Abstract
The aims of this study were to investigate the population dynamics and distribution of cyanobacteria in three New Zealand lakes, to detect the presence of microcystin-producing mcyE genes in potentially toxigenic strains and to correlate the finding with microcystin production. A total of 18 samples collected over a 6-week period from Lakes Rotorua, Rotoiti and Rotoehu were found to be predominantly composed of Microcystis spp., Anabaena spp., Coelosphaerium spp. and Aphanocapsa spp. The 820-bp-long region of the microcystin synthetase gene (mcyE) was successfully amplified for 11 out of the 18 water samples in genera of Microcystis and Anabaena. This provides the first documented evidence of Anabaena sp. carrying the potential to produce microcystins in New Zealand. Microcystin-LR was the only variant detected by matrix-assisted laser desorption/ionisation time-of-flight mass spectrometry (MALDI-TOF MS) analysis and was present in all samples from Lake Rotoehu.
Introduction
More than 2000 cyanobacterial species (c. 150 genera) have been recorded worldwide, some of which include toxin-producing strains (at least eight genera in freshwater habitats; Skulberg et al. Citation1993; Codd et al. Citation2005). Cyanotoxins, as they are commonly known, pose a threat to humans and animals either through drinking or via contact with contaminated water.
The most frequently found cyanotoxins are the hepatotoxic microcystins (MCs), produced by species from the genera Microcystis, Anabaena, Planktothrix, Nostoc and Anabaenopsis (Sivonen & Jones Citation1999). There are around 90 variants of MC (Zurawell et al. Citation2005; Pearson et al. Citation2010), all with varying toxicity. MCs are extremely stable peptides that are resistant to heat, chemical hydrolysis and oxidation; if cell-bound, under dark conditions or as dried scums on the shore, MCs may persist for months or years (Sivonen & Jones Citation1999).
The gene cluster involved in MC synthesis (mcyA-J) has been identified and sequenced (Dittmann et al. Citation1997; Nishizawa et al. Citation1999; Tillett et al. 2000) allowing early detection of MC production potential from environmental samples by polymerase chain reaction (PCR) (Kurmayer & Kutzenberger Citation2003; Vaitomaa et al. Citation2003). Out of the many potentially usable MC genes, the mcyE gene was chosen for this study. It encodes an enzyme, polyketide peptide synthetase, responsible for the synthesis of the two most toxic amino acids in the MC molecule, the Adda moiety (Welker & Döhren Citation2006), which is fundamental for interacting with phosphatase enzymes (Barford & Keller Citation1994; Goldberg et al. Citation1995) and D-glutamate. The increased conservation of this region as compared with the other parts of the MC molecule made the mcyE gene a more reliable marker for detecting MC producers (Rantala et al. Citation2006).
The Rotorua lakes region (central North Island, New Zealand) incorporates 12 main lakes () ranging in trophic status from oligotrophic to eutrophic. Over the last 40 years, the water quality of many of these lakes has decreased significantly resulting in a rise in frequency of cyanobacterial blooms (Wood et al. Citation2006a, Citationb). This study focused on three of the eutrophic lakes in this region: Rotorua, Rotoiti and Rotoehu. In 1993, local residents from around the Rotorua lakes region were forced to find alternative water supplies after complaints of unpleasant taste and smell from domestic water supplies (Power & Donald Citation1993). Following this incident, monitoring of Lake Rotoehu began and elevated concentrations of cyanobacteria were detected. Monitoring of Lakes Rotorua and Rotoiti did not begin until 1997 when the occurrence of a toxic cyanobacterial bloom prompted an investigation (Wilding Citation2000). Regional authorities now routinely analyse cyanobacterial concentrations. However, cyanobacterial concentrations are not necessarily related to cyanotoxin concentrations (Wood et al. Citation2006a, Citationb) and there is a lack of information on which cyanobacterial species are responsible for the production of MC in these lakes.
Figure 1 Location of the Rotorua Lakes region (North Island, New Zealand) and the sampling sites (☆).
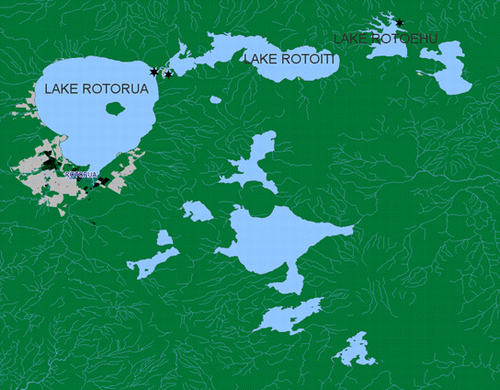
A major factor driving changes in toxin levels appears to be the successive replacement of toxic and non-toxic genotypes within a water body. Recent advances in molecular methods provide promising tools for determining cyanobacterial composition in environmental samples and providing additional information on toxin production potential (Tillett et al. Citation2001; Baker et al. Citation2002; Yoshida et al. Citation2005; Mankiewicz-Boczek et al. Citation2006; Kim et al. Citation2010). The main objectives of this study were to identify MC-producing species by PCR and to monitor how mcyE genotypes changed over a 6-week period.
Methods
Sampling sites and sample collection
Lake Rotorua (38°03′S, 176°19′E, ), has a surface area of 80.2 km2, maximum depth of 45 m and is currently classified as eutrophic (Etheredge & Pridmore Citation1987; Hofstra et al. Citation2009). Cyanobacterial cell concentrations have, at times, been almost 1000 times higher (March 1997; Wilding Citation2000) than the management guideline value of 100,000 cyanobacterial cells/ml that would lead to a moderate health alert and recommended by WHO for safe practice in recreational waters (Lawton et al. Citation1999; WHO Citation2003).
Lake Rotoehu (38°00′S, 176°32′E, ) is a small, shallow lake with a maximum depth of 8.2 m and a surface area of 8 km2 (Etheredge & Pridmore Citation1987; Hofstra et al. Citation2009). The lake is hypereutrophic and has experienced severe blooms since 2001.
Lake Rotoiti (38°03′S, 176°20′E, ), is a deep lake (maximum depth 93.4 m) of moderate size with a surface area of 34.6 km2 (Etheredge & Pridmore Citation1987; Hofstra et al. Citation2009). It is categorised as eutrophic with decreasing water quality. Health warnings are commonly issued in summer because of high concentrations of potentially toxic cyanobacteria (Wood et al. Citation2006a).
Surface water samples were collected weekly between 19 February 2007 and 27 March 2007 from one sampling site at each lake: Okawa Bay (Lake Rotoiti), Ohau Channel (Lake Rotorua) and Otautu Bay (Lake Rotoehu). Samples were collected in sterile bottles (400 ml) without concentration. The water samples were well mixed and a 50 ml subsample preserved using Lugol's Iodine. The remainder was stored in the dark at −20 °C until further analysis.
Cyanobacterial enumeration and identification
Identification and enumeration of cyanobacteria was carried out using an inverted Zeiss microscope and Utermöhl settling chambers (Utermöhl Citation1958). Identification to species level was made with taxonomic guides of Baker (Citation1991, Citation1992), Baker & Fabbro (Citation2002), McGregor & Fabbro (Citation2001), and Wood et al. (Citation2005).
Genomic DNA extraction and PCR amplification
A simple and effective technique (Wood SA, pers. comm.) was used to break open the cyanobacterial gas vesicles and reduce buoyancy prior to centrifugation: the environmental samples were subjected to pressure by placing them into a parafilmed syringe. A subsample (10 ml) was centrifuged (3000g, 20 min) and the supernatant decanted. The pellets were re-suspended (vortexed) in the remaining liquid, transferred to a clean Eppendorf tube and further centrifuged (3000g, 10 min). DNA extractions were carried out using a PureLink Genomic DNA Minikit (Invitrogen) following the Gram-negative bacterial protocol as per manufacturer's instructions. Extracted DNA was quantified and stored at −80 °C until further use.
Each sample was analysed using five different primer sets (). Final concentrations in the 25-µl reaction volumes were: approximately 10 ng DNA, 24 µM BSA, 200 µM dNTPs, 1x-Mg buffer, 2.5 mM MgCl2, 48 µM of each relevant forward and reverse primer and 0.25 units of Platinum Taq DNA Polymerase (Invitrogen). Thermal cycling conditions were: 95 °C for 2 min followed by 95 °C for 30 s, 56 °C for 30 s and 72 °C for 1 min, repeated for 35 cycles with a final extension of 72 °C for 7 min. The Anabaena mcyE gene conditions were: 94 °C for 4 min followed by 94 °C for 30 s, 52 °C for 30 s and 72 °C for 1 min, repeated for 35 cycles with a final extension of 72 °C for 7 min. PCR reactions were run on an iCycler (Biorad). PCR products were combined and visualised on a 1.5% agarose gel. The Anabaena mcyE PCR products were purified using a High Pure PCR product purification kit (Roche Diagnostics, New Zealand) and sequenced using the BigDye Terminator v3.1 Cycle Sequencing Kit (Applied Biosystems). Sequencing employed primers AnamcyE-F2-2 and AnamcyE-R12-2.
Table 1 Primers used in detecting mcyE and 16S, rRNA genes.
Microcystin analysis by MALDI-TOF MS
Matrix-assisted laser desorption/ionisation time-of-flight mass spectrometry (MALDI-TOF MS)) is a reliable technique for the rapid detection and identification of MC variants in small samples (Welker et al. Citation2002; Saker et al. Citation2005; Haande et al. Citation2007). The analytical chemical technique was used to detect the presence or absence of MC in the surface waters of the study lakes.
Subsamples (15 ml) were lyophilised (FreeZone6, Labconco, Kansas City, MO, USA) and extracted in 90% methanol containing 0.1% trifluoroacetic acid (TFA) and cell debris pelleted by centrifugation (1200g, 2 min). An aliquot of the supernatant was mixed 1:1 with α-cyano-4-hydroxycinnamic acid saturated with 1:1:2 acetonitrile/methanol/0.1% TFA and placed directly on a 600-µm anchorchip (Bruker Daltonics). Once dry, each spot was washed with 0.1% TFA in 10 mM NH4H2PO4.
Positive ions from each sample were analysed on an AutoFlex II (Bruker Daltonics) MALDI-TOF mass spectrometer using pulsed ion extraction of 60 ns and suppressing ions below 500 Da. Sample m/z ratios over a range of 600–2200 were assessed using the reflector detector with an acceleration voltage of 19 kV and a reflector voltage of 20 kV. The instrument was calibrated every four samples using a peptide calibration standard (Bruker Daltonics) laid between samples.
Results
Three Anabaena species were recorded in Lake Rotorua: A. circinalis, A. planktonica and A. torulosa (A). The highest concentration (9.8×104 cells/ml) of all combined Anabaena species was recorded on the 26 February. Microcystis spp. were highest on the third week of March (2.9×105 cells/ml; ).
The Lake Rotoehu samples contained the highest cyanobacterial cell concentrations of all three lakes peaking at 1.8×107 cells/ml on the last week of March 2007. Water samples were dominated by dense colonial aggregations of Microcystis spp. () and A. circinalis. The highest cell concentrations of Microcystis spp. were recorded in the first (1.1×106 cells/ml) and final weeks of March 2007 (1.8×107 cells/ml).
Figure 3 Microcystis spp. concentration (log scale) in water samples collected over a 6-week period from the three Rotorua lakes.
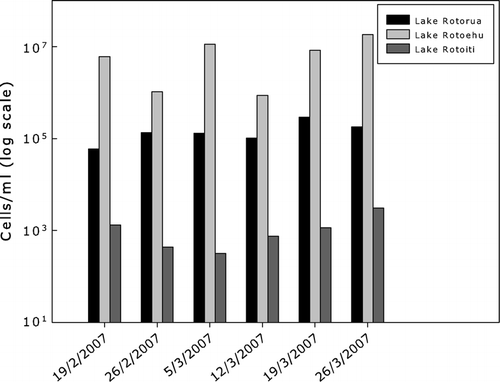
The lowest cyanobacterial cell concentrations, including Microcystis spp., were recorded for Lake Rotoiti (). The Lake Rotoiti samples contained high cell concentrations of Aphanocapsa holsatica and Coelosphaerium spp., which fluctuated over the 6-week sampling period (C).
Molecular characterisation
The generic MC synthetase gene (mcyE) was successfully amplified in 11 out of the 18 lake samples (). The mcyE gene was not detected in samples collected on the 19 and 26 February 2007, and the 5 March 2007 from Lakes Rotoiti and Rotorua. Additionally, the mcyE gene was not detected in the Lake Rotorua sample on the 26 March 2007 ().
Table 2 Method comparison between cyanobacteria counts carried out with microscopy versus polymerase chain reaction (PCR) detection of the microcystin synthetase gene E (mcyE) and the 16S rRNA gene.
A PCR product of approximately 250 bp long was successfully amplified using the Microcystis specific mcyE primers in two samples from Lake Rotorua (12 and 19 March 2007), in three samples from Lake Rotoiti (12, 19 and 26 March 2007) and in five out of six samples from Lake Rotoehu (). A 244-bp-long PCR product was successfully amplified using the Anabaena specific mcyE primers in all samples from Lakes Rotoehu and Rotoiti except for the samples collected on the 26 March 2007 in Lake Rotoehu and the 26 February 2007 in Lake Rotoiti. Microscopic analysis did not detect any cyanobacteria in the Lake Rotoehu sample and there were only 175 cells/ml in the Lake Rotoiti sample. No Anabaena specific mcyE genes were detected in the Lake Rotorua samples.
As a replacement to a positive control that was lacking, a partial trimmed sequence (144 bp) of the Anabaena species-specific mcyE gene was acquired from a sample from Lake Rotoehu collected on the 19 March 2007 (HQ654050). Using BlastN the sequence was 100% similar to Anabaena lemmermannii (AY382547) and several other Anabaena sp. sequences (e.g. EF565285 and AY212249).
In general, the PCR results of the 16S rRNA Anabaena and Microcystis spp. correlated well with the identification of these genera via microscopy in all three lakes (). In the samples collected in February 2007 in Lakes Rotorua (A) and Rotoiti (B) and also on the 5 and 26 March 2007 in Lake Rotoiti, Microcystis species were not detected by PCR of the 16S rRNA gene.
Microcystin-LR was the only variant detected by MALDI-TOF MS (limit of detection, LOD = 86 µg/l) and it was present in all samples from Lake Rotoehu. The latter correlated with the presence of the generic mcyE gene by PCR and the presence of Microcystis and Anabaena cells via microscope counts (C) in all samples except for the one collected in the last week of March 2007. Although the mcyE gene was detected in lakes Rotoiti and Rotorua, no MCs were found in these lakes (A and B).
Discussion
Eutrophication and the development of cyanobacterial blooms are of great environmental concern in waters all around the globe (Gobler et al. Citation2007). The advent of PCR techniques aimed at detecting genes responsible for toxin production has allowed the development of methods that can track changes in the proportion of toxigenic strains (Tillett et al. Citation2001; Baker et al. Citation2002; Yoshida et al. Citation2005; Mankiewicz-Boczek et al. Citation2006; Kim et al. Citation2010). These methods could be incorporated into current monitoring programmes in New Zealand, which are based primarily of cell counts and biovolumes (Wood et al. Citation2009).
The misidentification of cyanobacterial species by the use of standard light microscopy techniques, may lead to the assignment of certain isolates to the wrong genus, e.g. Aphanocapsa spp. to Microcystis spp. and vice versa (Wilson et al. Citation2000). The current study has demonstrated the validity of PCR use for the detection of toxigenic cyanobacterial strains in eutrophic waters and contrasted the method with the traditional use of microscopy.
Bloom dynamics of cyanobacteria
The eutrophic nature of Lakes Rotorua, Rotoiti and Rotoehu affects the dynamics of the phytoplankton community and promotes the continued presence of bloom-forming species such as Microcystis and Anabaena over the summer period. This is especially the case with Lakes Rotorua and Rotoehu, as the process of eutrophication is of a more advanced nature than in Lake Rotoiti. Furthermore, as nutrient load increases in the lakes, cyanobacterial blooms will tend to occur earlier in the year and last longer as has been observed for Lakes Rotoiti and Rotoehu (Pick & Lean Citation1987).
Water samples collected over a 6-week period from Lakes Rotorua, Rotoiti and Rotoehu were found to be predominantly composed of Microcystis spp., Anabaena spp., Coelosphaerium spp. and Aphanocapsa spp.
In 2004, Anabaena spp. and Microcystis spp. accounted for 90% of the cyanobacterial cell abundance in Lakes Rotoiti and Rotoehu (Wood et al. Citation2006a). In 2007, blooms in Lake Rotoiti were composed primarily of Microcystis spp. (9.4×105 cells/ml) until replaced by Anabaena spp. at the end of the summer. In contrast, the current study, showed water samples dominated by Coelosphaerium spp. and Aphanocapsa spp. and only 30% abundance of either Anabaena spp. or Microcystis spp. in Lake Rotoiti (C).
Differences in cell concentrations may be attributable to sampling regime. High variation is common in samples taken just a few minutes or 100 m apart (Sivonen & Jones Citation1999). Hence, temporal and spatial variation of cyanobacterial cell density within a water body, even in shallow lakes, may be an important factor to take into account when collecting replicate samples.
The variation of cyanobacterial cell concentration between sampling weeks may have been related to the displacement of cyanobacterial blooms from the sampling site at the time of collection by environmental factors such as wind, which promotes the patchy distribution of planktonic cells. These fluctuations could also be attributed to the rapid growth rate of Microcystis spp. or as a result of changes in recruitment to surface populations because of vertical migration of benthic species (Preston et al. Citation1980; Carmichael & Gorham Citation1981; Vezie et al. Citation1998; Latour & Giraudet Citation2004). Blooms in Lake Rotoiti have been known to change from blooms dominated by non-toxic Anabaena spp. to blooms mainly containing toxic Microcystis spp. in the course of just 2 weeks (Wood et al. Citation2006a).
The 16S rRNA and mcyE gene targets
The use of species-specific 16S rRNA primers provides a relatively new method for identification of the presence of potentially toxigenic strains of cyanobacteria in a sample (Rudi et al. Citation1997; Wilmotte & Herdman Citation2001).
The lack of detection of the 16S rRNA gene in Microcystis spp. in two samples from Lake Rotorua (A) and in four samples from Lake Rotoiti may represent a misidentification of cyanobacterial species by microscopy, variation in primer sensitivity with cell concentration or presence of inhibiting compounds. The latter can be addressed by the use of Internal Control Fragments (ICF) in the PCR reactions (Fergusson & Saint Citation2000).
Positive PCR detection of the 16S rRNA gene with either set of primers (Anabaena or Microcystis) occurred at a minimum cell concentration of approximately 700 cells/ml (Lake Rotoiti, data not shown) which corresponds to previous cyanobacterial studies (Fergusson & Saint Citation2000; Baker et al. Citation2001). However, standard curves at a range of cyanobacterial cell concentrations were not performed, so the detection limit for obtaining a positive 16S rRNA PCR could have been lower. Fergusson & Saint (Citation2000) found the PCR detection limit for Anabaena spp. to be 2000 cells/ml and mentioned the possibility of detecting lower levels with careful optimisation of the PCR conditions. Similarly, Baker et al. Citation2001 obtained positive results with the presence of 1,000 toxigenic cyanobacterial cells/ml and noted that cell concentrations as low as 10 cells/ml may be sufficient for PCR detection. The latter can be achieved by the use of fluorescent fragment detection PCR (FFD-PCR) to detect highly toxigenic species in low abundance (Coyne et al. Citation2001).
The presence of the mcyE gene in only some samples in Lakes Rotorua and Rotoiti and its detection in all samples of Lake Rotoehu (A) is indicative of the presence of potentially toxigenic strains of cyanobacteria. It is important to mention that as cyanobacterial cell concentrations increase in a lake prior to the formation of a bloom, the proportion of potentially hepatotoxic genotypes within the population may also increase. This raises the level of health hazard presented to animals and humans associated with the relevant water bodies.
Previous research has shown that cyanobacterial strains of the genera Anabaena, Microcystis Planktothrix, Nodularia, Nostoc, Pseudanabaena and Chroococcus may be carriers of the mcy gene clusters and therefore are considered potential MC producers (Börner & Dittmann Citation2005; Jungblut & Neilan Citation2006; Sipari et al. Citation2010; Sivonen & Börner Citation2008). However, presence of the toxin-producing gene is not a guarantee of MC production (Mikalsen et al. Citation2003; Via-Ordorika et al. Citation2004) and neither Planktothrix, Nodularia, Nostoc or Chroococcus were detected in the current study.
The present results have confirmed both Anabaena spp. and Microcystis spp. as carriers of the mcyE gene in the samples mentioned above. As a result of the limited scope of this project, other cyanobacterial species were not tested and, therefore, could have also been carriers of the toxigenic gene. For example, in Lake Rotorua the mcyE gene was detected during the second and third weeks of March 2007 and attributed to Microcystis as a result of the simultaneous detection of the gene with the genera-specific primers (A). However, other cyanobacterial genera were also detected by microscopy including Aphanocapsa, Coelosphaerium and Planktolyngbya (A) and, unless other molecular methods are included into this approach (e.g. cloning and sequencing), the possibility of the latter genera having the potential to also produce MCs cannot be excluded.
The current approach that combines two methods (with the possibility of incorporating other molecular techniques) for the monitoring of cyanobacterial blooms has the added benefit of relating the presence of the toxigenic mcyE gene to the various morphospecies confirmed by microscopy. For instance, both Microcystis and Anabaena genera were found to carry the mcyE gene in Lakes Rotoiti and Rotoehu (B and C). Through microscopy, A. circinalis, A. planktonica and A. torulosa were observed in these lakes (including some unidentified Anabaena spp.) and either one or all of these species may carry the potential to produce MCs.
Methodological approach: integration of traditional microscopy and molecular techniques for monitoring of toxic cyanobacterial blooms
The methods that have been presented in the project exhibit both advantages and disadvantages. The enumeration of cyanobacterial cells by microscopy has the advantage of being able visually to identify the presence of potentially toxic genera or species. It is also a cheap and technologically simple method compared with molecular methods. However, depending upon the accuracy required, microscopy may become very time-consuming and the ability to distinguish toxigenic strains from non-toxigenic strains from a determined population is not possible.
Molecular methods, such as PCR, although of an extremely sensitive nature, specific and reproducible with the ability for adaptation to high-throughput systems, require the prior knowledge of the gene sequence to be targeted by the primers. Stringent conditions must be in place to ensure a sterile environment is maintained while setting up the samples as DNA contamination may lead to the amplification of non-specific products. Moreover, the prediction and assessment of biases occurring as a result of variable primer annealing efficiencies as PCR cycles increase may be difficult. The bias may be caused by nucleotide mismatches between the primer and some of the target sites and it is possible to reduce this effect by the adequate choice and careful design of primers (Felske and Osborn Citation2005).
The combination of standard microscopy with the power of molecular tools for routine monitoring surveillance programmes of cyanobacterial blooms will be valuable in differentiating an early signal of potential producers of MC in water bodies at a national or international level. DNA primers are able to be custom-designed according to the local genetic cyanobacterial population present in the specific lake that is being monitored. The cost of DNA extractions may be removed in lakes with cyanobacterial cell concentrations that approach 1x106 cells/ml and that are composed mainly of intact cells by employing an alternative whole-cell method of PCR template preparation (Baker et al. Citation2001).
In addition to PCR, other molecular methods that have been employed in the past to detect and discriminate between toxic cyanobacteria using mcy genes include Southern hybridisation, dot blot hybridisation, denaturing gradient gel electrophoresis (DGGE), restriction fragment length polymorphism (RFLP), cloning and sequencing (Dittmann & Börner Citation2005; Kim et al. Citation2010; Okello et al. Citation2009). In the absence of other genera-specific primers (apart from Microcystis and Anabaena), some of these procedures (e.g. cloning and sequencing) enable the identification of other toxigenic gene carriers to the species level.
The PCR method employed in the current project was able only to detect the presence or absence of the MC gene and hence is not quantitative. Another option is the use of high-throughput methods of mcyE detection and quantification. For example, qPCR assays (Kurmayer & Kutzenberger Citation2003; Vaitomaa et al. Citation2003; Yoshida et al. Citation2005), together with DNA microchip technology (Rantala et al. Citation2008; Sipari et al. Citation2010), provide a more complex and expensive alternative for the assessment of the cyanobacterial community in relation to the active MC producers present through the measurement of gene copy numbers. This more complex technique may provide an improved correlation between the presence of mcyE and the production of MC in real time.
Production and detection of microcystins (MC)
Cyanobacteria produce more than 90 MC variants (Zurawell et al. Citation2005; Pearson et al. Citation2010) with a single strain capable of producing more than one variant of the toxin at any one time (Sivonen & Jones Citation1999). The presence of the mcy gene cluster enables the synthesis of MC variants within a given strain (Dittmann et al. Citation1997; Tillett Citation2000).
Of all the possible variants, only MC-LR was positively identified by MALDI-TOF MS in samples from Lake Rotoehu. Two samples from Lake Rotorua (A) and three samples from Lake Rotoiti (B) were found to carry the MC-producing gene but MC-LR was not detected.
The lack of correlation between MC concentration and the presence of mcyE genes in Lakes Rotorua and Rotoiti (as detected in cyanobacterial cells by PCR amplification; A and B) may have been related to: (1) the presence of novel mcyE gene variants in the population that would have remained undetected by the primers used; (2) the lower cell numbers in Lakes Rotoiti and Rotorua; (3) the intermittent mcyE gene expression with consequent MC production; (4) presence of MC-LR at lower levels than the LOD (86 µg/l) or (5) presence of an inactive mcyE gene. The latter has been previously demonstrated (Rantala et al. Citation2006; Gobler et al. 2007; Doblin et al. Citation2007).
It is also worth mentioning that covalently bound MCs are not detected by MALDI-TOF (Jonathan Puddick, pers. comm.). The possibility of inactive MCs (toxic cells in a stationary phase of growth, toxin stores within the sediment or dead cells present on the shore) having the ability to remain undetected with the potential of becoming active at some point in time upon their release could also account for the lack of correlation between the presence of the MC-producing gene and MC.
Conclusion
This study provides the first documented evidence of Anabaena sp. carrying the potential to produce MCs in New Zealand.
The PCR products obtained in this study are current proof that demonstrates the value of using this analytical procedure for the determination of potentially toxic cyanobacterial genotypes of the genera Microcystis and Anabaena. This may allow the prompt application of adequate corrective actions for health hazard prevention in the environmental monitoring of NZ eutrophic lakes.
Current environmental monitoring strategies in New Zealand by the relevant authorities require the rapid and accurate detection of potentially problematic cyanobacterial species such as Microcystis spp. and Anabaena spp. This includes issuing public health warnings when cyanobacterial cell concentrations are >19,000 cells/ml irrespective of the concentration of toxigenic strains present (Wood et al. Citation2009).
Routine monitoring by light microscopy lacks both the sensitivity and accuracy required for species-specific detection and enumeration of these species especially at the low levels normally found in non-bloom conditions. This deficit can be efficiently eliminated by the combined use of light microscopy with PCR thereby presenting water managers with a higher amount of informative data while increasing sample throughput. Furthermore, the ability to detect toxigenic cyanobacterial strains is an added benefit of the suggested strategy while saving on cost and sample preparation.
Acknowledgements
I thank Jonathan Puddick (Waikato University) for MALDI-TOF MS analysis and Sushila Pillai for the confocal images (Victoria University).
Susie Wood (Cawthron), Joe Zuccarello (Victoria University) and Irene Salinas (NIWA) are thanked for helpful advice throughout the project.
References
- Baker PD . 1991 . Identification of common noxious cyanobacteria: Part I , Nostocales . Melbourne, Urban Water Research Association of Australia .
- Baker PD . 1992 . Identification of common noxious cyanobacteria: Part II , Chroococcales & Oscillatoriales . Melbourne, Urban Water Research Association of Australia .
- Baker , JA , Entsch , B , Neilan , BA and McKay , DB . 2002 . Monitoring changing toxigenicity of a cyanobacterial bloom by molecular methods . Applied and Environmental Microbiology , 68 : 6070 – 6076 .
- Baker PD , Fabbro LD . 2002 . A guide to the identification of common blue-green algae (Cyanoprokaryotes) in Australian freshwaters. Identification Guide No. 25 . Canberra, Cooperative Research Centre for Freshwater Ecology .
- Baker , JA , Entsch , B , Neilan , BA and McKay , DB . 2001 . Identification of cyanobacteria and their toxigenicity in environmental samples by rapid molecular analysis . Environmental Toxicology , 16 : 472 – 482 .
- Barford , D and Keller , J . 1994 . Co-crystallization of the catalytic subunit of the serine/threonine specific protein phosphatase 1 from humanin complex with microcystin-LR . Journal of Molecular Biology , 235 : 763 – 766 .
- Börner T , Dittmann E 2005 . Molecular biology of cyanobacterial toxins . In : Huisman J, Matthijs HCP and Visser PM . Harmful cyanobacteria. Berlin, Springer . Pp. 25 – 40 .
- Carmichael WW, Gorham PR 1981 . The mosaic nature of toxic blooms of cyanobacteria . In : Carmichael WW . The water environment: algal toxins and health. New York, Plenum Press. Pp. 161 – 172 .
- Codd , GA , Morrison , LF and Metcalf , JS . 2005 . Cyanobacterial toxins: risk management for health protection . Toxicology and Applied Pharmacology , 203 : 264 – 272 .
- Coyne , KJ , Hutchins , DA , Hare , CE and Cary , SC . 2001 . Assessing temporal and spatial variability in Pfiesteria piscicida distributions using molecular probing techniques . Aquatic Microbial Ecology , 24 : 275 – 285 .
- Dittmann , E and Börner , T . 2005 . Genetic contributions to the risk assessment of microcystin in the environment . Toxicology and Applied Pharmacology , 203 : 192 – 200 .
- Dittmann , E , Neilan , BA , Erhard , M , von Döhren , H and Börner , T . 1997 . Insertational mutagenesis of a peptide synthesase gene which is responsible for hepatotoxin production in the cyanobacterium Microcystis aeruginosa PCC 7806 . Molecular Microbiology , 26 : 779 – 787 .
- Doblin , MA , Coyne , KJ , Rinta-Kanto , JM , Wilhem , SW and Dobbs , FC . 2007 . Dynamics and short-term survival of toxic cyanobacteria species in ballast water from NOBOB vessels transiting the Great Lakes—implications for HAB invasions . Harmful Algae , 6 : 519 – 530 .
- Etheredge MK , Pridmore RD . 1987 . The freshwater planktonic blue-greens (Cyanophyta/Cyanobacteria) of New Zealand—a taxonomic guide . Wellington, Ministry of Works and Development, Water and Soil. Miscellaneous Publication No. 111 .
- Felske A , Osborn AM 2005 . DNA fingerprinting of microbial communities . In : Osborn AM , Smith CJ . Molecular microbial ecology . Abingdon, Taylor & Francis . Pp. 65 – 96 .
- Fergusson , KM and Saint , CP . 2000 . Molecular phylogeny of Anabaena circinalis and its identification in environmental samples by PCR . Applied and Environmental Microbiology , 66 : 4145 – 4148 .
- Gobler , CJ , Davis , TW , Coyne , KJ and Boyer , GL . 2007 . Interactive influences of nutrient loading, zooplankton grazing and microcystin synthetase gene expression on cyanobacterial bloom dynamics in a eutrophic New York lake . Harmful Algae , 6 : 119 – 133 .
- Goldberg , J , Huang , H , Kwon , Y , Greengard , P , Nairn , AC and Kuriyan , J . 1995 . Three-dimensional structure of the catalytic subunit of protein serine/threonine phosphatase-1 . Nature , 376 : 745 – 753 .
- Haande , S , Ballot , A , Rohrlack , T , Fastner , J , Wiedner , C and Evdvardsen , B . 2007 . Diversity of Microcystis aeruginosa isolates from East-African water bodies . Archives of Microbiology , 188 : 15 – 25 .
- Hofstra , DE , Edwards , T , Clayton , JS and Shearer , JF . 2009 . New record of fungus Mycoleptodiscus terrestris from New Zealand . New Zealand Journal of Botany , 47 : 411 – 415 .
- Jungblut , AD and Neilan , BA . 2006 . Molecular identification and evolution of the cyclic peptide hepatotoxins, microcystin and nodularin, synthetase genes in three orders of cyanobacteria . Archives of Microbiology , 185 : 107 – 114 .
- Kim , S , Joung , S , Ahn , C , Ko , S , Boo , SM and Oh , H . 2010 . Annual variation of Microcystis genotypes and their potential toxicity in water and sediment from a eutrophic reservoir . FEMS Microbiology Ecology , 7 : 93 – 102 .
- Kurmayer , R and Kutzenberger , T . 2003 . Application of real-time PCR for quantification of microcystin genotypes in a population of the toxic cyanobacterium Microcystis sp . Applied and Environmental Microbiology , 69 : 6723 – 6730 .
- Latour , D and Giraudet , H . 2004 . Factors influencing the spatio-temporal distribution of benthic Microcystis aeruginosa colonies (cyanobacteria) in the hypertrophic Grangent Reservoir (Loire, France) . Comptes Rendus Biologies , 327 : 753 – 761 .
- Lawton L, Marsalek B, Padisák J, Chorus I 1999 . Chapter 12: Determination of cyanobacteria in the laboratory . In : Chorus I, Bartram J . Toxic cyanobacteria in water—a guide to their public health consequences, monitoring and management. London, E and FN Spon. Published on behalf of UNESCO, WHO and UNEP Online at: http://www.who.int/water_sanitation_health/resourcesquality/toxicyanbact/en/ [Retrieved 1 May 2007] .
- Mankiewicz-Boczek , J , Urbaniak , Z , Romanowska-Duda , Z and Izydorczyk , K . 2006 . Toxic cyanobacteria strains in Lowland dam reservoir (Sulejow Res., Central Poland): amplification of mcy genes for detection and identification . Polish Journal of Ecology , 54 : 171 – 180 .
- McGregor GB, Fabbro LD . 2001 . A guide to the identification of Australian freshwater planktonic Chroococales (Cyanoprokaryota/Cyanobacteria) . Albury, Cooperative Research Centre for Freshwater Ecology .
- Mikalsen , B , Boison , G , Skulberg , OM , Fastner , J , Davies , W , Gabrielsen , TM , Rudi , K and Jakobsen , KS . 2003 . Natural variation in the microcystin synthetase operon mcyABC and impact on microcystin production in Microcystis strains . Journal of Bacteriology , 185 : 2774 – 2785 .
- Nishizawa , T , Asayama , M , Fujii , K , Harada , K and Shirai , M . 1999 . Genetic analysis of the peptide synthetase genes for a cyclic heptapeptide microcystin in Microcystis spp . Journal of Biochemistry , 126 : 520 – 526 .
- Okello , W , Portmann , C , Erhard , M , Gademann , K and Kurmayer , R . 2009 . Occurrence of microcystin-producing cyanobacteria in Ugandan freshwater habitats . Environmental Toxicology , 25 : 367 – 380 .
- Pearson L, Mihali T, Moffitt M, Kellmann R, Neilan B 2010 . On the chemistry, toxicology and genetics of the cyanobacterial toxins, microcystin, nodularin, saxitoxin and cylindrospermopsin . Marine Drugs 8 : 1650 – 1680 ; doi: doi:10.3390/md8051650 .
- Pick , FR and Lean , DRS . 1987 . The role of macronutrients (C, N, P) in controlling cyanobacterial dominance in temperate lakes . New Zealand Journal of Marine and Freshwater Research , 21 : 425 – 434 .
- Power FM Donald RC 1993 . Blue-green algal bloom—Lake Rotoehu. Report presented to the Environmental Monitoring Committee. Bay of Plenty Regional Council . 8 June 1993 .
- Preston , T , Stewart , WDP and Reynolds , CS . 1980 . Bloom-forming cyanobacterium Microcystis aeruginosa overwinters on the sediment surface . Nature , 288 : 365 – 367 .
- Rantala , A , Fewer , DP , Hisbergues , M , Rouhiainen , L , Vaitomaa , J , Börner , T and Sivonen , K . 2004 . Phylogenetic evidence for the early evolution of microcystin synthesis . Proceedings of the National Academy of Sciences USA , 101 : 568 – 573 .
- Rantala , A , Rajaniemi-Wacklin , P , Lyra , C , Lepistö , L , Rintala , J , Mankiewicz-Boczek , J and Sivonen , K . 2006 . Detection of microcystin-producing cyanobacteria in Finnish lakes with genus-specific microcystin synthetase gene E (mcyE) PCR and associations with environmental factors . Applied and Environmental Microbiology , 72 : 6101 – 6110 .
- Rantala , A , Rizzi , E , Castiglioni , B , de Bellis , G and Sivonen , K . 2008 . Identification of hepatotoxin-producing cyanobacteria by DNA-chip . Environmental Microbiology , 10 : 653 – 664 .
- Rudi , K , Skulberg , OM , Larsen , F and Jacobsen , KS . 1997 . Strain characterization and classification of oxybacteria in clone cultures on the basis of 16S rRNA sequences from the variable regions V6, V7 and V8 . Applied and Environmental Microbiology , 63 : 2593 – 2599 .
- Saker , ML , Fastner , J , Dittmann , E , Christiansen , G and Vasconcelos , VM . 2005 . Variation between strains of the cyanobacterium Microcystis aeruginosa isolated from a Portuguese river . Journal of Applied Microbiology , 99 : 749 – 757 .
- Sipari , H , Rantala-Ylinen , A , Jokela , J , Oksanen , I and Sivonen , K . 2010 . Development of a chip assay and quantitative PCR for detecting microcystin synthetase E gene expression . Applied and Environmental Microbiology , 76 : 3797 – 3805 .
- Sivonen K, Börner T 2008 . Bioactive compounds produced by cyanobacteria . In : Herrero A, Flores E . The cyanobacteria: molecular biology, genomics and evolution. Norfolk, Caister Academic Press . Pp. 159 – 197 .
- Sivonen K, Jones GJ 1999 . Chapter 3. Cyanobacterial toxins. In: Chorus I, Bartram J ed. Toxic cyanobacteria in water—a guide to their public health consequences, monitoring and management . London, E and FN Spon. Published on behalf of UNESCO, WHO and UNEP Online at: http://www.who.int/water_sanitation_health/resourcesquality/toxicyanbact/en/[Retrieved 1 May 2007] .
- Skulberg OM, Carmichael WW, Codd GA, Skulberg R 1993 . Taxonomy of toxic Cyanophyceae (Cyanobacteria) . In : Falconer IA . Algal toxins in seafood and drinking water. London, Academic Press . Pp 145 – 163 .
- Tillett D 2000 . Taxonomy and molecular genetics of the saxitoxin producing cyanobacterium Anabaena circinalis . Unpublished PhD thesis, University of New South Wales .
- Tillet , D , Parker , DL and Neilan , BA . 2001 . Detection of toxigenicity by a probe for the microcystin synthetase A gene (mcyA) of the cyanobacterial genus Microcystis: comparison of toxicities with 16S rRNA and phycocyanin operon (phycocyanin intergenic spacer) phylogenies . Applied and Environmental Microbiology , 67 : 2810 – 2818 .
- Utermöhl , H . 1958 . Zur Vervollkommnung der quantitativen Phytoplankton-Methodik . Mitteilungen Internationale Vereinigung Theoretische und Angewandte Limnologie , 9 : 1 – 38 .
- Vaitomaa , J , Rantala , A , Halinen , K , Rouhiainen , L , Tallberg , P , Mokelke , L and Sivonen , K . 2003 . Quantitative real-time PCR for determination of microcystin synthetase E copy numbers for Microcystis and Anabaena in Lakes . Applied and Environmental Microbiology , 69 : 7289 – 7297 .
- Vezie , C , Brient , L , Sivonen , K , Bertru , G , Lefeuvre , JC and Saljinoja-Salonen , M . 1998 . Variation of microcystin content of cyanobacterial blooms and isolated strains in Grand-Lieu Lake (France) . Microbial Ecology , 35 : 126 – 135 .
- Via-Ordorika , L , Fastner , J , Kurmayer , R , Hisbergues , M , Dittmann , E , Komarek , J , Erhard , M and Chorus , I . 2004 . Distribution of microcystin-producing and non-microcystin-producing Microcystis sp. in European freshwater bodies: detection of microcystins and microcystin genes in individual colonies . Systematic and Applied Microbiology , 27 : 592 – 602 .
- Welker , M , Fastner , J , Erhard , M and von Dohren , H . 2002 . Applications of MALDI-TOF MS analysis in cyanotoxin research . Environmental Toxicology , 17 : 367 – 374 .
- Welker , M and von Döhren , H . 2006 . Cyanobacterial peptides—nature's own combinatorial biosynthesis . FEMS Microbiology Reviews , 30 : 530 – 563 .
- WHO 2003 . Cyanobacterial toxins: Microcystin-LR in drinking water. Background document for preparation of WHO guidelines for drinking-water quality . Geneva, World Health Organization .
- Wilding TK 2000 . Rotorua Lakes Algae Report . EBoP Environmental Report .
- Wilmotte A, Herdmann M 2001 . Phylogenetic relationships among cyanobacteria based on 16S rRNA sequences . In : Boone DR, Castenholz RW . Bergey's manual of systematic bacteriology , vol. 1 . New York, Springer . Pp. 487 – 493 .
- Wilson , KM , Schembri , MA , Baker , PD and Saint , CP . 2000 . Molecular characterization of the toxic cyanobacterium Cylindrospermopsis raciborskii and design of a species-specific PCR . Applied and Environmental Microbiology , 66 : 332 – 338 .
- Wood , SA , Crowe , ALM , Ruck , JG and Wear , RG . 2005 . New records of planktonic cyanobacteria in New Zealand freshwaters . New Zealand Journal of Botany , 43 : 479 – 492 .
- Wood , SA , Briggs , LR , Sprosen , J , Ruck , JG , Wear , RG , Holland , PT and Bloxham , M . 2006a . Changes in concentrations of microcystins in rainbow trout, freshwater mussels, and cyanobacteria in Lakes Rotoiti and Rotoehu . Environmental Toxicology , 21 : 205 – 222 .
- Wood SA, Hamilton DP , Paul WJ, Safi KA, Williamson WM 2009 . New Zealand guidelines for managing cyanobacteria in recreational freshwaters—interim guidelines . Wellington, Ministry for the Environment and Ministry of Health Wellington .
- Wood , SA , Holland , PT , Stirling , DJ , Briggs , LR , Sprosen , J , Ruck , G and Wear , RG . 2006b . Survey of cyanotoxins in New Zealand water bodies between 2001 and 2004 . New Zealand Journal of Marine and Freshwater Research , 40 : 585 – 597 .
- Yoshida , M , Yoshida , T , Takashima , Y , Kondo , R and Hiroishi , S . 2005 . Genetic diversity of the toxic cyanobacterium Microcystis in Lake Mikata . Environmental Toxicology , 20 : 229 – 234 .
- Zurawell , RW , Chen , H , Burke , JM and Prepas , EE . 2005 . Hepatotoxic cyanobacteria: a review of the biological importance of microcystins in freshwater environments . Journal of Toxicology and Environmental Health Part B: Critical Reviews , 8 : 1 – 37 .