Abstract
Progress towards development of a river plume ecosystem basis for management of coastal resources requires a thorough understanding of the nature and spatial extent of terrestrial influences on receiving water and seabed characteristics. As a case study, we estimate suspended sediment (SS) loading rates from the Motueka River catchment into Tasman Bay, New Zealand and describe benthic characteristics along a series of transects encompassing the coastal river plume. A mineral-rich geological formation in the headwaters of the river was identified as a storm-generated source of highly elevated concentrations of nickel and chromium in river margin sediments, and coastal sediments extending >5 km offshore. A major storm in 2005, focused in the upper catchment, resulted in an estimated SS discharge of 161,000 tonnes into the Bay. Spatial gradients of a suite of sediment trace metal signatures, organic content and infauna community structure were used to define a river plume depositional footprint of c. 180 km2. We suggest that the boundaries of the Motueka catchment be redefined to include this area in order to facilitate engagement of marine stakeholders in management decisions that may affect coastal biological resources.
Introduction
New Zealand's mountainous topography and abundant rainfall give rise to a coastal perimeter lined with a multitude of river outwelling plumes (). Historical changes and intensification of catchment land uses (e.g. the clearance of native vegetation for agricultural and residential development), in conjunction with the interactive and potentially synergistic effects of climate variability, have the potential to amplify the frequency and duration of buoyant river outwelling plumes carrying nutrients, suspended sediment (SS) and contaminants into coastal environments (Kaushal et al. Citation2010). The consequences, in terms of re-organisation of aquatic ecosystems can be substantial and long lasting (Carpenter & Brock Citation2006). Although this is a global issue, the rapid growth of marine aquaculture in New Zealand has accentuated the need for development of a knowledge base suitable to facilitate management of coastal ecosystems (and the resources they contain) in the context of the effects of land runoff from adjacent catchments on both the water column and benthic environments. This can only be realistically achieved through an integrative approach involving terrestrial, freshwater and coastal marine investigations (Bowden et al. Citation2004; Fenemor et al. Citation2008). In New Zealand, relatively little is known about the ecological influence of small- and medium-sized river outwelling plumes in situations where they may impinge directly on aquaculture growing areas () and/or offshore fish and shellfish resources.
Figure 1 Location of river outwelling plumes and major aquaculture development areas along the New Zealand coastline.
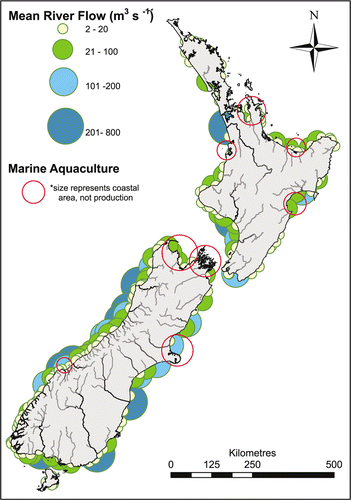
The need for such a knowledge base is particularly relevant for Tasman Bay, located at the northern end of the South Island of New Zealand (). Tasman Bay is a large (c. 1500 km2), relatively shallow (mainly within the 30-m depth contour) embayment with a primarily soft-sediment seabed habitat. The Bay is recognised as an important regional and national resource with a variety of amenity and economic values (MacKenzie Citation2004). Currently, it supports a number of commercially and recreationally important fin- and shellfish species. Along with Golden Bay to the northwest and the Marlborough Sounds to the east, Tasman Bay comprises the area of New Zealand's major scallop (Pecten novaezelandiae) fishery although, because of depleted stocks, Tasman Bay has not contributed to commercial scallop harvests since 2005 (New Zealand Ministry of Fisheries Citation2008). The Motueka catchment, covering a land area of 2180 km2, contributes about 62% of the freshwater inflow to the Bay (Basher Citation2003; Gillespie et al. Citation2011). Three aquaculture management areas (AMAs) have been designated in western Tasman Bay (). The AMAs, covering a total of 4190 ha, consist of three separate zones for the longline culture of GreenshellTM mussel (Perna canaliculus) and/or the collection of both mussel and scallop spat for commercial use. The central zone, located between approximately 5 and 8 km offshore from the mouth of the Motueka River, is presently being used for the staged development of mussel farming.
Figure 2 Sampling locations and aquaculture management areas in Tasman Bay. Site designations refer to geographic locations and mean water depth. Stippled polygons represent aquaculture management areas.
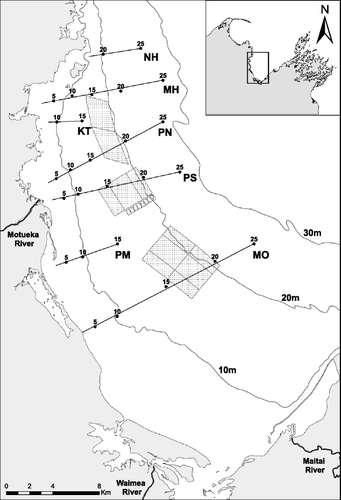
Forrest et al. (Citation2007) reported elevated concentrations of nickel, chromium and copper in marine sediments within an area of Tasman Bay affected by the outwelling plume from the Motueka River. These sediments were thought to have originated from a large exposed ultramafic ophiolite mineral belt in the upper Motueka River catchment known as the Red Hills (Challis Citation1965; Basher Citation2003).
Progressing from findings of Forrest et al. (Citation2007), we estimate the SS loading rates into Tasman Bay and provide further evidence of the location of the catchment source of elevated concentrations of trace metals in river margin and bay sediments. We also investigate the spatial distribution patterns of a suite of trace metal concentrations, organic matter content and infauna community structure in sediments along a series of transects within and beyond the region of Tasman Bay thought to be within the sedimentation footprint of the river plume as predicted by Tuckey et al. (Citation2006) and Forrest et al. (Citation2007).
Methods
Suspended sediment yields
In order to determine SS discharge rates into Tasman Bay and the relative contributions of major subcatchments, continuous SS monitoring was undertaken at four sites in the Motueka catchment () from December 2002 to November 2009 as described by Hicks & Basher (Citation2008). The Motueka at Woodmans Bend site is about 7 km inland from the coast and characterises the discharge of sediment to the Bay. Three other sites characterise the input of sediment from major sub-catchments: Motupiko River at Christie's Bridge, Wangapeka River at Walter Peak and the Motueka headwaters at Motueka Gorge, which drains from the Red Hills mineral belt. During the monitoring period, one particularly large flood event in March 2005, with an annual recurrence interval of about 50 years, had a major effect on the SS yield at the Motupiko, Motueka Gorge and Woodmans Bend sites (Hicks & Basher Citation2008).
Figure 3 River margin sediment sampling locations (squares = main stem of the Motueka River, dots = tributaries) and suspended sediment sampling locations (triangles); u/s refers to upstream.
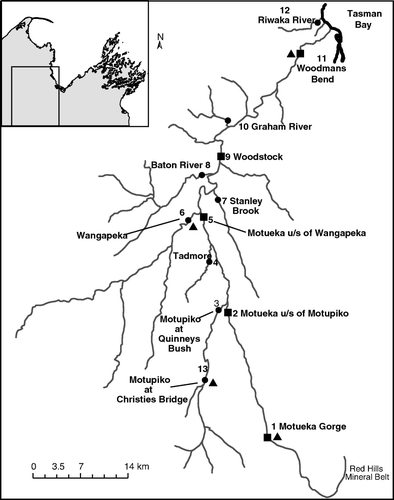
Turbidity, as a surrogate for SS concentration (SSC), along with discharge (Q) were continuously monitored at each site (Basher et al. Citation2011). Water samples were collected during most flood events using automatic samplers and analysed for SSC to enable determination of calibration relationships between turbidity and SSC at the sensor positions. This data was used to compile a continuous record of SSC. SS yields (SY) during storm events were determined by integrating the SSC and Q series records. Relationships between event SY and peak flow (Q p) were determined and used to estimate SY in unmeasured events (caused by turbidity probe malfunction) over the complete flow record at all four sites.
The field data collection and analytical procedures are described in detail in Wild et al. (Citation2006) and Hicks & Basher (Citation2008). Long-term sediment yields were also calculated based on both sediment rating relations (combining long-term discharge records with SSC–Q relationships) and the event–load ratings (combining the short term event SY–Q p relationship with long-term discharge records). Because the discharge record for the Motueka at Woodmans Bend site commenced recently (2001), a synthetic flow record was derived from the longer-term site on the Motueka River at Woodstock with which the Woodmans Bend site is well correlated (Basher et al. Citation2011).
River bed and sea bed sampling
River margin sediments were collected on 29 June 2005, approximately 3 months after the main flood event, from five stations along the main stem of the Motueka River, six stations along tributaries of the Motueka River and one station from a neighbouring catchment along the lower Riwaka River (). River flow at the time of sampling was relatively low. Four to five samples of the top c. 20-mm layer of exposed sediment at the river's edge were collected with an acid-washed plastic scoop along a 100-m stretch at each station and mixed into one composite sample for subsequent grain size and trace metal analyses.
Benthic samples were collected from Tasman Bay (3–10 May 2006) at approximately 5-m depth intervals from 5–25 m along four transects extending from near shore out to 7–8 km offshore (). Two transects (PN and PS) extended from the mouth of the Motueka River through the river plume depositional area as indicated by Forrest et al. (Citation2007), with further transects north (MH) and south (MO) of the river mouth designed to theoretically sit outside of the plume's primary depositional footprint. Three additional transects (NH, KT and PM) were sampled during July 2007 to provide a more thorough coverage of the key signatures of Ni and Cr within the study area. At each sampling station, five replicate grab samples (0.1 m2 surface area) were collected from within a 50×50 m2 area using a modified van Veen grab sampler. The upper 25-mm layer was subsampled with acid-washed plastic scoops for grain size, organic matter and trace metal analyses. An additional subsample was collected for infauna analyses by pushing a Perspex tube (0.013 m2 internal diameter) c. 150 mm into the sediment. The infauna sample was washed through a 0.5-mm mesh and the organisms retained were preserved in a 70% ethanol, 4% glyoxal solution.
Grain size and organic matter
Sediment particle size fractions, gravel (>2 mm), sand (2 mm to 0.063 mm) and silt/clay (<0.063 mm), were separated by wet sieving and analysed gravimetrically after oven drying at 105 °C. Bulk organic matter content was measured as % ash-free dry weight after drying at 105 °C then ashing at 550 °C for 6 h (Luczak et al. Citation1997, modified).
Trace metals
A suite of trace metals comprising nickel (Ni), chromium (Cr), copper (Cu), aluminium (Al), strontium (Sr), vanadium (V), barium (Ba) and lead (Pb) was analysed in acid digests of the whole sediments using inductively coupled plasma mass spectrometry (ICP-MS). These metals were selected to cover a broad range of those thought potentially to be sourced from the catchment. Sediments from river margins and Bay transects NH, KT and PM were analysed only for Cr and Ni by inductively coupled plasma optical emission spectrometry (ICP-OES) according to USEPA 200.2 mod. (APHA Citation1998). All analyses were carried out following a hot/strong acid digest using BDH Aristar brand, AR grade acids in a mixture of 50% HNO3 and 25% HCl according to a modified version of the USEPA 200.2 method (APHA Citation1998). This digestion technique has been shown to dissociate trace elements complexed in precipitated hydroxides, carbonates, sulphides, oxides and organic materials. Surface metals, but not those which are part of the lattice structure of the sediment (interstitially bound elements), will be desorbed from clay mineral particulates. This process is considered a moderately weak acid leaching and has been used in previous studies to examine the ‘bio-available’ trace metal content (Loring & Rantala Citation1992).
The precision of the ICP-MS analyses and presence of contamination was determined by running duplicates of every fifth sample as well as using standard additions, method blanks and comparison with the analysis of a certified reference material (PACS-1 and BCSS-1, Marine Sediment Reference Materials for Trace Elements and Other Constituents, National Research Council, Canada). Standard addition calibration standards were measured several times during a sampling run as unknowns to provide an indication of any instrumental drift. In all cases, instrumental drift was found to be less than 3%, which was considered acceptable (Loring & Rantala Citation1992; Angelidis & Aloupi Citation2000).
Trace metal concentrations were expressed either as mg/kg of whole dry sediment or normalised to 100% silt/clay based on the dry weight of the <0.063-mm size fraction.
Infauna
Infauna were sorted, identified to the lowest practical taxonomic level and counted with the aid of a binocular microscope. The communities were evaluated according to taxa richness, abundance and diversity, i.e. Shannon–Weiner diversity index (H’, Shannon Citation1948). A non-metric multi-dimensional scaling (MDS) ordination procedure based on Bray–Curtis (Bray & Curtis Citation1957) similarities (PRIMER v. 6) was used to describe spatial patterns in taxa distribution and dominance between sites, and evaluate variations in the community structure of benthic infauna with changing influences of the river plume. A fourth-root transformation was applied to the infauna data during this process to down-weigh the influence of the most dominant taxa (Clarke & Warwick Citation1994). The major taxa contributing to similarities between groups were identified using analysis of similarities (SIMPER; Clarke & Warwick Citation1994). The BIOENV procedure in PRIMER v. 6 was applied to elucidate which measured environmental variables (i.e. depth, sediment grain size distribution, metals and organic matter concentrations) most closely correlated with the changes in infauna community composition. The level of correlation was determined according to the Spearman rank correlation coefficient, ρ (Clarke & Ainsworth Citation1993). Sediment data were log-transformed to meet the assumptions of normality, and all data were standardised (by subtracting the mean and dividing by the standard deviation) prior to analysis. By processing the data in this way, each value was expressed on a common, dimensionless measurement scale (Clarke & Gorley Citation2006).
Spatial delineation
The analytical data were averaged for each sampling station and interpolated into graduated-colour contour maps using the kriging (with default settings) technique in SURFER v.7 surface mapping software to determine the spatial pattern of several sediment characteristics.
The associated raster files were imported into a geographic information system (ArcGIS v.9.2) and rectified into a New Zealand Map Grid (1949) coordinate system. Each raster dataset was then reclassified into 10, equal-interval categories in order to compare the spatial patterns of key terrestrially influenced sediment characteristics. The different ranges of data were converted into an ordinal scale between 1 and 10, representing the smallest and largest concentration or diversity, to assign all datasets equal weight in further spatial analyses. Simple summary calculations were carried out between the reclassified raster datasets using the ‘Raster Calculator’ in ArcGIS extension, Spatial Analyst. Composites of reclassified datasets were averaged by the number of raster datasets used.
Results
Suspended sediment river discharge
The monthly patterns of SS discharge from the four Motueka catchment sites () were strongly influenced by a small number of flood events. During the entire 7-year monitoring period, the sediment loads at Motueka Gorge and Motupiko were dominated by the March 2005 flood event with 49% and 69% respectively carried during this storm. The March 2005 storm contributed less of the total load at Woodmans Bend (15%) but it was still a highly significant event. Approximately 161,000 t of sediment was discharged into Tasman Bay during this single event, with at least 65% coming from the Red Hills. The total amount of sediment measured from the three tributary sites exceeded that discharged to the coast by about 18%, implying a proportion of the suspended load was re-deposited before being exported into Tasman Bay. However, the difference is within the uncertainty on the estimates of yield from the Motueka Gorge and Motupiko sites during the March 2005 storm for which there is a lack of turbidity data and the SY is calculated from the Q–SSC relationship. Nevertheless, the majority of sediment deposited in Tasman Bay during this flood was clearly derived from the Red Hills region of exposed ultramafic rock.
Figure 4 Monthly suspended sediment load at four sites in the Motueka catchment. See for site locations.
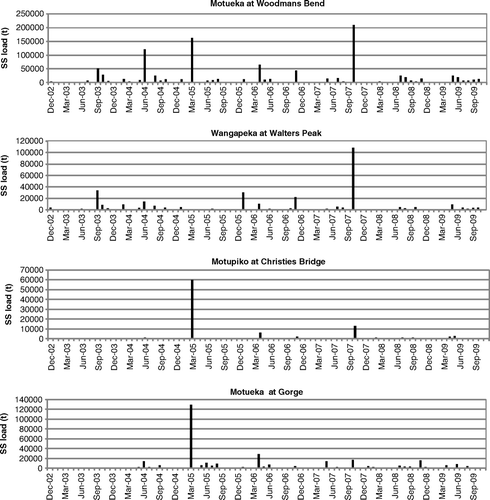
The SS data also shows that the average yields measured at three of the four sites during the 7-year monitoring period were lower than average yields over the period 1990–2009. The average annual sediment load delivered to the coast from the Red Hills (Motueka Gorge site) over either the 7-year or 20-year period () is far lower than measured during the March 2005 flood.
Table 1 Specific sediment yield and sediment load estimates for four sampling sites in the Motueka catchment.
The long-term average sediment load (1969–2010) discharged to the coast is about twice that measured between December 2002 and November 2009. This difference results from a lower frequency of floods between 2002 and 2009 compared with the long-term discharge record. During the monitoring period about 32% of the sediment discharged to the coast came from the Red Hills (from 8% of the catchment area), and the long-term average contribution was similar (31%). These data show that the Red Hills provides a high proportion of sediment delivered to the coast relative to the area of the catchment, particularly during large storm events when the contribution can be >65%.
Grain size and organic matter
The majority of sediments sampled in Tasman Bay were composed predominantly of the silt/clay or mud fraction (i.e. >80%), but four distinctly different areas were found () where the <0.063-mm fraction comprised <40% of the total. One of these was near the mouth of the Motueka River (site PS5) with sediments containing 40–45% sand. A second was offshore to the north of the river mouth (site MH25) where sediments contained significant amounts of gravel sized particles; predominantly broken shell and bryozoan fragments. Two sites south of the river mouth (PM5 and PM15) were composed primarily of sand and gravel, respectively.
Figure 5 Particle size, gravel (>2 mm), sand (2–0.063 mm) and muds/fines (<0.063 mm), of Tasman Bay sediments.
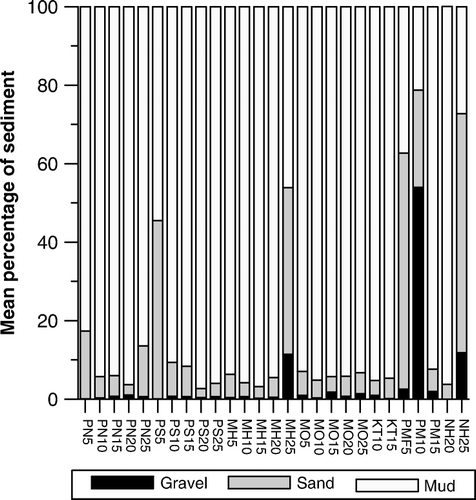
Total organic content (expressed as % ash free dry weight) was highest in sediments sampled from water depths of 10–20 m to the north of the river mouth and peaked at c. 7% at station PN15 on the northern transect extending off the river mouth (). Organic matter levels in sediments collected close to the river mouth were relatively low (c. 4%) compared with the sediments from surrounding regions ().
Trace metals
Unusually high concentrations of Ni and Cr were observed in river margin sediments from the main stem of the Motueka River (). Highest concentrations (i.e. 1200 and 330 mg/kg, for Ni and Cr, respectively) in whole (i.e. non-fractionated) sediments were found at the upper catchment Motueka Gorge site. Ni and Cr concentrations in sediments bordering tributary streams were not unusually elevated. Although main stem Motueka Ni and Cr concentrations decreased progressively downstream as they were mixed with sediments from other parts of the catchment, they were still noticeably elevated at the lower reaches of the river.
Figure 7 Ni and Cr concentrations in river margin sediments from stations along the main stem Motueka River (grey bars) and tributaries (white bars). ISQG refers to interim sediment quality guidelines. See for site locations 1–12.
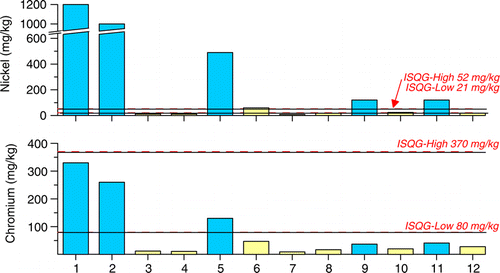
High concentrations of Ni, Cr, Cu and Ba (normalised to 100% silt/clay) were also observed in coastal sediments in the vicinity of the Motueka River mouth (A–D) with concentrations decreasing with distance away from the river mouth out to about 9 km offshore. An additional peak in the concentrations of some metals (i.e. Ba, V, Al and Pb) was observed to the northeast of the river mouth (D–G). Highest Sr concentrations occurred at station MH25 offshore to the northeast of the river mouth within a region of higher gravel-sized particles containing shell and bryozoan debris. Concentrations of Sr (H) were not elevated in the vicinity of the river mouth.
Infauna
Eighty-seven macrofaunal taxa were recorded within the study area with the total number of taxa per core ranging from four to 29 (A). There were no large differences in the Shannon–Weiner diversity index between sites (B), and, in general, richness reflected abundance patterns (C). Sites with higher abundances generally had higher numbers of taxa. For example, the highest average number of taxa was found at MH20 and MH25, with averages of 17 and 20 taxa per core respectively, and these sites also had relatively high abundances with averages of 74 and 103 organisms per core respectively (C). However, the communities nearest the river mouth (PN05, PS05 and PS10) also had high abundances but richness values were not elevated relative to the other sites. These communities were dominated by a few small, disturbance-tolerant taxa including elevated abundances of the capitellid worm, Heteromastus filiformis, and the small, non-indigenous Asian bivalve, Theora lubrica (D). A general trend for transects PN, PS and MO was that abundances at deeper sites were reduced; however the opposite of this was evident for the MH transect, with higher abundances at the 20- and 25-m sites.
Figure 9 Spatial variation in: A, taxa richness; B, Shannon–Weiner diversity values; C, total abundance; and D, abundance of Heteromastus filiformis (grey bars) and Theora lubrica (white bars). Data are averages + standard error (n=5 cores).
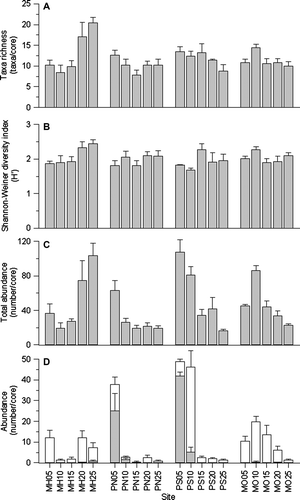
Three groups were distinguished through the MDS analysis; Group B comprised the two sites nearest the river mouth (PN05 and PS05), Group C comprised MH20, MH25, PS10 and MO10, and Group A comprised the rest of the sites (A). The abundance of H. filiformis contributed the most in distinguishing the three groups. Communities in Group A (containing the most sites) were characterised by a variety of polychaetes (lumbrinerids, cirratulids, Aglaophamus sp. and Cossura consimilis) and the bivalve T. lubrica. They had fewer H. filiformis and Prionospio sp. polychaetes than Groups C and B, and fewer Nucula nitidula (bivalve), T. lubrica and cumaceans than Group C. There was a strong contribution of disturbance-tolerant taxa in distinguishing Group B nearest the river mouth. These communities were dominated by H. filiformis, and T. lubrica, Prionospio sp., Agalophamus sp. and an unidentified species of amphipod also contributed to their similarity. Ostracods (present in both of the other groups) were absent. Group C communities had abundant T. lubrica, and polychaetes (C. consimilis and cirratulids) were common.
Figure 10 Multi-dimensional scaling (MDS) ordination showing: A, similarity of sites based on their infaunal taxa composition (2D stress = 0.18, sites circled were ≥59% similar), and B–D, bubble plots indicating relative values for the most correlated (Spearman ρ-values) explanatory variables.
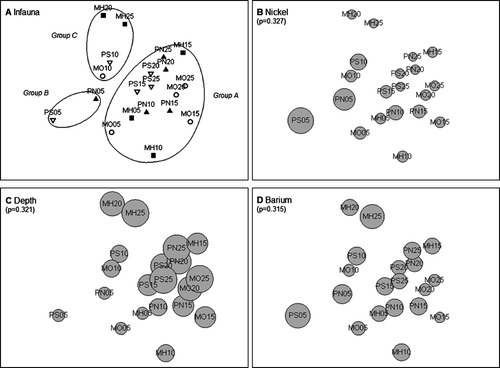
Pairwise contrasts of environmental data showed that Ni concentration was highly correlated with Cr concentration (r=0.98). Bubble plots in B–D show the relative levels of the variables identified through BioEnv analysis with respect to their correlation with the ordination pattern in A. The size of each bubble is proportional to the respective variable, e.g. for depth, a smaller bubble indicates shallower sites. A combination of depth, Ni concentration and Ba concentration had the highest correlation value (Spearman ρ-value 0.473), and when the model was limited to single variables, Ni, depth and Ba had correlations of 0.327, 0.321 and 0.315, respectively.
Spatial delineation
A large amount of spatial co-linearity was present between the different trace metal concentrations, as demonstrated by the individual metal plots (A–G). To remove the spatial effect of this redundancy on the mean composite, correlation coefficients were calculated for all possible metal combinations. This enabled the use of Ni and Ba as spatial proxies for all other metals, with the exception of Pb. The spatial distribution patterns for these two proxy metals were summarised as a single composite (). A second composite was created by combining the spatial patterns for organic matter and Pb (). This was considered appropriate because sediment Pb concentrations followed a spatial pattern more closely associated with organic matter concentrations. The resulting composites represent effective indicators for the overall spatial extent of influence of the Motueka River plume on the seabed environment. Although a third descriptor of plume influence, the Shannon–Weiner diversity index, was considered in order to incorporate biological effects of total infauna abundance and species richness, this was not included in the figure because interpretation was unclear and the overall plume influenced region was not noticeably extended beyond the patterns shown in .
Discussion
Ideally, coastal management should consider the implications of both water column and benthic gradients arising from river outwelling plumes. However, it can be problematic to define management boundaries based on water column characteristics that are extremely variable and mobile. Such boundaries can of course be roughly predicted according to river flow, wind and hydrodynamic characteristics (Tuckey et al. Citation2006) and can be particularly important with regard to management of shellfish resources (Cornelisen et al. Citation2011; Gillespie et al. Citation2011). The present investigation focuses on benthic gradients that provide a more stable criterion as a basis for management considerations.
Sediment metals
Comparison of trace metal concentrations of river margin sediments from the Motueka River with those from tributaries indicated the source of elevated sediment Ni and Cr levels to be a natural geological formation called the Red Hills that is located within the catchment area of the upper main stem Motueka River. The very high Ni levels measured in sediments from the Motueka Gorge site approach those reported for sediments in other freshwater environments that are clearly polluted from anthropogenic activities. For example, Ni concentrations ranging from 1500 to 2100 mg/kg dry weight were reported for surface sediments in Clearwater Lake, Ontario, Canada, which receives significant atmospheric input from a nearby Ni smelter (Carignan & Nriagu Citation1985). Because the whole-sediment concentrations of Ni and Cr observed in parts of the catchment were thought to be particularly high for a relatively pristine environment (i.e. unaffected by industrial wastes), they were compared with ANZECC (Citation2000) Interim Sediment Quality Guideline (ISQG) values to illustrate their potential contaminant status. These guidelines can be applied to both freshwater and marine sediments. The ISQG High threshold level is reported to equate to ‘probable’ biological effects, whereas the ISQG Low threshold level is reported to equate to ‘possible’ biological effects (ANZECC 2000). The Ni concentration of whole-sediment from the uppermost Motueka River site, as shown in , was 23 times greater than the ISQG High threshold, whereas the Cr concentration was four times greater than the ISQG Low threshold. Although the different sediment particle size fractions were not analysed separately, the metals were assumed to be largely associated with the fine particulates (Koppelman & Dillard Citation1975). This assumption is supported by observations of up to 1000 and 300 mg/kg of Ni and Cr in SS collected from the lower Motueka River during a moderate rainfall event (c. 240 m3/s on 25 August 2005; Gillespie, unpubl.). If we express the metals results solely with respect to the <0.063 size fraction (i.e. normalised to 100% silt/clay), Ni and Cr concentrations of 13,800 and 3800 mg/kg are achieved indicating that variation in sediment particle size distribution was likely a major contributing factor. Expression of the results in this manner obviously overestimates actual environmental Ni and Cr concentrations, but it raises questions concerning the potential for biological effects within accumulated pockets of fine-grained sediments that requires further investigation.
Concentrations of Ni in Tasman Bay sediments (maximum 188 mg/kg in whole sediments) were more than three times the maximum concentrations reported for estuarine sediments in highly urbanised areas near Auckland, New Zealand (Glasby et al. Citation1988) and double those reported for a highly industrialised estuary in Chesapeake Bay, USA (Conrad et al. Citation2007). Sediment Ni concentrations in the vicinity of the Motueka River mouth also exceeded the ANZECC ISQG High threshold by up to 3.6 times. Thus a portion of the depositional region of the river plume may have exerted some degree of contaminant stress on benthic communities, but, as discussed in ANZECC (Citation2000), the expression of biological effects can be extremely variable and may not occur at all depending on a variety of environmental factors.
It is difficult to explain why the spatial pattern of Sr concentrations shows the opposite trend to that of Ni and Cr given that Sr is also known to be elevated in the Red Hills ultramafic rock (Sano et al. Citation1997). Sr concentrations in sediments collected from near the Motueka River mouth were in fact the lowest of any sites measured in the present study. A possible explanation is that Sr was not effectively desorbed under the moderate acid digest conditions used in the present study, therefore remaining bound within the particle matrix. Under certain conditions, however, Sr may substitute for Ca during the process of bio-mineralisation as organisms form aragonite calcareous tests or skeletons (Dodd Citation1965; Amiel et al. Citation1973). The acid extraction procedure used here would be expected to digest carbonate material efficiently in the sediments thus releasing any Sr contained within it. This may explain why the highest Sr concentrations were measured at site MH25, which contained shell and bryozoan debris.
Sediment organic matter and Pb
A relatively small change in sediment organic matter content (i.e. c. 3%) was observed within the study area. This was to be expected as the nutrient loading rates from the catchment have been shown to be too low to result in eutrophic conditions (Gillespie et al. Citation2011). However, previous findings (Forrest et al. Citation2007) provide evidence that catchment-derived organic materials influence sediment characteristics within the vicinity of the Motueka river plume. Fine-grained particulate organic matter, consisting primarily of non-living plant and animal remains or detritus, would be expected to remain suspended longer than coarser-grained or heavier sediment materials. As such, particulate organic matter would be carried further within the buoyant river plume than trace metals-associated with inorganic particles. The observed spatial distribution of sediment organic matter content () is consistent with a clockwise tidal circulation pattern within Tasman Bay in which waters from the Motueka River are directed northward, with additional wind forcing, along the western shoreline (MacKenzie & Adamson Citation2004; Tuckey et al. Citation2006). We recognise that organic materials of marine origin can also contribute to spatial gradients as a result of enhanced sedimentation of phytoplankton in nutrient enriched plume waters (Gibbs Citation2001; MacKenzie Citation2004). Thus, although the observed concentration gradients are modest, the distribution pattern appears to be related to the river outwelling plume.
Although sediment Pb concentrations were low, suggesting no significant anthropogenic sources from within the Motueka catchment, they were strongly correlated with sediment organic content (ρ = 0.004); a relationship that has also been reported for coastal sediments elsewhere (e.g. Gupta & Chen Citation1975).
Infauna
Abundance and composition of infauna communities were weakly correlated with depth and concentrations of Ni and Ba, but it is likely that other, non-measured variables ultimately drive the biological patterns observed in Tasman Bay. For example, physical disturbance could have a marked influence on infauna communities. Sites close to the river mouth were dominated by opportunistic taxa that are often abundant in highly disturbed environments, e.g. H. filiformis (Carvalho et al. Citation2005) and T. lubrica (Hayward Citation1997). Both species are indicative of organic enrichment or otherwise stressed environments (Pearson & Rosenberg Citation1978; Johnston Citation2005). However, H. filiformis burrows are relatively deep, and, as such, can withstand surficial sediment disturbance. After large flood events, taxa capable of enduring physical disturbance may initially be the only ones remaining until recolonisation and recovery of more sensitive taxa occurs. Therefore, the time of sampling relative to the occurrence of flood events could influence patterns of dominance. This study involved sampling immediately after a minor event and revealed high abundances close to the river mouth, and richness values similar to, or slightly higher than, those observed offshore. Forrest et al. (Citation2007) sampled similar sites, directly following the major flood in March 2005. In contrast to the present study, they found low diversity and abundances near to the river mouth, in comparison to offshore sites. It seems likely that the March 2005 flood disturbance had removed much of the infauna community, and may explain the differences in infauna communities between the two studies.
Mechanism of metals transport and deposition
The March 2005 storm was centred in the Red Hills and the upper Motupiko catchment. The SS data clearly shows that a high proportion of sediment load delivered into Tasman Bay during this event came from the Red Hills. Because major rainfall events focused in the upper Motueka catchment are very infrequent, we propose a long-term scenario whereby the initial storm-related mobilisation of trace metals-enriched sediments results in deposition at various downstream locations in the river and the river plume affected region of Tasman Bay. Hicks & Basher (Citation2008) describe the 2005 flood as one example of a threshold event that is triggered by a storm with a recurrence interval >20 years. This event resulted in spatially extensive and temporally persistent changes in SS generation (Basher et al. Citation2011), and consequently ongoing pulses of metals-enriched sediments through the main stem of the river and into the Bay. The overall mechanism, summarised in , depicts dilution along with downstream transport and reduction in metals concentrations as enriched sediments are mixed with non-enriched sediments from tributary streams. Further dilution occurs in Tasman Bay as the SS carried by the plume are deposited on the seabed, repeatedly resuspended (Gibbs Citation2001; Gillespie & Rhodes Citation2006) and transported horizontally and mixed with existing sediments by tidal currents. Thus we would expect that metal concentrations in sediments of the plume-affected region of the Bay would reduce over time. However, subsequent and more frequent rainfall events focused in other parts of the catchment would result in additional pulses of metals-enriched but partially diluted sediments remobilised from depositional locations in the mid to lower river channel. This would result in further discharges of metals-enriched sediments into the Bay along with further dilution. Our May 2006 sampling period was followed by a moderate rainfall event (river flow of 420 m3/s) on 12 June 2006. Measurement of the Ni and Cr concentrations in whole sediments before and after this event indicated a slight increase (i.e. 6%) for both metals at site PS5 and 2% and 5% for Ni and Cr, respectively at site PS10. Although such minor changes were not statistically significant, the consistent pattern is in agreement with the threshold concept described above. Pulses of metals-enriched sediments delivered to, and deposited within, the river plume should gradually decrease over a period of years until another major rainfall event focused in the upper Motueka catchment begins the sequence again. This overall mechanism is supported by observations of reduced Ni and Cr concentrations in sediments sampled from 5–10-m depths of water near the river mouth in May 2006, compared with those of Forrest et al (2007), sampled shortly after the major flood event of March 2005 (i.e. 52% and 31% reduction respectively based on whole sediments).
Redefining the boundaries of the Motueka catchment
Management of the river plume ecosystem (RPE) in the context of catchment influences requires an understanding of the spatial patterns of deposition of river-sourced particulate materials. Combining the indicator composites shown in into a single overall representation and adding previously mapped intertidal and shallow subtidal habitats that are known to be structured by the river's flow regime (Thompson et al. Citation2005) provides a more complete picture of the area of seabed that is strongly influenced by the river plume. However, because the intensity of expression varied considerably amongst the indicator composites, they could not be simply averaged. Instead, a summary diagram was constructed manually () in order to capture the dominant features of the indicator components. We recognise that the mechanisms controlling the distribution patterns for the depositional indicators are complex and in some cases unclear; however their strength for delineating the river plume's influence is enhanced by the unique geology of the catchment (Basher Citation2003) and a good understanding of the prevailing currents (Tuckey et al. Citation2006).
There are a number of caveats associated with construction of this summary diagram. As the shoreward and the northern boundaries of the organic matter/Pb composite are uncertain because of the limited extent of the sampling area, they were extended slightly northward to provide a more realistic representation of the entire depositional footprint. The observed macrofaunal gradients, although omitted from the summary diagram, suggest that they are at least partly plume-related but more localised than the chemical indicators. The high macrofauna richness and abundance zone at the offshore northern edge of the study area () was assumed to be not directly related to the plume.
The area of seabed strongly influenced by the depositional footprint of the river outwelling plume, as defined in , was estimated to be c. 180 km2. We consider it appropriate, in an integrated catchment management (ICM) context, to extend the seaward boundary of the Motueka catchment to include this area. Of course, it should be recognised that widely fluctuating water column characteristics also have important management implications. It is hoped that redefining the catchment boundaries accordingly will facilitate inclusion of marine stakeholders into an expanded management infrastructure, enabling all parties to consider downstream implications of land use practices on marine resources. For example, the expanded catchment described here suggests that a portion of the declining Tasman Bay scallop fishery may be affected by SS deposition from the Motueka River plume. Further work would be required in order to determine whether the observed elevated sediment metals concentrations may have a direct or indirect effect on body loads within biological communities. This has been demonstrated however for the Hudson River plume, which drains from a highly urbanised catchment (Wright et al. Citation2010).
The extended catchment, as defined here, encompasses the entire northern block of the AMA and one half of the central block currently being developed for long-line culture of mussels. Although the southern block of the AMA would be less affected by the plume, it may also receive significant influence from the Waimea and Maitai River catchments at the head of the Bay. Overlapping plume effects from these catchments have not yet been assessed.
The integrated approach developed here could be effectively applied to facilitate improved management of numerous other catchments in New Zealand () and elsewhere that extend into high-value coastal marine or lacustrine receiving environments. Although RPEs can be intrinsically very different, the techniques described here could be employed at other locations to provide the definition required to facilitate an understanding of catchment linkages as an important step towards more effective coastal management.
Acknowledgements
This project was funded by the Foundation for Research Science and Technology, Contract C09X0305. Some of the data presented here was collected as part of an unpublished MSc thesis, Trace metals in sediments and biota of the Motueka River Plume, Tasman Bay, completed in 2007 by Reid Forrest through the University of Otago, Dunedin, New Zealand. The authors gratefully acknowledge the Community Trust of Otago for their generous contribution enabling the establishment of the Community Trust of Otago Centre for Trace Element Analysis. NIWA staff Rob Merrilees, Michelle Wild and Jo Hoyle provided suspended sediments samples, field data and analyses.
References
- Amiel , AJ , Friedman , GM and Miller , DS . 1973 . Distribution and nature of incorporation of trace metals in modern aragonitic corals . Sedimentology , 20 : 47 – 64 .
- Angelidis , MO and Aloupi , M . 2000 . Geochemical study of coastal sediments influenced by river transported pollution: Southern Evoikos Gulf, Greece . Marine Pollution Bulletin , 40 : 77 – 82 .
- ANZECC 2000 . Australian and New Zealand Guidelines for Fresh and Marine Water Quality 2000 Volume 1 . National Water Quality Management Strategy Paper No. 4. Canberra, Australian and New Zealand Environment and Conservation Council and Agriculture and Resource Management Council of Australia and New Zealand.
- APHA 1998 . Standard Methods for the Examination of Water and Wastewater , 20th edition In: LS Clesceri , AE Greenberg , AD Eaton . Washington, DC : American Public Health Association .
- Basher LR (compiler) 2003 . The Motueka and Riwaka catchments: a technical report summarising the present state of knowledge of the catchments, management issues and research needs for integrated catchment management . Lincoln, Landcare Research .
- Basher LR , Hicks DM , Clapp B , Hewitt T 2011 . Sediment yield responses to large storm events and forest harvesting, Motueka River, New Zealand . New Zealand Journal of Marine and Freshwater Research 45 : 333 – 356
- Bowden , WB , Fenemor , AD and Deans , N . 2004 . Integrated water and catchment research for the public good: The Motueka River–Tasman Bay Initiative, New Zealand . Water Resources Development , 20 : 311 – 323 .
- Bray , JR and Curtis , JT. 1957 . An ordination of the upland forest communities of southern Wisconsin . Ecological Monographs , 27 : 325 – 49 .
- Carignan , R and Nriagu , JO . 1985 . Trace metal deposition and mobility in the sediments of two lakes near Sudbury, Ontario . Geochimica et Cosmochimica Acta , 49 : 1753 – 1764 .
- Carpenter , SR and Brock , WA . 2006 . Rising variance: a leading indicator of ecological transition . Ecological Letters , 9 : 311 – 318 .
- Carvalho , S , Moura , A , Gaspar , MB , Pereira , P , de Fonseca , LC , Falcao , M , Drago , T , Leitao , F and Regala , Joao . 2005 . Spatial and inter-annual variability of the macrobenthic communities within a coastal lagoon (Obidos lagoon) and its relationship with environmental parameters . Acta Oecologica , 27 : 143 – 159 .
- Challis , GA . 1965 . The origin of New Zealand ultramafic intrusions . Journal of Petrology , 6 : 322 – 364 .
- Clarke , KR and Ainsworth , M . 1993 . A method of linking multivariate community structure to environmental variables . Marine Ecology Progress Series , 92 : 205 – 219 .
- Clarke KR , Gorley RN 2006 . PRIMER v6 . User manual/tutorial. Plymouth, PRIMER-E .
- Clarke , KR and Warwick , RM . 1994 . Changes in marine communities: an approach to statistical analysis and interpretation , United Kingdom : Natural Environmental Research Council .
- Conrad , CF , Fugate , D , Daus , J , Chisholm-Brause , CJ and Kuehl , SA . 2007 . Assessment of the historical trace metal contamination of sediments in the Elizabeth River, Virginia . Marine Pollution Bulletin , 54 : 385 – 395 .
- Cornelisen , C , Gillespie , P , Kirs , M , Barter , P , Goodwin , E , Forrest , R , Knight , B and Quarterman , A . 2011 . Motueka River plume facilitates transport of ruminant faecal contaminants into shellfish growing waters, Tasman Bay New Zealand . New Zealand Journal of Marine and Freshwater Research , 45 : 477 – 495 .
- Dodd , JR . 1965 . Environmental control of strontium and magnesium in Mytilus . Geochimica Cosmochimica Acta , 29 : 385 – 398 .
- Fenemor , A. , Deans , N , Davie , T , Allen , W , Dymond , J , Kilvington , M , Phillips , C , Basher , L , Gillespie , P , Young , R , Sinner , J , Harmsworth , G , Atkinson , M and Smith , R . 2008 . Collaboration and modelling—tools for integration in the Motueka catchment, New Zealand . Water South Africa , 34 : 448 – 455 .
- Forrest , BM , Gillespie , PA , Cornelisen , CD and Rogers , KM . 2007 . Multiple indicators reveal river plume influence on sediments and benthos in a New Zealand coastal embayment . New Zealand Journal of Marine and Freshwater Research , 41 : 13 – 24 .
- Gibbs , MM . 2001 . Sedimentation suspension and resuspension in Tasman Bay and Beatrix Bay, New Zealand, two contrasting coastal environments which thermally stratify in summer . New Zealand Journal of Marine and Freshwater Research , 35 : 951 – 970 .
- Gillespie , PA , Forrest , RW , Knight , BR , Cornelisen , CD and Young , RG . 2011 . Variation in nutrient loading from the Motueka River into Tasman Bay, New Zealand, 2005–2009: implications for the river plume ecosystem . New Zealand Journal of Marine and Freshwater Research , 45 : 497 – 512 .
- Gillespie P , Rhodes L 2006 . The quantity and quality of suspended particulate material of near-bottom waters in the Motueka River outwelling plume, Tasman Bay . Prepared for Stakeholders of the Motueka Integrated Catchment Management Programme. Cawthron Report No.1114 .
- Glasby , GP , Stoffer , P , Walter , P , Davis , KR and Renner , RM . 1988 . Heavy-metal pollution in Manukau and Waitemata Harbours, New Zealand . New Zealand Journal of Marine and Freshwater Research , 22 : 295 – 611 .
- Gupta , SK and Chen , KY . 1975 . Partitioning of trace metal in selective chemical fractions of near shore sediments . Environmental Letters , 10 : 129 – 158 .
- Hayward , B . 1997 . Introduced marine organisms in New Zealand and their impact in the Waitemata Harbour, Auckland . Tane , 36 : 197 – 223 .
- Hicks , DM and Basher , LR . 2008 . The signature of an extreme erosion event on suspended sediment loads: Motueka River Catchment, South Island, New Zealand. Sediment dynamics in changing environments (Proceedings of a symposium held in Christchurch, December 2008) . IAHS Publ. , 325 : 184 – 191 .
- Johnston OR 2005 . Distribution and biology of the marine invasive bivalve Theora lubrica (Semelideae) . Unpublished Master of Science Thesis, School of Biological Sciences , University of Canterbury , Christchurch .
- Kaushal , SS , Pace , ML , Groffman , PM , Band , LE , Belt , KT , Mayer , PM and Welty , C . 2010 . Land use and climate change variability amplify contaminant pulses. EOS, Transactions . American Geophysical Union , 91 : 221 – 222 .
- Koppelman , MH and Dillard , JG . 1975 . An ESCA study of sorbed metal ions on clay minerals . ACS Symposium Series , 18 : 186 – 201 .
- Loring , DH and Rantala , RTT . 1992 . Manual for the geochemical analyses of marine sediments and suspended particulate matter . Earth Science Reviews , 32 : 235 – 283 .
- Luczak , C , Janquin , MA and Kupka , A . 1997 . A. simple standard procedure for the routine determination of organic matter in marine sediment . Hydrobiologia , 345 : 87 – 94 .
- MacKenzie , L . 2004 . River inputs, re-mineralisation and the spatial and temporal distribution of inorganic nutrients in Tasman Bay, New Zealand . New Zealand Journal of Marine and Freshwater Research , 38 : 681 – 704 .
- MacKenzie , L and Adamson , J . 2004 . Water column stratification and temporal distribution of phytoplankton biomass in Tasman Bay, New Zealand: implications for aquaculture . New Zealand Journal of Marine and Freshwater Research , 38 : 705 – 728 .
- New Zealand Ministry of Fisheries 2008 . Final evaluation—Tasman Interim Aquaculture Management Areas . Appendix 4, Table 2 .
- Pearson , TH and Rosenberg , R . 1978 . Macrobenthic succession in relation to organic enrichment and pollution of the marine environment . Oceanography and Marine Biology Annual Review , 16 : 229 – 311 .
- Sano , S , Tazaki , K , Koide , Y , Nagao , T , Watanabe , T and Kawachi , Y . 1997 . Geochemistry of dike rocks in Dun Mountain Ophiolite, Nelson, New Zealand . New Zealand Journal of Geology and Geophysics , 40 : 127 – 136 .
- Shannon CE 1948 . A mathematical theory of communication . Bell System Technical Journal 27 : 379 – 423 and 623–656 .
- Thompson S , Gillespie P , Robertson B , Tuckey B , Keeley N , Hopkins G 2005 . Broad Scale Mapping of Motueka River Delta: Summary of the intertidal and subtidal habitats . Prepared for Stakeholders of the Motueka Integrated Catchment Management Programme. Cawthron Report No. 976 .
- Tuckey , BJ , Gibbs , MT , Knight , BR and Gillespie , PA . 2006 . Tidal circulation in Tasman and Golden Bays: implications for river plume behaviour . New Zealand Journal of Marine and Freshwater Research. , 40 : 305 – 324 .
- Wild M , Hicks DM , Merrilees R 2006 . Suspended sediment monitoring in the Motueka catchment: data report to 1 May 2006 . NIWA Client Report CHC2006-087 , National Institute of Water and Atmospheric Research , Christchurch .
- Wright , DD , Frazer , TK and Reinfelder , JR . 2010 . The influence of river plume dynamics on trace metal accumulation in calanoid copepods . Limnology and Oceanography , 55 : 2487 – 2502 .