/botm.1979.22.7.431
- Homer L, Moore P. 1989. Reading the rocks: a guide to geological features of the Wairarapa coast. Wellington: New Zealand Geological Publications, DSIR, Landscape Publications Ltd. 64 p.
- Hornibrook NDB. 1978. Paleontology. In: Suggate RP, Stevens GR, Te Puna MT, editors. The geology of New Zealand Volume 2. Wellington: EC Keating, Government Printer; p. 407–443.
- Kaiser A, Balfour N, Holden C, Lichfield M, Gerstenberger M, D’Anastasio E, Horspool N, McVerry G, Ristau J, Bannister S, et al. 2017. The 2016 Kaikōura, New Zealand earthquake: preliminary seismological report. Seismological Research Letters. 88(3):725–726. doi: 10.1785/0220170018
- Kettles H, Smith F, Shears N. 2017. Subtidal reef and rockwall communities of the greater Foveaux strait region, Southland, New Zealand . Science for Conservation 329. Wellington: The Publishing Team, Department of Conservation. 92 p.
- Knox GA. 1953. The intertidal ecology of Taylor’s mistake, Banks Peninsula. Transactions and Proceedings of the Royal Society of New Zealand. 81(2):189–220.
- Laing RM. 1894. Art. XXXVII–the algae of New Zealand: their characteristics and distribution. Transactions of the New Zealand Institute. 27:297–318.
- Lichfield NJ, Clark KJ. 2015. Fluvial terrace formation in the lower Awhea and Pahaoa River valleys, New Zealand: implications for tectonic and sea-level controls. Geomorphology. 231:212–228. doi: 10.1016/j.geomorph.2014.12.009
- Litchfield NJ, Norris RJ. 2000. Holocene motion on the Akatore Fault, south Otago coast, New Zealand. New Zealand Journal of Geology and Geophysics. 43:405–418. doi: 10.1080/00288306.2000.9514897
- Long R. 2010. A life on Gorge River: New Zealand’s remotest family. Auckland: Random House. 304 p.
- McGlone MS. 2002. The late Quaternary peat, vegetation and climate history of the southern oceanic islands of New Zealand. Quaternary Science Reviews. 21:683–707. doi: 10.1016/S0277-3791(01)00044-0
- McKellar IC, Soons JM. 1991. Chapter 23 Fiordland. In: Soons JM, Selby MJ, editors. Landforms of New Zealand. 2nd ed. Auckland: Longman Paul; p. 505–515.
- McSaveney MJ, Graham IJ, Begg JG, Beu AG, Hull AG, Zondervan A. 2006. Late Holocene uplift of beach ridges at Turakirae Head, south Wellington coast, New Zealand. New Zealand Journal of Geology and Geophysics. 49(3):337–358. doi: 10.1080/00288306.2006.9515172
- Menge BA, Lubchenco J, Bracken MES, Chan F, Foley MM, Freidenburg TL, Gains SD, Hudson G, Leslie H, et al. 2003. Coastal oceanography sets the pace of intertidal community dynamics. Proceedings of the National Academy of Sciences of the United States of America. 100(21):12229–12234. doi: 10.1073/pnas.1534875100
- Millar AJK. 1999. Marine benthic algae of Norfolk Island, South Pacific. Australian Systematic Botany. 12:479–547. doi: 10.1071/SB98004
- Moore MF, Murdoch RC. 1993. Physical and biological observations of coastal squirts under non-upwelling conditions. Journal of Geophysical Research. 98(C11):20043–20061. doi: 10.1029/93JC01857
- Naylor MN. 1953. The New Zealand species of Durvillea. Transactions of the Royal Society of New Zealand. 80:277–297.
- Neale D. 1992. Rocky shore ecology of Ocean Beach, Jackson Head. Unpublished report for the Department of Conservation, Hokitika Office; 16 p.
- Neale D, Nelson W. 1998. Marine algae of the west coast, South Island, New Zealand. Records of the Museum of New Zealand Te Papa Tongarewa. 10:87–118.
- Nelson WA, Adams NM. 1984. The marine algae of the Kermadec Islands–a list of species. National Museum of New Zealand Miscellaneous Series. 10:1–29.
- Nelson WA, Adams NM, Fox JM. 1992. Marine algae of the northern South Island. National Museum of New Zealand Miscellaneous Series. 26:1–179.
- Nelson WA, Villouta E, Neill KF, Williams GC, Adams NM, Slivsgaard R. 2002. Marine macroalgae of Fiordland, New Zealand. Tuhinga. 13:117–152.
- New Zealand Weather Summary. 1983. www.localweather.net.nz/smf/1980-84/21/nz-weather-summary-may-1983/1070/
- Parvici E, Craw D, Waters JM. 2019. Kelp DNA records late Holocene paleoseismic uplift of coastline, southeastern New Zealand. Earth and Planetary Science Letters. 520:18–25. doi: 10.1016/j.epsl.2019.05.034
- Proctor R, Carter L. 1989. Tidal and sedimentary responses to the late quaternary closure and opening of Cook Strait, New Zealand: results from numerical modeling. Paleooceanography. 4(2):167–180. doi: 10.1029/PA004i002p00167
- Ridgway NM. 1970. Aerial radiometry of sea surface temperatures off the east coast of New Zealand. New Zealand Journal of Marine and Freshwater Research. 4:474–478. doi: 10.1080/00288330.1970.9515361
- Schiel DR. 2011. Biogeographic patterns and long-term changes on New Zealand coastal reefs: non trophic cascades from diffuse and local impacts. Journal of Experimental Marine Biology and Ecology. 400(1–2):35–51. doi: 10.1016/j.jembe.2011.02.026
- Schiel D, Gerrity S, Alestra T, Pirker J, Marsden I, Dunmore R, Tait L, South P, Taylor D, Thomsen M. 2018. Summary of impacts and changes in nearshore marine communities. In: Hendtlass C, Neale D, Shand T, editors. Shaky shores–coastal impacts and responses to the 2016 Kaikōura earthquakes. Wellington: New Zealand Coastal Society; p. 20–23.
- Setchell WB. 1893. IV on the classification and geographical distribution of the Laminariaceae. Transactions of the Connecticut Academy of Arts and Sciences. 9:333–375.
- Shears NT. 2007. Biogeography, community structure and biological habitat types of subtidal reefs on the South Island west coast, New Zealand. Wellington: Science and Technical Publishing, Department of Conservation.
- Shears NT, Babcock RC. 2007. Quantifying New Zealand’s shallow subtidal reef communities . Science for Conservation 280. Wellington: Science and Technical Publishing, Department of Conservation. 126 p.
- Shirtcliffe TGL, Moore MI, Cole AG, Viner AB, Baldwin R, Chapman B. 1990. Dynamics of the Cape Farewell upwelling plume, New Zealand. New Zealand Journal of Marine and Freshwater Research. 24(4):555–568. doi: 10.1080/00288330.1990.9516446
- South GR, Adams NM. 1976. Marine algae of the Kaikoura coast–a list of species. National Museum of New Zealand Miscellaneous Series. 1:1–71.
- Stanton BR. 1976. Circulation and hydrology off the west coast of South Island, New Zealand. New Zealand Journal of Marine and Freshwater Research. 19:445–461. doi: 10.1080/00288330.1976.9515629
- Stephenson WJ, Dickson ME, Denys PH. 2017. New insights on the relative contributions of coastal processes and tectonics to shore platform development following the Kaikōura earthquake. Earth Surfaces Processes and Landforms. 42:2214–2230. doi: 10.1002/esp.4176
- Surf-forecast.com. n.d. Requires searching by region for surf break. Tortola: Meteo365.com. [accessed 10 June 2019]. www.surf-forecast.com/breaks/
- Taylor R. 1855. Te Ika Maui or New Zealand and its inhabitants. London: Wertheim and MacIntosh.
- Taylor DI, Schiel DR. 2003. Wave related mortality of habit-forming algae from different exposures in southern New Zealand: the importance of ‘stickability’. Journal of Experimental Marine Biology and Ecology. 290(2):229–245. doi: 10.1016/S0022-0981(03)00094-7
- Thomsen MS, Mondardini L, Alestra T, Gerrity S, Tait L, South PM, Lilley SA, Schiel DR. 2019. Local extinction of bull kelp (Durvillaea spp.) due to a marine heatwave. Lausanne: Frontiers in Marine Science. [accessed 21 June 2019]. https://www.frontiersin.org/articles/10.3389/fmars.2019.00084/full
- Tide predictions. n.d. Wellington: Land Information New Zealand; [accessed 10 June 2019]. https://www.linz.govt.nz/sea/tides/tide-predictions
- Weber XA, Edgar GJ, Banks SC, Waters JM, Fraser CI. 2017. A morphological and phylogenetic investigation into divergence among sympatric Australian bull kelps (Durvillaea potatorum and D. amatheiae sp. nov.). Molecular Phylogenetics and Evolution. 107:630–643. doi: 10.1016/j.ympev.2016.12.027
- Williams PW, McGlone M, Neil H, Zhao J. 2015. A review of New Zealand palaeoclimate from the last Interglacial to the global last glacial maximum. Quaternary Science Reviews. 110:92–106. doi: 10.1016/j.quascirev.2014.12.017
- Young DJ. 1978. South Westland. In: Suggate RP, editor. The geology of New Zealand. Volume 2. Wellington: EC Keating, Government Printer; p. 501–503.
Appendix to Figure 1
Detail of sites and observers. The author’s observations denoted with (!) and dates. Other observers: (FTW) FT Williams; (GR) Geoff Rennison; HW (Hugh Wilson); (PF) Peter Fletcher; (SK) Sandra King; (TMCH) Tom Hay; (KJW) Kerry-Jayne Wilson; (NMA) Nancy M Adams.
1 Fossil Point: KJW 2 Sep 1975; GR 2 Sep 1975. 2 Archway Islands: KJW Jul 1975;! Dec 1985; Anderson Oct 1996 WELT A21871 in Neale and Nelson (Citation1998). 3 Reef off Kahurangi Light:! Dec 1998; Neale in Neale and Nelson (Citation1998). 4 Wekakura Point, north of Kohaihai River: Neale in Neale and Nelson (Citation1998). 5 Cape Foulwind:! Sep 1973, Oct 1978, 1986; Nelson Mar 1996 WELT A21374 in Neale and Nelson (Citation1998). 6 Constant Bay, Charleston: TMCH Apr 1970;! Jan 1972, Sep 1973, Oct 1978, 1986; Neale Jan 1995 in Neale and Nelson (Citation1998); KJW May 2019. 7 Woodpecker Bay:! Sep 1973, Oct 1978. 8 Punakaiki:! Sep 1973, Oct 1978, Dec 1996. 9 Twelve Mile Bluff: TMCH 1982;! 1996; Greig’s Coast Road: Neale in Neale and Nelson Citation1998. 10 Okarito Bluff: KJW Aug 1972; PF 23 Jun 1975; Neale Sep 1995 WELT A21786 in Neale and Nelson (Citation1998). 11 Gillespies Point: GR 17 Jun 1975; Neale in Neale and Nelson (Citation1998). 12 Bruce Bay: Lawn Feb 1948 CHR 63516 in Neale and Nelson (Citation1998). 13 North side Caswell Sound entrance:! Apr 1991. 14 Easternmost of the outer Gilbert Islands, entrance to Breaksea Sound:! Apr 1991. 15 west coast of Five Fingers Peninsula, Resolution Island:! Mar 1972. 16 Anchor Island:! Mar 1972. 17 West Cape:! Mar 1972. 18 Chalky Island:! Mar 1972; Small Craft Harbour Islands, Cunaris Sound:! Apr 1991. 19 Puysegur Point, below Light:! Feb 1972. Coal Island: Kettles et al. (Citation2017). 20 Windsor Point:! Feb 2014; Gates Harbour:! Mar 1972, Feb 2011, Feb 2014. 21 Solander Islands, North-east Bay: KJW Jan 1973, Feb 1973. 22 Grace Burn; Green Islets; Andrew Burn:! Feb 2011, Feb 2012. 23 Big River (draining Lake Hakapoua); west and east sides and Prices Harbour:! Dec 2010, Feb 2011, Feb 2012, Dec 2015. 24 Sandhill Point:! Jan 1972, Dec 2010, Apr 1993, Feb 201, Feb 2012. 25 Port Craig and Blow Holes (2.5 km S of Track Burn):! Jan 1972, Apr 1993, Jan 2011, Feb 2012. 26 Wakaputa and Oraka Points:! Jan 1972. 27 The Rocks, Riverton:! Jan 1972, 1993; Pig Island: Kettles et al. (Citation2017). 28 Between Barracouta Point and Bluff:! 1972-3, 1990; Tiwai Point: Kettles et al. (Citation2017). 29 North Red Head:! Dec 2012; N and S ends of West Ruggedy Beach:! Jan 2014. 30 SW shore Codfish Island: SK 28 Mar 1988. 31 Ernest Islands, S end Mason Bay:! Mar 2013. 32 N and S ends of Doughboy Bay:! Mar 2013. 33 Broad Bay: Willa Aug 1946 Herb Lindauer No. 8823 = AKU 89, designated LECTOTYPE by Hay (1979; Figure 12). 34 Port Pegasus:- Pearl Island; S end Nobel Island; northern islet of The Sisters: SK 18 Oct 1988. 35 Wharepuaitaha Island, Titi or Muttonbird Islands: KJW 1973. 36 Tia Island, entrance to Port Adventure: KJW 1973. 37 Paterson Inlet entrance:- Ringaringa and Moses Nugget: Willa Oct 1945, Herb Lindauer No. 6700 AKU; Norris Jan 1963, WTU 244612 and Conway and Adams Nov 1971 in Adams et al. (Citation1974);! Feb 1974, 2006–2019. Sydney Cove: Hare (Citation1992). NE corner Ulva Island:! Feb 1988. 38 Approaches to Paterson Inlet:- Bunker Islets: KJW 1973. S side Bench Island; E side Native Island; Mamaku Point:! Feb 1988. 39 Waipapa Point:! Jan 1972, Mar 2014. 40 Slope Point:! Jan 1972, Mar 2014. 41 Curio Bay:! 1972-75, Mar 2014. 42 Chaslands Mistake:! Feb 1973, 2016. 43 Tautuku Peninsula:! 1972–76, 2010. 44 Long Point:! Jan 1972, Mar 1973. 45 Cannibal Bay:! Jan 1972; Tuhawaiki Island, Catlins River Mouth:! Oct 1990. 46 Nugget Point:! Jan 1972, Oct 1990. 47 N end Crystalls Beach; Bull Creek:! Jul 2012, Jun 2019. 48 Quoin Point; reef S of Taieri River Mouth:! Jul 2012, Jun 2019. 49 Rocks S of St. Clair, Dunedin:! 1972–73, 2004–2019; NMA Feb 1981. Brighton: NMA Feb 1981. 50 Cape Saunders, Otago Peninsula: KJW 1972–73;! 1974. 51 Little Papanui, Otago Peninsula: Batham (Citation1958; Figure 4, Pl 48, fig 3). 52 Otago Harbour entrance:- Taiaroa Head; The Mole:! 2004-2019. 53 S side Karitane Peninsula:! Oct 1990. 54 Shag Point:! 1972–74; NMA Feb 1981. 55 Katiki and Moeraki Points:! 1972–74 Oct 1990. Orore Point: NMA Feb 1981. 56 Kakanui:! Jan 1972. 57 Cape Wanbrow, Oamaru:! Oct 1990. 58 Timaru:- Dashing Rocks, Smithfield; rocks at outlet of Washdyke Lagoon:! Oct 1989. 59 Te Oka Bay, Banks Peninsula:! Jan 1972, 1975. 60 Gateway Point near Akaroa Head:! Jan 1972, Mar 1976, 1989. 61 Hickory Bay, Banks Peninsula:! Jan 1972. 62 Okains Bay, Banks Peninsula:! Jan 1972; KJW 1973; HW May 2019. 63 Boulder Bay; rocks below Godley Head near WWII searchlight batteries:! Jan 1972, Dec 1996. Taylor’s Mistake: Knox (Citation1953, Pl 15). 64 Napenape:! Apr 1973. 65 Haumuri Bluffs:! Apr 1973. Oaro:! 1972-76; NMA Nov 1973, WELT A8282. 66 Kaikōura Peninsula:! various dates 1972-76. 67 Ohau Point:! 1972-76. 68 Kekerengu:! 1972-74. 69 Long Point:! 1972. 70 Cape Campbell: TMCH 1973. 71 Robertson Point; entrance to Port Underwood:! Sep 1995. 72 Fighting Bay:! Mar 1990. 73 Tory Channel:! 1972, 1996. 74 The Brothers: FTW 17 Feb 1975. 75 Castle Point, reef S of Light:! Mar 1993. 76 Whakataki: Moore Mar 1947 CHR 57280 in Adams (Citation1972). 77 Mataikona: Hoggard May 1970, WELT A3757 in Adams (Citation1972).