Abstract
Juvenile foraging ability is limited due to physiological and morphological constraints. Restricted foraging skills can result in reduced juvenile survival, which can lead to population decline. Given the important role mass plays in influencing foraging behaviour and survival, we deployed satellite-linked time-depth recorders (TDRs) on seven dependent yearling New Zealand sea lions (Phocarctos hookeri) over three seasons from 2008–2010 at the New Zealand subantarctic Auckland Islands to study how mass influenced at sea movements and diving behaviour. Mass was significantly negatively related to dive depth and descent rate, indicating yearling diving behaviour may be influenced by their mass. The yearlings demonstrated inter-individual variation in foraging locations and strategies. This individual variation was likely attributed to the bathymetry of different foraging areas dictating possible dive depths. Thus, when studying foraging behaviour, in addition to physiological and morphological influences, researchers must examine external factors such as the physical characteristics of the foraging habitat.
Introduction
For young mammals, the transition from nursing to independent foraging can lead to increased mortality (Hayssen Citation1993). Because the success of this transition is dependent on the development of sufficient foraging skills, it is important to understand factors that influence juvenile foraging behaviour (Burns Citation1999). Juvenile foraging ability is limited compared to adults due to physiological and morphological constraints (e.g. lower oxygen stores, higher mass-specific metabolic rates, more drag per unit mass; Schreer & Kovacs Citation1997). Furthermore, factors such as learning to locate prey and experience capturing and handling prey are critical for foraging success, but juveniles also lack foraging experience (e.g. Horning & Trillmich Citation1997). The ability of an animal to search for food may play a critical role in survival, especially when food is scarce (Pitcher et al. Citation2005) and poor juvenile survival may be a direct consequence of restricted foraging skills (Craig & Ragen Citation1999; Irvine et al. Citation2000; Daunt et al. Citation2007). This has important implications for population dynamics since population declines of various mammal and bird species have been partly attributed to poor juvenile survival (Craig & Ragen Citation1999; McMahon et al. Citation2003, Citation2005; Jenkins & Barten Citation2005; Finkelstein et al. Citation2010). Given the vital connection between juvenile foraging ability and survival, examining factors that affect foraging behaviour is essential in understanding how these factors influence population dynamics (Raum-Suryan et al. Citation2004).
Because the shift from dependence on parental care to independent foraging is a critical stage in the life history of juvenile animals, studies of the foraging behaviour of young-of-year are vital (Merrick et al. Citation1987; Burns Citation1999). In naïve young-of-year pinnipeds (sea lions, seals and walruses), mass is positively associated with foraging ability (Burns et al. Citation1997) and survival (McMahon et al. Citation2000). For example, larger weaned southern elephant seal (Mirounga leonina) pups dive deeper, longer and have higher aerobic dive limits than smaller weaned pups (Hindell et al. Citation1999). Larger weaned elephant seal pups also have higher survival rates than smaller weaned pups (McMahon et al. Citation2000) and this may be due to their greater foraging ability.
The New Zealand (NZ) sea lion (Phocarctos hookeri) provides a particularly interesting system to study foraging behaviour. Lactating NZ sea lions are one of the deepest, longest diving otariids (sea lions and fur seals), operate close to their physiological maximum (Gales & Mattlin Citation1997) and forage further from breeding rookeries than any other otariid (Chilvers et al. Citation2005). Lactating NZ sea lions demonstrate distinct benthic or pelagic foraging strategies (Chilvers & Wilkinson Citation2009). Given the extreme diving behaviour of adult NZ sea lions, and that larger animals have disproportionately greater diving capabilities, understanding how juveniles successfully forage is important information for conservation management. The endemic NZ sea lion is one of the rarest, most highly localised otariids in the world (Chilvers Citation2008a) and is listed as Vulnerable by the International Union for Conservation of Nature (IUCN Citation2012) and as Nationally Critical under the New Zealand threat classification system (Baker et al. Citation2010). The main anthropogenic threat to NZ sea lions is commercial trawl fishing for arrow squid (Nototodarus sloanii), through bycatch mortality and potential resource competition (Robertson & Chilvers Citation2011).
Juvenile NZ sea lions and yearlings, in particular, have lower survival than adult NZ sea lions and juveniles of other otariid species (Chilvers & Mackenzie Citation2010). This study is the first to investigate yearling NZ sea lion foraging behaviour. Yearling NZ sea lions are rarely seen at colonies and the majority present are observed suckling (< 5% of lactating females are observed nursing yearlings even though NZ sea lion pups are typically weaned at 9 months; Chilvers & Mackenzie Citation2010). Our study aimed to answer: how does mass influence the foraging locations and diving behaviour of dependent yearling NZ sea lions? We predicted mass would have a positive relationship with foraging behaviour in yearling NZ sea lions, as demonstrated by heavier yearlings travelling further from the study site and diving deeper and longer. However, predictions for diving speed differ depending on foraging strategy. Since larger individuals are capable of staying underwater for longer periods (Schreer & Kovacs Citation1997), for pelagic divers, we expected larger yearlings to dive slower (i.e. lower descent rates) to maximise their prey search time within the water column. For benthic divers, we predicted larger yearlings to dive faster (i.e. higher descent rates) to maximise foraging time along the ocean floor.
Materials and methods
Capture and deployment
We collected data over three austral summers from January–February, 2008–2010 at Sandy Bay, Enderby Island in the New Zealand subantarctic Auckland Islands (50°30′S, 166°17′E; ). All research was conducted with approval from the Animal Ethics Committees: Department of Conservation (DOC) AEC158 (10 December 2007), DOC AEC 200 (2 November 2009) and University of Otago AEC 28/10. We captured between two or three suckling yearlings each season and concurrently captured the mothers for a separate study investigating whether yearlings accompanied their mothers on foraging trips. We caught the animals using a specially designed hoop net and physically restrained them by two handlers (Chilvers et al. Citation2005). Each animal was anaesthetised using isoflurane delivered with oxygen to a mask via a field-portable vaporiser (Gales & Mattlin Citation1998). The animals were then strapped into a custom designed restraint frame and weighed using a 200 kg capacity scale (±0.5 kg, Salter Housewares) suspended from an aluminium tripod.
Sea lions were instrumented with satellite-linked time-depth recorders (TDRs; SPLASH, 100 mm×35 mm×35 mm, 150 g, Wildlife Computers, Redmond, Washington, USA) and also very high frequency (VHF; 3 cm×5 cm×2 cm, 15 g, Sirtrack, Havelock North, New Zealand) transmitters to facilitate recaptures. We attached the instruments to the dorsal pelage of the animal below the shoulder blades on the back midline using two-part epoxy resin. Instrument deployments with epoxy were found to have minimal short and long-term negative impacts on phocid seals (Field et al. Citation2012); thus, this technique is likely benign and appropriate for otariid seals as well. Once the instruments were securely attached, we stopped the flow of anaesthetic and the sea lion was allowed to recover. We observed each animal until it was fully conscious and had returned to the location of capture. We recaptured the animals before the end of the field season (18 February) to retrieve instruments.
Satellite location analysis
We used a random walk modelling approach that uses forward particle sampling to correct and interpolate the raw satellite locations (Tremblay et al. Citation2009). Complete foraging trips were defined as trips with the start and end locations at Sandy Bay or within 10 km of Enderby Island (Chilvers Citation2008b). We restricted calculations of mean distance travelled per trip and total distance travelled to complete foraging trips. Maximum distance travelled from Enderby Island was measured as the straight-line distance from the furthest recorded point to Sandy Bay in ArcGIS 9.3.1 (Esri Citation2009).
Dive analysis
TDRs sampled dive depth (±0.5 m) every 5 s when wet. Depth readings were corrected for shifts in the pressure transducer at the surface of the time-depth recorders before analyses. Because very shallow dives could be misidentified due to error in surface correction or in wave action, we only used dives ≥ 6 m in depth for statistical analysis. Furthermore, dives < 6 m were considered to be non-foraging dives primarily associated with travel (Gales & Mattlin Citation1997). We analysed diving data by producing summary statistics for each dive using the package diveMove (Luque Citation2007) in R software v2.11.1 (R Development Core Team Citation2010). The dive summary file for each individual included the date, time, maximum depth, duration and descent rate for each dive. Otariid dives usually occur in bouts (i.e. the majority of dives occur in rapid succession, with the remaining dives separated by longer periods) (Luque & Guinet Citation2007). We used a maximum likelihood estimation method based approach to identify dive bouts (Luque & Guinet Citation2007) and calculated the number of dives in each bout, number of bouts and bout duration for each individual.
A period ashore began after the tag was dry for 20 min and ended after the tag was wet for 30 s. Foraging trips were identified if continuous wet activity (i.e. continuous depth readings) were available for at least 1 h. This limit was chosen to exclude trips to sea that were for non-foraging activities. Foraging trip duration was calculated from the at sea duration and the subsequent ashore duration. Summary statistics (dives per hour at sea, per cent time spent diving, transiting and ashore) were calculated for each foraging trip.
Statistical analysis
Although inter-annual variation may influence the foraging behaviour observed, small sample sizes each year precluded the differentiation of annual and individual differences. Furthermore, foraging studies on individual adult female NZ sea lions followed across several years indicate no annual variation in foraging behaviour (Chilvers Citation2008b). Hence, we pooled the data across all years. We used one-way ANOVAs to determine if there was significant variation (P<0.05) in various foraging characteristics between individual yearlings. Post-hoc comparisons using Tukey's honest significant differences multiple comparisons of means procedure were done to determine which individuals were significantly different from each other. For data that were not normally distributed or had equal variances, differences between yearlings were established with non-parametric Kruskal-Wallis one-way analysis of variance on ranks and Dunn's post-hoc test was used.
We ran multiple linear mixed effects models (LMEs) using foraging behaviour characteristics as response variables, mass as the predictor variable and individual animal as the random effect on repeated measures data, using the R package nlme (Pinheiro et al. Citation2010). Foraging behaviour was represented by diving behaviour and at sea movements, and was analysed at the scale of individual dives and foraging trips. For individual dives, diving behaviour was characterised by the overall mean depth, duration and descent rate. At the scale of foraging trips, diving behaviour was characterised by the maximum dive depth, duration and descent rate, and by dives per hour, number of dives per bout, bout duration and per cent time spent diving, transiting and ashore per foraging trip. At sea movements were characterised by the mean and maximum trip distance and maximum straight-line distance from the study site. All response variables were power, square root, log or logit transformed to improve the normality of the model residuals. The predictor variable, mass, was centred and standardised to improve the interpretability of regression coefficients (Schielzeth Citation2010).
Results
From January–February, 2008–2010, instruments were deployed on a total of four male and three female yearlings. One female yearling (F3) lost her satellite-linked TDR and only summarised histogram dive data (uplinked via satellite) and satellite data are available for this individual. Values reported for yearlings grouped together are means±1 SEM and for individual animals are means±1 SD.
Foraging behaviour
On average, yearling NZ sea lions spent almost 70% of their time on land, with more of their time at sea spent diving than in transit (). The yearlings foraged within 20 km from the study area () and yearling mean trip distance was one-third of the longest maximum trip distance recorded (). There was a large range in the maximum straight-line distance travelled from Sandy Bay, with the furthest yearling travelling six times further than the closest yearling (). Dive duration and depth were positively related for all yearlings (LME, F 1, 3931=12736, P<0.001). The maximum dive duration was twice as long as the mean duration, with the maximum dive depth three times deeper than the mean depth reached (). Maximum descent rates were over three times faster than mean descent rates (). Ninety-six per cent of all recorded dives occurred in bouts, with the maximum number of dives per bout and bout duration six times higher than the mean number of dives and duration ().
Figure 2 Satellite tracks of seven male and female yearling New Zealand sea lions (Phocarctos hookeri). The 50 and 100 m bathymetry contour lines are indicated in light grey. The inset map indicates the different depths around Enderby Island; depth data (Land Information New Zealand 2012).
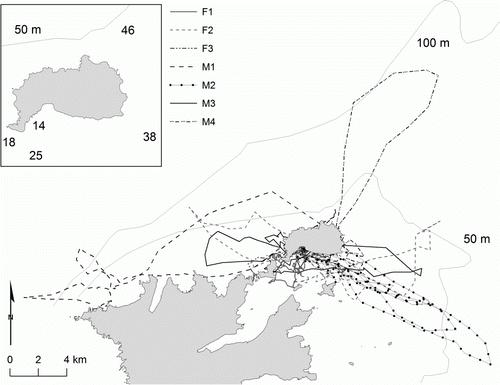
Table 1 Summary of foraging trip characteristics for seven male and female yearling New Zealand sea lions (Phocarctos hookeri). F3 lost her tag and only archival dive data and satellite data are available for this individual.
Table 2 Summary of dive and bout characteristics for seven male and female yearling New Zealand sea lions (Phocarctos hookeri). F3 lost her tag and only archival dive data are available for this individual.
There was inter-individual variation in all of the foraging characteristics measured. Most of the yearlings foraged in different locations from each other, with varying seafloor depths (). However, there was no significant variation among individuals in mean trip distance (). There was significant variation among individuals in mean dive frequency, depth, duration, descent rate, number of dives per bout and bout duration (P<0.001 in all cases). Post-hoc comparison of these characteristics revealed that most of the yearlings significantly differed from each other for all dive characteristics ().
Figure 3 Boxplots of the foraging characteristics for each yearling New Zealand sea lion (Phocarctos hookeri). Groups of individuals not significantly different from each other are indicated by the same letter. The boxplot horizontal lines represent medians, the boxes delineate quantiles and the vertical lines illustrate the range excluding outliers. A, dive frequency. B, dive depth. C, descent rate. D, dive duration. E, number of dives per bout. F, dive bout duration.
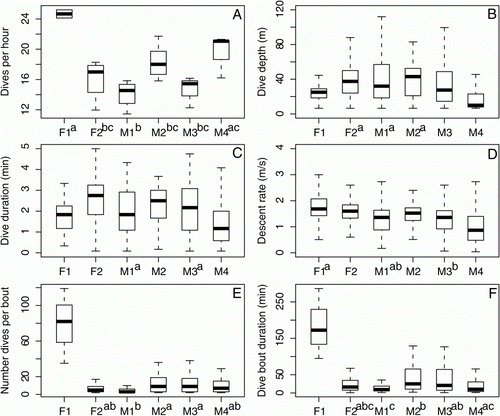
Dive frequency varied across the animals and ranged from 14–24 dives per hour at sea (; A). In addition to each individual diving to different maximum depths (), they also varied in the most frequently used depth ranges (). Most of the yearlings mainly dove to depths ≤ 30 m although yearlings F2 and M2 largely dove to depths ≥ 40 m (c. 60% of dives). Some yearlings tended to dive mainly at shallower depths, while others dove continuously near their maximum depth (). A common trend for all the yearlings is that they appear to be benthic feeders, foraging along the ocean floor ().
Figure 4 Frequency plots of dive depth for each yearling New Zealand sea lion (Phocarctos hookeri). A, F1. B, F2. C, M1. D, M2. E, M3. F, M4.
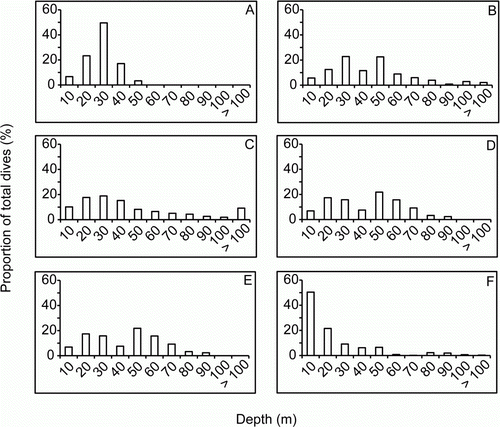
Figure 5 Dive profiles for each yearling New Zealand sea lion (Phocarctos hookeri). A, F1. B, F2. C, M1. D, M2. E, M3. F, M4.
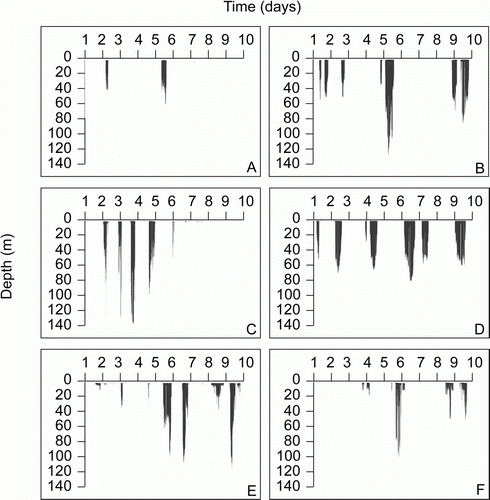
The two female yearlings (F1 and F2) for which full dive data are available had completely different dive profiles from each other (). F2 had the second deepest maximum dive depth and also the second highest maximum dive duration (). In contrast, F1 dove in the shallowest areas, with the shallowest maximum dive depth and also had the shortest maximum dive duration (). F1 showed dive bout behaviour different to the other yearlings, with over seven times the mean number of dives per bout and over six times longer mean dive bout duration than the other yearlings (, ). Though not as markedly different as the two female yearlings, the four male yearlings also had different diving behaviour from each other. Males foraged in areas with different bathymetry (), diving to maximum depths ranging from 83–135 m.
Mass effects
Yearling mass did not differ between sexes (; males 53.5±9.3 kg vs. females 53.7±6.8 kg; Wilcoxon rank sum test, W=7, P=0.86). The second lightest yearling tagged (M1) travelled the furthest straight-line distance from the breeding area, had the highest mean trip distance and foraged in the deepest waters of all the yearlings. However, yearling mass was not significantly correlated with any of the foraging range characteristics. For the dive characteristics, mass was significantly negatively correlated with dive depth (LME, F 4,3932=16.68, P=0.015) and descent rate (LME, F 4,3932=8.2, P=0.04). The lighter yearlings had the deepest mean and maximum dive depths and also the highest dive rates (; ). The heaviest male yearling (M4) had the longest maximum dive duration (5.5 min) and spent the least amount of time on land (). The yearlings spent varying amounts of time on land, transiting and diving (). M4 spent the largest amount of time diving while the lightest female (F1) spent the least.
Discussion
Mass influenced the foraging behaviour of dependent yearling NZ sea lions, with mass being negatively related to dive depth and descent rate. Dependent yearling NZ sea lions demonstrated inter-individual variation in foraging behaviour. The yearlings foraged in different locations of different seafloor depths. The bathymetry in these areas constrained diving behaviour by dictating possible dive depths and, consequently, likely accounted for the observed individual variation.
Foraging behaviour
Although dependent yearling NZ sea lions did not reach adult or juvenile dive depths or durations (e.g. adult females, mean dive depth = 129.4±5.3 m, mean duration = 3.9±1.0 min; Chilvers et al. 2006; 2-year-old females, mean dive depth = 96.2±4.7 m, mean duration = 3.1±0.1 min; E. Leung, unpublished data), their dive records indicate benthic diving. Within dive bouts, yearling sea lions dove to consecutively similar depths, suggesting that the seafloor limited dive depth. Since the local maritime environment surrounding Enderby Island consists of relatively shallow on-shelf waters (< 100 m in depth) (Land Information New Zealand 2012), yearling NZ sea lions were able to dive and forage benthically, but at shallower depths than juveniles and adults. This has important implications for foraging strategies as it indicates that the yearlings are feeding at shallower depths than older individuals, which may influence the prey targeted by these animals.
NZ sea lions are generalist predators, feeding on a wide range of prey items such as fin fish (e.g. opalfish, Hemerocoetes spp., rattail, Coelorhynchus aspercephalus, hoki, Macruronus novaezelandiae and red cod, Pseudophycis bachus) and cephalopods (squid, Nototodarus spp. and octopus, Enteroctopus zelandicus) (Childerhouse et al. Citation2001; Meynier et al. Citation2009, Citation2010). A constraint on benthic foraging is limited available foraging habitat due to the finite extent of the continental shelf. This limitation is stronger on yearlings, which cannot reach adult foraging depths and likely restricts the prey species that can be exploited by yearlings. As seafloor depth increases with distance from shore, yearling foraging habitat is limited by the extent they can reach seafloor depth to exploit benthic prey and may explain the small foraging ranges of the tagged yearlings. Alternatively, it is possible that dependent yearlings forage farther and deeper, but this was not observed in this study perhaps due to the short duration of instrument deployment. Furthermore, we cannot rule out that dependent yearling NZ sea lions may be capable of foraging further to areas with deeper waters and exploiting prey at deeper depths, but they may not do so because their diet is supplemented by milk and they need to return ashore periodically to nurse.
Satellite locations and diving records indicate dependent yearling NZ sea lions do not accompany their mothers at sea (E. Leung, unpublished data). However, the constraint of having to stay near Enderby Island to nurse likely restricted the foraging ranges of the tagged yearlings, since their mothers returned ashore on average every 48.4±5.8 h (E. Leung, unpublished data), but the yearlings likely do not know when their mothers will return. The restricted foraging areas resulted in the foraging ranges of dependent yearling NZ sea lions being largely encompassed within the 12 nautical mile marine reserve surrounding the Auckland Islands. However, it is likely that independent yearlings forage differently since nursing does not confound their behaviour and they are self-reliant for their diet. Thus, independent yearlings may target different prey at deeper depths and/or further locations, and may forage outside the marine reserve where they are at risk of fisheries impacts such as bycatch and resource competition. Indeed, yearling NZ sea lions have been incidentally caught on squid trawl boats that operate on the Auckland Island shelf (Duignan et al. Citation2003). Unfortunately, it is logistically difficult to conduct foraging studies on independent yearling NZ sea lions since they are rarely seen at study sites.
The satellite and dive data of dependent yearling NZ sea lions demonstrate inter-individualvariation in foraging locations and behaviour. NZ sea lion yearlings foraged in different areas () and at diverse depths for varying durations (). Most of the yearlings significantly differed from each other in mean dive frequency, depth, duration, descent rate, number of dives per bout and bout duration (). The individual variation in the foraging behaviour of dependent yearling NZ sea lions observed may be indicative of individual-based foraging strategies, but due to low sample size we cannot differentiate if the differences observed are due to factors intrinsic to each individual or are artefacts of the low sample size. Furthermore, this individual variation may be attributed to the seafloor depth of different foraging locations dictating possible dive depths. Bathymetry can play a large role in influencing foraging strategies (Halsey et al. Citation2006). Animals that only have access to deep water where they are unable to reach the seafloor cannot forage benthically, whereas animals that forage in areas where they can reach the seafloor are able to forage benthically or pelagically. Thus, when investigating factors influencing foraging behaviour, researchers must take into account the physical characteristics of the foraging habitat, in addition to physiological and morphological factors. However, it is only recently that studies have considered physical factors such as bathymetry when interpreting foraging behaviour (e.g. Halsey et al. Citation2006).
Mass-related differences in foraging behaviour
Physiological capacity strongly affects foraging behaviour (Costa et al. Citation2001), with larger animals being able to dive longer and deeper than smaller animals (Schreer & Kovacs Citation1997). Larger size confers physiological and morphological advantages, such as lower mass-specific metabolic rates, higher oxygen stores and lower drag per unit mass (Schreer & Kovacs Citation1997). However, in this study it may be difficult to clearly elucidate the underlying relationship of mass with diving behaviour, since the foraging behaviour of dependent NZ sea lion yearlings was confounded by their diet being supplemented by milk. Thus, the tagged yearlings may be capable of diving deeper or longer, but do not need to expend more foraging effort in order to obtain sufficient nutrition. As predicted, the heaviest yearling NZ sea lion had the longest maximum dive duration. Of the various measures of foraging ability, dive duration is expected to be most responsive to variation in mass (Burns et al. Citation1997). Smaller animals have lower aerobic dive capacity, restricting their ability to make long dives (Burns Citation1999). But against our expectations, mass was negatively related to descent rate and dive depth in yearling NZ sea lions. Similarly, larger yearling Weddell seals made longer, but shallower dives than smaller yearlings (Burns et al. Citation1997). It is more difficult to elucidate the relationship of mass with descent rate and dive depth as body size is less likely to constrain either of these (Burns et al. Citation1997). Larger yearlings may be more buoyant due to higher blubber stores (although buoyancy was not measured in this study), and buoyancy is negatively related to descent rate (Webb et al. Citation1998). Additionally, behavioural preferences (Salmon et al. Citation2004) would also influence diving behaviour. For instance, juvenile green turtles (Chelonia mydas) were capable of deeper dives when sufficiently motivated, but in the absence of that motivation, the turtles chose to dive within 10 m of the ocean surface (Salmon et al. Citation2004). Therefore, the negative relationship between mass and dive depth may simply be attributed to larger individual yearling NZ sea lions choosing not to dive as deep as they are capable of. For example, male juvenile NZ sea lions are larger and dove to maximum depths 1.5 times deeper than female juveniles, but on average, the female juveniles dove 30% deeper than the males (E. Leung, unpublished data).
Furthermore, mass is not the only influence on diving behaviour, as the physical properties of the foraging habitat would dictate dive depth (Halsey et al. Citation2006). The heaviest yearling (M4) was 30% heavier than the mean mass of the other yearlings; we expected this higher mass to result in deeper and longer dives, as larger animals experience less drag per unit mass (Schreer & Kovacs Citation1997) and are able to remain submerged longer (Burns Citation1999). Although M4 had the longest maximum dive duration, on average this yearling had the shallowest dive depth, shortest dive duration and also the slowest swim speed (). In contrast, the second lightest yearling (M1) had the deepest mean and maximum dive depth, longest mean trip distance and travelled the furthest from the breeding area (Tables 1, 2). These differences are probably attributed to M1 foraging in the deepest waters closest to Enderby Island (). Thus, although the foraging behaviour of yearling NZ sea lions may be influenced by their size and perhaps behavioural preferences, bathymetry also plays a strong role in influencing their diving behaviour. Oceanographic conditions also affect foraging behaviour, as has been observed in juvenile loggerhead turtles (Caretta caretta; Howell et al. Citation2010) and juvenile shy albatross (Thalassarche cauta; Alderman et al. Citation2010), but is likely not applicable in this study due to the small range of movements of dependent yearling NZ sea lions.
Conclusions
Dependent yearling NZ sea lion mass was negatively related to dive depth and descent rate and yearlings also demonstrated inter-individual variation in foraging behaviour. Mass effects on foraging behaviour are likely due to size-related physiological and morphological factors, such as lower mass-specific metabolic rates, higher oxygen stores and lower drag per unit mass for larger animals (Schreer & Kovacs Citation1997). However, bathymetry also played a strong role in influencing foraging behaviour by dictating possible dive depths. Bathymetry likely accounted for most of the observed inter-individual variation in foraging behaviour characteristics since the yearlings foraged in different areas with varying seafloor depths. This advocates for the consideration of external influences that can constrain dive behaviour (e.g. bathymetry) and impact foraging strategies, in addition to examining physiological and morphological factors in foraging studies.
Acknowledgements
We thank A. Augé, L. Boren, H. McConnell, J. Amey, W. Roe, L. Meynier, K. Geschke, R. Hood, J. Fyfe, K. McInnes, N. McNally, G. Oakes and M. Riki for their assistance with animal captures. We thank P. Robinson for running the particle filter on the satellite data. A. Jacob and two anonymous reviewers provided helpful comments that greatly improved this manuscript. Data presented in this paper were collected from a long-term study funded by the New Zealand Department of Conservation (DOC) investigation no. 1638, in parallel with fieldwork undertaken for the DOC Conservation Services Program (DOC project Pop 2007/01; www.doc.govt.nz/mcs) and through a levy principally on the quota holders of SQU 6T. Funding was also provided by the University of Otago Zoology Department. Animal Ethics Committee Approval: DOC AEC158 (10 December 2007), DOC AEC 200 (2 November 2009) and University of Otago AEC 28/10. E. Leung was supported by a University of Otago Postgraduate Scholarship.
References
- Alderman , R , Gales , R , Hobday , AJ and Candy , SG . 2010 . Post-fledging survival and dispersal of shy albatross from three breeding colonies in Tasmania . Marine Ecology Progress Series , 405 : 271 – 285 . doi: 10.3354/meps08590
- Baker , CS , Chilvers , BL , Constantine , R , DuFresne , S , Mattlin , RH , van Helden , A and Hitchmough , R . 2010 . Conservation status of New Zealand marine mammals (suborders Cetacea and Pinnipedia), 2009 . New Zealand Journal of Marine and Freshwater Research , 44 : 101 – 115 . doi: 10.1080/00288330.2010.482970
- Burns , JM . 1999 . The development of diving behavior in juvenile Weddell seals: pushing physiological limits in order to survive . Canadian Journal of Zoology , 77 : 737 – 747 . doi: 10.1139/z99-022
- Burns , JM , Schreer , JF and Castellini , MA . 1997 . Physiological effects on dive patterns and foraging strategies in yearling Weddell seals (Leptonychotes weddellii) . Canadian Journal of Zoology , 75 : 1796 – 1810 . doi: 10.1139/z97-809
- Childerhouse , S , Dix , B and Gales , N . 2001 . Diet of New Zealand sea lions (Phocarctos hookeri) at the Auckland Islands . Wildlife Research , 28 : 291 – 298 . doi: 10.1071/WR00063
- Chilvers , BL . 2008a . New Zealand sea lions Phocarctos hookeri and squid trawl fisheries: bycatch problems and management options . Endangered Species Research , 5 : 193 – 204 . doi: 10.3354/esr00086
- Chilvers , BL . 2008b . Foraging site fidelity of lactating New Zealand sea lions . Journal of Zoology , 276 : 28 – 36 . doi: 10.1111/j.1469-7998.2008.00463.x
- Chilvers , BL and Mackenzie , D . 2010 . Age and sex specific survival estimates incorporating tag loss for New Zealand sea lions, Phocarctos hookeri . Journal of Mammalogy , 91 : 758 – 767 . doi: 10.1644/09-MAMM-A-285.1
- Chilvers , BL and Wilkinson , IS . 2009 . Diverse foraging strategies in lactating New Zealand sea lions . Marine Ecology Progress Series , 378 : 299 – 308 . doi: 10.3354/meps07846
- Chilvers , BL , Wilkinson , IS , Duignan , PJ and Gemmell , NJ . 2006 . Diving to extremes: are New Zealand sea lions (Phocarctos hookeri) pushing their limits in a marginal habitat? . Journal of Zoology , 269 : 233 – 240 .
- Chilvers , BL , Wilkinson , IS , Duignan , PJ and Gemmell , NJ . 2005 . Summer foraging areas for lactating New Zealand sea lions Phocarctos hookeri . Marine Ecology Progress Series , 304 : 235 – 247 . doi: 10.3354/meps304235
- Costa , DP , Gales , NJ and Goebel , ME . 2001 . Aerobic dive limit: how often does it occur in nature? . Comparative Biochemistry and Physiology , 129 : 771 – 783 . doi: 10.1016/S1095-6433(01)00346-4
- Craig , MP and Ragen , TJ . 1999 . Body size, survival, and decline of juvenile Hawaiian monk seals, Monachus schauinslandi . Marine Mammal Science , 15 : 786 – 809 . doi: 10.1111/j.1748-7692.1999.tb00843.x
- Daunt , F , Afanasyev , V , Adam , A , Croxall , JP and Wanless , S . 2007 . From cradle to early grave: juvenile mortality in European shags Phalacrocorax aristotelis results from inadequate development of foraging proficiency . Biology Letters , 3 : 371 – 374 . doi: 10.1098/rsbl.2007.0157
- Duignan PJ , Gibbs NJ , Jones GW 2003 . Autopsy of pinnipeds incidentally caught in commercial fisheries, 2001/02. DOC Science Internal Series 131 Wellington New Zealand Department of Conservation 40
- Esri 2009 . ArcGIS Desktop 9.3.1 . Redlands , CA .
- Field , IC , Harcourt , RG , Boehme , L , de Bruyn , PJN , Charrassin , J-B , McMahon , CR , Bester , MN , Fedak , MA and Hindell , MA . 2012 . Refining instrument attachment on phocid seals . Marine Mammal Science , 28 : E325 – E332 . doi: 10.1111/j.1748-7692.2011.00519.x
- Finkelstein , ME , Doak , DF , Nakagawa , M , Sievert , PR and Klavitter , J . 2010 . Assessment of demographic risk factors and management priorities: impacts on juveniles substantially affect population viability of a long-lived seabird . Animal Conservation , 13 : 148 – 156 . doi: 10.1111/j.1469-1795.2009.00311.x
- Gales , NJ and Mattlin , RH . 1997 . Summer diving behaviour of lactating New Zealand sea lions, Phocarctos hookeri . Canadian Journal of Zoology , 75 : 1695 – 1706 . doi: 10.1139/z97-796
- Gales , NJ and Mattlin , RH . 1998 . Fast, safe, field-portable gas anesthesia for pinnipeds . Marine Mammal Science , 14 : 355 – 361 . doi: 10.1111/j.1748-7692.1998.tb00727.x
- Halsey , LG , Blackburn , TM and Butler , PJ . 2006 . A comparative analysis of the diving behaviour of birds and mammals . Functional Ecology , 20 : 889 – 899 . doi: 10.1111/j.1365-2435.2006.01170.x
- Hayssen , V . 1993 . Empirical and theoretical constraints on the evolution of lactation . Journal of Dairy Science , 76 : 3213 – 3233 . doi: 10.3168/jds.S0022-0302(93)77659-6
- Hindell , MA , McConnell , BJ , Fedak , MA , Slip , DJ , Burton , HR , Reijnders , PJH and McMahon , CR . 1999 . Environmental and physiological determinants of successful foraging by naive southern elephant seal pups during their first trip to sea . Canadian Journal of Zoology , 77 : 1807 – 1821 .
- Horning , M and Trillmich , F . 1997 . Ontogeny of diving behaviour in the Galapagos fur seal . Behaviour , 134 : 1211 – 1257 . doi: 10.1163/156853997X00133
- Howell , EA , Dutton , PH , Polovina , JJ , Bailey , H , Parker , DM and Balazs , GH . 2010 . Oceanographic influences on the dive behavior of juvenile loggerhead turtles (Caretta caretta) in the North Pacific Ocean . Marine Biology , 157 : 1011 – 1026 . doi: 10.1007/s00227-009-1381-0
- Irvine , LG , Hindell , MA , van den Hoff , J and Burton , HR . 2000 . The influence of body size on dive duration of underyearling southern elephant seals (Mirounga leonina) . Journal of Zoology , 251 : 463 – 471 . doi: 10.1111/j.1469-7998.2000.tb00802.x
- IUCN (International Union for Conservation of Nature) 2012 . IUCN Red List of threatened species , version 2012.2. Phocarctos hookeri (Hooker's Sealion, Hooker's Sea Lion, New Zealand Sealion, New Zealand Sea Lion). http://www.iucnredlist.org/details/17026/0 (accessed 1 November 2012) .
- Jenkins , KJ and Barten , NL . 2005 . Demography and decline of the Mentasta caribou herd in Alaska . Canadian Journal of Zoology , 83 : 1174 – 1188 . doi: 10.1139/z05-111
- Land Information New Zealand 2012 . Chart NZ 286 Auckland Islands . Wellington New Zealand http://www.linz.govt.nz/hydro/charts/nz202-chart-catalogue/acknowledge.aspx?chart_num=286 (accessed 13 April 2012) .
- Luque , SP . 2007 . Diving behavior analysis in R . R News , 7 : 8 – 14 .
- Luque , SP and Guinet , C . 2007 . A maximum likelihood approach for identifying dive bouts improves accuracy, precision and objectivity . Behaviour , 144 : 1315 – 1332 . doi: 10.1163/156853907782418213
- McMahon , CR , Burton , HR and Bester , MN . 2000 . Weaning mass and the future survival of juvenile southern elephant seals, Mirounga leonina, at Macquarie Island . Antarctic Science , 12 : 149 – 153 . doi: 10.1017/S0954102000000195
- McMahon , CR , Burton , HR and Bester , MN . 2003 . A demographic comparison of two southern elephant seal populations . Journal of Animal Ecology , 72 : 61 – 74 . doi: 10.1046/j.1365-2656.2003.00685.x
- McMahon , CR , Hindell , MA , Burton , HR and Bester , MN . 2005 . Comparison of southern elephant seal populations, and observations of a population on a demographic knife-edge . Marine Ecology Progress Series , 288 : 273 – 283 . doi: 10.3354/meps288273
- Merrick , RL , Loughlin , TR and Calkins , DG . 1987 . Decline in abundance of the northern sea lion, Eumetopias jubatus, in Alaska, 1956–1986 . Fishery Bulletin , 85 : 351 – 365 .
- Meynier , L , Mackenzie , DDS , Duignan , PJ , Chilvers , BL and Morel , PCH . 2009 . Variability in the diet of New Zealand sea lion (Phocarctos hookeri) at the Auckland Islands, New Zealand . Marine Mammal Science , 25 : 302 – 326 . doi: 10.1111/j.1748-7692.2008.00252.x
- Meynier , L , Morel , PCH , Chilvers , BL , Mackenzie , DDS and Duignan , PJ . 2010 . Quantitative fatty acid signature analysis on New Zealand sea lions: model sensitivity and diet estimates . Journal of Mammalogy , 91 : 1484 – 1495 . doi: 10.1644/09-MAMM-A-299.1
- Pinheiro J , Bates D , DebRoy S , Sarkar D , R Development Core Team 2010 . nlme: linear and nonlinear mixed effects models . R package, version 3.1-97 .
- Pitcher , KW , Rehberg , MJ , Pendleton , GW , Raum-Suryan , KL , Gelatt , TS , Swain , UG and Sigler , MF . 2005 . Ontogeny of dive performance in pup and juvenile Steller sea lions in Alaska . Canadian Journal of Zoology , 83 : 1214 – 1231 . doi: 10.1139/z05-098
- R Development Core Team 2010 . R: a language and environment for statistical computing . Vienna , , Austria , R Foundation for Statistical Computing .
- Raum-Suryan , KL , Rehberg , MJ , Pendleton , GW , Pitcher , KW and Gelatt , TS . 2004 . Development of dispersal, movement patterns and haul-out use by pup and juvenile Steller sea lions (Eumetopias jubatus) in Alaska . Marine Mammal Science , 20 : 823 – 850 . doi: 10.1111/j.1748-7692.2004.tb01195.x
- Robertson , BC and Chilvers , BL . 2011 . The population decline of the New Zealand sea lion Phocarctos hookeri: a review of possible causes . Mammal Review , 41 : 253 – 275 . doi: 10.1111/j.1365-2907.2011.00186.x
- Salmon , M , Jones , TT and Horch , KW . 2004 . Ontogeny of diving and feeding behavior in juvenile sea turtles: leatherback sea turtles (Dermochelys coriacea L) and green sea turtles (Chelonia mydas L) in the Florida current . Journal of Herpetology , 38 : 36 – 43 . doi: 10.1670/228-01A
- Schielzeth , H . 2010 . Simple means to improve the interpretability of regression coefficients . Methods in Ecology and Evolution , 1 : 103 – 113 . doi: 10.1111/j.2041-210X.2010.00012.x
- Schreer , JF and Kovacs , KM . 1997 . Allometry of diving capacity in air-breathing vertebrates . Canadian Journal of Zoology , 75 : 339 – 358 . doi: 10.1139/z97-044
- Tremblay Y , Robinson PW , Costa DP 2009 . A parsimonious approach to modeling animal movement data . PLoS ONE 4 .
- Webb , PM , Crocker , DE , Blackwell , SB , Costa , DP and Le Boeuf , BJ . 1998 . Effects of buoyancy on the diving behavior of northern elephant seals . Journal of Experimental Biology , 201 : 2349 – 2358 .