Abstract
Intermittent streams are predicted to harbour macroinvertebrate taxa with particular life history traits that allow them to complete development in a limited time and survive periods without water. Three intermittent headwater streams in Hawke's Bay, New Zealand, had more taxa with very small body size, plurivoltine life cycles, low dissemination potential, long-lived adults and asexual reproduction than three nearby perennial streams. We traced the population size structure and adult flight periods of six aquatic insect taxa, and the population size structure of an aquatic snail, in three intermittent streams to provide basic information on larval growth rates and infer whether they use particular life history stages to survive summer drought. The insect taxa appeared to over-summer primarily as eggs, whereas the snail Potamopyrgus antipodarum over-summered at various stages of maturity. Final instar larvae and adults of most insect taxa appeared well ahead of stream drying, suggesting they can survive early-onset drought. However, late-onset drought would allow more larvae to complete development.
Introduction
Macroinvertebrates that inhabit intermittent streams can persist by either recolonising each year from perennial waters, or surviving the dry period in situ. Those that survive in situ employ behavioural, physiological or life history strategies to survive stream drying. Life history strategies require organisms to complete development of their aquatic stages within the flowing-water period and to synchronise the most desiccation-resistant stage of their life cycle with the dry period (Williams Citation2006).
Williams (Citation2006) predicted that intermittent waters would be characterised by taxa with particular traits that enable them to use one of the above strategies. These traits tend to be r-selected, including small body size, high fecundity, short life cycle and high dissemination potential. Temperature-linked growth rates, possession of diapausing or otherwise-protected eggs, long-lived adults and terrestrialisation of immatures also have been shown to be characteristic life history traits among intermittent stream macroinvertebrates (Hart Citation1985; Williams Citation2006). The first aim of our study was to determine whether, in Hawke's Bay, New Zealand, the intermittent stream fauna shows a high proportion of life history traits that enable them to survive periodic drying, compared with the perennial stream fauna.
Macroinvertebrate life-history information is required for basic biological applications such as quantifying trophic relationships (Resh & Rosenberg Citation2010), estimating secondary production (e.g. Huryn Citation1998) and conducting species trait analyses (e.g. Doledec et al. Citation2006). Although life history information is essential in conducting modern ecological research (Resh & Rosenberg Citation2010), such information for New Zealand aquatic macroinvertebrates is patchy. Some taxa are well characterised (e.g. Winterbourn Citation1974; Winterbourn Citation2010; Towns Citation1981; Citation1983; Forsyth Citation1986; Scrimgeour Citation1991; Linklater & Winterbourn Citation1993; Huryn Citation1996; Winterbourn et al. Citation2008), but for many others, data on larval growth rates and longevity are lacking. Many New Zealand taxa show asynchronous development, extended flight periods and extended egg hatching (Winterbourn et al. Citation1981; Scarsbrook Citation2000). As a result, at any time of year most aquatic taxa are present as larvae at all stages of maturity, which can make it difficult to discern their growth rates and longevity from field data (e.g. Winterbourn Citation1974; Towns Citation1981; Scrimgeour Citation1991). Intermittent streams are expected to be useful habitats for studying the life history patterns of aquatic invertebrates. Since many mayflies, caddisflies and other insect taxa either survive the dry season in situ as eggs (e.g. Dieterich & Anderson Citation1990; Williams Citation1996; Wissinger et al. Citation2003) or recolonise from nearby perennial waterways by laying eggs in rewetted channels (Williams Citation2006), we may expect that in intermittent streams the start of a generation will be clearly discernible, allowing larval growth rates and longevity to be determined. The second aim of our study was to describe the growth rates and longevity of a few common intermittent stream taxa by examining their size distributions and, for the insects, flight periods, over several months.
Life history data also can be used to infer whether aquatic invertebrate taxa in intermittent streams use particular life history strategies to survive the dry season. In addition to desiccation-resistant eggs, desiccation-resistant larvae and pupae are known among aquatic insects (Harper & Hynes Citation1970; Imhof & Harrison Citation1981; Sommerhauser et al. Citation1997; Scarsbrook Citation2000; Winterbourn Citation2004). Insects may also survive the dry period as terrestrial adults if adults are sufficiently long-lived or are able to diapause (Bouvet Citation1977; Sommerhauser et al. Citation1997; Williams Citation2006). Our third aim was to infer, from larval size distributions and adult flight periods, whether the selected invertebrate taxa use particular life history stages to survive summer drought.
The length of dry period in intermittent streams often is highly sensitive to inter-year changes in temperature and rainfall. Such variability may exceed the abilities of macroinvertebrates to adapt, and failure of populations during particularly dry years is not uncommon (McElravy et al. Citation1989; Erman & Erman Citation1995; Fritz & Dodds Citation2005). Furthermore, global climate change is expected to bring drier conditions and more frequent occurrence of drought to eastern parts of New Zealand (Mullan et al. Citation2005). Therefore, the fourth aim of our study was to investigate whether the life histories of New Zealand intermittent stream macroinvertebrates allow them to survive dry years and take advantage of wet years.
Sites
This study was conducted from March 2005 to May 2007. Study sites were located in the foothills of the Wakarara Range at the edge of the Ruataniwha Plains in central Hawke's Bay, east coast of the North Island, New Zealand (39.8°S, 176.4°E altitude 300–400m a.s.l.; ). Rainfall reaches a maximum in July (114mm/month in the vicinity of the sampling sites) and a minimum in January (69mm/month), while evapotranspiration varies from 18mm/month in July to 113mm/month in January (30-year averages, Hawke's Bay Regional Council Citation2003). Maximum daily air temperatures on the Ruataniwha Plains range from 14°C in July to 24°C in January.
The three intermittent study sites (named INT1–3) were located on small first-order tributaries that originate at the edge of the plain (Fig.1) and dry to their headwaters most summers for 1–4months between December and April. In 2005, stream flow began with heavy rains in mid-March, and ceased in the first week of January 2006, though at INT2 and INT3, surface flow may have stopped up to five times for several days each time during this period (see Storey & Quinn Citation2008 for details). After flow cessation in January, surface water remained in isolated pools for 4–8weeks. In 2006, flow resumed with heavy rains between 21 and 27 March. At the sample locations, these streams were 1.5–14cm deep and 0.5–1.1m wide during average winter baseflow conditions. These three intermittent streams flow into perennial streams, respectively 3km, 300m and 500–800m downstream of the study sites (in the latter case it was not clear how far upstream the perennial flow extended).
Three perennial sites (PER1-3) were located as near as possible to the INT sites, also in rolling hills at the edge of the plains and underlain by alluvial gravels. At the sample locations the PER streams were 7–8cm deep and 2.3–3m wide during winter baseflow conditions. All of the study streams had a similar coarse substrate type dominated by pebbles and cobbles.
The main vegetation cover in the study catchments was pasture for sheep and beef farming, but some pockets of native forest remained, mostly in river gullies. West of the study sites, there was a large pine plantation that was being cropped and replanted in stages. All of the study streams were surrounded by intact native forest patches at least 30m wide on each bank and extending at least 300m upstream of the study site. Riparian vegetation cover directly over the stream channel was about 80% at the INT sites and 60–80% at the PER sites.
Methods
Benthic macroinvertebrates were sampled from the INT (intermittent headwater) sites every 2weeks from the end of March 2005 (2weeks after flowing water appeared) until May, and continued once per month thereafter until mid-January 2006 (when only disconnected pools remained). Benthic samples were taken from the PER (perennial) sites once in mid-May.
Benthic macroinvertebrates were collected using a Surber sampler with a 0.1-m2 base and a 250-µm mesh net. At each site, four samples were taken at riffles, over a range of substrate sizes, water depths and velocities. Effort was made to sample a comparable range of substrate sizes, water depths and velocities at each site. In summer (January), macroinvertebrates were sampled from pools by disturbing 0.1m2 of the pool bed sediments and sweeping the area with a 250-µm mesh hand net. Macroinvertebrate samples were preserved on-site with 70% ethanol.
In the lab, benthic macroinvertebrates were counted and identified under 50× magnification using the keys of Winterbourn et al. (Citation2000) for insects, Towns & Peters (Citation1996) for leptophlebiid mayflies, Smith & Ward (Citation2007) for hydrobiosid caddisflies and Winterbourn (Citation1973) for molluscs. Life history analysis focused on seven macroinvertebrate taxa: mayflies Deleatidium and Zephlebia (Leptophlebiidae), the stonefly Acroperla trivacuata (Tillyard) (Gripopterygidae), caddisflies Polyplectropus (Polycentropodidae), Psilochorema and Hydrobiosis (Hydrobiosidae) and the gastropod Potamopyrgus antipodarum (Gray) (Hydrobiidae). First-instar larvae of Deleatidium and Zephlebia could not be distinguished from one another, nor could first-instar larvae of Psilochorema and Hydrobiosis, therefore small larvae were assigned to genera in the same abundance ratios as larger, identifiable individuals. Except for A. trivacuata, insect larvae were routinely identified only to genus level. Adult and targeted larval identifications indicated that at the INT sites these genera included three species of Deleatidium (D. vernale, D. fumosum and D. lillii), four species of Zephlebia (Z. borealis, Z. dentata, Z. versicolor and Z. spectabilis), two species of Polyplectropus (P. aurifusca and P. altera), two species of Hydrobiosis (H. umbripennis and H. parumbripennis) and three species of Psilochorema (P. mimicum, P. macroharpax and P. donaldsoni). Sampling at PER and other nearby sites (R. Storey unpublished data) added Deleatidium autumnale, Deleatidium angustum and Deleatidium magnum, Zephlebia inconspicua, Hydrobiosis clavigera, Hydrobiosis soror, Hydrobiosis budgei and Hydrobiosis copis, and Psilochorema leptoharpax.
The size of macroinvertebrates was recorded as head width (distance across the eyes) for the insect taxa, and height of the shell for P. antipodarum. Measurements were made to the nearest 0.02mm, using either an ocular micrometer or a digitising tablet and software, at 50× magnification.
Adult mayflies, stoneflies and caddisflies were sampled at all INT sites using Malaise traps. The traps were 2m wide by 1m high, set across the wetted channel so they could trap insects flying upstream or downstream along the channel. In 2005–06, samples were collected monthly from mid-October (10weeks before flow ceased at INT sites) to mid-April (3weeks after flow resumed at INT sites). In 2007, samples were collected bi-weekly from mid-January (3weeks after flow ceased at all sites) to May (when streams were still dry at all sites). Adults were identified at 50× magnification using the key of Towns & Peters (Citation1996) for leptophlebiid mayflies, Smith & Ward (Citation2007), Ward (Citation1993) and Neboiss (Citation1986) for caddisflies and Riek (Citation1970) and McLellan (1998) for stoneflies.
Species trait composition
The invertebrate communities at intermittent and perennial sites in May 2005 were characterised in terms of eight life history traits. Affinities for these traits among the taxa in this study were derived from the New Zealand macroinvertebrate trait database (https://secure.niwa.co.nz/fbis/index.do), which collates knowledge from published scientific studies. The traits included maximum potential larval body size (five categories from <5 to >40mm), maximum number of descendants (four categories from <100 to >3000), maximum number of reproductive cycles per year (semivoltine, univoltine or plurivoltine), life duration of adults (five categories from 1 to >365days), reproductive technique (asexual, male–female or hermaphroditic), oviposition site (water surface, submerged, terrestrial or endophytic), dissemination potential (<10, 10–1000, >1000 m) and which life stages are aquatic (adult and larva, adult or larva, larva and pupa). To characterise the sites according to these traits, we followed the method of Doledec et al. (Citation2006). This involved giving each taxon (see for list of taxa used) an affinity score for each trait category, using the trait database, then multiplying the proportion of each category per trait by the fourth-root transformed abundance of each taxon to produce a trait-by-site array that contained the relative abundance of each trait category in each site. We then tested the differences in trait category scores (arcsine transformed) between intermittent and perennial sites using t-tests (not assuming equal variances) in SPSS™ v11.
Table 1 Taxonomic composition of benthic macroinvertebrates at the six study sites in May 2005.
Results
Life history trait differences between INT and PER sites
In May 2005, intermittent sites had a distinctly different taxonomic composition from the perennial sites () and this translated into significant differences between the two habitat types in almost all trait categories (). Intermittent sites had proportionally more taxa than perennial sites with the smallest (≤5 mm) and third-smallest (10–20 mm) body sizes, low numbers of descendants, plurivoltinism, long-lived adults, asexual reproduction, submerged or endophytic oviposition, low dissemination potential, and with both adult and larval aquatic stages. Perennial sites had proportionally more taxa than intermittent sites with medium-small (5–10 mm) and medium-large (20–40 mm) bodies, high numbers of descendants, univoltinism and semivoltinism, adults living <1 or 1–10 days, male–female reproduction, water surface and terrestrial egg-laying, medium dissemination potential, and with either adult or larval aquatic stages.
Table 2 T-test of differences between intermittent and perennial sites with respect to each of the species traits for samples collected in May 2005.
Size structure of macroinvertebrates in INT vs PER sites
Deleatidium
At the start of the flow period (March 2005), two middle-instar larvae were found at the INT sites (A) but all other Deleatidium appeared as first-instar larvae in April, a month after flow began. The size of the largest larvae in the samples increased steadily until October, when the first final-instar (black wingpad) Deleatidium larvae were seen. The modal size class also grew steadily over the same time period, suggesting that most eggs hatched early in the flow period. However, small larvae were present in low numbers throughout the year, suggesting some staggered egg hatching or different hatching times for different Deleatidium species. In October, November and January, the size distribution was bimodal, possibly indicating hatching of a second generation or another Deleatidium species, though the growth trajectory of the younger generation over this period is uncertain and it is not clear whether these larvae were able to mature. Deleatidium larvae in a range of sizes were present in the January pools. In May all Deleatidium in the INT sites were restricted to first or second instar, whereas in the PER sites all size classes were found (A).
Figure 2 Population size structure of: A, Deleatidium, B, Zephlebia, C, Acroperla trivacuata, D, Polyplectropus, E, Hydrobiosis, F, Psilochorema, G, Potamopyrgus antipodarum at intermittent headwater sites (INT1–3) from March 2005 to January 2006. Bar width is proportional to number of individuals. Where bars are truncated, the total number is printed on the bar. Numbers above graphs indicate total numbers of individuals collected in the corresponding month.
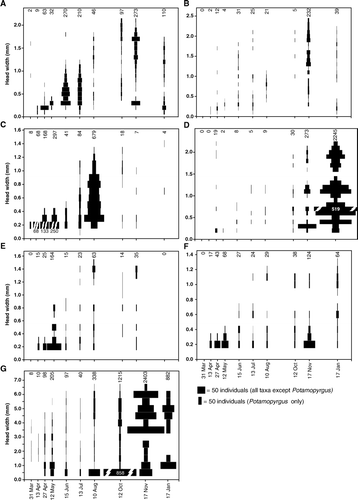
Figure 3 Population size structure of: A, Deleatidium, B, Zephlebia, C, Hydrobiosis, D, Psilochorema, E, Potamopyrgus antipodarum in May 2005 at intermittent headwater (INT) and perennial (PER) sites. Bar width is proportional to number of individuals. Where bars are truncated, the total number is printed on the bar. Numbers above graphs indicate total numbers of individuals in the corresponding month.
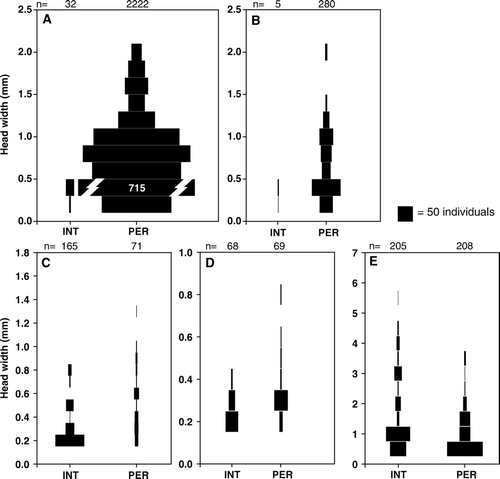
Adult Deleatidium were present at the INT sites from the start of Malaise trapping in October-November (). Adult numbers declined steadily thereafter, but were present until the last sampling date in March. In 2007, when INT streams dried by early January, only five (unidentified) leptophlebiid mayflies were caught at the INT sites during the 22 January to 30 April sampling period (); these were caught in late February–early March and late April.
Figure 4 Numbers of adult insects caught in Malaise traps at all intermittent sites (INT1–3) between 12 October 2005 and 18 April 2006. Bar height is proportional to numbers of adults caught. Numbers to the right of the bars indicate total numbers of each taxon.
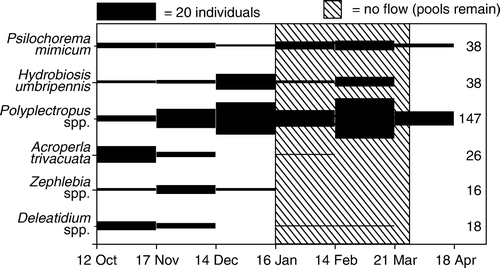
Figure 5 Numbers of adult insects caught in Malaise traps at all intermittent sites (INT1–3) between 22 January and 29 April 2007. Water flow ceased about 7 January, and surface pools disappeared between 22 January and 7 February. Bar height is proportional to numbers of adults caught. Numbers to the right of the bars indicate total numbers of each taxon. Leptophlebiid mayflies could not be identified further because of damage.
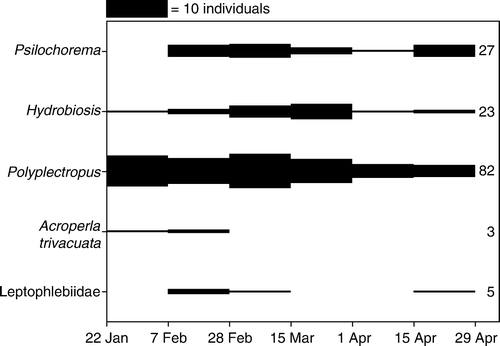
Zephlebia
Some middle-instar Zephlebia were found at the INT sites in late April (B); however, most Zephlebia at the start of the flow period were first-instar larvae. Mature (black wingpad) larvae were first seen in November. Between April and October, a wide range of sizes was found at the INT sites, but low numbers make it difficult to discern the presence of size cohorts. Summer pools contained a wide range of size classes. In May, all Zephlebia in the INT sites were restricted to first or second instar, whereas in the PER sites a much wider range of instars was found (B).
Adult Zephlebia at the INT sites were present from October, the start of the trapping period (), and reached peak numbers in November–December. None was found after mid-January.
Acroperla trivacuata
Acroperla trivacuata appeared at the INT sites from the start of the flowing-water period as first-instar larvae (no large larvae were found at beginning of flow period; C), and small larvae were found throughout the sampling period, suggesting continuous egg hatching. The size of the largest larvae in the benthic samples increased rapidly until August, after which no larger individuals were seen. However, these larvae did not appear to be fully mature. During sampling trips, larvae were seen walking on dry stones by the stream channel, and it is likely that A. trivacuata completed their development out of the water. After a peak in August, numbers of A. trivacuata declined to very low numbers in October and November, providing further evidence that larval development is completed on land. Only two larvae were found in summer pools. In May, A. trivacuata larvae at the INT sites were restricted to small size classes, but the size range cannot be compared between INT and PER, as no A. trivacuata were found in the PER sites.
Adult A. trivacuata at the INT sites were caught from the beginning of the trapping period in mid-October (). Numbers declined to mid-February, after which time no adults were collected. In 2007, three A. trivacuata were caught at INT2 in late January and early February, up to 4 weeks after this stream dried ().
Polyplectropus
Larvae were first found at INT sites in late April, mostly as first-instar larvae, though some middle-instar individuals were also found (Fig. 2D). The maximum size of Polyplectropus larvae increased from May to November. Small larvae continued to occur throughout the year, suggesting continuous egg hatching and possibly different timing of hatching between the two species. The total number of larvae increased in November, perhaps because larvae were confined within a smaller habitat as flow ceased in the stream channels, but highest numbers were found in pools (the preferred habitat of Polyplectropus) in January. Polyplectropus were very abundant in summer pools, with all size classes represented. In May Polyplectropus larval head widths at PER sites ranged between 0.25 and 0.75 mm, compared with a maximum of 0.25 mm at INT sites (data not shown), but numbers were too small to compare INT and PER sites confidently.
Adult Polyplectropus at the INT sites were present in all months of the trapping period, but reached peak numbers between mid-February and mid-March (). In 2007, adult Polyplectropus were caught at INT1 and INT2 throughout the trapping period, up to 14 weeks after the streams dried, though numbers declined during this time ().
Hydrobiosis
Early in the flowing water period (March-early April), all Hydrobiosis larvae were small, but appeared to grow rapidly (E). The largest-sized larvae first appeared in July or August, earlier than all other taxa except Psilochorema, and Hydrobiosis pupae appeared from August (). Early instar Hydrobiosis larvae were present in all samples, suggesting that egg hatching occurred throughout the year. Larvae were absent from summer pools. In May, some medium-sized larvae were present at the INT sites as well as a large number of first- or second-instar larvae; however, the range of sizes was still narrower in INT sites than in PER sites (C).
Adult Hydrobiosis were found at the INT sites from the start of the trapping period in mid-October, and reached peak numbers from mid-December to mid-January (). No adult Hydrobiosis were found after mid-March. In 2007, adult Hydrobiosis were caught at INT1 and INT2 throughout the trapping period, up to 14 weeks after the streams dried, though numbers declined during this time ().
Psilochorema
The population structure and growth of Psilochorema larvae were similar to Hydrobiosis, except that Psilochorema pupae first appeared in October rather than August, and Psilochorema larvae were present in summer pools (F and 3D). In November, a large number of first-instar Psilochorema larvae were found, possibly representing a second generation, but it is unclear what became of these individuals in January.
Adult Psilochorema at the INT sites were present in all Malaise samples (). Numbers were fairly constant throughout the sampling period, but were slightly higher from mid-February to mid-March. In 2007, adult Psilochorema were caught at INT1 up to 10 weeks after the stream dried and at INT2 up to 14 weeks after the streams dried, numbers remaining constant over this time ().
Potamopyrgus antipodarum
At the beginning of the flowing water period, only medium-sized individuals were found (G). Minute individuals were present from late April to November, suggesting continuous release of juveniles occurred during this period. Many minute P. antipodarum appeared in August and October, coinciding with the time the largest individuals first appeared. November had much higher densities than other months, though it is not clear why. From August to January, the size distribution appeared to show two overlapping generations. At the INT sites, P. antipodarum were present in summer pools, though numbers were lower than in November. In May, the INT sites contained a wider range of sizes than did the PER sites (E).
Discussion
Life history traits in intermittent streams
The first aim of our study was to determine whether the intermittent stream fauna showed a greater proportion than the perennial stream fauna of life history traits suitable for surviving periodic drying. Strong differences were apparent in almost all trait categories, but not all the patterns were clear or matched our expectations.
Plurivoltinism was more common in intermittent than perennial streams, consistent with Williams (Citation2006) and Arscott et al. (Citation2010). A short life cycle and rapid growth is of obvious advantage in a temporary and unpredictable habitat. Adults living longer than 10 days were more common in intermittent streams, reflecting, in part, that intermittent streams had a higher proportion of non-insects such as copepods and snails. In some insect orders (e.g. stoneflies and caddisflies), terrestrial adults may be sufficiently long-lived to survive the dry period as adults, and in these orders, species or genera with longer-lived adults may be favoured over those with shorter-lived adults. Among caddisflies there was a slight indication that longer-lived species or genera (e.g. Hydrobiosis, Psilochorema, Polyplectropus and Oeconesus, according to the species trait database) were more common in intermittent streams, but numbers were too low to make strong conclusions. No pattern could be seen among stoneflies.
The low dissemination potential, high prevalence of asexual reproduction and high occurrence of aquatic juvenile and adult stages among intermittent stream taxa also are mostly related to the high proportion of non-insects, which exhibit these traits more strongly than insects. Dissemination potential was expected to be higher in intermittent streams (Williams Citation2006; Bonada et al. Citation2007), because of taxa that recolonise each year by flight. However, at our sites, unpublished data suggest that the most common strategy to survive drought was to remain in dry stream bed sediments as a resistant life stage.
Individuals with the smallest body size were more common in intermittent than perennial streams, as predicted by Williams (Citation2006). However, 5–10-mm individuals were more common in perennial streams whereas 10–20-mm individuals were more common in intermittent streams; thus the relationship between body size and intermittency was not strong. Lower fecundity among intermittent stream taxa was opposite to the predictions of Williams (Citation2006) but consistent with the results of Arscott et al. (Citation2010), suggesting that this r-selected trait is not a feature of New Zealand intermittent streams.
Like Bonada et al. (Citation2007) and Arscott et al. (Citation2010), we have assumed that for each taxon, populations in intermittent streams have similar trait affinities to those in perennial streams. For some taxa, intermittent flow may lead to slight shifts in trait affinities, such as reduced size at emergence (Robinson & Buser Citation2007) and shorter generation time (Williams et al. Citation1995), possibly leading to changes in other traits, such as reduced number of descendants. The measured changes in life history traits in these studies are small relative to the size of the trait categories, and such changes are unlikely to alter the results presented here significantly. However, certain changes, such as parthenogenesis among mayflies and caddisflies in intermittent streams (B. Smith, NIWA, unpublished data), could significantly affect trait analyses. Thus further study of life history plasticity in intermittent waters would be valuable.
Growth rates and longevity of selected taxa
Our second aim was to describe the larval growth rates and longevity of selected insects and the growth rate of a freshwater snail. Assuming that eggs began to hatch from the time water returned in mid-March, then over the winter period Deleatidium reached final instar in a maximum of 7 months, Zephlebia and Polyplectropus in a maximum of 8 months, Hydrobiosis in a maximum of 4–5 months and Psilochorema in a maximum of 5 months.
Our results for Deleatidium are within the range of those found for winter generations of this genus in previous studies. For example, Deleatidium larvae reached maximum size in 9–11 months in two high country South Island streams (Huryn Citation1996), 7–9 months in a lowland South Island river (Scrimgeour Citation1991) and 6–9 months in two lowland North Island streams (Towns Citation1983). For Zephlebia, no corresponding published data could be found.
For Polyplectropus, the only data are from Michaelis (Citation1974), who observed one generation per year in a coldwater spring, and Huryn (1998), who calculated the larval lifespan as 12–14 months in two high country South Island streams. Huryn (Citation1998) also provides the only comparable data for Hydrobiosis and Psilochorema, calculating their larval lifespans as 14–27 and 9–16 months, respectively, in two high country South Island streams.
Huryn's estimates for the three caddisflies are considerably longer than we found. The most likely reason for the faster larval growth rates in our study is a difference in water temperature (average 9–10°C in INT1-3, compared with 2–3°C in Huryn 1996 between May and August). In addition to temperature, certain cues in intermittent streams, e.g. falling water levels, may stimulate accelerated larval development in some taxa (Williams Citation2006; Robinson & Buser Citation2007), but whether such cues influenced growth rates in the present study is not clear. Surface flow ceased briefly on several occasions during larval development in INT2 and INT3, but not in INT1 (Storey & Quinn Citation2008) and there were no apparent differences in larval growth rates among these sites. Therefore, it is likely that the growth rates described here apply to perennial as well as to intermittent streams.
In our study, final instars of A. trivacuata were not seen, as this species appeared to complete larval development on land; larvae disappeared from the streams about 5 months after first-instar larvae were first seen. These observations are similar to those of Winterbourn (Citation1966), who suggested that A. trivacuata larvae develop over a period of 4 months, with maximum emergence occurring from August to November.
Growth rates of P. antipodarum were not easy to determine, as individuals of various sizes were present at the start of the flowing water period. However, our data appeared to indicate two overlapping generations per year, similar to the observations of Towns (1981). The seasonality of generations was also comparable with previous studies; the influx of small snails that we noted in October corresponded to that in Towns (1981), whereas the influx we observed in May/June was later than a corresponding influx in Michaelis (Citation1974) and Towns (Citation1981). The delay in the May/June influx at our sites may be because after the summer drought, adults needed to recover biomass before they could invest in developing embryos (as in Winterbourn Citation1970).
Life history patterns in intermittent streams
Our third aim was to infer whether selected stream macroinvertebrates use particular life history stages to survive summer drought. In the INT sites, all the insect taxa studied showed a similar pattern in their life history. At the start of the flow period, almost all larvae were very small and their maximum size gradually increased from April until August, October or November (depending on genus). This was in contrast with the perennial sites, where, in May, the wide size range of Deleatidium, Zephlebia, Hydrobiosis and Psilochorema indicated aseasonal and asynchronous life histories, and possibly differences among species within each genus. It seems most likely, therefore, that at the INT sites the insect taxa studied survive the summer mostly as eggs, though we cannot exclude the possibility that they over-summered as first-instar larvae (e.g. Williams Citation2006). A few larger larvae of Deleatidium, Zephlebia and Polyplectropus were noted at the INT sites at the start of the flow period, implying that they had over-summered in the moist bed sediments as larvae. However, only a very few of these larger larvae were seen, and, except in the case of Zephlebia, they did not re-appear in subsequent samples.
Potamopyrgus antipodarum, the one non-insect taxon in this study, was the only taxon showing a broad range of size classes throughout the study period. This suggests that P. antipodarum can survive summer drying in various stages of maturity, as found by Winterbourn (Citation1970) in a pond that was drained for 1 month. This is not surprising as the snail's shell and operculum would provide an effective defence against desiccation. At the beginning of the flow period, the smallest size classes were absent, possibly because these did not have a well-enough developed shell to withstand desiccation. Therefore, despite an interruption of development during the summer dry period, the life history patterns of P. antipodarum that we observed in summer-dry streams appear quite similar to those in perennial streams.
Surviving the dry period as aerial adults is another strategy available to insect taxa with relatively long adult lifespans. Adult Polyplectropus, Hydrobiosis and Psilochorema were present at the intermittent sites up to 3 months after stream drying, their abundances in Malaise traps declining only slightly, if at all, after flow ceased. Individuals in these samples may have survived the entire dry period as adults, dispersed from perennial waters more than 700 m away in neighbouring catchments (Kovats et al. Citation1996; Briers et al. Citation2004; Winterbourn et al. Citation2007), emerged from dry stream bed sediments, or undergone a period of adult dormancy (e.g. Sommerhauser et al. Citation1997; Smith et al. Citation2002).
Adaptability of fauna to dry and wet years
The fourth aim was to determine how well adapted New Zealand intermittent stream macroinvertebrates are to surviving dry years and taking advantage of wet years. During a dry year, the INT streams in this study may stop flowing for up to 5 months, whereas during a wet year, they may stop for only 2–3 weeks in February/March or not at all (R. Storey, unpublished data).
Most of the taxa in this study are probably able to survive if the dry season lasts for 5 months or less. For all taxa except Zephlebia and Polyplectropus, larvae reached their final instar by October (7 months after flow began) and adults were in flight from the earliest sampling in October–November. With larvae that left the water sometime before October, A. trivacuata may be particularly well-adapted to survive an early-onset drought, though the terrestrial larval stage still requires cool and damp conditions (Winterbourn Citation1966), and therefore may be affected by a warm, dry spring. Polyplectropus and Zephlebia are the only taxa that appeared likely to suffer decline during dry years. Polyplectropus larvae collected in November and January included a high proportion of small individuals that would not survive an early-onset drought, and their adult flight activity peaked in February, later than the other taxa.
Some taxa may be better able to survive a dry year than appears from their development rates during a normal year. Some Deleatidium species, for example, are able to hasten adult emergence if they detect environmental cues associated with drying (Robinson & Buser Citation2007), while holometabolous insects, in this case the caddisflies, have a pupal stage that may be able to resist some drying (e.g. Williams & Williams Citation1975). Overall, most or all of the taxa described here appear able to survive most extremes of drought experienced in the current climate. However, if future climate change resulted in dry periods of longer than 5 months, some of the taxa may not persist.
Most of the taxa in this study would also be able to take advantage of wet years, increasing survivorship and productivity. Almost all of the taxa had immature larvae near the end of the flow period, larvae that would be able to complete development if the flow period were extended. Only A. trivacuata may not be affected by a longer flow period, since during summer its larvae are rare and egg hatching is delayed, even in perennial streams (Winterbourn Citation1966; McLellan Citation1998).
Acknowledgements
We would like to thank Brian Smith for his expert advice and help with identifications; Ngaire Phillips for help with species trait analysis; Carola and Mike Hudson, Spencer and Gordon Macdonald for help with sample collection and their observations of local flow patterns; Graham Sevicke-Jones, Brett Stansfield, Vickie Hansen and Andrew Lamason for help with sample collection; and Holly Ferguson, Claire Taylor and Nathan Wagstaff for help with sample sorting. This research was funded by the Foundation for Research, Science and Technology through a postdoctoral fellowship (NIWX0401) to R. Storey.
References
- Arscott , DB , Larned , ST , Scarsbrook , MR and Lambert , P . 2010 . Aquatic invertebrate community structure along an intermittence gradient: Selwyn River, New Zealand . Journal of the North American Benthological Society , 29 : 530 – 545 .
- Bonada , N , Rieradevall , M and Prat , N . 2007 . Macroinvertebrate community structure and biological traits related to flow permanence in a Mediterranean river network . Hydrobiologia , 589 : 91 – 106 .
- Bouvet , Y . 1977 . “ Adaptations physiologiques et compartementales des Stenophylax (Limnephilidae) aux eaux temporaires ” . In Proceedings of the Second International Symposium on Trichoptera Edited by: Crichton , MI . 117 – 119 .
- Briers , RA , Gee , JHR , Cariss , HM and Geoghegan , R . 2004 . Inter-population dispersal by adult stoneflies detected by stable isotope enrichment . Freshwater Biology , 49 : 425 – 431 .
- Dieterich , M and Anderson , NH . 1990 . Temporary stream communities in western Oregon: Life histories of dominant mayflies and stoneflies . Northwest Science , 64 : 105
- Doledec , S , Phillips , N , Scarsbrook , MR , Riley , RH and Townsend , CR . 2006 . Comparison of structural and functional approaches to determining landuse effects on grassland stream invertebrate communities . Journal of the North American Benthological Society , 25 : 44 – 60 .
- Erman , NA and Erman , DC . 1995 . Spring permanence, Trichoptera species richness, and the role of drought . Journal of the Kansas Entomological Society , 68 : 50 – 64 .
- Forsyth , DJ . 1986 . Distribution and production of Chironomus in eutrophic Lake Ngapouri . New Zealand Journal of Marine and Freshwater Research , 20 : 47 – 54 .
- Fritz , KM and Dodds , WK . 2005 . Harshness: characterisation of intermittent stream habitat over space and time . Marine and Freshwater Research , 56 : 13 – 23 .
- Harper , PP and Hynes , HBN . 1970 . Diapause in the nymphs of Canadian winter stoneflies . Ecology , 51 : 925 – 927 .
- Hart , RC . 1985 . Seasonality of aquatic invertebrates in low-latitude and Southern Hemisphere inland waters . Hydrobiologia , 125 : 151 – 178 .
- Hawke's Bay Regional Council 2003 . Ruataniwha Plains Water Resources Investigation (Technical Report) .
- Huryn , AD . 1996 . Temperature-dependent growth and life cycle of Deleatidium (Ephemeroptera: Leptophlebiidae) in two high-country streams in New Zealand . Freshwater Biology , 36 : 351 – 361 .
- Huryn , AD . 1998 . Ecosystem-level evidence for top-down and bottom-up control of production in a grassland stream system . Oecologia , 115 : 173 – 183 .
- Imhof , JGA and Harrison , AD . 1981 . Survival of Diplectrona modesta Banks (Trichoptera: Hydropsychidae) during short periods of desiccation . Hydrobiologia , 77 : 61 – 63 .
- Kovats , Z , Ciborowski , J and Corkum , L . 1996 . Inland dispersal of adult aquatic insects . Freshwater Biology , 36 : 265 – 276 .
- Linklater , W and Winterbourn , MJ . 1993 . Life histories and production of two trichopteran shredders in New Zealand streams with different riparian vegetation . New Zealand Journal of Marine and Freshwater Research , 27 : 61 – 70 .
- McElravy , EP , Lamberti , GA and Resh , VH . 1989 . Year-to-year variation in the aquatic macroinvertebrate fauna of a northern California stream . Journal of the North American Benthological Society , 8 : 51 – 63 .
- McLellan , ID . 1998 . A revision of Acroperla (Plecoptera: Zelandoperlinae) and removal of species to Taraperla new genus . New Zealand Journal of Zoology , 25 : 185 – 203 .
- Michaelis FB 1974 . The Ecology of Waikoropupu Springs . Unpublished thesis , University of Canterbury , Christchurch .
- Mullan B , Porteous AS , Wratt D , Hollis M 2005 . Changes in drought risk with climate change . National Institute of Water and Atmospheric Research client report WLG2005–23 .
- Neboiss , A . 1986 . Atlas of Trichoptera of the Southwest Pacific-Australian Region , Dordrecht : W. Junk Publishers .
- Resh , VH and Rosenberg , DM . 2010 . Recent trends in life-history research on benthic macroinvertebrates . Journal of the North American Benthological Society , 29 : 207 – 219 .
- Riek E 1970 . Plecoptera . In: Division of Entomology CSIRO The Insects of Australia . Melbourne University Press , Canberra .
- Robinson , CT and Buser , T . 2007 . Density-dependent life history differences in a stream mayfly (Deleatidium) inhabiting permanent and intermittent stream reaches . New Zealand Journal of Marine and Freshwater Research , 41 : 265 – 271 .
- Scarsbrook , MR . 2000 . “ Life-histories ” . In New Zealand stream invertebrates: ecology and implications for management , Edited by: Collier , KJ and Winterbourn , MJ . Christchurch, New Zealand Limnological Society .
- Scrimgeour , GJ . 1991 . Life history and production of Deleatidium (Ephemeroptera: Leptophlebiidae) in an unstable New Zealand river . New Zealand Journal of Marine and Freshwater Research , 25 : 93 – 99 .
- Smith , BJ , Collier , KJ and Halliday , NJ . 2002 . Composition and flight periodicity of adult caddisflies in New Zealand hill-country catchments of contrasting land use . New Zealand Journal of Marine and Freshwater Research , 36 : 863 – 878 .
- Smith BJ , Ward JB 2007 . A guide to the New Zealand Hydrobiosidae caddisflies , National Institute of Water and Atmospheric Research .
- Sommerhauser M , Robert B , Schumacher H 1997 . Flight periods and life histories of caddisflies in temporary and permanent woodland brooks in the Lower Rhine area (Germany) . Proceedings of the 8th International Symposium on Trichoptera 1995 . Pp. 425 433 .
- Storey , RG and Quinn , JM . 2008 . Composition and temporal changes in macroinvertebrate communities of intermittent streams in Hawke's Bay, New Zealand . New Zealand Journal of Marine and Freshwater Research , 42 : 109 – 125 .
- Towns , DR . 1981 . Life histories of benthic invertebrates in a Kauri forest stream in northern New Zealand . Australian Journal of Marine and Freshwater Research , 32 : 191 – 211 .
- Towns , DR . 1983 . Life history patterns of six sympatric species of Leptophlebiidae (Ephemeroptera) in a New Zealand stream and the role of interspecific competition in their evolution . Hydrobiologia , 99 : 37 – 50 .
- Towns , DR . 1985 . “ Life history patterns and their influence on monitoring invertebrate communities ” . In Biological monitoring of freshwaters: Proceedings of a seminar. Part 2 , Edited by: Pridmore , RD and Cooper , AB . 225 – 240 . Wellington : National Water and Soil Conservation Authority .
- Towns , DR and Peters , WL . 1996 . Leptophlebiidae (Insecta: Ephemeroptera). Fauna of New Zealand No. 36 , Lincoln : Manaaki Whenua Press .
- Ward JB 1993 . Trichoptera adults: unpublished key .
- Williams , DD . 1996 . Environmental constraints in temporary fresh waters and their consequences for the insect fauna . Journal of the North American Benthological Society , 15 : 634 – 650 .
- Williams DD 2006 . The biology of temporary waters . 1st edition . Oxford University Press , Oxford .
- Williams , DD and Williams , NE . 1975 . A contribution to the biology of Ironoquia punctatissima (Trichoptera: Limnephilidae) . Canadian Entomologist , 107 : 829 – 832 .
- Williams , DD , Williams , NE and Hogg , ID . 1995 . Life history plasticity of Nemoura trispinosa (Plecoptera: Nemouridae) along a permanent-temporary water habitat gradient . Freshwater Biology , 34 : 155 – 163 .
- Winterbourn , MJ . 1966 . Studies on New Zealand stoneflies. 2. The ecology of Zelandoperla maculata (Hare) and Aucklandobius trivacuatus (Tillyard)-(Gripopterygidae) . New Zealand Journal of Science , 9 : 312 – 323 .
- Winterbourn , MJ . 1970 . Population studies on the New Zealand freshwater gastropod, Potamopyrgus antipodarum (Gray) . Proceedings of the Malacological Society of London , 39 : 139 – 149 .
- Winterbourn , MJ . 1973 . A guide to the freshwater mollusca of New Zealand . Tuatara , 20 : 141 – 159 .
- Winterbourn , MJ . 1974 . The life histories, trophic relations and production of Stenoperla prasina (Plecoptera) and Deleatidium sp. (Ephemeroptera) in a New Zealand river . Freshwater Biology , 4 : 507 – 524 .
- Winterbourn , MJ . 2004 . “ Stream invertebrates ” . In Freshwaters of New Zealand , Edited by: Harding , J , Mosley , P , Pearson , C and Sorrell , B . Christchurch : New Zealand Hydrological Society and New Zealand Limnological Society .
- Winterbourn , MJ . 2010 . Life histories of two stoneflies (Plecoptera: Gripopterygidae) in two streams on the West Coast, New Zealand . New Zealand Natural Sciences , 35 : 1 – 8 .
- Winterbourn , MJ , Rounick , JS and Cowie , B . 1981 . Are New Zealand stream ecosystems really different? . New Zealand Journal of Marine and Freshwater Research , 15 : 321 – 328 .
- Winterbourn MJ , Gregson KLD , Dolphin CH 2000 . Guide to the Aquatic Insects of New Zealand . 3rd edition . Bulletin of the Entomological Society of New Zealand 13
- Winterbourn , MJ , Chadderton , WL , Entrekin , SA , Tank , JL and Harding , JS . 2007 . Distribution and dispersal of adult stream insects in a heterogeneous montane environment . Fundamental and Applied Limnology , 168 : 127 – 135 .
- Winterbourn , M , Cadbury , S , Ilg , C and Milner , A . 2008 . Mayfly production in a New Zealand glacial stream and the potential effect of climate change . Hydrobiologia , 603 : 211 – 219 .
- Wissinger , SA , Brown , WS and Jannot , JE . 2003 . Caddisfly life histories along permanence gradients in high-altitude wetlands in Colorado (U.S.A.) . Freshwater Biology , 48 : 255 – 270 .