Abstract
An experimental approach was used to calculate the spawning times and hatching dates for the oviparous chondrichthyan, Callorhinchus milii. The duration of the embryonic stages, from the formation of the area opaca to hatching, was determined at water temperatures of 10°, 15° and 20°C for egg cases collected from the Marlborough Sounds and Canterbury Bight, using novel observation techniques. Development times for temperatures 9–20°C were calculated using these data, enabling the prediction of spawning times, development times and hatching dates at four spawning sites in New Zealand. In the Marlborough Sounds, peak spawning was in April, with a predicted development time of 32 weeks and a predicted hatching time in November. In Canterbury Bight, peak spawning was in December, with a 25-week predicted development time, and a predicted peak hatching in late May. At Dunedin, peak spawning was in December, predicted development was 34 weeks and hatching would occur in late July. In Te Waewae Bay, peak spawning during January yielded a predicted 34-week development period and peak hatching in late August.
Introduction
Chondrichthyans exhibit all the major vertebrate reproductive modes, from retained and extended oviparity to placental and aplacental viviparity (Compagno Citation1990; Dulvy & Reynolds 1997; Carrier et al. Citation2004). Extended oviparity involves the depositing, externally, of a fertilised egg enclosed in a tough egg case, which can vary enormously in size and shape. For oviparous species, the general trend is for year-round ova production but seasonal deposition of fertilised eggs (Hamlett & Koob Citation1999).
Elephantfish (Callorhinchus milii) (Bory de Saint-Vincent 1823) (Family Callorhinchidae), of the Subclass Holocephali (chimaeroids), are the lesser known cousins to the sharks, skates and rays (Subclass Elasmobranchii), which together make up the Class Chondrichthyes. Mature C. milii aggregate in shallow inshore waters during summer and autumn, where they spawn (Gorman Citation1963; Sullivan Citation1981; Freer & Griffiths Citation1993; McClatchie & Lester Citation1994; Francis Citation1997; Didier et al. Citation1998; Didier Citation2004) and deposit large leathery egg cases up to 30 cm long (Gorman Citation1963). Their major coastal spawning sites in New Zealand include Pegasus Bay, Canterbury Bight and Te Waewae Bay in the South Island. There are no major spawning sites in the North Island, but juveniles and adults have been found at southern North Island sites, such as Wellington Harbour, Porirua Harbour and Pauatahanui Inlet (Jones & Hadfield Citation1985). A commercial trawl and set net fishery exists for the species, with an annual catch of 1200 tonnes (Ministry of Fisheries 2008).
The inshore aggregations of adults were the basis for the initial assumptions that the C. milii spawning season was between November and February on the east coast of the South Island (Gorman Citation1963; Francis Citation1997). In the Marlborough Sounds, newly laid egg cases with blastodiscs have been found in June (Francis Citation1997), indicating an extended spawning period at that site. In the Canterbury Bight, the length of embryonic development has been calculated as 26 weeks (average) (Francis Citation1997) and 21–34 weeks (Gorman Citation1963) based on the time between peak laying and peak hatching. Callorhinchus milii egg cases kept at the Portobello Marine Laboratory in Dunedin, where the water is considerably cooler than in Canterbury Bight, took at least 43 weeks to hatch (Gorman Citation1963). Mature female C. milii produce two egg cases simultaneously, with each egg case containing a single large fertilised yolky egg. Egg cases are deposited at spawning sites with sand or mud substrata and in water less than 30 m deep (Gorman Citation1963).
Temperature is a key abiotic factor in oviparous embryonic development (Tullis & Peterson Citation2000; Tullis & Baillie Citation2005; Hoff Citation2008), with the length of embryonic development varying directly with temperature (Parsons Citation1993; Hoff Citation2008). Exposure to high temperatures is likely to result in 100% mortality (Laugen et al. Citation2003). Most early research into chondrichthyan embryonic development was descriptive, with few studies characterising embryo development into definable stages (Balfour 1876, 1878; Schauinsland 1903; Dean 1906; Clark 1927; Smith 1942). The first complete embryonic developmental series for any oviparous chondrichthyan was developed for Scyliorhinus canicula from observations of numerous living eggs rather than preserved specimens (Ballard et al. Citation1993). The first complete description of batoid embryonic development was for Raja eglanteria (Luer et al. Citation2007). For the holocephali, Didier et al. (Citation1998) customised the Ballard et al. (Citation1993) embryonic development stages for chimaeroids using observations of the external features of C. milii. Here embryonic growth of C. milii at three constant water temperatures is described using stages based on the work of Ballard et al. (Citation1993) and Didier et al. (Citation1998), and the findings are used to infer site-specific spawning, development and hatching periods.
Materials and methods
To view oviparous embryos of Scyliorhinus canicula, Ballard et al. (Citation1993) shaved a small window in the pigmented outer layer of the egg case and used gravity to place the developing embryo in front of that window. We developed a novel flip-top-lid method that is an advance on that technique. The entire pigmented dorsal layer of the C. milii egg case (the central bulbous spindle) was removed, allowing an uninterrupted view of the developing embryo and yolk sac through the egg case's undisturbed, thin, transparent inner layer. A shallow 1-mm (scalpel) incision was made that ran from the anterior end of the egg case, along one side of the central bulbous portion and onto the tapered posterior spindle. Another cut, similar to the first, was then made around the other side of the central bulbous spindle, and joined the previous cut on the ridge of the posterior spindle. Where the two cuts met on the posterior spindle, the outer egg case layer was pried apart from the inner layer with forceps until the separation point reached the anterior slits (). Leaving the anterior end of the central spindle intact allowed the un-cut section to act as a hinge, so that the outer layer could be lowered onto the inner layer when no observations were being made. It was essential that the scalpel did not puncture the inner transparent layer, which must remain intact to retain the internal structural integrity of the egg case.
Figure 1 Callorhinchus milii egg case with outer pigmented egg case layer peeled back to expose the transparent inner layer still protecting the developing embryo and egg yolk.
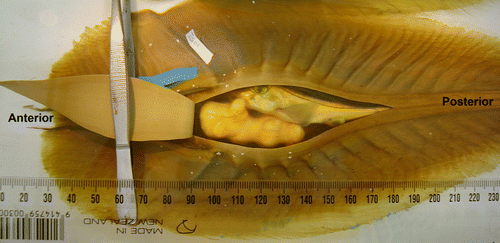
Cut egg cases were individually labelled, the embryonic stage was identified and the cases were placed in one of three randomly selected temperature-controlled rooms (see below). The transparent inner layer of the egg case is soft and pliable, and to prevent movement of the growing embryo from breaking this layer during later embryonic stages and hatching prematurely, rubber bands were placed around the central spindle, after the outer flanges had been cut.
Egg case collection and preparation
We collected C. milii egg cases from two sites within the Canterbury Bight (north and south of Timaru), and two sites in the Marlborough Sounds (Queen Charlotte and Pelorus Sounds) (). The egg cases collected from Canterbury Bight were either obtained directly from a commercial bottom-trawl net or were extracted from pregnant females caught in the same net (). As a female nears the end of egg case formation, a section of the egg case protrudes from her cloaca. When females were found in this condition, a longitudinal cut was made along the ventral surface to gain access to the uterus, from which the egg cases were removed. Only removed egg cases that were at least three quarters completed were used. These were artificially closed using a quick setting Araldite epoxy adhesive, which held the posterior tips of the unfinished egg case together. Care was taken not to seal the posterio-ventral slits that open during prehatching.
Table 1 Details of Callorhinchus milii egg case collection and rearing conditions.
Embryo rearing conditions
All egg cases were housed at the University of Canterbury Edward Percival Field Station in Kaikoura, in tubs in three temperature-controlled rooms (10°, 15° and 20°C) (). Egg cases were placed ventral side down, the natural position in which they rest on the seafloor. Although the design of the egg case promotes passive movement of water circulation through it (Leonard et al. Citation1999; Flammang et al. Citation2007) and myogenic movements of the embryo aid this, air pumps were also installed to actively circulate water in the tubs. The temperature-controlled rooms did not have recirculating water systems, but the water was filtered through a 1-µm filter and passed by an ultraviolet light source, and was changed weekly or when necessary (such as after a mortality event).
Observations of embryos
The developing embryos were monitored daily, with slight variations for some stages. To identify how long an individual embryo took to reach each successive developmental stage, the egg case was removed from the temperature-controlled room and placed in an observation bath for examination with a microscope (Wild Heerbrugg M7, illuminated with a Leica CLS 150× fibre-optic light source). Descriptions of changing features provided by Ballard et al. (Citation1993) were used for the early stages and those of Didier et al. (Citation1998) for mid- to late stages (Appendix 1). The time taken by each embryo to develop from one stage to the next was determined by identifying the changing embryonic features with those listed in Appendix 1, and when a new embryonic stage had been reached, it was from that time until the next set of developing features were observed that was recorded as a stage length and included as part of .
Table 2 Callorhinchus milii embryonic development times.
Water temperatures representing those of the Marlborough Sounds were obtained from a salmon (Oncorhynchus tshawytscha) farm approximately 5 km north-east of our sampling site at Kumutoto Bay in Queen Charlotte Sound, where daily readings were taken from a depth of 5 m. The Dunedin temperature data are weekly readings, depth also 5 m, taken at Murderer's Beach as part of a long-term study by the Portobello Marine Laboratory. Mean monthly water temperatures were calculated from these data. No such readings were available for Canterbury Bight and Te Waewae Bay, where there was much more mixing of coastal waters, so National Institute of Water and Atmospheric Research data for monthly mean sea surface temperatures, calculated from the National Oceanic and Atmospheric Administration (NOAA) and Geostationary Meteorological Satellite (GMS) data, were used.
Estimates of spawning and development
In oviparous chondrichthyans, fertilisation may happen days before egg case production is complete or before egg cases are laid (Ballard et al. Citation1993). Some early embryonic stages occur between fertilisation and egg case completion, and further stages may occur between egg case completion and deposition. Because of this uncertainty, we refer to the deposition of egg cases on the seafloor as spawning, and used ‘time since egg case completion’ for the cumulative development times in . Clearly, the egg cases collected from the seafloor had an uncertain spawning date, while those removed from adult females had not yet been spawned, but the latter could be used to determine a ‘time since egg case completion’, which was used as a proxy for spawning date. Using egg cases that were 95–99% complete enabled the calculation of ‘time since egg case completion’, but because of the time taken to return from the vessels, the earliest embryonic stage lengths could not be determined. Four methods were used to determine C. milii spawning peaks, one for each site. Literature reports were used for the Canterbury Bight (Gorman Citation1963; Sullivan Citation1981; McClatchie & Lester Citation1994; Francis Citation1997); anecdotal evidence for Dunedin; fishing industry logbook data for Te Waewae Bay (SeaFIC Citation2009); and back calculation based on Appendix 2 for the Marlborough Sounds. Once the developmental stage of an embryo had been determined, the number of days since spawning was determined using information in Appendix 2. That number was then subtracted from the collection date to yield spawning period.
Development times other than those observed were interpolated and extrapolated from the data in using Equation (Equation1), which predicts development times for all stages at temperatures 9°–20°C. Specifically, a method of alternating conditional expectation (Breiman & Friedman Citation1985), as implemented in the Splus function ‘ace’ (Statistical Sciences Citation1993), yielded non-parametric transformations of the independent variables (development stage and temperature) that would make their relationship with the dependent variable (the logarithm of development time) approximately linear. (We chose to work with a log transformation of development time because it seemed likely that prediction errors would be multiplicative rather than additive.)
In mathematical terms, this approach leads to a prediction function of the form
Results
Observations of embryos
Each embryonic stage of C. milii developed faster in warmer water (20°C) than in cooler water (10°C). For example, embryonic stage lengths at 20°C were on average 38±7% shorter than the same stages at 15°C, which were on average 121±13% shorter than the same stage at 10°C (Table 2). The predicted embryonic stage lengths (Appendix 2) for stages 5–39 (hatching) were the same or shorter in warmer waters at all temperatures matching C. milii spawning sites (9°–20°C). Embryonic stage lengths were recorded for embryos from stages 6 to 36 at 10°C, from stages 5 to 39 (hatching) at 15°C and from stages 15 to 35 at 20°C (). The missing data for final stage lengths in were the result of mortalities, the rate of which was 100% at 10°C, 55% at 15°C and 100% at 20°C. Consequently, the lengths of the final stage were predicted using Equation (Equation1). In terms of total embryonic development, of the 112 embryos kept at 15°C, 50 successfully hatched, with embryonic development taking 178±1 days (). Predicted hatching times of embryos reared at 10°C was 404 days and was 135 days for embryos reared at 20°C (). The time taken for each embryonic stage was higher for all temperatures from stage 27 onwards, which was the result of morphological changes rather than changes in physiological activity.
Estimates of spawning and development
Callorhinchus milii in the Marlborough Sounds were predicted to have a peak development time of 32 weeks, those in the Canterbury Bight 25 weeks, and for those in Dunedin and in Te Waewae Bay 34 weeks. Prediction errors associated with Equation (Equation1) were typically less than 2% (the median absolute percentage error was 1.7%) and always less than 15% and these errors, though greater for the youngest stages, otherwise showed no strong trend with increasing stage (Appendix 4).
The Marlborough Sounds () showed peak spawning in early April, with parturition events taking place at roughly 2-week (13–16-day) intervals from March to May, and few egg cases being laid in between. Peak hatching in the Marlborough Sounds was predicted to occur during November, based on the April spawning peak. There is still considerable spawning outside the peak spawning period, from March to May () and June (Didier et al. Citation1998), and development was predicted to take 30–33 weeks (31 weeks from June spawning events), and hatching was predicted to occur from September to January ().
Figure 3 Predicted spawning dates for Callorhinchus milii in the Marlborough Sounds (Pelorus Sound and Queen Charlotte Sounds) for 2004. The peak spawning events appear to follow a roughly fortnightly cycle.
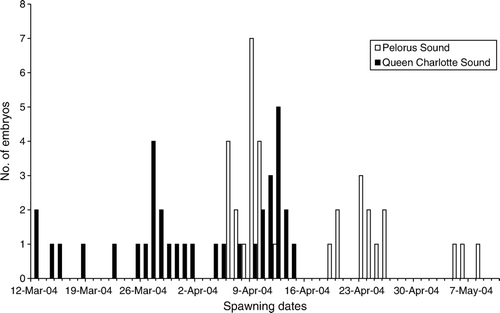
Figure 4 Predicted Callorhinchus milii embryonic development at differing spawning times for all four spawning sites. Marlborough Sounds embryos spawned in March, April and May hatch in late September (30 weeks), mid-November (32 weeks), and mid-December (33 weeks) respectively. Canterbury Bight embryos spawned in November, December and January hatch in late April (26 weeks), late May (25 weeks), and late July (29 weeks) respectively. Dunedin embryos spawned in November, December and March hatch in mid-June (32 weeks), late July (34 weeks), and mid-December (41 weeks) respectively. Te Waewae Bay embryos spawned in December, January and March hatch in late June (29 weeks), late August (34 weeks), and late November (38 weeks) respectively.
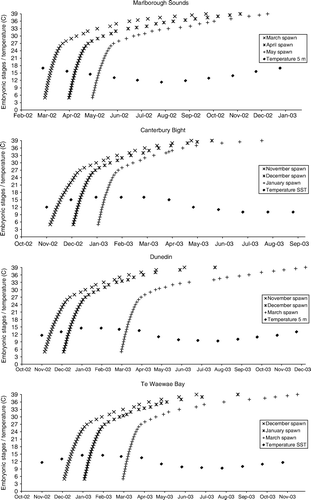
At our Canterbury Bight sites, spawning peaks occurred in mid-December as well as late January, so that the resulting predicted hatching period was from April to July, with a peak in May. Other research has found longer spawning periods, from October to May, which lead to predicted development periods of 27–40 weeks, resulting in predicted hatching from April to January ().
At Dunedin, the spawning period was from November to March, with a peak during December. The resulting development period was predicted to range from 32 to 41 weeks, with predicted hatching from June to December and peak hatching in July ().
At Te Waewae Bay, spawning occurred from December to March, with a peak during December. The resulting development period was predicted to range from 29 to 38 weeks, with predicted hatching from June to November and peak hatching in August ().
Discussion
Callorhinchus milii are ectothermic, so warmer water increases embryonic physiological activity, and therefore increases the rate of development and reduces hatching times (Parsons Citation1993; Hoff Citation2008). The water surrounding the South Island of New Zealand has a broad temperature range, from the warmer northern waters of the Marlborough Sounds to the cooler southern waters of Te Waewae Bay (). Consequently, the species would be expected to undergo variable embryonic development in different spawning sites.
Figure 5 Monthly mean water temperatures at Callorhinchus milii spawning sites around New Zealand. Standard error bars are on Marlborough Sounds and Dunedin temperatures; there are no error bars for Canterbury Bight or Te Waewae Bay satellite sea surface temperature data.

Embryonic observations
The novel egg case flip-top-lid observation method was highly successful. It allowed an uninterrupted view of the embryo and yolk sac as they developed, enabling observations, descriptions and the timing of C. milii embryonic stages from the formation of the area opaca to hatching. Previously this had either not been possible, had used only small windows cut into the outer egg case, or had involved observations of preserved embryos.
The temperatures used in this study (10°, 15° and 20°C) spanned the range to which embryonic C. milii would be exposed in the South Island. Ballard et al. (Citation1993) considered his 16°C as an optimal temperature and it fell within our range. The system worked very well except when a single mortality fouled the water, resulting in the death of all embryos in the tub. As mentioned in the Results, there are no data for the later embryonic stages at 10° and 20°C owing to 100% mortality at those temperatures. Although the water temperature in each of the tubs was constant (i.e. 10°, 15° or 20°C), temperatures at all C. milii spawning sites fluctuate throughout the year, so it was necessary to estimate C. milii development times at temperatures between 9° and 20°C (Appendix 2). It was not possible to estimate the likely error in the predicted development times for temperatures other those observed in this study. The problem was that the choice of a quadratic function to interpolate development time was arbitrary; use of a different curve would have produced different predicted development times. That said, the errors would have been no smaller than those for the observed temperatures (), and would have tended to be larger for temperatures more distant from the observed temperatures.
Estimates of spawning and development
Spawning periods for C. milii in the Marlborough Sounds were predicted to range from mid-March () to June (Francis Citation1997), with a peak in April (). There are no published estimates of development times or hatching dates for C. milii from the Marlborough Sounds, but a predicted 32-week development period would yield a predicted hatch date in mid-November. There is still considerable spawning outside the peak spawning period, between March and June, and if development periods were 30–33 weeks long, hatching would be predicted to occur between September and January. Early stage embryos found in the Marlborough Sounds in June (Didier et al. Citation1998) would, according to our study, take 31 weeks to reach hatching, in January.
Spawning periods for C. milii in the Canterbury Bight were predicted to range from October to May, and a spawning peak has been recorded between November and January (Gorman Citation1963; Sullivan Citation1981; McClatchie & Lester Citation1994; Francis Citation1997). The presence of juvenile C. milii in Canterbury Bight commercial fishing catches has been used to predict peak hatching between May and July (Francis Citation1997), and this was confirmed here, with peak hatching predicted to be between April and July. Further estimates by Francis (Citation1997) of Canterbury Bight C. milii embryonic development times suggested the period of embryonic development averages around 26 weeks. From the predictions made in this study, peak embryonic development from December and January spawning events was likely to take between 25 and 29 weeks, again matching the prediction made by Francis (Citation1997). Our predictions for embryonic development also fit with that proposed by Gorman (Citation1963) of between 21 and 34 weeks.
At Dunedin, C. milii spawning occurs between November and March (Graham Citation1938; McClatchie & Lester Citation1994), with an assumed peak in December. One December in the 1930s, several early stage C. milii egg cases were incubated at the Portobello Marine Laboratory, where they hatched a little over 43 weeks later (Gorman Citation1963). This was much longer than the 34 weeks that we predicted for C. milii spawning at Dunedin in December. However, if the egg cases mentioned by Gorman (Citation1963) had been laid in March rather than December, then embryonic development would be expected to take 41 weeks (in the cooler autumn and winter water temperatures) (), much closer to the Gorman (Citation1963) example. Furthermore, if the seawater in the tanks at the Dunedin Laboratory were cooler than the surrounding seawater, then we would expect embryonic development to take longer in the laboratory tanks. Egg cases laid during the earliest part of the Dunedin spawning range, in November, would take just 32 weeks to develop, which is less time than the predicted development time of 34 weeks for egg cases laid during the assumed December spawning peak. If a fast embryonic development were advantageous for C. milii, then it would be likely that November, not December, was the peak spawning month.
In Te Waewae Bay, C. milii spawning occurs between December and March, with a peak in January (SeaFIC Citation2009), there were no previous estimates of development times or hatching dates for C. milii from Te Waewae Bay. From a January spawning peak, development in Te Waewae Bay would take 34 weeks with hatching predicted for late August. Embryos resulting from December spawning are predicted to hatch 29 weeks later in June, 5 weeks less than the development times for embryos spawned in January. If fast embryonic development was advantageous for C. milii, then we might expect December, not January, to be the peak spawning period.
Research suggests that embryos would develop faster and hatch earlier at warmer northern sites and slower at more southern spawning sites (Parsons Citation1993; Hoff Citation2008), and our results seem to partially confirm this. Peak hatching of C. milii in the Canterbury Bight was predicted to take 25 weeks—9 weeks faster than the 34 weeks to peak hatching at the more southerly latitudes of Te Waewae Bay and Dunedin. However, predictions for Marlborough Sounds were not consistent with published findings: embryonic development was predicted to take 32 weeks—7 weeks longer than in the Canterbury Bight, despite the fact that mean water temperatures at the Marlborough Sounds were warmer than those at Canterbury Bight throughout the year (). This situation is probably the result of spawning in the Marlborough Sounds peaking in April, causing the C. milii embryos to develop during the colder periods of autumn, winter and spring. In the Canterbury Bight, peak spawning was in December, allowing the embryos to develop faster in the warmer Canterbury Bight summer waters, which are higher than the winter water temperatures of the Marlborough Sounds ().
Peak spawning period and peak hatching period were highly variable between sites, with spawning ranging from November through to April and hatching dates peaking from April to November; there seemed to be no uniform South Island spawning or hatching period. Our data suggests that egg laying of C. milii follows a fortnightly cycle, similar to McClatchie and Lester (Citation1994). In Pelorus Sound, parturition peaks occur every 15 or 16 days during the main spawning period, and every 13 days in Queen Charlotte Sound (). Water temperature may also be a spawning cue in some sites. With peak spawning in Marlborough Sounds occurring in April, after the warmer summer water temperatures had passed, C. milii embryos may then be exposed to temperatures that are more suitable for embryonic development. Further research into C. milii spawning cues is needed to clarify this issue.
It is now possible to estimate the spawning times and hatching dates for C. milii at any spawning site around coastal New Zealand. Our methodology can also be applied to other oviparous members of the Class Chondrichthyes. New Zealand has an obligation to conserve and manage all shark species found in its waters. This research fits into that obligation by furthering our knowledge of this Ministry of Fisheries managed species, enabling more informed management decisions.
tnzm_a_535496_sup_17788836.zip
Download Zip (94 KB)Acknowledgements
WL would like to thank Bill Davison from the University of Canterbury, Dominique Dagit from Millersville University and Clinton Duffy from the Department of Conservation for support. Thanks are also extended to Paul Steere of The New Zealand King Salmon Co Ltd. and Bev Dickson of the Portobello Marine Laboratory, for allowing the use of sea temperature recordings. Tony Adamson of Sanford South Island, Pete Dawson of South East Finfish, Eddie and Dick from the F.V. Ida Marion, Simon Bernard Shaw from the F.V. Trojan, Andy Hetherington from the F.V. Tengawai, Erika Mackay from NIWA, and the anonymous reviewers are also acknowledged. Special thanks go to Jack van Berkel at the University of Canterbury Edward Percival Field Station, Kaikoura.
Additional information
Notes on contributors
WS Lyon
Present address: National Institute of Water & Atmospheric Research Ltd, PO Box 14-901, Kilbrnie, Wellington, NewZealandReferences
- Balfour , FM . 1876 . On the development of elasmobranch fishes: the general features of the elasmobranch embryo at successive stages . Journal of Anatomy and Physiology : 555–576 , 10 : 24 – 26 .
- Balfour , FM . 1878 . A monograph on the development of elasmobranch fishes , London, Macmillan and Co .
- Ballard , WM , Mellinger , J and Lechenault , H . 1993 . A series of normal stages for development of Scyliorhinus canicula, the lesser spotted dogfish (Chondrichthyes: Scyliorhinidae) . The Journal of Experimental Zoology , 267 : 318 – 336 .
- Bory de Saint-Vincent , M . 1823 . Dictionnaire classique d'historie naturelle , Volume 3. Paris, Rey et Gravier .
- Breiman , L and Friedman , JH . 1985 . Estimating optimal transformations for multiple regression and correlation. (with discussion) . Journal of the American Statistical Association , 80 : 580 – 619 .
- Carrier , JC , Pratt , HL Jr and Castro , JI . 2004 . “ Reproductive biology of elasmobranchs ” . In Biology of sharks and their relatives , Edited by: Carrier , JC , Musick , JA and Heithaus , MR . 269 – 286 . Boca Raton, FL, CRC Marine Biology Series .
- Clark , RS . 1927 . Rays and skates. No. 2—Description of embryos . Journal of the Marine Biological Association of the U.K. , 14 : 661 – 683 .
- Compagno , LJV . 1990 . Alternative life-history styles of cartilaginous fishes in time and space . Environmental Biology of Fishes , 28 : 33 – 75 .
- Dean , B . 1906 . Chimaeroid fishes and their development , Carnegie Institution of Washington .
- Didier , DA , Leclair , EE and Vanbuskirk , DR . 1998 . Embryonic staging and external features of development of the Chimaeroid fish, Callorhinchus milii (Holocephali, Callorhinchidae) . Journal of Morphology , 236 : 25 – 47 .
- Didier , DA . 2004 . “ Phylogeny and classification of extant holocephali ” . In Biology of sharks and their relatives , Edited by: Carrier , JC , Musick , JA and Heithaus , MR . 115 – 135 . Boca Raton, FL, CRC Marine Biology Series .
- Dulvy , NK and Reynolds , JD . 1997 . Evolutionary transitions among egg-laying, live-bearing and maternal inputs in sharks and rays . Proceedings of the Royal Society of London Series B Biological Sciences , 264 : 1309 – 1315 .
- Flammang , BE , Ebert , DA and Cailliet , GM . 2007 . Egg cases of the genus Apristurus (Chondrichthyes: Scyliorhinidae): phylogenetic and ecological implications . Zoology , 110 : 308 – 317 .
- Francis , MP . 1997 . Spatial and temporal variation in the growth rate of elephantfish (Callorhinchus milii) . New Zealand Journal of Marine and Freshwater Research , 31 : 9 – 23 .
- Freer , DWL and Griffiths , CL . 1993 . The fishery for, and general biology of, the St Joseph Callorhinchus capensis (Dumeril) off the south-western Cape, South Africa . South African Journal of Marine Science , 13 : 63 – 74 .
- Gorman , TBS . 1963 . Biological and economic aspects of the elephantfish, Callorhynchus milii Bory, in Pegasus Bay and the Canterbury Bight , 8 Fisheries Technical Report No .
- Graham , DH . 1938 . Fishes of Otago harbour and the adjacent seas with additions to previous records . Transactions and Proceedings of the Royal Society of New Zealand , 68 : 399 – 419 .
- Hamlett , W and Koob , TJ . 1999 . “ Female reproductive system ” . In Sharks, skates, and rays: the biology of elasmobranch fishes , Edited by: Hamlett , WC . 398 – 443 . Baltimore & London, The Johns Hopkins University Press .
- Hoff , GR . 2008 . A nursery site of the Alaska skate (Bathyraja parmifera) in the eastern Bearing Sea . Fishery Bulletin , 106 : 233 – 244 .
- Jones , JB and Hadfield , JD . 1985 . Fishes from Porirua and Pauatahanui Inlets: occurrence in gill nets . New Zealand Journal of Marine and Freshwater Research , 19 : 477 – 484 .
- Laugen , AT , Laurila , A and Merilä , J . 2003 . Latitudinal and temperature-dependent variation in embryonic development and growth in Rana temporaria . Oecologia , 135 : 548 – 554 .
- Leonard , JBK , Summers , AP and Koob , TJ . 1999 . Metabolic rate of embryonic little skate, Raja erinacea (Chondrichthyes: Batoidea): the cost of active pumping . Journal of Experimental Zoology , 283 : 13 – 18 .
- Luer , CA , Walsh , CJ , Bodine , AB and Wyffels , JT . 2007 . Normal embryonic development in the clearnose skate, Raja eglanteria, with experimental observations on artificial insemination . Environmental Biology of Fishes , 80 : 239 – 255 .
- McClatchie , S and Lester , P . 1994 . Stock assessment of the elephantfish (Callorhinchus milii) , 94/6 New Zealand Fisheries Assessment Research Document .
- Ministry of Fisheries 2008 . Report from the Fisheries Assessment Plenary, May 2008: stock assessments and yield estimates . Wellington : Ministry of Fisheries .
- Parsons , GR . 1993 . Geographic variation in reproduction between two populations of the bonnethead shark, Sphyrna tiburo . Environmental Biology of Fishes , 38 : 25 – 35 .
- Schauinsland , H . 1903 . Beiträge zur Entwicklungsgeschichte und Anatomie der Wirbeltiere , 16 I. Sphendon, Callorhynchus, Chamaeleo. Zoologica .
- SeaFIC 2009 . Report to the Adaptive Management Programme Fishery Assessment Working Group: review of the ELE 5 Adaptive Management Programme . Unpublished manuscript available from the NZ Seafood Industry Council, Wellington .
- Smith , BG . 1942 . “ The heterodontid sharks: their natural history, and the external development of Heterodontus japonicus based on notes and drawings by Bashford Dean ” . In The Bashford Dean Memorial Volume—Archaic Fishes , Edited by: Gudger , EW . 651 – 769 . Volume VIII. New York, American Museum of Natural History .
- Statistical Sciences 1993 . S-PLUS guide to statistical and mathematical analysis, version 3.2. Seattle , WA, StatSci, a division of MathSoft, Inc ..
- Sullivan , KJ . 1981 . Trends in the Canterbury Bight trawl fishery from 1963 to 1976 , 24 Fisheries Research Division Occasional Publication .
- Tullis , A and Baillie , M . 2005 . The metabolic and biochemical responses of tropical whitespotted bamboo shark Chiloscyllium plagiosum to alterations in environmental temperature . Journal of Fish Biology , 67 : 950 – 968 .
- Tullis , A and Peterson , G . 2000 . Growth and metabolism in the embryonic white-spotted bamboo shark, Chiloscyllium plagiosum: comparison with embryonic birds and reptiles . Physiological and Biochemical Zoology , 73 : 271 – 282 .
Supplementary material available online
Appendices 1–4