Abstract
Every year, an area of sea ice forms around the continent of Antarctica that is inhabited by communities of microbes. Although the microbial component of this habitat has been postulated to play an ecologically important role in the Southern Ocean, our understanding of the diversity of Antarctic sea-ice bacteria is limited. The aim of this study was to identify bacterial components present in sea ice at Cape Hallett. Total community DNA was isolated from sea-ice cores and the 16S ribosomal RNA genes were amplified by polymerase chain reaction (PCR) using a range of universal and targeted primer sets. PCR products were de-replicated using restriction fragment length polymorphism (RFLP) and distinct RFLP types were sequenced. The major bacterial classes identified consisted of α-proteobacteria, γ-proteobacteria, Cytophaga–Flavobacteria–Bacteroides group and a small number of Gram-positive species. Our findings indicate that the bacterial component of sea ice at Cape Hallett is primarily heterotrophic. Cyanobacteria and Archaea were not detected using the methods and primer sets employed in this study. However, bacteria whose 16S rDNA sequences cluster with species that encode phototrophic genes were detected in our sea-ice samples. This raised the possibility that bacterial phototrophy may occur in Antarctic sea ice.
Introduction
Antarctic sea ice covers an area almost twice the size of Australia. Microrganisms inhabit the underside of the ice and the biomass they generate provide krill with an important source of nutrition to enable survival during the long, dark and unproductive polar winter (Staley Citation2002; Arrigo Citation2004; Atkinson Citation2004). The sea-ice microbial community (SIMCO) is therefore crucial to carbon flow and trophic dynamics throughout the marine food web (Garrison Citation1991; Gross Citation2005). Despite gradients in light, temperature, salinity and nutrient levels that characterise the sea-ice environment (Sullivan Citation1984), a complex community of micro-organisms flourish in microscopic brine channels within the ice matrix (Sullivan Citation1984; Garrison Citation1991).
Microbes were first detected in Antarctic sea ice in 1966 (Iizuka Citation1966), and by the 1980s, more in-depth analyses of SIMCO had begun (Sullivan Citation1984). These studies were largely based on visual phenotypic properties and were limited in their ability to determine the identification of each species. Considerable advances have been made in recent years with the introduction of culture-independent techniques such as polymerase chain reaction (PCR), restriction fragment length polymorphism (RFLP) and DNA sequencing to study sea-ice microbes (Bowman Citation1997; Staley Citation1999; Brown Citation2001; Junge 2002; Brinkmeyer 2003). These methods are normally based on the phylogeny of the highly conserved 16S and 18S ribosomal RNA genes, which allow organisms from the three domains of life to be discerned (Hugenholtz Citation2002). The primary objective of this study was to examine the SIMCO from sea-ice cores at the Cape Hallett Antarctic site using nucleic acid-based techniques and identify dominant species of prokaryotes present in this environment.
Materials and methods
Sea-ice sample collection
Sea-ice samples were collected from Cape Hallett (72° 19'S, 170° 16'E), Antarctica during November/December 2004. Sea-ice cores were drilled using an ice coring drill (Kovacs, USA) near Edisto Inlet. Multiple cores were collected from ice at three locations, 200 m apart (GPS coordinates: 72 18.970'S 170 10.865’ E; 72 18.842'S 170 11.24’ E; 72 18.689'S 170 11.705’ E) on the sea ice at the Cape Hallett site within the same 24-h period. The ice at the locations ranged in thickness from 1.25 to 1.70 m. The sea ice was first year ice with negligible snow cover (0–50 mm). There was no evidence of thawing and/or refreezing. There was a relatively strong current (0.5–1.0 m/s) that ablated and scoured the under surface of the Cape Hallett sea ice. The microbial community was most dense at the ice–water interface. Individual cores were placed directly in black tubular plastic bags at each sampling site and transported to the field camp with care to ensure the samples were not light shocked. An internal block measuring 40×40×100 mm was cut from the bottom 300 mm of ice core and melted into approximately 300 ml of 0.22-µm sterile filtered seawater in the dark. After melting of the ice core, microbial cells were concentrated using vacuum pump filtration onto 47 mm mixed-cellulose ester 0.22-µm filters (Millipore). The microbial cells were eluted from the filters by pipetting 2 ml of 0.22-µm filtered sterile seawater on to the filter and by concentrating the cells by centrifugation at approximately 5000×g. The cell pellets were stored at −80°C.
Construction of 16S rDNA clone libraries
Total genomic DNA was obtained from the sea-ice samples using a standard phenol/chloroform extraction method with proteinase K and sodium dodecyl sulphate (SDS) at a final concentration of 0.2 mg/ml and 1% (w/v), respectively, as previously described (Sambrook Citation2001). The 16S ribosomal RNA gene sequences were amplified using sets of universal 16S primers, archaea-targeted primers, and cyanobacteria-targeted primers. The first set used consisted of primers 27F and 1492R (Lane Citation1991). If no PCR product was obtained, the primers 519F/1406R (Lane Citation1991) were used. An α-proteobacteria-targeted primer set was designed by aligning up to 10 known α--proteobacterial sequences using Vector NTI version 10. Primers, AlphaF (5′-GGGTGAGTAACACGTGGGAA-3′) and AlphaR (5′-CCAACTCCCATGGTGTGACG-3′), complementary to the regions of conservation were designed using the default settings. For the Cytophaga–Flavobacteria–Bacteroides (CFB) group, the CFB-targeted primers Cf563 and Cf319a Weller 2000 were used. Samples were tested for the presence of cyanobacteria using combinations of the primers C106F, C359F, C781RA, C781RB (Nübel Citation1997) and 23S30R (Castiglioni Citation2004). Samples were tested for the presence of Archaea using combinations of the primers A340F (Vetriani Citation1999), A344F (DeLong Citation1992), A751F (Baker Citation2003), ArcF (5′-GGCGTACKGCTSAGTAACACGTGG-3′), Arc934R (Giovannoni et al. Citation1990) and A1115R (Reysenbach Citation1995). The final concentrations of reagents added to each 25-µl PCR reaction were: 1×PCR buffer containing 1.5 mM MgCl2, 0.16 µM of each dNTP, 0.4 µM of both forward and reverse primers, and 1.25 U of high-fidelity Platinum Taq polymerase (Invitrogen Ltd). PCR cycling conditions utilising the CFB targeted primers have been previously described (Kirchman Citation2003). For all other amplifications, the PCR cycling conditions consisted of an initial denaturation at 94°C for 3 min, 30 cycles of denaturation at 94°C for 45 s, annealing at 50°C, for bacterial, archaeal and cyanobacterial primers (except for the newly designed alpha primers for which the annealing temperature used was 55°C) for 1 min, extension at 72°C for 2 min, and a final extension at 72°C for 10 min. PCR products were visualised on a 1% (w/v) agarose gel containing 10 mg/ml ethidium bromide. PCR products were purified with the QIAquick purification kit (Qiagen Ltd).
PCR products were ligated into pCR2.1® (Invitrogen Ltd) and transformed into calcium-competent DH5α Escherichia coli cells using heat-shock transformation as previously described (Sambrook Citation2001). Transformants were screened by blue-white selection on Luria Broth (LB) agar containing 200 µg/ml ampicillin, 10 µg/ml isopropyl β-d-1-thiogalactopyranoside (IPTG) and 40 µg/ml 5-bromo-4-chloro-3-indolyl-b-d-galactopyranoside (X-gal). White colonies were picked and examined initially by colony PCR with M13 forward and reverse primers. The final concentrations of the PCR reagents were as described above, except that bovine serum albumin was added to a final concentration of 1 mg/ml. Colony PCR cycling conditions consisted of an initial denaturation at 94°C for 2 min, 30 cycles of denaturation at 94°C for 2 min, annealing at 60°C for 30 s, extension at 72°C for 1.5 min, followed by a final extension at 72°C for 7 min. PCR products were visualised on a 1% (w/v) agarose gel containing 10 mg/ml ethidium bromide. M13 PCR amplicons were purified using ExoSAP-IT (USB Ltd) following the manufacturer's instructions.
Restriction fragment length polymorphism (RFLP) analysis of clone libraries
Recombinant clones containing the insert were screened by RFLP analysis. A 3-µl aliquot of each M13 PCR amplicon was singly digested with Msp I and Hha I according to the manufacturer's instructions (New England Biolabs) at 37°C overnight. Digests were separated on a 3% (w/v) agarose gel containing 10 mg/ml ethidium bromide at 75 V for 1.5 h. A total of 282 16S rDNA clones derived from the three sea-ice sites were screened and clones with identical restriction patterns for each restriction enzyme were grouped together into RFLP types. A total of 89 unique RFLP types were identified and were sequenced.
DNA sequence analysis
The 16S ribosomal RNA genes from each of the unique RFLP types were sequenced at the Allan Wilson Centre Genome Sequencing Service, Massey University, New Zealand, using a capillary ABI3730 Genetic Analyzer (Applied Biosystems Inc). The 16S rRNA gene sequence data were aligned with rRNA gene sequences using evolutionary-conserved primary sequence and secondary structure (Lane Citation1991) in the Living Tree Project release 102 ARB database (Yarza et al. Citation2008) using ARB (Ludwig et al. Citation2004). The living tree project database was used as this provides high quality sequences of isolates with well defined taxonomy (Yarza et al. Citation2008). Evolutionary distances were calculated from sequence pair dissimilarities using only unambiguously sequenced positions. Phylogenetic relationships were estimated by neighbour-joining with Jukes–Cantor correction (Jukes & Cantor Citation1969) using programs contained in the Phylogeny Inference Package (PHYLIP) version 3.5 c (J. Felsenstein, University of Washington) with bootstrap (1000) analysis and a majority rule consensus tree was constructed. The 16S rRNA gene sequences determined in this study have been deposited in the EMBL database under accession numbers FJ998313 to FJ998372.
Results and discussion
This study presents a preliminary analysis of sea-ice bacteria at Cape Hallett, and provides an important first step in understanding the diversity of SIMCO in the Ross Sea region of Antarctica. Total genomic DNA was prepared from the bottom section of sea-ice cores collected at Cape Hallett. 16S rRNA gene clone libraries were generated using universal and targeted primer sets. The sequences obtained from the clone libraries have been assigned to five groups based on the phylogenetic analysis. It is important to note that clone libraries represent the genetic material that has been successfully extracted, amplified, cloned and sequenced. Libraries can be affected by factors such as differential DNA extraction, primer specificity and PCR bias.
The major class of bacteria in our 16S rDNA PCR clones detected was γ-proteobacteria and included the genera Colwellia, Marinomonas, Pseudoalteromonas and Psychrobacter with similarities ranging from 93.5% to 100% to known species within these genera ( and ). This is in agreement with earlier findings from Bowman et al. (Citation1997) and Brinkmeyer et al. (Citation2003) in which γ-proteobacteria, including Colwellia, Pseudoalteromonas and Psychrobacter, were found in sea ice isolated from the Vestfold Hills coast and from the Weddell Sea, respectively. Marinomonas has also been reported in sea-ice microbial communities (Brown et al. 2001). Other researchers have reported the predominance of γ-proteobacteria in Antarctic sea ice (Murray and Grzymski Citation2007) and seawater (Gentile et al. 2006) and also in Arctic sea ice (Junge et al. Citation2002).
Figure 1 Dendrogram (majority rule consensus tree) depicting the phylogenetic position of the Antarctic clones within the α-proteobacteria and γ-proteobacteria. Escherichia coli positions analysed, 135–1392. Total number of bases analysed, 1212. Out-group is Desulfovibrio marinus. Bootstrap values at each node that are higher than 400 out of 1000 are shown.
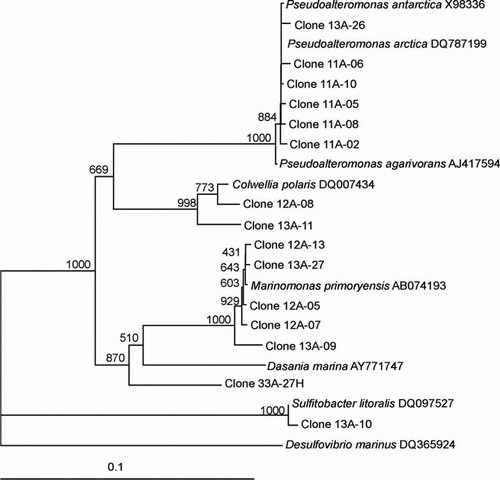
Figure 2 Dendrogram (majority rule consensus tree) depicting the phylogenetic position of the Antarctic clones within the α-proteobacteria and γ-proteobacteria. Escherichia coli positions analysed, 557–1368. Total number of bases analysed 753. Out-group is Desulfovirbio marinus. Bootstrap values at each node that are higher than 400 out of 1000 are shown.
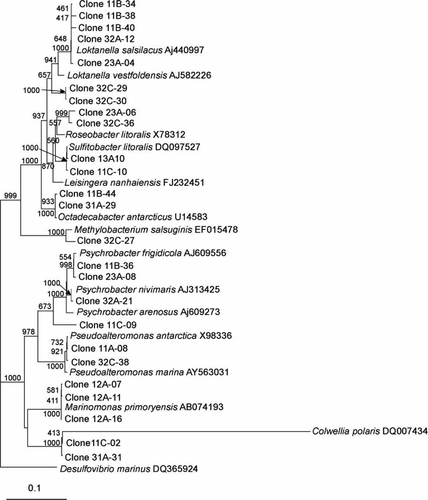
The clones that clustered within the α-proteobacteria included the genera Loktanella, Octadecabacter, Roseobacter, Sulfitobacter and Methylobacterium with similarities ranging from 96.0% to 100% (–). The presence of the genera Octadecabacter, Roseobacter and Sulfitobacter in Antarctic sea ice has been reported in other studies (Bowman et al. Citation1997; Brinkmeyer et al. Citation2003; Murray and Gryzmski Citation2007). Furthermore, Loktanella has been detected in sea ice from the Baltic Sea (Kaartokallio et al. 2008). β-Proteobacteria are known inhabitants of sea-ice surface melt pools derived from freshwater ice (Brinkmeyer Citation2004). Few β-proteobacteria were detected in our sea-ice samples, and this confirms previous reports that they are much less abundant in the interior sea-ice matrix (Gosink Citation1995; Brinkmeyer 2003, Citation2004). Few δ-proteobacteria and ϵ-proteobacteria were detected in our Antarctic sea-ice samples although they have been previously detected in Antarctic continental shelf sediments (Bowman Citation2000, 2003). Other bacteria detected included Polaribacter, Psychroserpens, Persicivirga and Winogradskyella of the Cytophaga–Flavobacteria–Bacteroides (CFB) group ( and ) and the Gram-positive genera Rhodococcus and Dietzia ().
Figure 3 Dendrogram (majority rule consensus tree) depicting the phylogenetic position of the Antarctic clones within the Rhodobactereaceae and Nocardiaceae. Escherichia coli positions analysed, 117–929. Total number of bases analysed, 851. Out-group is Deinococcus radiodurans. Bootstrap values at each node that are higher than 400 out of 1000 are shown.
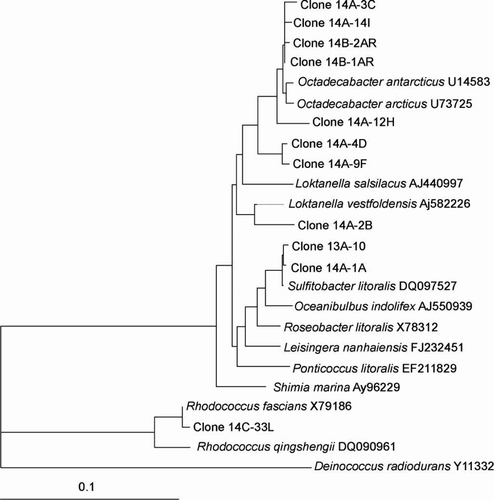
Figure 4 Dendrogram (majority rule consensus tree) depicting the phylogenetic position of the Antarctic clones within the Flavobacteriaceae and Rhodobactereaceae. Escherichia coli positions analysed, 525–1392. Total number of bases analysed, 842. Out-group is Deinococcus marimoris. Bootstrap values at each node that are higher than 400 out of 1000 are shown.
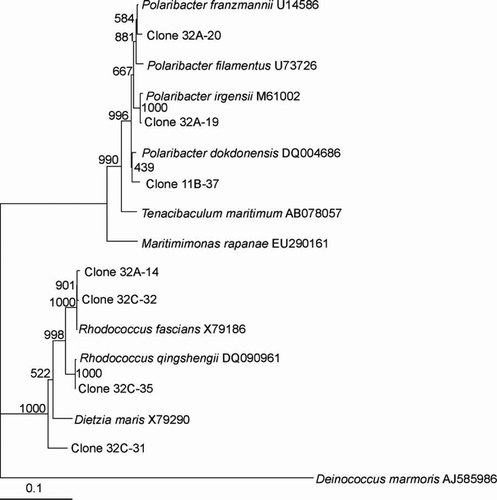
Figure 5 Dendrogram (majority rule consensus tree) depicting the phylogenetic position of the Antarctic clones within the Flavobacteriaceae. Escherichia coli positions analysed, 34–580. Total number of bases analysed, 600. Out-group is Chlorobium limicola. Bootstrap values at each node that are higher than 400 out of 1000 are shown.
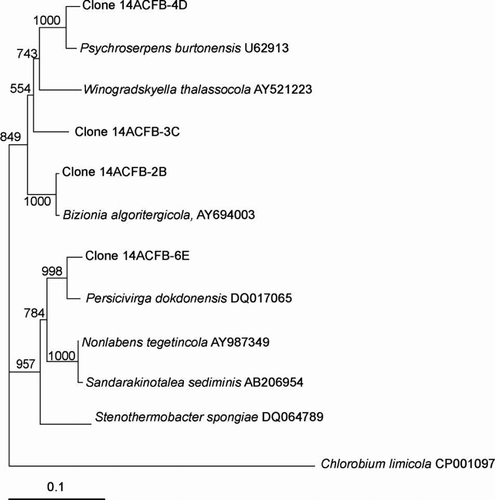
Archaea are known to be abundant in Antarctic waters and other cold marine environments (Murray & Grzymski Citation2007) and there has been evidence of Archaea residing in sea-ice habitats, although in low numbers (DeLong Citation1994; Bano Citation2004; Junge Citation2004). However, in this study no Archaea were detected in sea ice at Cape Hallett. Similarly, no cyanobacteria 16S rRNA gene sequences were detected in our samples. Synechococcus-like cyanobacteria have been detected in surface water on Arctic sea ice in the Greenland Sea and to a lesser extent within the Arctic sea ice itself (Gradinger Citation1998). In Antarctica, fresh water species have been found in lake ice (Gordon et al. Citation2000) and in extensive mats in terrestrial ponds (Vincent Citation2000). Flow cytometry experiments have also demonstrated the presence of cyanobacterial pigments, phycoerythrin and phycocyanin, in the sea ice of Ross Sea region (Andreoli Citation2000). However, their existence in Antarctic SIMCO has yet to be directly established (Mock Citation2005). It has been suggested that cyanobacteria are more likely to be associated with surface melt ponds or in coastal areas with freshwater runoff (Mock Citation2005).
Evidence for the presence of potential phototrophic bacteria was apparent with respect to the sea ice at Cape Hallett. Clones closely related to the Roseobacter clade ( and ), which have previously been shown to produce bacteriochlorophyll (Bchl) a (Shiba et al. Citation1991) and contain the associated genes required for aerobic anoxygenic photosynthesis (Pradella et al. Citation2004; Oz et al. Citation2005), were detected. Furthermore, clones closely related to photoheterotrophic bacteria were detected, including Polaribacter (), which have been reported to carry the gene for proteorhodopsin (Atamna-Ismaeel et al. Citation2008), a light driven proton pump that can harvest light to generate ATP (Wagner-Döbler Citation2006). Whether the bacteria we detected constitute phototrophs remains to be established, but the existence of phototrophic bacteria in Antarctic sea ice is supported by recent work in which bacterial proteorhodopsin gene sequences were detected in sea-ice core samples (Koh et al. Citation2010). Should further evidence establish the presence of other phototrophic bacteria in Antarctic sea ice, their role and importance to the ecology of sea-ice communities will deserve attention.
In summary, this study represents a preliminary phylogenetic analysis of the SIMCO at Cape Hallett. It is an important first step in determining the diversity of prokaryotes that inhabit sea ice from Cape Hallett to the edge of the permanent ice shelf in the Ross Sea. Extensive sampling at multiple points of latitude along the gradient is now required to ascertain the effect of latitudinal variation on SIMCO composition. Our data indicate that the bacterial component of SIMCO is composed primarily of chemoheterotrophs. These are expected to partake in the recycling of nutrients within the sea-ice ecosystem. No cyanobacteria or Archaea were detected despite the use of a range of oligonucleotide primers, indicating that these prokaryotes are either only present in very low numbers or are phylogenetically unique. In addition, our 16S rDNA data indicate the presence of bacteria in the sea ice, which are closely related to known phototrophic bacteria. The potential presence of phototrophic bacteria in Antarctic in sea ice and their role in this ecosystem requires further investigation. This study contributes to the Latitudinal Gradient Project overseen by Antarctica New Zealand. The long-term goal of the project is to determine how microbial ecosystem structure and function change over a latitudinal gradient, from Cape Hallett to the edge of the permanent ice shelf in the Ross Sea, under the Latitudinal Gradient Project (LGP) operated by Antarctica New Zealand. Further examination of the SIMCO along the latitudinal gradient on the Victoria Land Coast is being conducted to determine how microbial ecosystem structure and function change with respect to latitude.
Acknowledgements
We gratefully acknowledge the support of Antarctica New Zealand, the Foundation for Research, Science and Technology contracts VICX0219 and VICX0706, and the University Research Fund, Victoria University of Wellington, New Zealand.
References
- Andreoli , C , Moro , I , LaRocca , N , Dalle Valle , L , Masiero , L , Rascio , N and Dalla Vecchia , F . 2000 . Ecological, physiological and biomolecular surveys on microalgae from Ross Sea (Antarctica) . Italian Journal of Zoology , 67 : 147 – 156 .
- Arrigo , KR and Thomas , DN . 2004 . Large scale importance of sea ice biology in the Southern Ocean . Antarctic Science , 16 : 471 – 486 .
- Atamna-Ismaeel , N , Sabehi , G , Sharon , I , Witzel , KP , Labrenz , M , Jurgens , K , Barkay , T , Stomp , M , Huisman , J and Beja , O . 2008 . Widespread distribution of proteorhodopsins in freshwater and brackish ecosystems . ISME Journal , 2 : 656 – 62 .
- Atkinson , A , Siegel , V , Pakhomov , E and Rothery , P. 2004 . Long term decline in krill stock and increase in salps within the Southern Ocean . Nature , 432 : 100 – 103 .
- Baker , GC , Smith , JJ and Cowan , DA. 2003 . Review and re-analysis of domain-specific 16S primers . Journal of Microbiological Methods , 55 : 541 – 555 .
- Bano , N , Ruffin , S , Ransom , B and Hollibaugh , JT . 2004 . Phylogenetic composition of Arctic Ocean Archaeal assemblages and comparison with Antarctic assemblages . Applied and Environmental Microbiology , 70 : 781 – 789 .
- Bowman , JP and McCuaig , RD . 2003 . Biodiversity, community structural shifts, and biogeography of prokaryotes within Antarctic continental shelf sediment . Applied and Environmental Microbiology , 69 : 2463 – 2483 .
- Bowman , JP , McCammon , SA , Brown , MV , Nichols , DS and McEeekin , TA . 1997 . Diversity and association of psychrophilic bacteria in Antarctic sea ice . Applied and Environmental Microbiology , 63 : 3068 – 3078 .
- Bowman , JP , Rea , SM , McCammon , SA and McMeekin , TA. 2000 . Diversity and community structure within anoxic sediment from marine salinity meromictic lakes and a coastal meromictic marine basin, Vestfold Hills, Eastern Antarctica . Environmental Microbiology , 2 : 227 – 237 .
- Brinkmeyer , R , Glöckner , FO , Helmke , E and Amann , R. 2004 . Predominance of β-proteobacteria in summer melt pools on Arctic pack ice . Limnology and Oceanography , 49 : 1013 – 1021 .
- Brinkmeyer , R , Knittle , K , Jurgens , J , Weyland , H , Amann , R and Helmke , E . 2003 . Diversity and structure of bacterial communities in Arctic versus Antarctic pack ice . Applied and Environmental Microbiology , 69 : 6610 – 661 .
- Brown , MV and Bowman , JP . 2001 . A molecular phylogenetic survey of sea-ice microbial communities (SIMCO) . FEMS Microbiology Ecology , 35 : 267 – 275 .
- Castiglioni , B , Rizzi , E , Frosini , A , Sivonen , K , Rajaniemi , P , Rantala , A , Mugnai , MA , Ventura , S , Wilmotte , A , Boutte , C , Grubisic , S , Balthasart , P , Consolandi , C , Bordoni , R , Mezzelani , A , Battaglia , C and De Bellis , G . 2004 . Development of a universal microarray based on the ligation detection reaction and 16S rRNA gene polymorphism to target diversity of Cyanobacteria . Applied and Environmental Microbiology , 70 : 7161 – 7172 .
- DeLong , EF . 1992 . Archaea in coastal marine environments . Proceedings of the National Academy of Sciences of the United States of America , 89 : 5685 – 5689 .
- DeLong , EF , Ying Wu , K , Prezelin , BB and Jovine , RVM . 1994 . High abundance of Archaea in Antarctic marine picoplankton . Nature , 371 : 695 – 697 .
- Garrison , DL . 1991 . Antarctic sea ice biota . American Zoologist , 31 : 17 – 33 .
- Giovannoni , SJ , Britschgi , TB , Moyer , CL and Field , KG . 1990 . Genetic diversity in Sargasso Sea bacterioplankton . Nature , 345 : 60 – 63 .
- Gordon , DA , Priscu , J and Giovannoni , S . 2000 . Origin and phylogeny of microbes living in permanent Antarctic lake ice . Microbial Ecology , 39 : 197 – 202 .
- Gosink , JJ and Staley , JT . 1995 . Biodiversity of gas vacuolate bacteria from Antarctic sea ice and water . Applied and Environmental Microbiology , 61 : 3486 – 3489 .
- Gradinger , R and Ikavalko , J . 1998 . Organism incorporation into newly forming Arctic sea ice in the Greenland Sea . Journal of Plankton Research , 20 : 871 – 886 .
- Gross , L . 2005 . As the Antarctic ice pack recedes, a fragile ecosystem hangs in the balance . PLoS Biology , 3 : 557 – 561 .
- Hugenholtz , P . 2002 . Exploring prokaryotic diversity in the genomic era . Genome Biology , 3 : 1 – 8 .
- Iizuka , H , Tanabe , I and Meguro , H . 1966 . Microorganisms in plankton-ice of the Antarctic Ocean . Journal of General and Applied Microbiology , 12 : 101 – 102 .
- Jukes , TH and Cantor , CR. 1969 . “ Evolution of protein molecules ” . In Mammalian protein metabolism , Edited by: Munro , H . 21 – 132 . New York : Academic Press .
- Junge , K , Eicken , H and Deming , JW . 2004 . Bacterial activity at −2 to −20°C in Arctic wintertime sea ice . Applied and Environmental Microbiology , 70 : 550 – 577 .
- Junge , K , Imhoff , F , Staley , T and Deming , JW. 2002 . Phylogenetic diversity of numerically important Arctic sea-ice bacteria cultured at subzero temperature . Microbial Ecology , 43 : 315 – 328 .
- Kirchman , DL , Yu , L and Cottrell , MT. 2003 . Diversity and abundance of uncultured Cytophaga-like bacteria in the Delaware Estuary . Applied and Environmental Microbiology , 69 : 6587 – 6596 .
- Koh , EY , Atamna-Ismaeel , N , Martin , A , Cowie , RO , Beja , O , Davy , SK , Maas , EW and Ryan , KG . 2010 . Proteorhodopsin-bearing bacteria in Antarctic sea ice . Applied and Environmental Microbiology , 76 : 5918 – 5925 .
- Lane , DJ . 1991 . “ 16S/23S rRNA Sequencing ” . In Nucleic acid techniques in bacterial systematics , Edited by: Stackebrandt , E and Goodfellow , M . 115 – 175 . Chichester : John Wiley & Sons Ltd .
- Ludwig , W , Strunk , O , Westram , R , Richter , L , Meier , H , Yadhukumar , Buchner A , Lai , T , Steppi , S Jobb , G . 2004 . ARB: a software environment for sequence data . Nucleic Acids Research , 32 : 1363 – 1371 .
- Mock , T and Thomas , DN . 2005 . Recent advances in sea-ice microbiology . Environmental Microbiology , 7 : 605 – 619 .
- Murray , AE and Grzymski , JJ . 2007 . Diversity and genomics of Antarctic marine micro-organisms . Philosophical Transactions of the Royal Society B: Biological Sciences , 362 : 2259 – 2271 .
- Nübel , U , Garcia-Oichel , F and Muyzer , G . 1997 . PCR primers to amplify 16S rRNA genes from Cyanobacteria . Applied and Environmental Microbiology , 63 : 3327 – 3332 .
- Oz , A , Sabehi , G , Koblizek , M , Massana , R and Beja , O . 2005 . Roseobacter-like bacteria in red and mediterranean sea aerobic anoxygenic photosynthetic populations . Applied and Environmental Microbiology , 71 : 344 – 353 .
- Pradella , S , Allgaier , M , Hoch , C , Pauker , O , Stackebrandt , E and Wagner-Dobler , I . 2004 . Genome organization and localization of the pufLM genes of the photosynthesis reaction center in phylogenetically diverse marine Alphaproteobacteria . Applied and Environmental Microbiology , 70 : 3360 – 3369 .
- Reysenbach AL , Pace NR 1995 . Archaea: a laboratory manual—thermophiles . In: Robb FT , Place AR . New York , Cold Spring Harbour Press . Pp. 101 – 107 .
- Sambrook , J and Russell , D.W. 2001 . Molecular cloning: a laboratory manual , 3rd ed , New York : Cold Spring Harbor Press Ltd .
- Shiba , T , Shioi , Y , Takamiya , KI , Sutton , DC and Wilkinson , CR . 1991 . Distribution and physiology of aerobic bacteria containing bacteriochlorophyll a on the East and West Coasts of Australia . Applied and Environmental Microbiology , 57 : 295 – 300 .
- Staley , JT and Gosink , JJ . 1999 . Poles apart: biodiversity and biogeography of sea ice bacteria . Annual Review of Microbiology , 53 : 189 – 206 .
- Staley , JT and Reysenbach , AL . 2002 . Biodiversity of microbiological life , New York : Wiley-Liss .
- Sullivan , CW and Palmisano , AC . 1984 . Sea ice microbial communities: distribution, abundance, and diversity of Ice bacteria in McMurdo Sound, Antarctica, in 1980 . Applied and Environmental Microbiology , 47 : 788 – 795 .
- Vetriani , C , Jannasch , HW , MacGregor , BJ , Stahl , DA and Reysenbach , AL . 1999 . Population structure and phylogenetic characterization of marine benthic Archaea in deep-sea sediments . Applied and Environmental Microbiology , 65 : 4375 – 4384 .
- Vincent , WF , Bowman , JP , Rankin , LM and McMeekin , TA . 2000 . “ Phylogenetic diversity of picocyanobacteria in Arctic and Antarctic ecosystems ” . In Microbial biosystems: new frontiers. Proceedings of the 8th International Symposium on Microbial Ecology. Atlantic Canada Society for Microbial Ecology, Halifax, Canada Edited by: Bell , CR , Brylinsky , M and Johnson-Green , P . 317 – 322 .
- Wagner-Döbler , I and Biebl , H . 2006 . Environmental biology of the marine Roseobacter lineage . Annual Review of Microbiology , 60 : 255 – 280 .
- Yarza , P , Richter , M , Peplies , J , Euzeby , J , Amann , R , Schleifer , KH , Ludwig , W , Glockner , FO and Rossello-Mora , R . 2008 . The All-Species Living Tree project: a 16S rRNA-based phylogenetic tree of all sequenced type strains . Systematic and Applied Microbiology , 31 : 241 – 250 .