ABSTRACT
Interactions between plants and animals often play a major role in the ecology of both partners. While such interactions are well studied in vascular plants such as angiosperms and gymnosperms, they are less well understood in bryophytes. However, an extensive search of the scientific literature reveals several cases of known and suspected interactions between bryophytes and animals suggesting these relationships could be more widespread than previously thought. Here we discuss and synthesise research and observations concerning such relationships, termed here ‘bryo-zoophily’. These relationships can facilitate both fertilisation events as well as reproductive propagule dispersal. Using known examples such as the relatively well-documented signalling and dispersal strategy seen in the dung mosses (Splachnaceae) and laboratory-based experiments documenting microarthropod mediated sperm transfer, we explore potential signalling in bryophytes, such as the production of visual and chemical lures. Dispersal adaptations, such as the production of sticky mucilage, can be seen in various moss taxa and we discuss potential fitness benefits derived, such as dispersal to geographically and temporally limited habitat, increased sexual reproduction, and increased genetic heterogeneity. We identify three hallmarks of bryo-zoophily as the production of lures to attract potential dispersal agents, adaptations to facilitate dispersal of propagules or genetic material, and life histories potentially advantaged by zoophily. We conclude that bryo-zoophily is probably much more widespread than is currently understood and conclude with remarks about directions for possible research to further investigate this largely unexplored field.
Introduction
Many plant species rely heavily on associations with animal partners to disperse both propagules and pollen. Much attention has been paid to angiosperm adaptations to attract animals such as fruit and flowers; however, angiosperms only appeared relatively recently in the evolution of plants, possibly as early as 250 million years ago, definitively appearing in fossil records around 140 million years ago (Bell et al. Citation2005, Citation2010; Smith et al. Citation2010). Bryophytes appear much earlier, dating back to the first colonisation of land by the ancestors of all modern plants around 470 million years ago (Wellman et al. Citation2003). Some ancient lineages of land-dwelling invertebrates such as slugs and mites are known to consume bryophyte parts and in doing so move them about the landscape (Boch et al. Citation2015; Ignatov et al. Citation2017), hinting at the possibility that animals and bryophytes have been interacting since the colonisation of land. Evidence suggests that many bryophytes benefit from incidental interactions with a diversity of invertebrates and vertebrates (Kimmerer and Young Citation1995, Citation1996; Boch et al. Citation2015), and a growing body of research continues to document unusual structural and chemical adaptations () and suggest a reliance by some bryophyte species on animal partners (Ignatov and Ignatova Citation2001; Marino et al. Citation2009; Wyatt et al. Citation2022). Bryophytes may therefore represent an opportunity to explore the origins of plant-animal interactions and the development and transition of those from more passive and generalist interactions to more specialised and mutualistic relationships, as seen in higher order plants today. There may be many bryophytes that accrue low-level fitness benefits from incidental interactions with a diversity of insects and micro-invertebrates. At the other extreme, there is the well-documented relationship between Splachnaceae mosses and their insect partners (Marino et al. Citation2009) and growing evidence strongly suggestive that such relationships may occur in other bryophytes (Ignatov and Ignatova Citation2001; Cronberg et al. Citation2006; Rosenstiel et al. Citation2012; Wyatt et al. Citation2022).
Figure 1. Bryo-zoophily in action? (1) Polytrichum juniperinum ‘splash cups’ – structure produced in a variety of moss taxa. Thought to aid in sperm dispersal through physical splashing, could these bright colours indicate signalling to arthropod partners? Photograph: Stewart Zeyl with permission (2) Tetraplodon mnioides, a northern hemisphere dung moss – produces volatiles similar to those found in blueberries prior to capsules opening and decaying meat after opening. Photograph: Ryan deRegnier (3) Tetraphis pellucida vegetative – vegetative propagules held clear of surrounding vegetative structures. Photograph: Michael Lueths with permission (4) Acaulon muticum – small orange cleistocarpus capsules mimicking small berries? Photograph: Johannes Merz with permission (5) A mite positioned headfirst in the pergonium of a Philonotis spp. Photograph: Ryan deRegnier (6) Splachnum luteum – brilliant yellow sporophytes produced by this moose-dung specialist moss in the northern hemisphere. Photograph: Thorsten Stegman with permission.
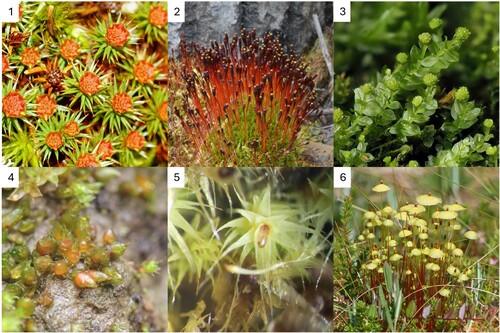
Bryophytes, consisting of mosses, liverworts, and hornworts, are the most basal group of plants (Morris et al. Citation2018) and possess conserved reproductive strategies which are not typically considered to involve animal partners. The different reproductive strategies of bryophytes and angiosperms make it likely that bryophyte interactions will involve different life stages and reproductive structures than is typical for angiosperm-animal interactions (e.g. fruit, pollen, etc.); however, the end goals, sexual reproduction and dispersal of propagules, remain largely the same. While all plant groups display an alternating lifecycle between a haploid and diploid (or polyploid) phase (Glime Citation2017b), bryophytes differ from other plant groups in that their haploid, or gametophyte, phase is dominant. The gametophyte germinates from a haploid spore and eventually grows sexual structures, antheridia, and archegonia, which produce male and female gametes respectively. The egg is housed and is fertilised in the archegonium and this gives rise to the diploid or sporophyte phase. The sporophyte, which is dependent on the gametophyte for support, facilitates sexual reproduction through the production of haploid spores (Glime Citation2017b). While spores are thought to be the major driver of mid to long range dispersal in bryophytes, asexually produced propagules as well as vegetative fragments play an important role in shorter range dispersal (Miles and Longton Citation1992; Kimmerer and Young Citation1995; Hedenås et al. Citation2003). Thus, interactions with animals may involve the dispersal of motile sperm cells, spores, asexual propagules, or vegetative fragments, all of which could advantage successful reproduction and colonisation of new or rare habitats for bryophytes.
Bryophytes face two reproductive challenges which are analogous to those of their more recently evolved angiosperm counterparts. The first is the fertilisation of egg cells by sperm. This is broadly similar to pollination in angiosperms and gymnosperms, wherein haploid gametes combine to give rise to a new diploid generation. The second is the dispersal of propagules, which is analogous to seed dispersal in gymnosperms and angiosperms, wherein a unit of reproduction is dispersed across the landscape with the function of colonising new habitats. It is generally held that both of these processes are, in bryophytes, achieved primarily through abiotic means via the presence of liquid water in the case of sperm cells and the action of wind and water in the case of propagules (Glime and Bisang Citation2017). This means that bryophyte reproduction and colonisation of new habitats is largely left up to the chance convergence of reproductive stages with ideal environmental conditions. However, whether distribution patterns are a result of these random abiotic processes remains untested in many bryophyte species.
Bryophytes are generally held to be efficient at dispersal via airborne spores (Sundberg Citation2005; Sundberg et al. Citation2006). Direct experimental evidence suggests that while spore densities rapidly decline with distance from a sporophyte, a consistent low-level density persists over much greater distances (Lönnell et al. Citation2012) while indirect approaches measuring genetic relatedness show an inverse isolation effect at large distances indicating steady low-level dispersal of propagules from a wide range of sources (Vanderpoorten et al. Citation2019). A number of adaptations observed in the sporophytes of bryophytes, specifically hygroscopic movements seen in the peristome of mosses (Gallenmüller et al. Citation2018) and capsule dehiscence and elater function in liverworts (Glime Citation2017a), are thought to facilitate spore release at opportune climatic conditions (e.g. low humidity) to maximise airborne dispersal. Despite this, the existence of endemism, sometimes extreme (Patiño et al. Citation2013), in bryophyte floras (Delgadillo Moya Citation1994) as well as adaptations which would preclude airborne dispersal such as capsules which fail to open at maturity (cleistocarpous) (Glime Citation2017a) and sticky mucilage binding spore masses (Koponen Citation1990; Ignatov and Ignatova Citation2001; Marino et al. Citation2009; Wyatt et al. Citation2022) seems to indicate that many bryophyte species are not effectively dispersed over large distances via air movements. While adaptation to specific microclimates and thus preclusion from other habitats may provide an adequate explanation for the former, it does little to explain the latter. One possible explanation is that such bryophytes have evolved alternative means of dispersal. A growing body of research provides tantalising hints that relationships with certain animals may play a larger role than previously guessed in the ecology of at least some bryophytes.
The goals of this review are to draw together and analyse reports and studies on the interactions and possible interactions between animals and bryophytes as they relate to reproduction, with an emphasis on identifying actual and potential traits and adaptations to facilitate this interaction, particularly moss odour and colour signals, and adhesive spores and propagules. We consider the fitness benefits for bryophytes, especially how animal-mediated dispersal could enhance competitive ability to colonise new habitat, and how animal-mediated sperm transfer could increase bryophyte sexual reproduction and outcrossing. We hypothesise that these interactions are much more widespread than conventionally thought and that adaptive relationships between animals and bryophytes play a critical role in ecology at this scale.
Methods
Google Scholar was searched for publications, in English, on the basis of varying combinations of search terms ‘bryophyte’, ‘moss’, ‘liverwort’ ‘interaction’, ‘dispersal’, ‘invertebrate’, ‘directed dispersal’, ‘insect mediated’, ‘invertebrate mediated’, ‘animal mediated’, and ‘fertilisation’. Publications were selected based on a review of firstly their title and secondly, their abstract. We aimed to incorporate any study or observation which showed actual adherence of bryophyte sperm or propagules to an animal, increased bryophyte fitness (reproduction or sexual or asexual activity) in the presence of animals, altered behaviour by animals in the presence of bryophytes or bryophyte reproductive structures, dispersal of propagules or sperm in controlled environments (i.e. a laboratory), or in situ dispersal of viable reproductive structures (i.e. spores, fragments, propagules) or sperm. Also included were publications with speculations on the functionality of certain traits in respect to their possible role in animal-mediated dispersal of propagules or sperm.
Results
We found 37 papers () detailing in situ observations of the dispersal of reproductive material (30 publications), experimental studies investigating dispersal (4), and reviews of the evidence of such phenomena (3). The publications covered a variety of bryophyte taxa encompassing 17 families of mosses and liverworts being dispersed (or potentially dispersed) by arthropods (15 publications), birds (7), mammals (3), and molluscs (3). Publications examined covered a range of material being dispersed (or potentially dispersed) including spores (10 publications), fragments (8), propagules (5), and sperm (5). Several studies were not clear on exactly what material was being dispersed and in one study the material being dispersed was unknown. Studies into northern hemisphere Splachnaceae spore dispersal by flies are heavily represented, but potential spore dispersal by arthropods is noted in two other moss families, the Amblystegiaceae and Schistostegaceae. Invertebrates such as millipedes, ants, slugs, and snails are also implicated in the dispersal of asexual propagules across a variety of different bryophyte families (Kimmerer and Young Citation1995; Rudolphi Citation2009; Boch et al. Citation2013, Citation2015; Zona Citation2013; Pasiche-Lisboa and Jesús Citation2018). Vertebrate interaction with bryophytes is largely limited to the dispersal of moss and liverwort fragments either internally or externally (Staaland et al. Citation1979; Moen et al. Citation1993; Heinken et al. Citation2001; Osorio-Zuñiga et al. Citation2014; Wilkinson et al. Citation2017). Of the three divisions within bryophytes, papers reviewed primarily examined mosses (Bryophyta). Seven papers reviewed examined interactions between liverworts (Marchantiophyta) and animals. Six of these documented the movement of spores and viable plant fragments by slugs, mammals, and birds. No papers were found documenting interactions between hornworts (Anthocerotophyta) and animals. There is a clear trend towards the publication of such interactions () in the years since 1991. These publications are overwhelmingly focused on systems in Europe and North America, however many of these same families, genera and species are also present in Oceania.
Figure 2. Publications per decade as presented as average publications per year for decades 1991–2000, 2001–2010, 2011–2020, and 2021–2030. Very few publications documenting interactions between bryophytes and animals previous to 1991 were examined and as such were lumped into the same bin.
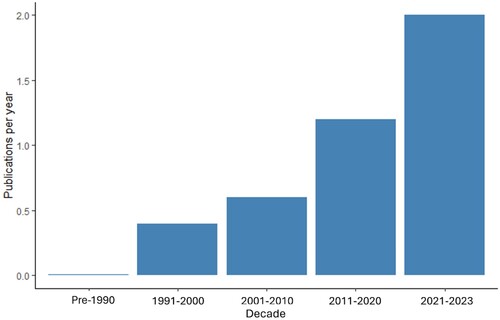
Table 1. Publications (37) examined in this review.
Discussion
Publications examined demonstrate a diverse array of interactions between bryophytes and animals. Some of these publications, most notably those examining northern hemisphere Splachnaceae (i.e. Koponen Citation1990; Marino et al. Citation2009; etc.), detail a thoroughly demonstrated interaction between mosses and their insect dispersers from the luring of a dispersal agent to eventual colonisation of suitable substrate. Others such as the elegant microcosm experiments by Cronberg et al. (Citation2006) and Rosenstiel et al. (Citation2012) demonstrate persuasively that micro-invertebrates, specifically Collembola and Oribatid mites, provide a reproductive benefit to at least some mosses in moving sperm across space which it would not otherwise be able to cross. Despite this, the exact nature of the relationship and the potential mechanisms underlying it remain unexplored in many cases examined. Observations such as the massing of Collembola on a reproductive moss (Bisang and Hedenäs Citation2015) or the movement of gemmae by invertebrates (Cronberg et al. Citation2008; Rudolphi Citation2009; Zona Citation2013) suggest the presence of a relationship but are only a first step in the exploration of what could be a widespread phenomenon. Such interactions likely span a range of degrees of relationship complexity from those in which the bryophyte partner benefits from the ordinary movements of animals within its environment with little adaptation on the bryophyte’s part or benefit to the animal in question to those with evolved signalling and dispersal strategies as well as rewards and benefits for the animal partner in question. However, that such interactions are occurring is a certainty. Using our understanding of how such relationships occur in vascular plant systems as well as those which are well documented in bryophytes (i.e. Splachnaceae) we will provide a starting point and a framework for moving forward with the exploration of this space.
Signalling in mosses
There is strong evidence that for angiosperms, fruits and flowers evolve visual and olfactory signals in order to attract dispersers and pollinators respectively (Willson and Whelan Citation1990; Johnson et al. Citation2000). The degree of specialisation can sometimes be extreme, for example, when the presence of effective pollinators is predictable (Johnson et al. Citation2000). This process has long been thought to be the province of more recently evolved plants, in particular angiosperms. However, the case studies and evidence we have compiled point to signalling adaptations potentially being present long before the evolution of such plants.
Many species of entomophilous Splachnaceae possess unique structural characteristics and colour patterns which are thought to be adaptations to aid in the attraction of potential insect dispersers (Koponen Citation1990). These visual lures can vary from the dramatically inflated and coloured structures present at the base of capsules (hypophysis) characteristic of the mosses of the genus Splachnum to the more understated wine-red capsules and setae of the genera Tayloria and Tetraplodon (Marino et al. Citation2009). A number of distinctive commonalities in visual display seem to be consistent across many genera of Splachnaceae including capsules which are held erect and yellowish spore masses exuding from the capsule mouth (Koponen Citation1990). While the role that the various shapes and degree of enlargement seen in the sporophytic structures of entomophilous Splachnaceae mosses may play in luring spore dispersers is the subject of speculation, the colours observed bear striking similarities to trends seen in angiosperm flowers. The presence of reddish pigments in the sporophytes of many species is consistent with the reddish colouration seen in flowers specialised to lure coprophilous insects, particularly flies where the reddish colouration may mimic potential oviposition sites (Chen et al. Citation2015). The colour yellow is also noted for its attractiveness to flies often being referred to as a ‘super-normal’ stimulus (Kelber Citation2001; Lunau Citation2006; Shi et al. Citation2009; Campbell et al. Citation2010). The yellow colouration of the dramatic sporophyte of Splachnum luteum and the spore masses present in many Splachnaceae species, often in conjunction with reddish capsules underneath, could plausibly harness this powerful stimulant in an effort to effectively disperse spores. Other colours noted such as the dark, almost black capsules of Splachnum sphaericum or the white capsules seen in Tayloria gunii and T. tasmanica, both endemic to Tasmania (Glime Citation2017a), are less understood. The role these colourful pigments in combination with unusual sporophytic structures play in the formation of an effective lure merits attention.
In conjunction with visual displays, olfactory lures are thought to play a major role in the dispersal strategy of the entomophilous Splachnaceae mosses (Koponen Citation1990). In the effort to locate resources of value (i.e. oviposition sites, food, etc) insects utilise a variety of chemoreceptors to detect volatile compounds corresponding to the species’ individual preferences (Depetris-Chauvin et al. Citation2015). A large number of volatile compounds are produced in the sporophyte capsule and hypophysis of entomophilous Splachnaceae mosses, many of which are broadly linked to compounds known from animal origins such as dung or carcasses (Pyysalo et al. Citation1978, Citation1983; Marino et al. Citation2009). Compounds such as indoles, cresols, and phenols, common in herbivore dung, as well as dimethyl disulphide and dimethyl trisulphide, characteristic of decaying animal tissue, have been isolated from several species of Splachnaceae from North America, South America, and Australia, while these compounds were found to be absent in the sporophytes of anemophilous Splachnaceae mosses from similar habitats (Marino et al. Citation2009). The sensitivity of an insect’s chemoreceptors to a certain compound or combination of compounds could facilitate the attraction of a specific suite of potential dispersers in a similar way as sexually deceptive orchids are able to precisely mimic pheromones used in the mating system of their target pollen disperser (Gaskett Citation2011), allowing for niche differentiation through the production of a specific bouquet of volatile compounds. Similarly, such specialised chemical signalling could be achieved through a ‘strength of signal’ approach rather than a specifically tailored chemical lure as seen in a South African carrion-mimic orchid where a low volume of volatile compounds produced is thought to target a specific subset of carrion flies which specialise on the colonisation of small carcasses (van der Niet et al. Citation2011). As it appears that the disperser communities play the dominant role in the colonisation of new substrates (Marino Citation1991b), these unique chemical profiles, along with the visitation of different disperser species, could provide evidence of niche partitioning among coexisting species of Splachnaceae.
While signals in the entomophilous Splachnaceae are the most comprehensively studied and are perhaps the most immediately conspicuous to human senses, many moss species possess unusual colour patterns or striking structural traits which remain largely unexplored but may well facilitate moss-animal interactions. Schistostega pennata, a moss known for its luminous properties achieved through structural adaptations in the cell walls of its protonema (Ignatov and Ignatova Citation2001), is suspected of engaging animals in spore dispersal based on its specialised habitat, rapid range expansion, and suspect adaptations (Ignatov et al. Citation2017). Oribatid mites have been observed feeding on S. pennata spores and subsequently becoming covered in intact sticky spores, raising the possibility that these spores may be dispersed by Oribatid mites (Ignatov et al. Citation2017). In contrast to the dispersers of Splachnaceae, these Oribatid mites appear to actively feed on the spores and so this relationship represents a potential mutualism as opposed to the deception seen in the Splachnaceae, where the insects receive no benefit or reward for their interaction with the moss. However, the slow movement of these mites and their apparent repulsion to light would suggest that while these organisms may play a role in dispersal of moss within a relatively local habitat, other organisms associated with the moss such as dipterans and coleoptera may be involved in its dispersal across longer distances.
Schistostega pennata produces at least two potential visual lures. The brownish-purple colouration of the capsule to contrast a yellow-green spore mass has been compared to those capsules with similar colouration seen in entomophilous Splachnaceae where it is strongly suspected of playing a role in luring flies to facilitate dispersal (Marino et al. Citation2009). The luminous nature of the persistent protonema of this moss for which it is so well known has been proposed as a potential lure (Ignatov et al. Citation2017). While it is generally thought the structure of the cell wall which produces this luminousness is an adaptation to focus low levels of light onto chloroplasts (Ignatov and Ignatova Citation2001) (similar in concept to the tapetum lucidum found in many vertebrate eyes), this adaptation could have knock-on effects as potential signalling. Moreover, the cellular structure of the setae gives it an appearance which is lighter than surrounding environment in the dark habitat of this species. Having been described as almost fluorescent by human observers, these pellucid setae have also been proposed as potential visual lures (Ignatov et al. Citation2017). Various families within the order Diptera have been observed within the unique microhabitat of this species including flies in the family Dolichopodidae which were noted to have spores stuck to their legs that were ‘probably’ those of S. pennata (Ignatov and Ignatova Citation2001). These pellucid setae and luminous protonema could function as light lures in a darkened habitat in much the same way that bioluminescence is thought to act as a lure in cave-dwelling glowworm larvae and fluorescence in some marine organisms (Willis et al. Citation2011; Haddock and Dunn Citation2015), however this remains untested.
Other potential visual attractants include unusually structured and coloured sporophyte capsules similar to those seen in Splachnaceae. Pleurophascum grandiglobum possesses large, green, globular capsules, traits that could be consistent with an adaptive visual lure mimicking fruit or similar rewarding plant matter. It has been noted that for P. grandiglobum many capsules appear to be grazed on by some animals and it is uncommon to see mature capsules present from one year to the next (Glime Citation2017a). Species in the genus Voitia (Splachnaceae), are known to grow exclusively on dung; however, unlike other Splachnaceae their capsules are cleistocarpous and they do not display entomophily (Goffinet et al. Citation2004). How Voitia spores reach such a specific habitat type is unexplored. However, it has been noted these capsules, less than a centimetre in diameter and coloured a reddish brown to deep purple at maturity, bear a strong resemblance to fruits of herbaceous plants which often grow alongside moss colonies (Glime Citation2017a). Several other species of moss also possess similar spherical or ‘inflated’ capsules which do not open at maturity such as Pleurophascum, Pleuridium, and cleistocarpous mosses within the Pottiaceae (Glime Citation2017a) which bear a marked visual similarity to berries or other small fruits. Grazing on large capsules is a commonly observed, but poorly documented phenomenon in other moss taxa (). In addition to this visual strategy a chemical lure could be being deployed in the case of Tetraplodon mnioides, another Splachnaceae, which produces volatile compounds in its unopened capsules which resemble those found in ripe blueberries. These volatiles are produced until the capsules open when odours mirroring those of decaying animal matter are produced instead (Lüth Citation2010). This combination of both fruit or floral odours and rotting odours is also present in other deceptive plants, e.g. carnivorous pitcher plants (Di Giusto et al. Citation2010) and could effectively broaden the potential suite of dispersers as well as reduce adaptive pressure on any one taxon in response to deceptive signalling.
Figure 3. The missing capsules. A mixture of grazed and ungrazed green, globose capsules on Philonotis scabrifolia (left) and a colony of Bryum spp. with nearly all its capsules grazed (right). The culprit grazer is, in both instances, unknown. Photographs: Ryan deRegnier.
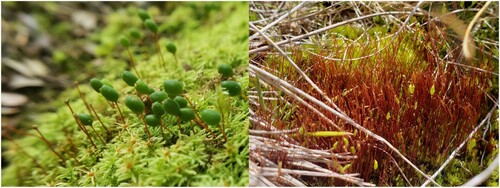
Signals could also be involved in bryophyte fertilisation events. In at least two species of moss, Collembola (springtails) and Oribatid mites seem to show a preference for fertile moss shoots over sterile shoots and a slight affinity for shoots bearing archegonia over those shoots bearing antheridia (Cronberg et al. Citation2006; Rosenstiel et al. Citation2012) Antheridia and archegonia of moss are known to produce a number of substances thought to aid in dispersing sperm and guiding them to the egg cells housed in the archegonia (Paolillo Citation1979; Kaiser et al. Citation1985). Lipids produced are thought to play a role in breaking surface tension of water films and allowing sperm cells to disperse greater distances (Muggoch and Walton Citation1942). Sucrose produced in the archegonia has been shown to play a dominant role in enabling sperm cells to find mature archegonia and navigate their way into the structure to the egg cell (Kaiser et al. Citation1985). Additionally, a number of volatile organic compounds are produced by antheridia and archegonia (Rosenstiel et al. Citation2012). The profile of this mixture of compounds varies according to the sexual structure producing it (Rosenstiel et al. Citation2012). What role these substances, and potentially others as yet undocumented, could potentially play in the attraction of micro-invertebrates to reproductive structures remains unknown; however, the possibility that these substances are used as an energy source in the case of lipids and sugars or direct chemical signalling in the case of the sex-specific volatile organic compounds warrants further investigation.
As well as sex-specific volatiles or nutrient provisioning, colour patterns of the sexual structures of bryophytes may also be involved in the attraction of animals to facilitate sperm transfer. In many cases, these colour patterns may be too small for humans to easily detect. However, a notable exception to inconspicuousness of sexual structures are found in mosses and some liverworts, which produce ‘splash cups’: structures ostensibly adapted to take advantage of raindrops or other falling water to propel sperm much farther than would be possible under normal circumstances (Brodie Citation1951; Clayton-Greene et al. Citation1977; Reynolds Citation1980). In addition to their unique structure, many of these ‘cups’ are distinctly coloured, perhaps most noticeably in some Polytrichum species where bright colourations are produced in the perigonial leaves forming these cups (Paolillo Citation1979; ). While the mechanics and effectiveness of the ‘splash’ has been studied in mosses which produce these cups (Brodie Citation1951; Clayton-Greene et al. Citation1977), less attention has been paid to bright colouration seen in some species. Colours such as bright red are commonly seen in other insect dispersed organisms (Chen et al. Citation2015). The presence of such colours in the reproductive structures of certain mosses could be an adaptation to facilitate a mode of sperm transfer in addition to the splashing mechanism. Indeed, in observations by Gibson and Miller-Brown (Citation1927) a variety of invertebrate were noted to visit the splash-cups of Polytrichum commune where they ‘greedily lap’ mucilage produced and subsequently become covered in sperm cells.
Potential signalling adaptations seen in a number of moss species suggests a degree of specialisation beyond opportunistic incidental dispersal by animals. The investment of resources in visual displays, olfactory lures, or other means of animal attraction seen in the Splachnaceae mosses represents a well-documented shift away from random, abiotically driven dispersal to biotic relationships with a particular subset of animal dispersers. If confirmed through robust study, the presence of these signalling adaptations could fundamentally change our understanding of bryophyte ecology.
Structural adaptations to facilitate animal-mediated dispersal
In addition to colour and olfactory lures discussed previously, some mosses also appear to have evolved structural features to facilitate or enhance dispersal by insects. The Splachnaceae, known for their entomophily, again present the most well-studied example of adaptations for animal-mediated dispersal in mosses. The most apparent of these adaptations is the sticky material exuded from the capsule along with the spores that adheres the spores to the body parts of visiting flies (Koponen Citation1990; Marino et al. Citation2009). The spores themselves are oblong with a longitudinal indentation, a ‘coffee-bean’ shape (Marino et al. Citation2009), which could be an adaptation to increase overall surface area as well as provide more direct surface with which to stick to dispersers (Marino et al. Citation2009) In addition to these spore characteristics, the sporophytes themselves grow in such a way as to keep capsules erect and clear of the vegetative part of the moss colony (Koponen Citation1990; Marino et al. Citation2009) which could ensure that any visiting flying insect would contact spore masses upon landing.
At least two other moss species, Schistostega pennata and Anacamptodon splachnoides, are strongly suspected of engaging in invertebrate mediated spore dispersal and seem to share a number of traits generally associated with the entomophilous Splachnaceae, including similarities in the spores, sporophytic habit, and life history (Ignatov and Ignatova Citation2001; Wyatt et al. Citation2022). Spores in S. pennata, A. splachnoides, and entomophilous Splachnaceae spore seem to be reduced in size (7–15 µm), thin walled, yellowish green in colour, and encased in sticky mucilage precluding dispersal by wind. Sporophytes seen in these taxa bear erect capsules with thickening exothelial cells, which push the spore mass out of the capsule mouth. Lastly, these three mosses all grow in specialist habitat: dung and/or carcasses in the case of entomophilous Splachnaceae, holes in the trunks of trees in the case of A. splachnoides, and crevices and cavities often produced by fallen trees in the case of S. pennata (Ignatov and Ignatova Citation2001; Marino et al. Citation2009; Wyatt et al. Citation2022). Specifically, S. pennata has been documented to have several structural traits similar to those suspected or known to facilitate entomophily in the Splachnaceae family such as elliptical spores bearing a similar pitted reticulate surface ornamentation, a setae which continues to grow after capsule maturation, and a sexuality in which gametophores bearing either male or female gametangia emerge from the same protonematal structures, a phenomenon sometimes referred to as ‘pseudodioicous’ (Glime and Bisang Citation2017), ensuring a mix of both male and female shoots within the same isolated colony (Ignatov and Ignatova Citation2001). Meanwhile, in Anacamptodon splachnoides, there is seen to be a constriction below the mouth of the capsule as well as several similarities in the structure of the peristome such as the lack of an annulus, a reduced or lacking endostome, and, when dry, strongly reflexed exostome teeth. Moreover, the presence of spores stuck to visiting insects has been documented in this species (Wyatt et al. Citation2022). What role these features may play in invertebrate mediated spore dispersal is unexplored. While the role many of the features present in entomophilous Splachnaceae, S. pennata, and A. splachnoides may play in this dispersal requires further exploration, these similar traits could provide a road map for what to look for in other moss species.
While the previous three taxa appear to share adaptations for animal-mediated dispersal of spores, other mosses appear to have features for the dispersal of asexual propagules. Two mosses in particular, Aulacomnium androgynum (Rudolphi Citation2009) and Calymperes palisotii (Zona Citation2013), have asexual propagules dispersed by ants and millipedes respectively. In both these species, propagules are oblong to cylindrical in shape (Rudolphi Citation2009; Zona Citation2013), perhaps with similar function to the elongated spores seen in entomophilous Splachnaceae and Schistostega pennata. Moreover, the propagules in both A. androgynum and C. palisotii bear specialised cells which produce sticky mucilage, which allows the propagules to adhere to bodies of their invertebrate partners. Lastly, both species’ propagules are produced and held in clusters on structures which hold them clear of the moss colony, allowing for ease of contact with invertebrate partners (Rudolphi Citation2009; Zona Citation2013).
While there are comparatively few documented cases of adaptive dispersal of propagules in bryophytes, the instances we do see suggest a pattern of similar adaptations seen across a variety of bryophyte genera and life histories. Having sticky propagules is a shared dispersal strategy of the five taxa examined here. This strategy has likely arisen in different bryophyte lineages because it is an effective means to facilitate animal dispersal regardless of the specialisation, or lack thereof, in signalling adaptations. However, the adherence to animal partners is only one potential mechanism of animal-mediated dispersal. In angiosperm plants, a common vector of dispersal is endozoochory where propagules are borne internally by animals. As discussed previously, the sporophytes of a number of moss species appear to be mimicking fruits or edible parts of herbaceous plants (Glime Citation2017a). While the spores of some bryophytes have shown the capacity to withstand avian gut passage (Proctor Citation1961; deRegnier et al. Citation2023), the capacity of the spores or capsules of these particular species to survive gut passage is, as yet, unexplored.
Similarly, the process by which sperm cells adhere to or otherwise utilise animal transportation is also unexplored. While the natural movement of small invertebrates within reproductive bryophytes could result in the movement of some sperm, the possibility of adaptive characteristics facilitating a more specialised, directed relationship remains unexplored. An examination of the known Collembola mediated sperm dispersal could provide answers. Collembola are known to have a hydrophobic cuticle which could pose a difficulty for the adherence of water-borne sperm cells (Cronberg Citation2012). However, as noted above some mosses are known to produce lipids in male sexual structures (Paolillo Citation1979), ostensibly to aid freshly released sperm in overcoming surface tension (Muggoch and Walton Citation1942). Such lipids could play a role in allowing the adherence of those sperm cells to passing invertebrates sporting waterproof coats, but this possibility remains untested. Experiments testing for the ability of Collembola to transfer moss sperm, in the presence and absence of lipids, would be extremely interesting and useful.
Fitness benefits of animal-mediated propagule dispersal
Propagule dispersal in angiosperms plays an important role in the ecology of those plants (Wenny Citation2001). In many cases, particularly in early successional and refugial species, the habitat immediately surrounding a parent plant is unsuitable for the establishment and recruitment of new individuals into a population, thus selecting for dispersal (Wenny Citation2001). As bryophytes often occupy ecological peripheries, early stage successional regimes, or transient habitat types (Glime Citation2017b), dispersal of propagules to new suitable habitat and the establishment of new populations is of paramount importance, likely exerting strong selection on bryophytes to evolve dispersal mechanisms. While many species of bryophyte apparently rely on abiotic factors such as wind and water movements to move a great number of low-investment, easily-dispersed spores in a random fashion (Glime Citation2017a), bryo-zoophily offers a more efficient and effective means. Examining the traits seen in these bryophyte species may shed light on the reasons for the evolution of these relationships as well as provide a framework of characteristics which may indicate other, as yet unexplored bryophyte-animal relationships.
There are two key fitness benefits of bryo-zoophilous spore dispersal: (1) the ability to rapidly colonise habitats that are rare in physical and temporal space (i.e. decaying carcasses, cave habitats, etc) and (2) to increase genetic heterogeneity within new colonies. Bryophytes which occupy habitats which present unique challenges to abiotic spore dispersal benefit from animal-mediated dispersal. Entomophilous Splachnaceae grow on dung and carcasses (Koponen Citation1990; Marino et al. Citation2009). This sort of habitat is both quite ephemeral and relatively rare on the landscape. Insect mediated directed dispersal to new resources allows for the rapid colonisation of new habitats from nearby source colonies as they become available and indeed the colonisation of new habitat by entomophilous Splachnaceae is reliant on animal dispersal agents (Koponen Citation1990) Many of the unique traits associated with Splachnaceae mosses appear aimed at the attraction of these mostly insect disperser communities (Koponen Citation1990) and these insect dispersers appear to be one of the primary drivers of niche partitioning among Splachnaceae mosses (Koponen Citation1990; Marino et al. Citation2009).
Schistostega pennata grows in caves and large cavities (Ignatov and Ignatova Citation2001). This habitat presents its own challenges to dispersal as these areas often lack the abiotic conditions normally associated with bryophyte dispersal such as wind and rain (Ignatov et al. Citation2017). The co-option of resident Oribatid mites or other cave-inhabiting invertebrates, such as Dolichopodidae flies, is speculated to possibly allow the moss both to thoroughly establish in already occupied space and to colonise new, isolated habitats as they become available. Indeed, this moss has apparently experienced rapid expansion through Russia as previous logged forests age and produce new habitat in the cavities under the root masses of fallen trees (Ignatov and Ignatova Citation2001).
Similarly to the preceding two moss species, a species of liverwort, similarly habitat-restricted, has been shown to benefit from its interactions with animals. Riella americana is an aquatic liverwort found in isolated and often temporary water sources in the deserts of southwest North America (Howe and Underwood Citation1903). Following an observation of large numbers of waterfowl at a playa containing a large population of Riella americana, Proctor (Citation1961) conducted experiments which demonstrated the capability of the spores of this species to pass through the guts of ducks known to feed heavily on this species. While any manner of evolved adaptation on either the part of the liverwort or waterfowl remain unexplored in this system, the co-option of the movements of a water-seeking bird represents an efficient means of dispersal of an aquatic plant across a desert landscape.
Bryophytes engaged in animal-mediated spore dispersal may be able to able to disperse rapidly, establishing propagules in new habitat and gain a competitive advantage in colonising that habitat. While spores have been the focus of mid to long-distance bryophyte propagule distribution (Miles and Longton Citation1992; Kimmerer and Young Citation1995; Hedenås et al. Citation2003), a perhaps overlooked aspect of bryophyte dispersal is via vegetative propagules. While many bryophytes are able to reproduce via vegetative fragments (Glime Citation2017a), some species produce asexual brood bodies specifically adapted to disperse to new environments (Glime Citation2017a). Because brood bodies tend to be larger and heavier than spores it is generally thought that these play more of a role in direct colony expansion or, at most, short-range dispersal (Miles and Longton Citation1992; Kimmerer and Young Citation1995; Hedenås et al. Citation2003). However, brood bodies typically provide for much faster establishment than do spores (Kimmerer and Young Citation1995). Bryophytes which can overcome the challenges to longer range dispersal ability of asexual propagules would experience a competitive advantage to those bryophytes which are relying on spores for colonisation. This appears to be the case in Aulacomnium androgynum, which occupies habitat on rotting wood in temperate forests (Rudolphi Citation2009). Colonisation of this habitat requires dispersal of propagules over a space of unsuitable habitat. Instead of relying on spores to bridge the gap this moss has apparently co-opted the movements of a species of ant which occupies the same habitat and often lives in colonies spanning multiple resource patches in order to disperse its asexual propagules (Rudolphi Citation2009). Similarly, Calymperes palisotii is a corticolous species which spreads via asexual propagules dispersed on the bodies of millipedes which are often found in the same micro habitats on the tree trunk in which the moss grows (Zona Citation2013). Interestingly both of these species are known to very seldom produce sporophytes, and hence spores, and in some populations the production of sporophytes is completely unknown (Rudolphi Citation2009). This seems to indicate a seemingly total reliance on effective dispersal of an otherwise poorly dispersed sort of propagule.
Less well understood is the dispersal of bryophyte fragments by vertebrates and some invertebrates. Unlike propagules or gemmae, which are vegetative structures specifically adapted to facilitate asexual reproduction in bryophytes, fragments of gametophytes are not specifically adapted to disperse but are capable of growing and establishing new colonies nonetheless. A number of studies have documented the endozoochorous and ectozoochorous movement of viable fragments in birds (Chmielewski and Eppley Citation2019a; Lazaro et al. Citation2021; Lewis, Behling, et al. Citation2014; Wilkinson et al. Citation2017), flying foxes (Parsons et al. Citation2007), large and small terrestrial mammals (Staaland et al. Citation1979; Moen et al. Citation1993; Heinken et al. Citation2001; Pauliuk et al. Citation2011; Barbé et al. Citation2016), and molluscs such as snails and slugs (Boch et al. Citation2013, Citation2015). While such movement of viable material likely plays a role in the dispersal, perhaps over long distances (Lewis, Behling, et al. Citation2014), such movements likely represent a facultative relationship on the part of the bryophytes involved.
In contrast to the likely accidental ingestion or adherence of gametophyte fragments to animal dispersers is the intentional movement of bryophytes across the landscape facilitated by the nesting behaviour of many bird species. In the simplest cases, the movement of these bryophyte fragments for the purposes of nest building can facilitate the asexual colonisation of previously unoccupied sites. For example, in the case of skua in Antarctica, the building of nests using, among other materials, mosses, facilitates the movement of these mosses into new sites and allows regional colonisation events to occur (Ivanets et al. Citation2022). In a potentially much more complex relationship, a detailed study involving hummingbirds in southern South America, showed that certain hummingbird species utilise in an obligate manner a specific suite of moss species in the construction of their nests, that the birds’ selection of moss contains a higher density of reproductive structures than found in natural moss patches, and that after a period of a year, these nests maintained at least half their original biomass and reproductive structures. Being incorporated into a nest facilitates dispersal both by establishing a new site of asexual colonisation as well as providing an advantageous position for spore dispersal from reproductive structures. In a proposed mutualistic relationship, these ‘nest-mosses’ benefit by being advantageously dispersed across the forest landscape, while hummingbirds ensure a constant supply of an obligate nesting material (Osorio-Zuñiga et al. Citation2014). The relationship between birds and bryophyte nesting material remains an understudied aspect of the ecologies of both avian and bryophyte taxa.
Fitness benefits of animal-mediated sperm dispersal and fertilisation
Efficient and effective gamete dispersal is critical to success of sexual reproduction in plants (Howe and Smallwood Citation1982). Angiosperm investment in displays, lures, and rewards that facilitate animal-mediated pollination belies the fitness benefits of ensuring pollen reaches a receptive ovary (Mitchell et al. Citation2009). In many plants tight associations are formed with their animal pollinators (Johnson et al. Citation2000) and in many cases these associations go far back into evolutionary time (Kevan et al. Citation1975). We suggest that similar relationships, originating much deeper in the past, likely exist in more anciently diverged lineages of plants and animals.
Bryophyte sperm transfer is facilitated by and long thought obligate to the presence of a film of water in which the sperm cells swim from antheridia to archegonia (Glime Citation2017b). While some moss species were known to have adaptations, notably ‘splash cups’ present in mosses in the Polytrichaceae (Brodie Citation1951; Clayton-Greene et al. Citation1977; Reynolds Citation1980) among others, to enhance dispersal ability, the distances sperm cells appear able to disperse is limited to a maximum of around 10–12 centimetres in ideal conditions (Riemann Citation1972; Wyatt Citation1977).
More directed and efficient animal-mediated sperm delivery is reported for mosses. In at least two species of moss, Bryum argenteum and Ceratodon purpureus, sexual reproduction is documented to benefit from the presence of micro-arthropods, specifically species of Collembola and Oribatid mites (Cronberg et al. Citation2006; Rosenstiel et al. Citation2012). These invertebrates enable sporophyte production in the absence of a connecting water film between antheridia and archegonia, and, at least in C. purpureus increase sporophyte production even in the presence of a water film capable of supporting sperm cell movements (Rosenstiel et al. Citation2012). It is thought that sperm cells stick to the bodies of invertebrates as they move about the moss colony, enabling transfer from antheridia to archegonia across space that would not ordinarily be able to be crossed by the sperm cells alone While both Collembola and Oribatid mites have been shown to transfer sperm, the much more mobile Collembola appear to transfer the sperm over longer distances than do the slower-moving Oribatid mites (Cronberg et al. Citation2006; Rosenstiel et al. Citation2012). Moreover, documented attraction of invertebrates to active sexual structures shows a bias towards female structures over male structures, though both are more attractive than sterile shoots (Rosenstiel et al. Citation2012). A potential explanation for this phenomenon is that such a mechanism is adaptive to provide females a more diverse selection of potential mates. Collembola and other micro-arthropods have long been noted to be associated with bryophyte communities (Gerson Citation1969; Kevan et al. Citation1975; Davis Citation1981; Wehner et al. Citation2016) and this invertebrate mediated fertilisation may be more common than previously guessed.
There is growing evidence that bryophytes may have some mechanism to actively attract micro-invertebrates, in systems that may well be analogous to how flowers attract and reward their pollinators with nectar. This dispersal mechanism doesn’t appear to be incidental in nature. Sperm carrying invertebrates being drawn to sexual shoots allows for a more direct route between antheridia and archegonia, which eliminates the need for a continuous water film between these two structures. While all bryophytes require moisture to enable growth, this moisture does not always present in a way which enables sperm mobility. Invertebrate mediated fertilisation could facilitate sporophyte production in conditions which are not ideal for the mobility of sperm cells, reducing the time required to produce mature spores. The small number of species with documented invertebrate mediated fertilisation makes establishing any patterns or trends difficult, however testing the efficiency of fertilisation events is often as easy as counting sporophytes produced and tests exploring invertebrate attractions to certain substances can be done as simple choice tests.
The extent to which invertebrate mediated fertilisation plays a role in the sperm transfer of a particular bryophyte is likely to vary by species, life history, and habitat. It may not be a coincidence that the first two species for which this syndrome has been documented are both fast growing species of disturbed areas relying heavily on spores for reproduction (Rosenstiel et al. Citation2012). In bryophytes, such as those which exhibit a short lived ‘colonist life strategy’ (During Citation1979) which relies heavily on sporophyte production, it is imperative that fertilisation occurs. Further, both of these species are known to be dioicous and acrocarpous (Rosenstiel et al. Citation2012). These features could present challenges to fertilisation requiring an unbroken water film. These features present a framework for establishing which bryophytes would benefit most from invertebrate mediated fertilisation, however it is possible that while some species may benefit more than others, most sporophyte producing bryophytes could see a benefit as this would enable sexual reproduction in circumstances where it would otherwise be unlikely and reduce self-crossing in monecious species.
Summary and future directions
Bryo-zoophily has three primary hallmarks:
Production of lures. The presence of colourful displays or production of olfactory lures analogous to those produced by flowering plants would be a strong indicator that a bryophyte is engaged in a biotic interaction rather than being reliant on abiotic factors.
Presence of adaptations to facilitate dispersal by animals. In the cases observed, sticky mucilage is the primary adaptation to allow for ectozoochory. However, this does not preclude the possibility of other means such as endozoochory or spines or hooks seen in some angiosperm seed dispersal strategies.
Life history which would be advantaged by zoophily. Some bryophytes live in habitats which present challenges to abiotic dispersal; this could be an indication of a potential bryo-zoophilous relationship. In other cases, bryophytes are advantaged by a type of dispersal made difficult without such a relationship such as the long-distance dispersal of heavy propagules.
Adaptations observed range from the more specialised signalling seen in Splachnaceae mosses (Koponen Citation1990; Marino et al. Citation2009) to the more general associations between co-habiting invertebrates and bryophytes (Gerson Citation1969; Lawrence et al. Citation2013; Wehner et al. Citation2016), and the dispersal adaptations to take advantage of the animal movements throughout the habitat (Kimmerer and Young Citation1995; Rudolphi Citation2009; Zona Citation2013). While Splachnaceae signals are most apparent to human senses, and thus best studied, but this does not preclude the possibility of other quite specialised signalling relationships existing in a more cryptic fashion, beyond the limits of human sensory detection. The diversity of volatile organic compounds produced by sexual structures on at least two species of moss (Rosenstiel et al. Citation2012) hint at the possibility of a complex signalling system at this just-barely macroscopic level. Despite the possibility of some as yet discovered specialised bryo-zoophily, in all likelihood the majority of these interactions are likely to be less specialised, consisting of various adaptations to take advantage of passing animals or those already present in a particular ecosystem. However, these more generalised interactions are no less of a paradigm shift in the world of bryophyte ecology.
When observed adaptations are taken together in conjunction with life history traits consistent with benefit from animal-mediated dispersal, a framework starts to emerge to guide potential research into this largely unexplored area of ecology. While the documented syndromes of confirmed bryo-zoophily are few, evidence extrapolated from these cases points to many other species associations that warrant investigation. Moreover, as the specifics and mechanisms of this behaviour are clarified by further research, investigators will be able to fine tune their approach and potentially be able to detect increasingly subtle interactions between bryophytes and the animals with which they interact.
The consideration of the sensory ecology of those animals that would interact with bryophyte is a critical aspect of research into this field of study. An illustrative example is found in the Splachnaceae mosses where the sporophyte releases volatile compounds which mirror those found particular types of animal matter, either dung or carcasses, which is attractive to flies which rely on that material to feed and reproduce (Koponen Citation1990; Marino et al. Citation2009). In less well-studied partnerships, investigating a potential animal partner’s behaviour in regard to foraging, reproduction, habitat use, etc. and how their sensory systems are adapted to seek those resources could provide the first clues as to what to look for in the bryophytes with which it associates. However, the first step in establishing these relationships is determining which animals associate with which bryophytes and the behaviour of those animals when interacting with the bryophytes. Examples such as observations of Collembola massing in the tips of Tortula cernua by Bisang and Hedenäs (Citation2015) could be the first hint at a complex relationship between Collembola and moss as well as other bryophytes. While there are many observed associations, there has been less effort made to connect these associations with any sort of mutualistic relationships.
A systematic approach is likely the most efficient means to uncovering potential cases of bryo-zoophily and focusing on certain key aspects of the biology of bryophytes and potential dispersers will be key to this approach. To this end, we propose the following avenues of study as a means of expanding our understanding of bryo-zoophily.
Documentation and surveys of animal populations associated with bryophyte colonies, particularly over differing life stages of the bryophyte (i.e. Spore-producing, sexually fertile, etc.).
Documentation of the identity and purpose of potential signals such as volatile organic compounds or unusual colours seen in reproductive structures.
Investigation of reproductive structures, particularly those which bear similarities to known bryo-zoophilous species (i.e. erect capsules, sticky propagules, etc.).
Identifying potential rewards, or deceptive rewards, for animal partners (i.e. sucrose or lipids).
Testing the capacity of bryophyte spores, sporophytes, and vegetative propagules to survive gut passage.
Close examination of bryophytes with unusual life histories, particularly those species with rare, isolated, or ephemeral habitats.
Compiling case studies on the above factors to consider the incidence and extent of these relationships across various bryophyte families and life histories.
Conclusions
The discovery of widespread bryophyte-animal interactions would fundamentally alter our understanding and perception of bryophyte ecology. Long relegated to a passive role in the ecosystems they occupy, bryophytes could now be seen as not just being partners in ongoing relationships, but also actively manipulating these interactions and driving evolution of their own. The evolution of entomophily in Splachnaceae at several apparently independent points (Goffinet et al. Citation2004), shows that there is selection pressure and there are drivers which enable the development of these relationships. The possibility that these syndromes are in an active state of evolution would refute the idea that mosses and other bryophytes evolve ‘slowly’ (Schofield and Crum Citation1972; Stenøien Citation2008). However, the elucidation of these processes would not only change the way we view bryophytes but would also have implications further afield.
Expanding the scope of the potential research beyond implications for bryophyte ecology, these relationships between invertebrates and bryophytes could provide tantalising insights into the evolution of plant-animal interactions in general, pushing back the clock on this relationship long before the appearance of the first clear relationships in gymnosperms and angiosperms. Indeed, some of the most commonly associated invertebrates involved in these interactions are themselves quite old with Collembola-like arthropods being recovered from Devonian fossil deposits (Parry et al. Citation2016). Whether these relationships are indeed conserved from the ancient past or are products of more recent and ongoing evolution remains to be seen, but the discoveries in this largely unexplored field have the potential to rocket the previously overlooked bryophyte to the forefront of scientific consciousness.
Disclosure statement
No potential conflict of interest was reported by the author(s).
Additional information
Funding
References
- Barbé M, Chavel ÉE, Fenton NJ, Imbeau L, Mazerolle MJ, Drapeau P, Bergeron Y. 2016. Dispersal of bryophytes and ferns is facilitated by small mammals in the boreal forest. Écoscience. 23(3–4):67–76. doi:10.1080/11956860.2016.1235917.
- Bell CD, Soltis DE, Soltis PS. 2005. The age of the angiosperms: a molecular timescale without a clock. Evolution. 59(6):1245–1258. doi:10.1111/j.0014-3820.2005.tb01775.x.
- Bell CD, Soltis DE, Soltis PS. 2010. The age and diversification of the angiosperms re-revisited. American Journal of Botany. 97(8):1296–1303. doi:10.3732/ajb.0900346.
- Bequaert J. 1921. On the dispersal by flies of the spores of certain mosses of the family Splachnaceae. The Bryologist. 24(1):1–4. doi:10.2307/3237827.
- Bisang I, Hedenäs L. 2015. Mass-occurrence of springtails on Tortula cernua (Huebener) Lindb.: a field-observation of possible animal-mediated fertilization. Journal of Bryology. 37(4):339–341. doi:10.1179/1743282015Y.0000000012.
- Boch S, Berlinger M, Fischer M, Knop E, Nentwig W, Türke M, Prati D. 2013. Fern and bryophyte endozoochory by slugs. Oecologia. 172(3):817–822. doi:10.1007/s00442-012-2536-0.
- Boch S, Fischer M, Knop E, Allan E. 2015. Endozoochory by slugs can increase bryophyte establishment and species richness. Oikos. 124(3):331–336. doi:10.1111/oik.01536.
- Breil DA, Moyle SM. 1976. Bryophytes used in construction of bird nests. The Bryologist. 79(1):95–98. doi:10.2307/3241875.
- Brodie HJ. 1951. The splash-cup dispersal mechanism in plants. Canadian Journal of Botany. 29(3):224–234. doi:10.1139/b51-022.
- Campbell DR, Bischoff M, Lord JM, Robertson AW. 2010. Flower color influences insect visitation in alpine New Zealand. Ecology. 91(9):2638–2649. doi:10.1890/09-0941.1.
- Chen G, Ma X-K, Jürgens A, Lu J, Liu E-X, Sun W-B, Cai X-H. 2015. Mimicking livor mortis: a well-known but unsubstantiated color profile in sapromyiophily. Journal of Chemical Ecology. 41(9):808–815. doi:10.1007/s10886-015-0618-2.
- Chmielewski MW, Eppley SM. 2019a. Forest passerines as a novel dispersal vector of viable bryophyte propagules. Proceedings of the Royal Society B: Biological Sciences. 286(1897):20182253. doi:10.1098/rspb.2018.2253.
- Chmielewski MW, Eppley SM. 2019b. Species-specific interactions in avian–bryophyte dispersal networks. Royal Society Open Science. 9(1):211230. doi:10.1098/rsos.211230.
- Clayton-Greene KA, Green TGA, Staples B. 1977. Studies of Dawsonia superba. 1. Antherozoid dispersal. The Bryologist. 80(3):439–444. doi:10.2307/3242019.
- Cronberg N. 2012. Animal-mediated fertilization in bryophytes – parallel or precursor to insect pollination in angiosperms? Lindbergia. 35:76–85.
- Cronberg N, Natcheva R, Berggren H. 2008. Observations regarding the life cycle of silvermoss Bryum argenteum. In: Mohamed H, Baki BB, Nasrulhaq-Boyce A, Lee PKY, editors. Bryology in the new millennium. Kuala Lumpur: University of Malaya; p. 347–352.
- Cronberg N, Natcheva R, Hedlund K. 2006. Microarthropods mediate sperm transfer in mosses. Science. 313(5791):1255–1255. doi:10.1126/science.1128707.
- Davis RC. 1981. Structure and function of two Antarctic terrestrial moss communities. Ecological Monographs. 51(2):125–143. doi:10.2307/2937260.
- Delgadillo Moya C. 1994. Endemism in the neotropical moss flora. Biotropica. 26(1):12–16. doi:10.2307/2389105.
- Depetris-Chauvin A, Galagovsky D, Grosjean Y. 2015. Chemicals and chemoreceptors: Ecologically relevant signals driving behavior in Drosophila. Frontiers in Ecology and Evolution. 3:41. doi:10.3389/fevo.2015.00041.
- deRegnier RJ, Mussoi JG, Gaskett AC. 2023. Passage of spores of the dung moss Tayloria callophylla (Splachnaceae) through an avian digestive tract – a novel mode of dispersal? Bryophyte Diversity and Evolution. 46(1):Article 1. doi:10.11646/bde.46.1.18.
- Di Giusto B, Bessière J-M, Guéroult M, Lim LBL, Marshall DJ, Hossaert-McKey M, Gaume L. 2010. Flower-scent mimicry masks a deadly trap in the carnivorous plant Nepenthes rafflesiana: flower-scent mimicry in Nepenthes rafflesiana. Journal of Ecology. 98(4):845–856. doi:10.1111/j.1365-2745.2010.01665.x.
- During HJ. 1979. Life strategies of bryophytes: a preliminary review. Lindbergia. 5(1):2–18.
- Gallenmüller F, Langer M, Poppinga S, Kassemeyer H-H, Speck T. 2018. Spore liberation in mosses revisited. AoB PLANTS. 10(1):plx075. doi:10.1093/aobpla/plx075.
- Gaskett AC. 2011. Orchid pollination by sexual deception: pollinator perspectives. Biological Reviews. 86(1):33–75. doi:10.1111/j.1469-185X.2010.00134.x.
- Gerson U. 1969. Moss-arthropod associations. The Bryologist. 72(4):495–500. doi:10.2307/3241388.
- Gibson RJH, Miller-Brown D. 1927. Fertilization of Bryophyta. Polytrichum commune. (Preliminary note). Annals of Botany. 41(1):190–191. doi:10.1093/oxfordjournals.aob.a090064.
- Glime JM. 2017a. Adaptive strategies: spore dispersal vectors. In: Glime JM, editor. Bryophyte ecology. Vol. 1. Houghton: Michigan Technological University and the International Association of Bryologists; p. 1–45. https://digitalcommons.mtu.edu/cgi/viewcontent.cgi?article=1021&context=bryo-ecol-subchapters.
- Glime JM. 2017b. Life cycles and morphology. In: Glime JM, editor. Bryophyte ecology. Vol. 1. Houghton: Michigan Technological University and the International Association of Bryologists; p. 1–140. https://digitalcommons.mtu.edu/bryophyte-ecology1/1/.
- Glime JM, Bisang I. 2017. Sexuality: sexual strategies. In: Glime JM, editor. Bryophyte ecology. Vol. 1. Houghton: Michigan Technological University and the International Association of Bryologists; p. 1–38. https://digitalcommons.mtu.edu/cgi/viewcontent.cgi?article=1009&context=bryo-ecol-subchapters.
- Goffinet B, Shaw AJ, Cox CJ. 2004. Phylogenetic inferences in the dung-moss family Splachnaceae from analyses of cpDNA sequence data and implications for the evolution of entomophily. American Journal of Botany. 91(5):748–759. doi:10.3732/ajb.91.5.748.
- Haddock SHD, Dunn CW. 2015. Fluorescent proteins function as a prey attractant: experimental evidence from the hydromedusa Olindias formosus and other marine organisms. Biology Open. 4(9):1094–1104. doi:10.1242/bio.012138.
- Hedenås H, Bolyukh VO, Jonsson BG. 2003. Spatial distribution of epiphytes on Populus tremula in relation to dispersal mode. Journal of Vegetation Science. 14(2):233–242. doi:10.1111/j.1654-1103.2003.tb02148.x.
- Heinken T, Lees R, Raudnitschka D, Runge S. 2001. Epizoochorous dispersal of bryophyte stem fragments by roe deer (Capreolus capreolus) and wild boar (Sus scrofa). Journal of Bryology. 23(4):293–300. doi:10.1179/jbr.2001.23.4.293.
- Howe HF, Smallwood J. 1982. Ecology of seed dispersal. Annual Review of Ecology and Systematics. 13(1):201–228. doi:10.1146/annurev.es.13.110182.001221.
- Howe MA, Underwood LM. 1903. The Genus Riella, with descriptions of new species from North America and the Canary Islands. Bulletin of the Torrey Botanical Club. 30(4):214–224. doi:10.2307/2478779.
- Ignatov MS, Ignatova EA. 2001. On the zoochory of Schistostega pennata (Schistostegaceae, Musci). Arctoa. 10(1):83–96. doi:10.15298/arctoa.10.09.
- Ignatov MS, Sidorchuk EA, Ignatova EA. 2017. A Schistostega pennata (Bryophyta) spore devourer in flagranti – a troglophyle mite Kunstidamaeus lengersdorfi (Acari, Oribatida: Damaeidae). Bryophyte Diversity and Evolution. 39(1):59. doi:10.11646/bde.39.1.10.
- Ivanets V, Yevchun H, Miryuta N, Veselsky M, Salganskiy O, Konishchuk V, Kozeretska I, Dykyi E, Parnikoza I. 2022. Skua and plant dispersal: lessons from the Argentine Islands – Kyiv Peninsula region in the maritime Antarctic. Nordic Journal of Botany. 2022(6):e03326. doi:10.1111/njb.03326.
- Jofre J, Goffinet B, Marino P, Raguso RA, Nihei SS, Massardo F, Rozzi R. 2011. First evidence of insect attraction by a Southern Hemisphere Splachnaceae: the case of Tayloria dubyi Broth. in the reserve biosphere Cape Horn, Chile. Nova Hedwigia. 92(3–4):317–326. doi:10.1127/0029-5035/2011/0092-0317.
- Johnson SD, Steiner KE, Johnson SD, Steiner KE. 2000. Generalization versus specialization in plant pollination systems. Trends in Ecology & Evolution. 15(4):140–143. doi:10.1016/S0169-5347(99)01811-X.
- Kaiser K, Outlaw WH, Ziegler H. 1985. Sucrose content of receptive archegonia of the moss Bryum capillare Hedw. Naturwissenschaften. 72(7):378–379. doi:10.1007/BF00410604.
- Kelber A. 2001. Receptor based models for spontaneous colour choices in flies and butterflies. Entomologia Experimentalis et Applicata. 99(2):231–244. doi:10.1046/j.1570-7458.2001.00822.x.
- Kevan PG, Chaloner WG, Savile DBO. 1975. Interrelationships or early terrestrial arthropods and plants. Palaeontology. 18(2):391–417.
- Kimmerer RW, Young CC. 1995. The role of slugs in dispersal of the asexual propagules of Dicranum flagellare. The Bryologist. 98(1):149. doi:10.2307/3243652.
- Kimmerer RW, Young CC. 1996. Effect of gap size and regeneration niche on species coexistence in bryophyte communities. Bulletin of the Torrey Botanical Club. 123(1):16. doi:10.2307/2996302.
- Koponen A. 1990. Entomophily in the Splachnaceae. Botanical Journal of the Linnean Society. 104(1–3):115–127. doi:10.1111/j.1095-8339.1990.tb02214.x.
- Lawrence JF, Slipinski A, Jäger O, Pütz A. 2013. The Australian Byrrhinae (Coleoptera: Byrrhidae) with descriptions of new genera and species. Zootaxa. 3745(3):301. doi:10.11646/zootaxa.3745.3.1.
- Lazaro X, Mackenzie R, Jimènez J. 2021. Evidence of endozoochory in upland geese Chloephaga picta and whitebellied seedsnipes Attagis malouinus in sub-Antarctic Chile. Ecology and Evolution. 14(11):9191–9197. doi:10.22541/au.161469488.80502225/v1.
- Lewis LR, Behling E, Gousse H, Qian E, Elphick CS, Lamarre J-F, Bêty J, Liebezeit J, Rozzi R, Goffinet B. 2014. First evidence of bryophyte diaspores in the plumage of transequatorial migrant birds. PeerJ. 2:e424. doi:10.7717/peerj.424.
- Lewis LR, Rozzi R, Goffinet B. 2014. Direct long-distance dispersal shapes a new world amphitropical disjunction in the dispersal-limited dung moss Tetraplodon (Bryopsida: Splachnaceae). Journal of Biogeography. 41(12):2385–2395. doi:10.1111/jbi.12385.
- Lönnell N, Hylander K, Jonsson BG, Sundberg S. 2012. The fate of the missing spores – patterns of realized dispersal beyond the closest vicinity of a sporulating moss. PLoS One. 7(7):e41987. doi:10.1371/journal.pone.0041987.
- Lunau K. 2006. Stamens and mimic stamens as components of floral colour patterns. Botanische Jahrbücher Für Systematik, Pflanzengeschichte Und Pflanzengeographie. 127(1):13–41. doi:10.1127/0006-8152/2006/0127-0013.
- Lüth M. 2010. Double tracked dispersal strategy in Splachnaceae Doppelte Ausbreitungsstrategie bei Splachnaceae. Bryophyte Diversity and Evolution. 31(1):5. doi:10.11646/bde.31.1.4.
- Marino P. 1991a. Competition between mosses (Splachnaceae) in patchy habitats. The Journal of Ecology. 79(4):1031. doi:10.2307/2261096.
- Marino P. 1991b. Dispersal and coexistence of mosses (Splachnaceae) in patchy habitats. The Journal of Ecology. 79(4):1047. doi:10.2307/2261097.
- Marino P, Raguso R, Goffinet B. 2009. The ecology and evolution of fly dispersed dung mosses (Family Splachnaceae): manipulating insect behaviour through odour and visual cues. Symbiosis. 47(2):61–76. doi:10.1007/BF03182289.
- Miles CJ, Longton RE. 1992. Deposition of moss spores in relation to distance from parent gametophytes. Journal of Bryology. 17(2):355–368. doi:10.1179/jbr.1992.17.2.355.
- Mitchell RJ, Flanagan RJ, Brown BJ, Waser NM, Karron JD. 2009. New frontiers in competition for pollination. Annals of Botany. 103(9):1403–1413. doi:10.1093/aob/mcp062.
- Moen J, Lundberg PA, Oksanen L. 1993. Lemming grazing on snowbed vegetation during a population peak, Northern Norway. Arctic and Alpine Research. 25(2):130–135. doi:10.2307/1551549.
- Morris JL, Puttick MN, Clark JW, Edwards D, Kenrick P, Pressel S, Wellman CH, Yang Z, Schneider H, Donoghue PCJ. 2018. The timescale of early land plant evolution. Proceedings of the National Academy of Sciences. 115(10):E2274–E2283. doi:10.1073/pnas.1719588115.
- Muggoch H, Walton J. 1942. On the dehiscence of the antheridium and the part played by surface tension in the dispersal of spermatocytes in Bryophyta. Proceedings of the Royal Society of London. Series B – Biological Sciences. 130(861):448–461. doi:10.1098/rspb.1942.0012.
- Osorio-Zuñiga F, Fontúrbel FE, Rydin H. 2014. Evidence of mutualistic synzoochory between cryptogams and hummingbirds. Oikos. 123(5):553–558. doi:10.1111/j.1600-0706.2013.01027.x.
- Paolillo DJ. 1979. On the lipids of the sperm masses of three mosses. The Bryologist. 82(1):93. doi:10.2307/3241977.
- Parnikoza I, Dykyy I, Ivanets V, Kozeretska I, Kunakh V, Rozhok A, Ochyra R, Convey P. 2012. Use of Deschampsia antarctica for nest building by the kelp gull in the Argentine Islands area (maritime Antarctica) and its possible role in plant dispersal. Polar Biology. 35(11):1753–1758. doi:10.1007/s00300-012-1212-5.
- Parry SF, Noble SR, Crowley Q, Wellman C. 2016. A high-precision U–Pb age constraint on the Rhynie Chert Konservat-Lagerstätte: time scale and other implications. doi:10.6084/M9.FIGSHARE.3452846.V1.
- Parsons JG, Cairns A, Johnson CN, Robson SKA, Shilton LA, Westcott DA. 2007. Bryophyte dispersal by flying foxes: a novel discovery. Oecologia. 152(1):112–114. doi:10.1007/s00442-006-0639-1.
- Pasiche-Lisboa CJ, Jesús IS-D. 2018. Moss protonemata are dispersed by water, wind, and snails. American Journal of Botany. 105(4):788–795. doi:10.1002/ajb2.1065.
- Patiño J, Medina R, Vanderpoorten A, González-Mancebo JM, Werner O, Devos N, Mateo RG, Lara F, Ros RM. 2013. Origin and fate of the single-island endemic moss Orthotrichum handiense. Journal of Biogeography. 40(5):857–868. doi:10.1111/jbi.12051.
- Pauliuk F, Müller J, Heinken T. 2011. Bryophyte dispersal by sheep on dry grassland. Nova Hedwigia. 92(3–4):327–341. doi:10.1127/0029-5035/2011/0092-0327.
- Proctor VW. 1961. Dispersal of Riella spores by waterfowl. The Bryologist. 64(1):58–61. doi:10.2307/3240925.
- Pyysalo H, Koponen A, Koponen T. 1978. Studies on entomophily in Splachnaceae (Musci). I. Volatile compounds in the sporophyte. Annales Botanici Fennici. 15(4):293–296.
- Pyysalo H, Koponen A, Koponen T. 1983. Studies on entomophily in Splachnaceae (Musci). II. Volatile compounds in the hypophysis. Annales Botanici Fennici. 20(4):335–338.
- Reynolds DN. 1980. Gamete dispersal in Mnium ciliare. The Bryologist. 83(1):73–77. doi:10.2307/3242397.
- Riemann B. 1972. On the sex-distribution and the occurence of sporophytes in Rhytidiadelphus triquetrus (Hedw.) Warnst. In Scandinavia. Lindbergia. 1(3/4):219–224.
- Rosenstiel TN, Shortlidge EE, Melnychenko AN, Pankow JF, Eppley SM. 2012. Sex-specific volatile compounds influence microarthropod-mediated fertilization of moss. Nature. 489(7416):431–433. doi:10.1038/nature11330.
- Rudolphi J. 2009. Ant-mediated dispersal of asexual moss propagules. The Bryologist. 112(1):73–79. doi:10.1639/0007-2745-112.1.73.
- Schofield WB, Crum HA. 1972. Disjunctions in bryophytes. Annals of the Missouri Botanical Garden. 59(2):174. doi:10.2307/2394752.
- Shi J, Luo Y-B, Bernhardt P, Ran J-C, Liu Z-J, Zhou Q. 2009. Pollination by deceit in Paphiopedilum barbigerum (Orchidaceae): a staminode exploits the innate colour preferences of hoverflies (Syrphidae). Plant Biology. 11(1):17–28. doi:10.1111/j.1438-8677.2008.00120.x.
- Smith SA, Beaulieu JM, Donoghue MJ. 2010. An uncorrelated relaxed-clock analysis suggests an earlier origin for flowering plants. Proceedings of the National Academy of Sciences. 107(13):5897–5902. doi:10.1073/pnas.1001225107.
- Staaland H, Jacobsen E, White RG. 1979. Comparison of the digestive tract in Svalbard and Norwegian reindeer. Arctic and Alpine Research. 11(4):457–466. doi:10.2307/1550563.
- Stenøien HK. 2008. Slow molecular evolution in 18S rDNA, rbcL and nad5 genes of mosses compared with higher plants. Journal of Evolutionary Biology. 21(2):566–571. doi:10.1111/j.1420-9101.2007.01479.x.
- Sundberg S. 2005. Larger capsules enhance short-range spore dispersal in Sphagnum, but what happens further away? Oikos. 108(1):115–124. doi:10.1111/j.0030-1299.2005.12916.x.
- Sundberg S, Hansson J, Rydin H. 2006. Colonization of Sphagnum on land uplift islands in the Baltic Sea: time, area, distance and life history. Journal of Biogeography. 33(8):1479–1491. doi:10.1111/j.1365-2699.2006.01520.x.
- van der Niet T, Hansen DM, Johnson SD. 2011. Carrion mimicry in a South African orchid: flowers attract a narrow subset of the fly assemblage on animal carcasses. Annals of Botany. 107(6):981–992. doi:10.1093/aob/mcr048.
- Vanderpoorten A, Patiño J, Désamoré A, Laenen B, Górski P, Papp B, Holá E, Korpelainen H, Hardy O. 2019. To what extent are bryophytes efficient dispersers? Journal of Ecology. 107(5):2149–2154. doi:10.1111/1365-2745.13161.
- Wehner K, Norton RA, Blüthgen N, Heethoff M. 2016. Specialization of oribatid mites to forest microhabitats – the enigmatic role of litter. Ecosphere. 7(3):e01336. doi:10.1002/ecs2.1336.
- Wellman CH, Osterloff PL, Mohiuddin U. 2003. Fragments of the earliest land plants. Nature. 425(6955):282–285. doi:10.1038/nature01884.
- Wenny DG. 2001. Advantages of seed dispersal: a re-evaluation of directed dispersal. Evolutionary Ecology Research. 3(1):37–50.
- Wilkinson DM, Lovas-Kiss A, Callaghan DA, Green AJ. 2017. Endozoochory of large bryophyte fragments by waterbirds. Cryptogamie, Bryologie. 38(2):223–228. doi:10.7872/cryb/v38.iss2.2017.223.
- Willis RE, White CR, Merritt DJ. 2011. Using light as a lure is an efficient predatory strategy in Arachnocampa flava, an Australian glowworm. Journal of Comparative Physiology B. 181(4):477–486. doi:10.1007/s00360-010-0533-3.
- Willson MF, Whelan CJ. 1990. The evolution of fruit color in fleshy-fruited plants. The American Naturalist. 136(6):790–809. doi:10.1086/285132.
- Wyatt R. 1977. Spatial pattern and gamete dispersal distances in Atrichum angustatum, a dioicous moss. The Bryologist. 80(2):284–291. doi:10.2307/3242472.
- Wyatt R, Stoneburner A, Wyatt GE. 2022. Evidence for entomophily in “Knothole Moss” (Anacamptodon splachnoides). The Bryologist. 125(4):558–570. doi:10.1639/0007-2745-125.4.558.
- Zona S. 2013. Millipedes transport gemmae of Calymperes palisotii (Bryophyta: Calymperaceae). Nova Hedwigia. 97(3–4):477–483. doi:10.1127/0029-5035/2013/0132.