Abstract
The assessment of biological samples is critical for measuring the ‘health’ of New Zealand aquatic environments. Analysis of these samples commonly requires species identification and enumeration, which usually involves microscopy or microbiological methods. These techniques can be time-consuming, laborious, and are dependent on taxonomic expertise. Recent advances in molecular methods provide promising tools for assessing environmental samples. A range of molecular techniques are now used in New Zealand including: fluorescent in situ hybridisation; automated ribosomal intergenic spacer analysis; quantitative polymerase chain reaction; and, most recently, next-generation sequencing. The organisms (or targets) and environments monitored are equally diverse, ranging from cyanobacteria, rotifers and invasive fish in lakes, to macroinvertebrates, and biofilm communities in rivers, to bacteria, micro- and macro-algae and invertebrates in marine ecosystems. Despite research and validation demonstrating their potential, the application of these tools by monitoring agencies has been limited. Legislative requirements, costs, and a reluctance to change methodologies, are the most likely reasons for this. This review examines molecular tools that have been previously or are currently used for monitoring aquatic environments in New Zealand, and explores how these, and new techniques, may be applied in the future.
Indroduction
Marine and freshwater environments are an essential part of New Zealand's heritage. New Zealand encompasses more than 330 islands with 18,218 km of coastline (Ministry for the Environment Citation2007). The approximately 4 million km2 of ocean administered by the New Zealand government is the sixth largest in the world. It incorporates a diverse range of ecosystems that support more than 17,000 formally identified species (Ministry for the Environment Citation2007; Gordon Citation2012). New Zealand's marine environment is utilised for many purposes, including recreation, tourism and food gathering, and it has important spiritual and cultural values. Equally important and diverse are New Zealand's freshwater environments with approximately 425,000 km of rivers and streams, 4000 lakes larger than 1 ha, and 200 aquifers (Ministry for the Environment Citation2007). The clarity and quality of New Zealand's freshwater is vital for economic prosperity, environmental sustainability and human and stock health.
New Zealand's aquatic environments face a plethora of threats including urbanisation, farming (marine or land-based), catchment modification, nutrient enrichment and the introduction of new species (intentionally or unintentionally). Monitoring the ecological status of New Zealand aquatic ecosystems is critical to enabling long-term trends to be assessed, assisting in understanding the effects of anthropogenic activities, detecting the introduction of new species (ideally before they spread and impact native ecosystems), and ensuring the wellbeing of the animals and humans who use them. Environmental monitoring is carried out by a range of organisations for multiple purposes. For example, Regional Councils monitor a variety of water bodies as part of their State of the Environment programmes to track long-term trends in water quality, whereas shellfish farmers may need to monitor the concentrations of harmful algae or bacteria present, or benthic diversity to meet the requirements of the Resource Management Act 1991. Typically, data on a variety of chemical (e.g. nutrients), physical (e.g. water temperature) and biological parameters (e.g. the presence or numbers of indicator species) are collected. Measuring biological parameters usually involves the identification and enumeration of species within a sample, which almost always requires microscopy. Microscopy is often time-consuming, resource intensive and dependent upon expert taxonomic identification. Traditional morphological based taxonomy is currently not a very popular science, and numbers of scientists working in this area are reducing (Kim & Byrne Citation2006).
Molecular-based techniques are advancing rapidly and provide numerous methods for identifying and, in some cases, enumerating target species or genes in environmental samples. Molecular techniques also have some advantages over microscopic techniques as they can be developed to be high-throughput, allowing multiple samples to be processed simultaneously, and can also target functional genes (e.g. genes involved in toxin production), thus providing information on the functional potential of an organism (i.e. does it have the ability to produce toxins). Molecular tools need to be developed in concert with taxonomists since validation of many molecular tools requires voucher samples. It is likely that, in many instances, molecular tools will complement the current morphological-based techniques.
The aim of this review is to discuss the molecular tools that have been developed or validated in New Zealand for the purpose of monitoring aquatic environments. The first part of the review provides a brief overview of the molecular techniques that are detailed throughout the proceeding sections. The remainder of the review is divided into a series of sections, each of which explores the past and current use of molecular tools for differing environmental monitoring purposes. Finally, we speculate on the future of this rapidly advancing field, consider how new techniques can and will be used, and explore new applications for these tools. The use of molecular techniques for research in the aquatic science field has been far-reaching and is beyond the scope of this review. Our review focuses on molecular tools used for biomonitoring and does not include diseases or parasites that may live in or on aquatic animals.
Molecular techniques discussed in this review
A wide array of molecular-based techniques have been employed worldwide for the identification and monitoring of aquatic organisms (Bott et al. Citation2010; Humbert et al. Citation2010; Darling & Mahon Citation2011). These include a variety of hybridisation techniques, polymerase chain reaction (PCR)-based techniques and recent advances in sequencing technologies. The ‘Applications of molecular techniques’ section of this review provides examples of the development and applications of molecular genetic tools in aquatic monitoring in New Zealand. The descriptions in this section, and Figs. , provide a very brief overview of the molecular basis of each of the techniques referred to. These figures and text are designed for readers with some molecular knowledge. Readers wanting more detailed explanations are referred to the plethora of internet resources which provide animations and diagrams of these methods (e.g. the DNA Learning Centre: www.dnalc.org; and Molecular Station: www.molecularstation.com).The advantages, limitations and an indication of costs associated with each technique are summarised in .
Table 1 Examples of molecular methods used for environmental monitoring of aquatic habitats. Advantages and limitations, and examples of use in New Zealand are given.
Hybridisation techniques
Fluorescent in situ hybridisation
Fluorescent in situ hybridisation (FISH) involves the use of a fluorescently labelled complementary DNA or RNA probe that is designed to bind to a specific region of the target DNA or RNA (). The technique can be employed for the visual identification of species and often uses fluorescent microscopy to visualise where the fluorescent probe binds (i.e. location on chromosomes, tissue section and/or entire organism). FISH has advantages over more traditional in situ hybridisation (ISH); namely, it is faster, safer (no radioactivity) and has the added value that different fluorochromes can be used to discriminate different targets simultaneously (Bartlett & Bartlett Citation2004).
Figure 1 Schematic of fluorescent in situ hybridisation (FISH). A fluorescently labelled complementary DNA or RNA probe binds to a specific target. Fluorescent microscopy is used to visualise where the fluorescent probe binds. The photo shows a fluorescein-labelled species-specific oligonucleotide probe, targeted against rRNA in a New Zealand native starfish.
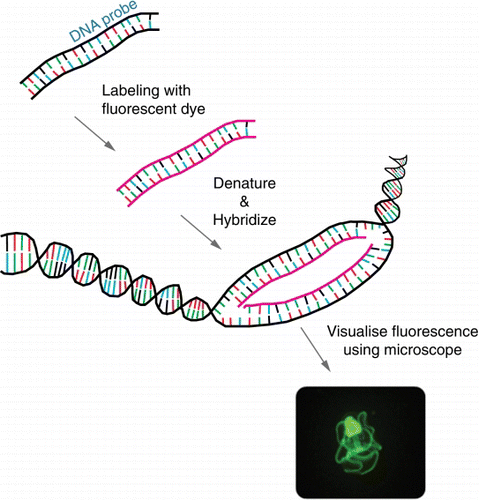
Sandwich hybridisation arrays
Sandwich hybridisation arrays (SHA) are a DNA or RNA probe-based method that can be used for rapid identification and enumeration of organisms. SHAs utilise two or more probes. The first is a capture probe, which hybridises to complementary nucleic acids. The bound nucleic acid is reacted with a second probe for detection, which is labelled with fluorescent, chemiluminescent or colorimetric labels. The ‘sandwich’ is achieved when the nucleic acids have complementary sequences and hybridise to both the capture and detection probes ().
Figure 2 Schematic of sandwich hybridisation array (SHA). SHA uses an oligonucleotide capture probe targeting a specific DNA/RNA target and one or more signal probes, which attach to the bound region, forming a ‘sandwich’. The presence of target DNA/RNA in the sample is represented colorimetrically, fluorescently or chemiluminescently.
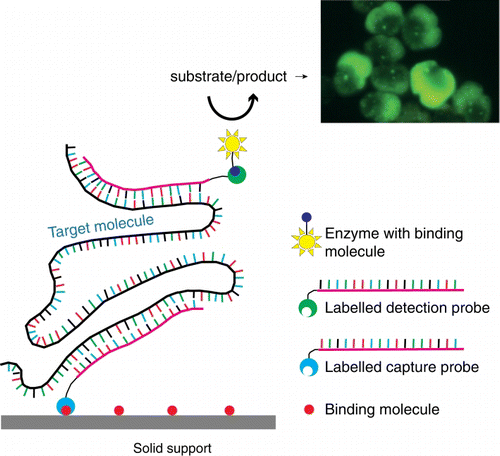
Polymerase chain reaction-based techniques
PCR and end-point PCR
Invented more than 30 years ago (Bartlett & Stirling Citation2003), PCR has revolutionised many areas of biological and medical research. The high specificity afforded by PCR makes it very effective for species and strain identification of a wide range of organisms. PCR allows amplification of as little as a single piece of DNA or RNA, generating thousands to millions of copies of a particular DNA/RNA sequence. PCR relies on thermal cycling and uses a combination of deoxyribonucleotides (dNTP), oligonucleotides (primers) and a polymerase enzyme to exponentially amplify the DNA/RNA template. In traditional PCR, the resulting ‘amplicon’ products are visualised on an agarose gel using a fluorescent dye (end-point PCR; ). Quantification of the initial sample concentration is not possible using end-point analysis since PCR efficiency decreases as the reaction nears the plateau phase.
Figure 3 Schematic of end-point PCR and quantitative PCR (QPCR). Using a combination of deoxyribonucleotides (dNTP), oligonucleotides (primers) which are specific to the target, a polymerase enzyme and a series of heating and cooling steps, the DNA/RNA template is exponentially amplified. The resulting ‘amplicon’ products are visualised on an agarose gel for end-point PCR (bottom left) or by measuring florescence for QPCR (bottom right).
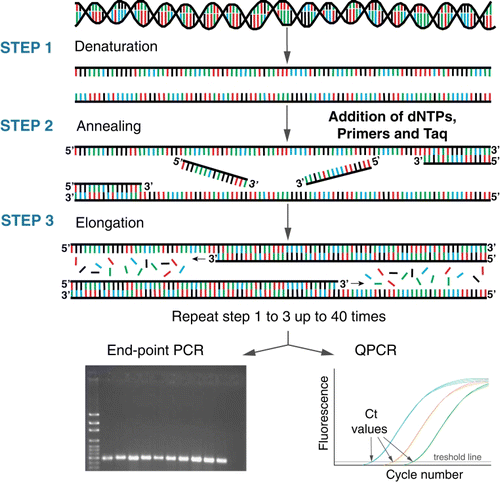
Quantitative PCR
Quantitative PCR (QPCR), also sometimes referred to as real-time PCR, allows the amplification of a target PCR amplicon to be monitored in real-time as amplification occurs. QPCR can be conducted rapidly (< 2 h) to determine whether a specific DNA/RNA sequence is present in a sample, while also calculating the number of copies of the target DNA/RNA sequences. The technique is routinely used in clinical applications for the detection of a wide range of organisms, including bacteria, fungi, parasites and other pathogens (Espy et al. Citation2006). In order to measure the amount of amplified product in real-time, QPCR uses either intercalating dyes such as SYBR Green (Becker et al. Citation1996), LCGreen (Wittwer et al. Citation2003), SYTO9 (Monis et al. Citation2005), EvaGreen (Wang et al. Citation2006) or highly-specific probe-based detection systems, including TaqMan (Heid et al. Citation1996), minor groove binder eclipse probe (Afonina et al. Citation2002), or fluorescence resonance energy transfer (Chen & Kwok Citation1999). Absolute quantification is determined by calculating a cycle threshold (CT) of test samples by simultaneously amplifying target DNA/RNA standards of known concentration. The CT is then calculated based on a standard curve generated from the DNA/RNA standards ().
Automated ribosomal intergenic spacer analysis
Automated ribosomal intergenic spacer analysis (ARISA) is an automated fingerprinting technique that specifically targets the non-coding internal transcribed spacer (ITS) regions of the small and large subunit (SSU and LSU) rRNA operon. ARISA has been most commonly employed for characterising spatio-temporal changes among microbial communities from environmental samples (Ranjard et al. Citation2001). This technique exploits the highly variable nature of ITS (i.e. variability in nucleotide sequence and length) as well as the conservative nature of the flanking SSU/LSU genes, to PCR-amplify all ITS copies from mixed community samples using a fluorescently labelled primer. The resulting amplicon fragments are then electrophoresed to separate them according to size, and the results are displayed as a series of peaks on an electropherogram (). The differences in peak patterns among samples are interpreted as changes in microbial community structure.
Figure 4 Schematic of automated ribosomal intergenic spacer analysis (ARISA). A PCR (see ) that targets the internal transcribed spacer (ITS) regions of the small and large subunit (SSU and LSU) rRNA operon is undertaken using a fluorescently labelled primer. The amplicon fragments are then separated by size on an electropherogram. The pattern of peaks provides information on the microbial community structure.
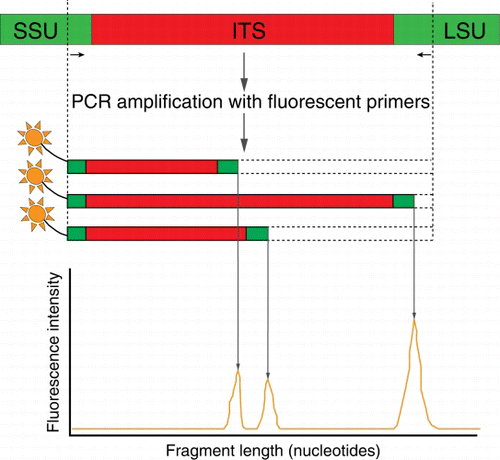
Terminal restriction fragment length polymorphism
Terminal restriction fragment length polymorphism (T-RFLP) was developed for generating a ‘fingerprint’ of an unknown microbial community based on sequence difference with the 16S rRNA gene (Liu et al. Citation1997). This technique is very similar to ARISA as it is also based on fluorescently labelled PCR amplifications of a target gene and visualisation of a collection of fragments of different sizes, which may be indicative of certain microbial communities. The technique differs from ARISA in that PCR amplicons are digested with specific restriction enzymes that ‘cut’ the DNA molecule at known sequences. The size of each resulting terminal restriction fragment is then translated into peaks on an electropherogram and the patterns can be compared to a database to identify microorganisms present in the sample.
DNA/RNA sequencing
Sanger sequencing
Starting in the 1970s, the advent of DNA sequencing has significantly accelerated biological research and discovery. Traditional ‘Sanger’ DNA sequencing—or ‘1st generation sequencing’—is a PCR-based method used for determining the order of the four nucleotide bases in a molecule of DNA or RNA (Sanger & Coulsen Citation1975). The technique uses a chain-termination method that requires a single-stranded DNA template, a DNA primer, a DNA polymerase, normal dNTPs, as well as modified nucleotides (dideoxy-NTPs) that terminate DNA/RNA strand elongation during the PCR reaction. Because each of the four dideoxy-NTPs chain terminators is labelled with a fluorescent dye, emitting light at a specific wavelength, the actual sequence read is translated from peak trace chromatograms (generated by size separation, detection and recording of dye fluorescence) using capillary electrophoresis ().
Figure 5 Schematic of Sanger sequencing. Each of the four dideoxyribonucleotides (ddNTPs) chain terminators are labelled with a fluorescent dye, emitting light at a specific wavelength, the actual sequence read is translated from peak trace chromatograms (generated by size separation, detection and recording of dye fluorescence) using capillary electrophoresis.
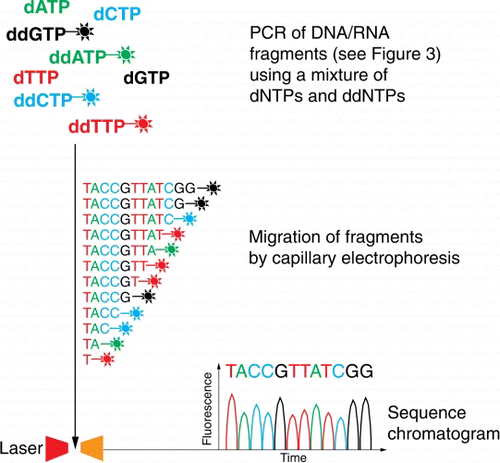
Next-generation sequencing
The recent arrival of next-generation sequencing (NGS) has drastically modified scientific approaches in basic, applied and clinical research (Ansorge Citation2009; Metzker Citation2010; Richardson Citation2010). The major advance offered by NGS is the ability to produce enormous volumes of sequence (DNA or RNA) data cheaply, allowing for rapid sequencing of entire genomes or transcriptomes, respectively. There are a wide range of applications afforded by NGS (Wold & Myers Citation2008; Petrosino et al. Citation2009; Wang et al. Citation2009). Additionally, it enables the biodiversity of complex environmental samples (e.g. soil, water, sediment, faeces, air) to be characterised rapidly (Hajibabaei et al. Citation2011; Lecroq et al. Citation2011; Anderson et al. Citation2012; Taberlet et al. Citation2012). Environmental sequences generated by NGS can be compared to a reference library in order to screen, not only for pest/introduced species or pathogens, but also for endemic species to provide a broader indication of environmental health and biodiversity (Bott et al. Citation2010).
Several NGS platforms have been commercialised, and the technology is improving at a rapid pace (Glenn Citation2011). Although available platforms utilise quite diverse chemistry and base incorporation/detection tools, they all share two main steps: library preparation (fragmentation or amplicon preparation); and detection of the incorporated nucleotide (Zhang et al. Citation2011). Available platforms differ in overall costs, duration of analysis, number of reads per run and read length, hence the choice of the platform often depends on the available budget and the scientific question under investigation (). Below, we provide a brief description of the three most commonly used NGS (i.e. ‘2nd generation’) platforms for sequencing environmental samples. However, here we do not address the 3rd generation sequencing platforms that are able to sequence individual DNA/RNA molecules in real-time (Glenn Citation2011).
Roche 454™
Roche 454™ was first commercialised in 2005. 454 uses millions of micro-beads that each hybridise with a single template, which in turn is amplified via emulsion PCR (emPCR; ). The beads are then loaded onto a picotitre plate designed so that each well can hold only a single bead. All beads are then sequenced in parallel by flowing pyrosequencing reagents across the plate, and the specific signal of incorporated nucleotides is detected in each well using a CCD camera.
Figure 6 Schematic of Roche 454™ next-generation sequencing. A PCR reaction using primers with specifically designed adaptors is undertaken. These templates are bound to beads and amplified via emulsion PCR. Each bead is loaded into a picotitre plate and sequenced in parallel by flowing pyrosequencing reagents across the plate.
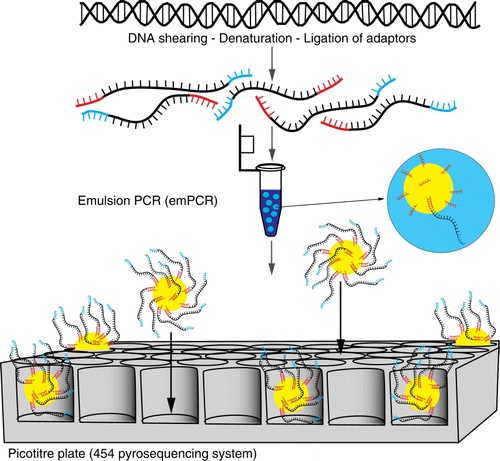
Ion Torrent™
The Ion Torrent™ is the newest and fastest NGS technology currently available. Similar to 454, Ion Torrent also relies on micro-beads and emPCR, but has pioneered an entirely new approach to sequencing using semiconductor technology and simple chemistry. Sequence data are acquired by directly sensing the ions produced by template-directed DNA polymerase synthesis on a massively parallel semiconductor sensing device. A typical 2-h run using an ion chip with 1.2 M sensors can read approximately 25 million bases (Rothberg et al. Citation2011).
Ilumina™
Previously known by the name Solexa, Illumina™ uses a solid glass surface to capture individual molecules and bridge PCR to amplify DNA/RNA into small clusters of identical molecules. The clusters are then sequenced with a similar strategy to Sanger sequencing, except only dye-labelled terminators are added, the sequence at that position is determined for all clusters, then the dye is cleaved and another round of dye-labelled terminators are added.
Applications of molecular techniques
Marine harmful algae
The seafood industry in New Zealand is expanding rapidly with the total seafood harvest in 2010–2011 being approximately 625×103 tonnes (including aquaculture; Seafood Industry Council statistics). The top ten export species in 2010 had a value of NZ$1.5 billion, with farmed green-lipped mussels (Perna canaliculus) alone earning nearly NZ$170 million that year. Closures to harvesting due to harmful algal blooms (HABs) and associated toxins are a major risk faced by the industry (Rhodes et al. Citation2012). Additionally, mass mortalities of farmed fish and wild marine fauna because of HABs have also been reported in New Zealand (Chang et al. Citation1990; Mackenzie Citation1991; Wear & Gardner Citation2001; MacKenzie et al. Citation2011a).
Monitoring of HABs in New Zealand, carried out to protect consumers and to ensure acceptance of shellfish product in export markets, began intensely in 1993 following a major neurotoxic shellfish poisoning event in the Hauraki Gulf (Jasperse Citation1993; Rhodes et al. Citation2001a). At that time regulatory closures were based on biotoxin results using a mouse assay (Rhodes et al. 2001a), but a phytoplankton monitoring programme was also established shortly thereafter. Voluntary trigger levels were developed for the known toxic phytoplankton species in New Zealand waters (i.e. cell concentrations at which shellfish harvesting would cease until shellfish flesh testing results were available). Phytoplankton monitoring is carried out using microscopy and an Utermöhl settling chamber, and considerable taxonomic experience is required to identify species (Utermöhl Citation1958; Godhe et al. Citation2007; Rhodes et al. Citation2012). Additionally, the species of interest may occur as a minor component of the plankton community, and it is often impossible to morphologically differentiate between toxic and non-toxic strains/species (e.g. Alexandrium tamarense, A. catenella, and A. fundyense; John et al. Citation2005). In recent years, the application of molecular methods for improving the detection of toxic phytoplankton species have increased as these techniques are generally rapid, species-specific and do not require specialised taxonomic expertise (Penna et al. Citation2007).
The phytoplankton monitoring programme in New Zealand is increasingly working towards a molecular-based detection system, supported by traditional morphological identification methods (Rhodes et al. Citation2012). To increase the value of the phytoplankton data for early biotoxin risk assessments and avoid costly disruptions to shellfish harvesting, the use of FISH assays () was introduced in New Zealand to differentiate between toxic and non-toxic species of diatoms from the genus Pseudo-nitzschia (Rhodes et al. Citation1998, Citation2001b). The FISH assays (which have international accreditation; NZS/ISI/IEC 17025) are undertaken by a commercial laboratory and are carried out on request if Pseudo-nitzschia concentrations increase above the agreed risk levels. Following the introduction of the assay, voluntary closures were substantially reduced due to the predominance of low risk species being present (Rhodes et al. Citation1998). The FISH assay allowed harvesting to resume within 24 hours following voluntary closures for areas that were deemed of no or low risk. When a risk is reported, harvesting would be halted until toxin clearance of shellfish was assured by chemical testing. Improvements in the chemical methods for the detection and quantification of domoic acid have resulted in the FISH assay only being used in recreational areas (dependent on the phytoplankton results) rather than commercial areas which are now regulated on the toxin concentrations in shellfish product.
SHAs (), originally developed in the US (Scholin et al. Citation1996, Citation1997, Citation2003), have been adapted for use in New Zealand for the toxin-producing species Pseudo-nitzschia australis, P. pungens and P. multiseries (Rhodes et al. Citation2001b; Tyrrell et al. Citation2002; Ayers et al. Citation2005; Rhodes et al. 2007). The first SHA was validated and internationally accredited for use in commercial laboratories in New Zealand in 2004 (NZS/ISI/IEC 17025; Ayers et al. Citation2005), but has not entered into routine commercial use (e.g. Rhodes et al. 2007) due to the high costs of consumables and insufficient detection sensitivities for some species. However, cell counts of toxic species were generally comparable for FISH, SHA and traditional microscope methods (Ayers et al. Citation2005; Godhe et al. Citation2007).
In recent years, QPCR () has been increasingly utilised for marine phytoplankton monitoring both in New Zealand and worldwide (Mackenzie et al. 2005; Dyhrman et al. Citation2006; Godhe et al. Citation2007; Zamor et al. Citation2012). During an Alexandrium catenella bloom in the Marlborough Sounds in 2011, QPCR assays developed by the Australian Water Quality Centre and the University of Tasmania (Mackenzie et al. 2005) were used to rapidly determine cell and cyst abundances (MacKenzie et al. Citation2011b). The assay has the potential to detect A. catenella at extremely low cell concentrations and track bloom development while minimising the onerous task of microscope-based cell counting. Additionally, a number of QPCR assays have been developed to distinguish morphologically similar toxic and non-toxic species present in New Zealand from the genera Karenia, Gymnodinium, Karlodinium and Takayama (Smith et al. unpublished data). These assays are routinely used for research purposes and to confirm identifications for the commercial monitoring programme (e.g. Smith et al. Citation2007). The development of a QPCR assay that targets a section of one of the genes involved in saxitoxin production is also underway (Murray et al. Citation2011).
Freshwater cyanobacteria and algae
In multiple lakes and rivers across New Zealand, cyanobacteria (blue-green algae) form planktonic or benthic blooms (Wood et al. Citation2006, Citation2007). These blooms are aesthetically unpleasant and can have detrimental effects on aquatic ecosystems through altering trophic structure and functionality (Havens Citation2008), and by decreasing water quality (Robarts et al. Citation2005). Additionally, many of the species responsible for these blooms produce natural toxins, known as cyanotoxins (Wood et al. Citation2006). These toxins are a threat to humans and animals if consumed in drinking water or contacted during recreational activities. Traditionally, cyanobacteria have been identified and enumerated using microscopic techniques (e.g. Utermöhl chambers [Utermöhl Citation1958]); however, this is time-consuming, requires specialised taxonomic skill, and cannot distinguish between toxic and non-toxic strains. Molecular methods provide promising tools for the identification and enumeration of specific genera or species and, additionally, the genes coding for the biosynthetic pathways of multiple cyanotoxins have now been elucidated (e.g. Tillet et al. Citation2000 ; Kellmann et al. Citation2008 ; Mihali et al. Citation2008), allowing for the development of PCR-based methods to detect toxin potential.
In New Zealand one of the most commonly used applications of a molecular tool is the identification of a bloom-forming species by end-point PCR () followed by Sanger sequencing (). This is usually performed on cultures which have been isolated from an environmental sample (Heath et al. Citation2010; Smith et al. Citation2011), although cloning and sequencing PCR products has also been used (Wood et al. Citation2007). The target is usually the 16S rRNA gene and a selected gene relevant to the toxin detected in the sample (e.g. sxtA as a proxy for saxitoxin production potential [Smith et al. Citation2011]; mcyE as a proxy for microcystin production potential [Wood et al. Citation2010a]). End-point PCR without sequencing has also been used to screen environmental samples for toxin potential. Wood et al. (Citation2010b) collected benthic mats samples from seven New Zealand rivers and used liquid chromatography-mass spectrometry (LC-MS) to determine the concentration of four cyanobacterial neurotoxins: anatoxin-a (ATX); homoanatoxin-a (HTX); dihydro-anatoxin-a; and dihydro-homoanatoxin-a. They also undertook PCR-amplification of a region of a polyketide synthase gene, which is involved in the biosynthetic pathway of ATX and HTX (Cadel-Six et al. Citation2009). The results from the PCR and LC-MS were in congruence in 58% of samples.
QPCR has become the preferred method for the identification and enumeration of specific species or target genes (e.g. genes involved in toxin synthesis) when monitoring cyanobacteria (Humbert et al. Citation2010). To date, despite their great potential for monitoring problematic cyanobacterial species, QPCR assays have only been used for research in New Zealand (e.g. Wood et al. Citation2010b), and have generally involved the use of assays developed overseas (e.g. Vaitomaa et al. Citation2003). One exception to this is a QPCR assay designed by Rueckert et al. (Citation2007) specifically targeting Anabaena planktonica. This species is a bloom-forming cyanobacterium that arrived in New Zealand in the early 2000s and rapidly became the dominant phytoplankton species in lakes across the North Island. The assay targets the hyper-variable region of the long intergenic transcribed spacer (ITS) and has a lower detection limit of at least five cells per sample. A comparison with samples collected from the Lower Karori Reservoir (Wellington, New Zealand) showed that cell concentrations determined by QPCR correlated significantly (95% confidence interval) with those determined by microscopy. For both end-point and QPCR assays the majority of this work has been undertaken for research or ‘proof of concept’ studies and has not yet been adopted into routine analysis.
Several issues have limited the uptake of QPCR assays for monitoring cyanobacteria in New Zealand. One problem is that most blooms are comprised of multiple species and the New Zealand Guidelines for Managing Cyanobacteria in Recreational Fresh Waters (Ministry for the Environment and Ministry of Health Citation2009) rely on enumeration and speciation of all cyanobacterial species, which is generally not possible unless many QPCR assays are run per sample. Another limitation of QPCR assays targeting only DNA is that they provide information about the toxin potential of the species and not actual toxin levels which are required by guidelines (Ministry for the Environment and Ministry of Health Citation2009). Toxin quotas (the amount of toxin produced per cell) of some species can change rapidly over relatively short time spans (Wood et al. Citation2012), and thus the numbers of copies of toxin genes may not provide an indication of actual risk. Rueckert and Cary (Citation2009) developed a reverse transcriptase-QPCR assay to monitor mcyE gene transcripts in Microcystis. One potential advantage of a reverse transcriptase-QPCR is that it is able to determine if the genes are present and also assess whether they are expressed (i.e. toxins are being produced). Their assay incorporates a ribonuclease-resistant armoured-RNA internal standard, which consists of a MS2 bacteriophage virus in which the genome has been altered to harbour the mcyE gene, with a unique internal probe-binding sequence for its differential detection. The advantage of this design is that target and standard sequences are virtually identical, resulting in both sequences undergoing reverse transcription and amplification with the same efficiency. Although this assay has the potential to be used as a monitoring tool, the additional steps required in the sample preparation protocol mean that it would not currently be cost-effective. The assay has recently been used to demonstrate for the first time that Microcystis sp. can ‘switch’ toxin production on and off during a bloom, with a > 400-fold change in mcyE gene expression and an approximate 20-fold change in microcystin quota measured over a 5-h period (Wood et al. Citation2010c). The expense of this assay prevents its routine use for commercial purposes and it is currently only used for research.
Cyanobacterial specific primers have been designed for an ARISA () assay (Wood et al. Citation2008b). The potential of the method for monitoring was assessed using samples from three eutrophic New Zealand lakes. When cell concentrations were > 200 cell mL−1 the ARISA reliably detected key problematic species. However, in many instances the correlation between fluorescence and actual cell counts was weak (Wood unpublished data). Using cyanobacterial cultures it was shown that changes in species composition caused shifts in relative fluorescence (which is sometimes used as a proxy for biomass) and that significant PCR biases, probably due to preferential amplification of some species because of differences in primer affinities, were evident (Wood unpublished data). Despite these limitations, ARISA is a useful screening tool that can be used to identify differences in community composition among samples (Wood et al. Citation2010a). With the use of an ITS ‘library’, specific species can be identified in the profiles (Wood et al. Citation2008a). For example, Wood et al. (Citation2008a) used 16S rRNA gene sequence and ITS clone libraries to identify the length of the ITS region for species in selected samples. They then used this information to infer the species composition of other environmental samples collected in close proximity. Evaluation of a NGS method that uses an approximately 300 bp fragment of the 16S rRNA gene and a multiplexing approach on the Ion Torrent™ is underway (Wood unpublished data).
The invasive single-celled diatom Didymosphenia geminata is the only freshwater algae for which a molecular monitoring tool is used extensively in New Zealand. Over the last two decades there has been a worldwide increase in the distribution and severity of blooms of D. geminata. This species has a unique ability to secrete unprecedented masses of mucilaginous stalks which forms mats that can severely impact native species and ecosystem functioning (Kilroy et al. Citation2009). Didymosphenia geminata was first detected in New Zealand in 2004 in the Waiau River (South Island; Kilroy Citation2004). Since its initial detection, D. geminata has spread throughout the South Island with more than 280 rivers now infested (www.didymosamplesdb.org.nz/). Didymosphenia geminata is usually identified by microscopic methods which are time-consuming, resource intensive, and dependent upon expert taxonomic identification, thus the extent of surveillance programmes was limited. Cary et al. (Citation2007) developed a TaqMan QPCR assay targeting a region of the 18S rRNA gene. Since 2007 a range of monitoring agencies (e.g. Regional Councils, hydroelectricity companies) have used the QPCR assay for delimitation surveys in North Island, New Zealand. Laboratory dilution experiments confirmed a lower detection limit of approximately two D. geminata cells per PCR reaction. A plankton net protocol (Cary et al. Citation2007) is recommended for collecting river samples for this QPCR assay and during a field trial positive results were obtained for samples with c. 60 cell m−3 (Cary unpublished data).
Marine invasive species
The introduction of a new species can dramatically modify indigenous biodiversity and ecosystems (Galil Citation2007; Wallentinus & Nyberg Citation2007; Sousa et al. Citation2009; Ehrenfeld Citation2010). The altered community may undergo degradation of associated ecological, economic and social values (Ehrenfeld Citation2010). As a consequence, the prevention of ecological invasions has become a priority for many governments, especially in island nations such as New Zealand (Meyerson & Reaser Citation2002; Hewitt et al. Citation2004). New Zealand has a targeted surveillance programme for the six marine pests that are currently listed on the New Zealand Register of Unwanted Organisms under the Biosecurity Act 1993, including: the European shore crab (Carcinus maenas); Chinese mitten crab (Eriocheir sinensis); Northern Pacific seastar (Asterias amurensis); Mediterranean fanworm (Sabella spallanzanii); Asian clam (Corbula amurensis); and the marine aquarium weed (Caulerpa taxifolia). Of these species, S. spallanzanii is already present in New Zealand (Read et al. Citation2011). Surveys are conducted twice yearly at 12 high-risk locations throughout New Zealand using SCUBA-diving transects, shore searches, crab condos, starfish traps and benthic sleds (Acosta & White Citation2011).
The detection of an invasive species soon after an incursion, when the population is still confined to a small area and at a low density, maximises the probability of effective management (e.g. the eradication of the black-striped mussel (Mytilopsis sallei) from Darwin, Australia; Simberloff Citation2001). Early detection of invasive species has in the past been problematic because morphological identification of the various life-history stages of different marine organisms cannot be easily determined (Darling & Blum Citation2007). Additionally, morphological identification in the marine environment may occur under difficult conditions (e.g. visual surveillance by snorkel or SCUBA in low visibility waters; Hayes et al. Citation2005). Consequently, there have been few surveillance programmes in which the water column has been monitored for dispersive life forms (e.g. planktonic larvae) of invasive species. The development of molecular-based methods that target dispersive life stages of marine organisms in the water column is now seen as an effective strategy for detecting marine invasive species in surveillance. In recent years a number of molecular methods have been developed to target different high profile marine pests (e.g. Asterias, Deagle et al. Citation2003; a range of marine invertebrate larvae, Jones et al. Citation2008; a range of bivalves and crustacean larvae and algae, Harvey et al. Citation2009; Asterias amurensis, Mountfort et al. Citation2007, Smith et al. Citation2011). Molecular techniques have the potential to be faster, more specific, and have greater standardisation compared with morphological analysis. Molecular methods also reduce the problem of a growing shortage of specialist taxonomists (Kim & Byrne Citation2006).
In New Zealand, RNA-targeted hybridisation assays such as FISH and SHA (, ) were initially trialled as tools for marine invasive species surveillance, specifically for the detection of species from the Register of Unwanted Organisms (Mountfort et al. Citation2007, Citation2012; Smith et al. Citation2011, Citation2012a). Both FISH and SHA assays have been developed for the Northern Pacific seastar (Asterias amurensis; Mountfort et al. Citation2007; Smith et al. Citation2011). A major advantage of these methodologies is the potential for simultaneous detection of a variety of target sequences in near real-time without some of the issues that occur with nucleic acid amplification, such as inhibition and the bias associated with PCR-based methods (Darling & Mahon Citation2011). The methods are also very simple and highly amenable to automation. However, in order to detect low abundance targets, such as planktonic larvae, more sensitive methods are generally required. Recently, QPCR () assays have either been developed (Smith et al. 2012a) or optimised for use in New Zealand (Bax et al. Citation2006; Kuhajek et al. Citation2011). A QPCR assay designed to detect the Asian clam (Corbula amurensis) showed high specificity and could successfully detect C. amurensis larvae from a range of environmental sample types (Smith et al. 2012a). This assay has the potential to be implemented in surveillance programmes for marine pests, and would enhance current monitoring methods, especially with regard to the detection of early life stages. The importance of specificity testing against New Zealand native species was highlighted by the optimisation of an assay for the Mediterranean fanworm (Sabella spallanzanii) developed in Australia. The assay cross-reacted with at least two New Zealand native sabellids and, if this had not been identified before the assay had been adopted into routine surveillance, false positives may have resulted in additional and costly follow-up sampling and analysis (Kuhajek et al. Citation2011). Despite the suite of tools that have been developed or validated for use in New Zealand, none of the above are currently used during the analysis of samples collected during the routine marine bioinvasives port surveys.
Another application of molecular methods to marine invasive species has been the identification of new incursions by DNA sequencing () (D'Archino et al. Citation2007; Smith et al. Citation2003, Citation2007, Citation2012b). Genetic identification of species is also known as ‘DNA barcoding’ (Hebert et al. Citation2003) and its use has greatly aided management decisions in New Zealand. For example, the identification by DNA sequencing of the invasive South African brown mussel (Perna perna) that arrived on an oil rig in Tasman Bay for defouling led to seafloor dredging to remove any mussels that may have dropped to the seafloor (Hopkins & Forrest Citation2010).
Next-generation sequencing () has made it possible to bypass the expensive and time-consuming step of cloning and Sanger sequencing of PCR products (Taberlet et al. Citation2012). Research underway at the Cawthron Institute (Nelson, New Zealand) is focused on evaluating the detection limits of NGS for the surveillance and monitoring of multiple international marine pests using a multiplexing approach on a Roche 454™ GS Junior pyrosequencer (Pochon unpublished data). Our preliminary results indicate that it is possible to detect a single Asterias larva when it is spiked into a c. 0.3 mg of sediment or concentrated (>2000 organisms per mL) plankton sample using pyrosequencing (Pochon unpublished data). Despite some significant technical challenges associated with these techniques (Shokralla et al. Citation2012), NGS technologies provide unprecedented opportunities for the development of innovative diagnostic tools able to detect multiple species from a variety of life stages in environmental samples, and for the early detection, management and prevention of invasive species worldwide.
Freshwater biotic indices
Biotic indices have been developed to provide information on the health of an ecosystem over an extended time period, rather than the one time-point information obtained by sampling for chemical (e.g. nutrients) or physical data (e.g. temperature). The most commonly used biotic index in New Zealand's freshwater environments is the Macroinvertebrate Community Index (MCI; Stark Citation1985). The MCI uses the presence of a range of macroinvertebrates (e.g. mayflies, caddisflies, snails) to assess stream/river health. Most macroinvertebrates possess a life cycle of at least a year, do not travel large distances, and are confined to relatively small areas of a water body, thus their communities provide an indication of stream/river health over an extended time period. The MCI has been a mainstay of Regional Councils’ State of the Environment monitoring programmes throughout New Zealand for over 20 years. However, it is reliant on morphological identifications, which are time-consuming, expensive and dependent on specialist knowledge. Its requirements for the detection of multiple species simultaneously, and the need for only presence/absence data, make the MCI very amenable to the development of a molecular-based tool using a NGS approach.
Most macroinvertebrates in New Zealand are endemic and, until recently, there has been limited sequence information available on worldwide databases. Researchers have now characterised approximately 50% of the diversity of New Zealand freshwater macroinvertebrates by sequencing an approximately 650 base-pair region of the mitochondrial cytochrome c oxidase subunit 1 (CO1; Olsen et al. Citation2007; Hogg et al. Citation2009; McCulloch Citation2011). The CO1 gene has been widely used for barcoding a large diversity of organisms. As of 2012, the barcode of life CO1 database (www.boldsystems.org) contains sequences for more than a million specimens from over 100,000 species of animals, more than is available for any other gene. A research programme underway jointly between the Cawthron Institute and the University of Waikato (Hamilton, New Zealand) is evaluating the feasibility and detection limits of using NGS to develop a molecular MCI using a multiplexing approach on the Ion Torrent™. Pilot trials using contrived communities are underway, with plans to test environmental samples in the summer of 2012–2013.
A similar NGS approach is being developed for measuring lake heath using rotifers. Duggan et al. (Citation2001a, Citationb) found that lake trophic state was the major determinant of rotifer distribution among North Island lakes, and developed a biotic index of lake trophic state using rotifer community composition characterised by morphological identifications. The Rotifer Trophic Lake Index correlated closely with the Trophic Level Index (TLI) developed by Burns et al. (Citation1999) from physical and chemical data. The advantage of the rotifer-based index is that it integrates water quality over time. Scientists at Waikato University have sequenced a region of the CO1 for most rotifer species in New Zealand, and have explored several techniques including T-RFLP for the development of a molecular method to determine rotifer diversity (Banks et al. Citation2010a). They are currently experimenting with a NGS approach using the Ion Torrent™.
The Bacterial Community Index (BCI) for assessment of stream health has recently been developed using the ARISA method (; Lewis et al. Citation2011). The ease of processing and analysis of biofilm samples makes the BCI a potentially cheap and effective way to analyse large sample sets and provide an additional measure of stream health and insights into base-level carbon and nutrient cycling in stream/river ecosystems. The BCI was established by using ARISA to characterise bacterial biofilm communities in 1026 samples collected from 254 streams representing a variety of regions and land uses throughout New Zealand. When the ARISA data were compared with land use or water quality data, ARISA did not predict stream health. However, when analysed in conjunction with macroinvertebrate index data, a predictive model to estimate the BCI could be developed (Lear et al. Citation2009; Lear & Lewis Citation2009; Lewis et al. Citation2011). Potentially, the BCI could provide a rapid and cost-effective approach to monitoring stream/river health in New Zealand. Further investigations are required to determine the robustness of the BCI between years, establish exactly how the BCI relates to ecosystem health and enhance the sensitivity of BCI at the extremes of the index (Lewis et al. Citation2011).
Non-indigenous fish
Worldwide, there are numerous examples of the transfer of fish to exotic habitats having unanticipated effects resulting in the deterioration of habitats (Vitousek et al. Citation1997). The introduction of new species into freshwater habitats has had detrimental effects on physical properties such as water quality and adversely affected other species (e.g. native freshwater fish; McDowall Citation1990), as well as introducing pathogens and parasites to new areas (McDowall Citation2000, Citation2004; Townsend Citation2003). New Zealand is no exception, with approximately 21 species of introduced fish present in freshwaters (McDowall et al. Citation2000), many of which have resulted in a suite of adverse effects. These range from minor effects, as in the case of the sailfin molly (Poecilia latipinna) with its limited distribution to geothermal areas around the southern end of Lake Taupō (McDowall 1990), through to major adverse effects, such as reduced diversity of native fish and zooplankton (Townsend 2003). For example, koi carp (Cyprinus carpio) in the Waikato area, are associated with increased turbidity, reduced macrophyte diversity and increased algal concentrations, probably through their benthic feeding uprooting macrophytes and resuspending nutrients from the sediments into the water column (Lougheed et al. Citation1998). The relatively low diversity of New Zealand freshwater ecosystems may increase their vulnerability to the adverse effects of introduced species (Townsend & Winterbourn Citation1992).
The first stage of reducing the risk of adverse effects from the introduction of fish is comprehensive biosecurity at borders. Currently, 1010 species of freshwater fish are permitted to be imported into New Zealand under the 2011 Import Health Standard for Ornamental Fish and Marine Invertebrates from All Countries. However, the identification of fish, especially larval fish, can be difficult because of the lack of identifying morphological features, and there is evidence that unapproved species have been imported into New Zealand in the past (McDowall 2004). Molecular tools can improve biosecurity by ensuring the accurate identification of imported fish. With the development of genetic databases such as Genbank (Benson et al. Citation2009) and the Barcode of Life database (BOLD; Ratnasingham & Hebert Citation2007), it is relatively simple to sequence a reference gene such as CO1 and compare the sequence obtained to the sequence on file for a voucher specimen. Identifying sequences can be obtained from specimens of any age including larval fish (Collins et al. Citation2012; Banks et al. Citation2010b), mutilated specimens (Banks et al. Citation2010b) and even from carcasses that are several months old (Banks et al. Citation2010b). One particularly interesting application of molecular techniques to biosecurity issues was the identification of fish through analysis of DNA shed into water in aquaria (Knox et al. 2009) or, in the case of imported fish at the border, into plastic bags being used to transport fish (Collins et al. 2012), allowing non-invasive testing of fish before release from quarantine. Although these studies have demonstrated the applicability of the methods, they are not currently being used for routine biosecurity surveillance.
Molecular tools have also been applied to the containment and eradication of the unwanted fish species. Eradication is made easier by early detection and accurate data on the extent of an introduced species’ range (Myers et al. Citation2000; Wittenberg & Cock 2001). Additionally, detecting surviving pockets of individuals allows the targeting of control measures (Myers et al. Citation2000; Wittenberg & Cock Citation2001), but determining the distribution of introduced fish within a defined area, and effective evaluation of eradication efforts, are significant challenges to removing fish from waterways. Traditionally, fish distributions have been determined using visual methods such as spotlight surveys and/or capture-based methods, such as electric fishing, gill nets and traps. The effectiveness of these methods is affected by the behaviour and habitat of the target species; for example, electric fishing is relatively ineffective in deeper water. Environmental DNA (eDNA) is a promising method to detect the presence of introduced aquatic species (Ficetola et al. Citation2008; Jerde et al. Citation2011). In the case of freshwater vertebrates, DNA from material such as sloughed skin cells or gut epithelium is likely to be present in the environment and has been used to infer the range of introduced Asian carp (Hypophthalmichthys molitrix) and bighead carp (H. nobilis; Jerde et al. Citation2011), frogs and salamanders (Goldberg et al. Citation2011), and six species of fish in the Yura River, Japan (Minamoto et al. Citation2011). Similar techniques have also been used to characterise marine fish fauna (Thomsen et al. Citation2012).
In New Zealand, the feasibility of using eDNA, for example from water samples, to detect introduced fish such as koi carp and goldfish has been investigated (Knox et al. Citation2009). DNA from goldfish was successfully amplified from water samples taken from aquaria and from faecal samples obtained from fish in aquaria. The suitability of several regions of the mitochondrial genome was evaluated for the detection of fish from environmental samples, including portions of the 12S and 16S ribosomal RNA genes, CO1 gene and the mitochondrial d-loop region. All regions showed suitable levels of variation among species, but the d-loop region was ruled out because of the likely lack of sites for ‘universal’ primers (primers that would amplify a wide range of fish species). Of the other three genes evaluated, CO1 was selected as more reference sequences were available on publicly accessible databases such as Genbank (Benson et al. Citation2009) compared with the number of sequences available for the 12S and 16S rRNA genes.
The usefulness of eDNA was also recently evaluated during the eradication of brown trout (Salmo trutta) from the Zealandia Sanctuary in Wellington, New Zealand as part of a project to restore the fauna in the sanctuary (Banks unpublished data). Using species-specific primers, PCR product specific to brown trout were amplified from water samples taken immediately before the application of rotenone (the poison used to kill the brown trout) to some of the sanctuary's streams, but not in samples collected three months after the dosing. This suggested that the use of eDNA will be a useful tool for monitoring the success of remedial actions.
There are a large number of potential uses for eDNA from biosecurity at the border through to assessing the effectiveness of projects to remove introduced fish from different ecosystems. A particular advantage of eDNA over traditional methods is the removal of risks to non-target species that occur with netting and electric fishing. Future work includes assessing the rates of false positives (a positive PCR but the target species is not present) and false negatives (failure to detect the target species when it is present), the minimum detectable biomass in a water body, and the effect of differences in physical parameters such as water chemistry and turbidity on the detectability of introduced fish.
Ecotoxicology
Ecotoxicology assesses the risk of environmental stressors of anthropogenic origin on the receiving environment. In New Zealand, this field was poorly developed until toxicity testing using whole animal protocols was introduced in 1986 (Hickey Citation1995). Since then, more sophisticated methodologies to understand the effects of environmental contaminants have been used, including biomarkers. Biomarkers are direct indicators of chemical exposures usually at the biomolecular and biochemical levels of biological organisation which typically display a range of specificities and sensitivities to stressors (Adams & Tremblay Citation2003). Biomarkers are playing an increasingly important role in ecological risk assessment frameworks and they are being incorporated into effects-based regulations such at the Resource Management Act 1991 (Adams et al. Citation2001). Biochemical biomarkers are based on variations of protein concentrations or enzymatic activities, and increasingly are measured by the amount of mRNA coding for these proteins or enzymes by reverse transcriptase-QPCR (Pina et al. Citation2007).
Evans et al. (Citation2001) developed a quantitative competitive (qc) reverse transcriptase-PCR for a metallothionein (MT) in the yellowbelly flounder (Rhombosolea leporine). Metallothioneins are low molecular-weight cytoplasmic proteins that act as biological chelators of metals and are well-established biomarkers (Adams et al. Citation2001). The assay was validated on liver tissues from fish sampled in the Auckland region and showed that the level of mRNA was correlated with the level of zinc and copper residues in the liver (Evans et al. Citation2001). Another MT reverse transcriptase-QPCR method was developed for the common bully (Gobiomorphus cotidianus) and the assay showed promise as an indicator for copper toxicity (Laurie Citation2004). A reverse transcriptase-QPCR method was developed for the vitellogenin (Vtg) in the exotic mosquitofish (Gambusia affinis; Leusch et al. Citation2005). Vitellogenin is a glycolipophosphoprotein precursor to egg yolk produced in the liver of mature female fish under oestrogenic stimulation. It can be induced in males exposed to endocrine-disrupting chemicals with oestrogenic activity. The assay has been successfully used in field studies in New Zealand (Tremblay unpublished data) but is yet to be adopted for analysis of routine commercial samples.
Amplified fragment length polymorphisms (AFLPs) fingerprinting was used to assess the effects of pulp mill effluent on the common bully G. cotidianus (van den Heuvel et al. Citation2007). The genetic structure indicated highest posterior probability for three genotypes within common bully sampled from sites receiving various levels of contaminants. However, it was not possible to correlate genetic differences with anthropogenic stressors. In an algal study, identification of strains was based on morphological observations and gene sequences (Novis et al. Citation2009). The approach was to partially sequence the rbcL gene, a commonly used gene in plants with a large database of algal strains, to identify algae isolated in environments receiving various levels of contamination. The approach successfully identified that higher levels of stressors lead to more resistant strains, which could be due to natural selection. Molecular techniques are increasingly used in ecotoxicology and generate data that will greatly improve environmental risk assessment processes.
Microbial source tracking
Currently, the risk of humans developing infectious diseases from contact with contaminated water or eating contaminated seafood is assessed by measuring the concentrations of faecal indicator bacteria (FIB; Griffin et al. Citation2001; Scott et al. Citation2002). FIB are commonly used as proxies for pathogen concentrations as these bacteria are generally not pathogenic in themselves, but their concentrations are indicative of the degree of contamination by faecal material (Griffin et al. Citation2001). The advantages of testing for indicator microorganisms are that the tests are cheap, widely recognised by regulatory authorities around the world (Griffin et al. Citation2001), and the organisms are present in faecal material at much higher concentrations than pathogens (Field & Samadpour Citation2007). Indicator organisms are also monitored as the actual pathogens are often rare, difficult to culture, have patchy distributions and can be infectious at low concentrations (Field & Samadpour Citation2007).
There are, however, several disadvantages of using FIBs (e.g. Escherichia coli and Enterococcus) for monitoring infection risk. The ideal indicator microorganism should give an accurate signal of the risk of developing disease, be non-pathogenic itself and host specific, not persist in the environment longer than the pathogens of interest, and not replicate in the environment to avoid giving misleadingly high estimates of the risk (Griffin et al. Citation2001). The ubiquity of E. coli in warm-blooded vertebrates (Souza et al. Citation1999) means that it does not provide any information on the source of contamination (Field & Samadpour Citation2007). Additionally, FIBs such as E. coli can replicate in the environment under some conditions (Byappanahalli et al. Citation2003, Citation2006) resulting in an overestimation of the persistence of faecal contamination.
Microbial source tracking (MST) provides a method to identify contaminant sources contributing to water quality degradation. MST uses DNA extracted from environmental samples, such as water or shellfish tissue, as the template for either end-point PCR or QPCR (; Scott et al. Citation2002). The advantage of MST over FIB is that it is possible to use primers that only amplify DNA from host-specific bacteria, thus allowing identification of the source of the faecal contamination (Field & Samadpour Citation2007) and prioritisation of mitigation measures. One group of bacteria that shows promise for identifying the source of faecal contamination is the order Bacteroidales, which live in the gastrointestinal tracts of animals. Many of the bacteria from the Bacteroidales order are host-specific and the use of primers that only amplify DNA from a specific host allows identification of the source of the faecal contamination. Bacteroidales are obligate anaerobes so do not replicate outside of the gut and their abundance is correlated with human enteric viruses (Jofre et al. Citation1989). However, maintaining the anaerobic conditions needed to culture Bacteroidales limited their usefulness for routine monitoring of water quality until the development of molecular methods of identifying bacteria removed the need for culture-based assays (Scott et al. Citation2002).
To date, most of the MST undertaken in New Zealand has used assays developed overseas. For example; Kirs and Cornelisen (Citation2011) found that concentrations of Bacteroidales DNA extracted from the digestive gland of green-lipped mussels (Perna canaliculus) and measured by QPCR were significantly correlated with concentrations of E. coli. They were also able to detect the DNA from human- and ruminant-derived Bacteroidales in green-lipped mussel tissue. MST using primers that amplified host-specific Bacteroidales identified a human origin for the faecal contamination of the lower Maitai River, Nelson, New Zealand (Kirs et al. Citation2008, Citation2011). Similarly, Moriarty and Gilpin (Citation2009) used MST to attribute the source of persistently high concentrations of faecal indicator bacteria in the Avon River, Christchurch, New Zealand, to dogs and wildfowl, and suggested measures to reduce the contamination. In 2011, a large validation study was undertaken in conjunction with water quality monitoring programmes for nine Regional Councils around New Zealand, involving the collection of over 200 water samples (Cornelisen et al. Citation2012). The samples were analysed using QPCR for a universal Bacteroidales marker (UBac), a Bacteroidales marker specific to humans (HBac), a Bacteroidales marker associated with ruminant animals (RBac) such as sheep and cows, and a bovine Bacteroidales marker (BBac) specific to cows. The UBac marker was detected in all but one sample and there was a relatively poor correlation between concentrations of the UBac marker and those of enterococci measured from the same water samples. The RBac marker was detected in approximately half (51%) of the water samples, indicating pastoral farming is a major driver of faecal contamination in many of the areas sampled. The more specific bovine BBac marker was far less prevalent and was detected in only 4% of the water samples. The human HBac marker was detected in only 6% of the samples, probably reflecting the efficacy of treatment of human-sourced wastewater.
An alternative to using non-pathogenic indicator microorganisms to assess water quality is to monitor the concentrations of the actual pathogens. For example, QPCR identified contaminated drinking water as the source of a norovirus outbreak at a New Zealand ski field (Hewitt et al. Citation2007) and QPCR was used to identify contaminated oysters imported from Korea as the source of a norovirus outbreak at a New Zealand–Ireland rugby test match in 2007 (Simmons et al. Citation2007). Directly monitoring for human pathogenic viruses is beneficial as the viruses can be present when faecal indicator bacteria are not detected (Lipp et al. Citation2001; Girones et al. Citation2010). However, the distribution of viruses can be patchy and virus concentrations can be below detectable limits and yet still sufficiently high to cause infections (Girones et al. Citation2010). An additional complicating factor is that concentrations of viruses in water calculated by QPCR can vary depending on the DNA extraction method, type of environmental sample and the presence or absence of chemicals inhibiting PCRs that can be extracted in conjunction with the DNA (Girones et al. Citation2010).
As the extent of wastewater treatment in New Zealand has increased (Ministry for the Environment Citation2007x), many of the easily identifiable point sources of pollution have been rectified. The challenge now lies in identifying non-point sources of water contamination, such as leaks from sewerage systems and farm run-off which are generally diffuse and often intermittent (Girones et al. Citation2010). A combination of routine MST-based molecular methods to identify host-specific indicator bacteria and monitoring for the pathogenic microorganism is likely to become more widespread because of the increased information obtained in comparison with that from traditional faecal indicator bacteria such as E. coli. A range of end-point and QPCR assays for a variety of MST markers are offered commercially by several laboratories in New Zealand. These services are being used regularly by Regional Councils, shellfish farmers and other water managers.
The future
Despite research and validation in New Zealand demonstrating their potential, the uptake of molecular tools by agencies monitoring aquatic systems is relatively limited. The areas with the greatest use occur when the techniques have been thoroughly validated and a level of accreditation achieved (e.g. the Pseudo-nitzschia FISH assays which have international accreditation; NZS/ISI/IEC 17025), or where the tool provides additional information that cannot be obtained by a ‘traditional’ technique (e.g. the identification of the source of bacteria by MST). Identification of individuals by DNA sequencing or barcoding is relatively straightforward, but the technical challenges associated with detecting target species in environmental samples are significantly greater (Darling & Blum Citation2007; Bott et al. Citation2010). Even if technically feasible, the logistical, economic and political barriers to implementation are great (Darling & Mahon Citation2011). Additionally, due to the greater sensitivity of molecular methods it is often impossible to confirm positive results with traditional methods (Darling & Mahon Citation2011). A further barrier to uptake is that there is some reluctance to change methodologies by monitoring agencies; in many cases this is understandable as long-term datasets exist. However, we anticipate that over the next 5–10 years the uptake of molecular tools will markedly increase as water managers become more familiar with the methodologies and the advantages they confer. The current cost of sample preparation and analysis, which in some cases is only slightly less than traditional techniques, may also limit use of these methods. Costs can be reduced by analysing large numbers of samples which enables the high-throughput capabilities of some techniques to be utilised. At present QPCR, because of its high-throughput capabilities (30–380 samples simultaneously, depending on the instrument used) is the most cost-effective and rapid method. The main limitation of QPCR is that only a few (usually no more than three) targets can be detected simultaneously.
Recent advances in microarray technologies have provided several potential tools for the monitoring of multiple targets at once. For example, Gescher et al. (Citation2008) and Ki and Han (Citation2006) have developed microarrays for toxic marine phytoplankton and Castiglioni et al. (Citation2004) for cyanobacteria. Microarrays are limited to detection of the targets that they are designed for and we anticipate that for environmental monitoring purposes they will be superseded by NGS. Over the past 5–7 years NGS has dramatically changed biological sciences. Using NGS it is now possible to recover thousands of DNA (or RNA) sequences directly from environmental samples (e.g. Sogin et al. Citation2006; Stoeck et al. Citation2010). The practical applications of NGS in biomonitoring are still relatively limited, although several studies have demonstrated its enormous potential in applications similar to those used for biomonitoring in New Zealand. For example, Hajibabaei et al. (Citation2011) used 454™ pryosequencing to monitor macroinvertebrates in Canadian rivers. It is currently still relatively expensive to analyse samples via NGS but costs can be reduced through multiplexing capabilities, which are continually improving. For example, Life Technologies (suppliers of the Ion Torrent™) have developed barcode sequences for up to 96 samples which, based on New Zealand prices in 2012, could reduce consumable costs to less than NZ$20 per sample. As specialised bioinformatics, algorithms for data analysis, and techniques to visualise outputs improve, biomonitoring using NGS will become faster and cheaper, and we speculate that within the next decade NGS will play a significant role in many aquatic monitoring programmes in New Zealand. Our review shows a move to molecular-based monitoring with many projects assessing these techniques underway (e.g. marine bioinvasives, rotifers, macroinvertebrates and cyanobacteria).
Regardless of the technique used, the application of molecular tools for the detection of aquatic organisms usually involves the collection of samples and their transportation back to a laboratory for processing and analysis. This can result in delays between collection and analysis ranging from hours to days. Several ‘portable’ PCR instruments (e.g. Agrawal et al. Citation2007) have been developed that may enable analysis to be undertaken at the sampling site, although the DNA extraction step and prevention of contamination while working in the field remain a challenge.
One solution may be the development of remotely operated, semiautonomous instruments capable of carrying out DNA extractions from environmental samples such as water and then conducting QPCR and/or hybridisation assays. Researchers from the Cawthron Institute in collaboration with scientists from the Monterey Bay Aquarium Research Institute (MBARI) and Stanford University, CA, recently trialled an environmental sample processor (ESP; Scholin et al. Citation2009) developed by a team of scientists and engineers at MBARI. The ESP is a robotic underwater molecular biology laboratory moored in the open ocean that automatically collects water samples and detects the presence of specific organisms from PCR amplification of their DNA and by hybridisation assays. Results are transmitted back to the laboratory in real-time via radio or cellular communications. The ESP also measures environmental parameters such as water temperature, salinity and turbidity. Laboratory and field trials have demonstrated near-real time detection of bacteria, harmful algal bloom species, the algal biotoxin domoic acid and invertebrates (Greenfield et al. Citation2008; Jones et al. 2008; Preston et al. 2011; Ryan et al. Citation2011; Harvey et al. Citation2012). The MBARI ESPs have been trialled in many coastal waters including Monterey Bay, Florida, Massachusetts and Hawaii. The trial in New Zealand was the first time an ESP has been deployed in the southern hemisphere. It was situated in Tasman Bay for four weeks during May and June 2012 and focused on targeting organisms that are a significant problem for the aquaculture industry, including harmful microbes and algal bloom species.
Traditionally, the ESP has employed SHA arrays that are very robust and allow the simultaneous detection of tens to hundreds of unique organisms in a single sample (Greenfield 2008; Harvey et al. 2012). However, this method is not very sensitive and may miss the detection of harmful microbes at low densities. Recently, QPCR assays have been adapted for the ESP (Preston et al. Citation2011) allowing the detection of organisms at extremely low concentrations providing early warning of contamination and bloom events. Equipment like the ESP, which incorporates molecular techniques to assess biological parameters, could be part of a broader network for monitoring the state of New Zealand's coastal waters or freshwater systems such as lakes. These could form a nationwide network providing long-term environmental and biological data to a range of end users.
Molecular tools have played, and will continue to play, a major role in monitoring aquatic environments in New Zealand. New Zealand has been relatively active in developing and adapting overseas tools; however, this has not necessarily resulted in the uptake of these tools for routine monitoring. In the forthcoming decades we speculate that the use of molecular tools by regulatory agencies and industry for monitoring aquatic environments will increase markedly. When information on multiple targets is required, we believe that NGS will become the preferred method. Although the ESP can also detect multiple targets, the capital costs and time involved in their deployment is likely to limit wide-scale uptake of these technologies in the near future. Robotic technology will continue to improve and, by using ESPs or similar, real-time data from a network of sites may ultimately be obtainable. For highly quantitative, single-target assays, QPCR is likely to remain the ‘gold standard’. In addition, we suggest that there is substantial scope for the application of molecular tools for monitoring aquatic environments that have not yet been considered, such as assessing the environmental impact of shellfish farms, mining sites, ocean outfalls and dredge disposal sites.
Acknowledgements
This work was funded by the New Zealand Ministry of Ministry of Business, Innovation & Employment funded programmes C01X0502, CAWX0703, CAWX0804, UOWX0505. We thank the New Zealand Ministry of Environment sustainable management fund and Australian Research Council for their support of the ecotoxicology research.
References
- Acosta , H and White , P . 2011 . Atlas of biosecurity surveillance , 51 Wellington , , New Zealand : Ministry of Agriculture and Forestry .
- Adams , SM and Tremblay , LA . 2003 . Integration of chemical and biological tools in environmental management and regulation . Australasian Journal of Ecotoxicology , 9 : 157 – 164 .
- Adams , SM , Giesy , JP , Tremblay , LA and Eason , CT . 2001 . The use of biomarkers in ecological risk assessment: recommendations from the Christchurch conference on biomarkers in ecotoxicology . Biomarkers , 6 : 1 – 6 . doi: 10.1080/135475001452724
- Afonina , IA , Reed , MW , Lusby , E , Shishkina , IG and Belousov , YS . 2002 . Minor groove binder conjugated DNA probes for quantitative DNA detection by hybridization-triggered fluorescence . Biotechniques , 32 : 940 – 949 .
- Agrawal , N , Hassan , YA and Ugaz , VMA . 2007 . Pocket-sized convective PCR thermocycler . Angewandte Chemie International Edition , 46 : 4316 – 4319 . doi: 10.1002/anie.200700306
- Andersen , K , Bird , KL , Rasmussen , M , Haile , J , Breuning-Madsen , H , Kjaer , KH , Orlando , L , Gilbert , MT and Willerslev , E . 2012 . Meta-barcoding of ‘dirt’ DNA from soil reflects vertebrate biodiversity . Molecular Ecology , 8 : 1966 – 1979 . doi: 10.1111/j.1365-294X.2011.05261.x
- Ansorge , WJ . 2009 . Next-generation DNA sequencing techniques . New Biotechnology , 25 : 195 – 203 . doi: 10.1016/j.nbt.2008.12.009
- Ayers , K , Rhodes , LL , Tyrrell , JV , Gladstone , M and Scholin , CA . 2005 . International accreditation of sandwich hybridisation assay format DNA probes for micro-algae . New Zealand Journal of Marine and Freshwater Research , 39 : 1225 – 1231 . doi: 10.1080/00288330.2005.9517388
- Banks JC , Duggan ID , Hogg ID 2010a . Barcoding freshwater zooplankton . In : Lake Ecosystem Restoration New Zealand Annual Science Review Meeting . Hamilton , New Zealand Waikato University .
- Banks JC , Hicks BJ , Lobb R , Blair J 2010b . Genetic identification of fish . CBER Report 109 . 17
- Bartlett , JMS and Stirling , D . 2003 . A short history of the polymerase chain reaction . PCR Protocols , 226 : 3 – 6 .
- Bartlett , JM and Bartlett , JMS . 2004 . Fluorescence in situ hybridization: technical overview . Methods in Molecular Medicine , 97 : 77 – 87 .
- Bax N , Dunstan P , Gunasekera R , Patil JG , Sutton C 2006 . Evaluation of national control plan management options for the North Pacific seastar Asterias amurensis . Final report for the Department of Environment and Heritage. Canberra, Australia, CSIRO Marine Research . 85
- Becker , A , Reith , A , Napiwotzki , J and Kadenbach , B . 1996 . A quantitative method of determining initial amounts of DNA by polymerase chain reaction cycle titration using digital imaging and a novel DNA stain . Analytical Biochemistry , 237 : 204 – 207 . doi: 10.1006/abio.1996.0230
- Benson , D , Karsch-Mizrachi , I , Lipman , D , Ostell , J and Sayers , E . 2009 . GenBank . Nucleic Acids Research , 37 : D26 – 31 . doi: 10.1093/nar/gkn723
- Biosecurity New Zealand 2011 Import health standard for ornamental fish and marine invertebrates from all countries . Wellington , Ministry of Agriculture and Forestry . 72
- Bott NJ , Ophel-Keller KM , Sierp MT , Herdina , Rowling KP , McKay AC , Loo MGK , Tanner JE , Deveney MR 2010 . Toward routine, DNA-based detection methods for marine pests . Biotechnology Advances 28 : 706 – 714 . doi: 10.1016/j.biotechadv.2010.05.018
- Burns , NM , Rutherford , JC and Clayton , JS . 1999 . A monitoring and classification system for New Zealand lakes and reservoirs . Journal of Lake and Reservoir Management , 15 : 255 – 271 . doi: 10.1080/07438149909354122
- Byappanahalli , M , Fowler , M , Shively , D and Whitman , R . 2003 . Ubiquity and persistence of Escherichia coli in a midwestern coastal stream . Applied and Environmental Microbiology , 69 : 4549 – 4555 . doi: 10.1128/AEM.69.8.4549-4555.2003
- Byappanahalli , MN , Whitman , RL , Shively , DA , Ting , WTE , Tseng , CC and Nevers , MB . 2006 . Seasonal persistence and population characteristics of Escherichia coli and enterococci in deep backshore sand of two freshwater beaches . Journal of Water and Health , 4 : 313 – 320 .
- Cadel-Six , S , Iteman , I , Peyraud-Thomas , C , Mann , S , Ploux , O and Méjean , A . 2009 . Identification of a polyketide synthase coding sequence specific for anatoxin-a-producing Oscillatoria cyanobacteria . Applied Environmental Microbiology , 75 : 4909 – 4912 . doi: 10.1128/AEM.02478-08
- Cary SC , Hicks BJ , Coyne KJ , Rueckert A , Gemmill CEC , Barnett CME 2007 . A sensitive genetic-based detection capability for Didymosphenia geminata (Lyngbye) M. Schmidt: Phases Two and Three . CBER Contract Report 62. Client report prepared for MAF Biosecurity New Zealand Hamilton New Zealand Centre for Biodiversity and Ecology Research, University of Waikato 87
- Castiglioni , B , Rizzi , E , Frosini , A , Sivonen , K , Rajaniemi , P , Rantala , A , Mugnai , MA , Ventura , S , Wilmotte , A , Boutte , C , Grubisic , S , Balthasart , P , Consolandi , C , Bordoni , R , Mezzelani , A , Battaglia , C and De Bellis , G . 2004 . Development of a universal microarray based on the ligation detection reaction and 16S rRNA gene polymorphism to target diversity of cyanobacteria . Applied Environmental Microbiology , 70 : 7161 – 7172 . doi: 10.1128/AEM.70.12.7161-7172.2004
- Chang , FH , Anderson , C and Boustead , NC . 1990 . First record of a Heterosigma (Raphidophyceae) bloom with associated mortality of cage-reared salmon in Big Glory Bay, New Zealand . New Zealand Journal of Marine and Freshwater Research , 24 : 461 – 469 . doi: 10.1080/00288330.1990.9516437
- Chen , X and Kwok , PY . 1999 . Homogeneous genotyping assays for single nucleotide polymorphisms with fluorescence resonance energy transfer detection . Genetic Analysis: Biomolecular Engineering , 14 : 157 – 163 . doi: 10.1016/S1050-3862(98)00016-3
- Collins , RA , Armstrong , KF , Meier , R , Yi , Y , Brown , SDJ , Cruickshank , RH , Keeling , S and Johnston , C . 2012 . Barcoding and border biosecurity: identifying cyprinid fishes in the aquarium trade . PLoS ONE , 7 : e28381 doi: 10.1371/journal.pone.0028381
- Cornelisen CD , Kirs M , Gilpin B , Scholes P 2012 . Microbial source tracking (MST) tools for water quality monitoring . Prepared for Regional Councils and the Coastal Special Interest Group, Cawthron Report 204 Nelson New Zealand Cawthron Institute 28
- D'Archino , R , Nelson , WA and Zuccarello , GC . 2007 . Invasive marine red alga introduced to New Zealand waters: first record of Grateloupia turuturu (Halymeniaceae, Rhodophyta) . New Zealand Journal of Marine and Freshwater Research , 41 : 35 – 42 . doi: 10.1080/00288330709509894
- Darling , JA and Blum , MJ . 2007 . DNA-based methods for monitoring invasive species: a review and prospectus . Biological Invasions , 9 : 751 – 765 . doi: 10.1007/s10530-006-9079-4
- Darling , JA and Mahon , AR . 2011 . From molecules to management: adopting DNA-based methods for monitoring biological invasions in aquatic environments . Environmental Research , 111 : 978 – 988 . doi: 10.1016/j.envres.2011.02.001
- Deagle , BE , Bax , N and Patil , JG . 2003 . Development and evaluation of a PCR-based test for detection of Asterias (Echinodermata: Asteroidea) larvae in Australian plankton samples from ballast water . Marine and Freshwater Research , 54 : 709 – 719 . doi: 10.1071/MF03031
- Duggan , IC , Green , JD and Shiel , RJ . 2001a . Distribution of rotifers in North Island, New Zealand, and their potential use as bioindicators of lake trophic state . Hydrobiologia , 446/447 : 155 – 164 . doi: 10.1023/A:1017503407240
- Duggan , IC , Green , JD and Thomasson , K . 2001b . Do rotifers have potential as bioindicators of lake trophic state? . Verhandlungen—Internationale Vereinigung für Theoretische und Angewandte Limnologie , 27 : 3497 – 3502 .
- Dyhrman , ST , Erdner , DL , La Du , J , Galac , M and Anderson , DM . 2006 . Molecular quantification of toxic Alexandrium fundyense in the Gulf of Maine using real-time PCR . Harmful Algae , 5 : 242 – 250 . doi: 10.1016/j.hal.2005.07.005
- Ehrenfeld , JG . 2010 . Ecosystem consequences of biological invasions . Annual Review of Ecology, Evolution, and Systematics , 41 : 59 – 80 . doi: 10.1146/annurev-ecolsys-102209-144650
- Espy , MJ , Uhl , JR , Sloan , LM , Buckwalter , SP , Jones , MF , Vetter , EA , Yao , JD , Wengenack , NL , Rosenblatt , JE , Cockerill , FR III and Smith , TF . 2006 . Real-time PCR in clinical microbiology: applications for routine laboratory testing . Clinical Microbiology Reviews , 19 : 165 – 256 . doi: 10.1128/CMR.19.1.165-256.2006
- Evans , CW , Wilson , DA and Mills , GN . 2001 . Quantitative competitive (qc) RT-PCR as a tool in biomarker analysis . Biomarkers. , 6 : 7 – 14 . doi: 10.1080/135475001452733
- Ficetola , GF , Miaud , C , Pompanon , F and Taberlet , P . 2008 . Species detection using environmental DNA from water samples . Biology Letters , 4 : 423 – 425 . doi: 10.1098/rsbl.2008.0118
- Field , KG and Samadpour , M . 2007 . Fecal source tracking, the indicator paradigm, and managing water quality . Water Research , 41 : 3517 – 3538 . doi: 10.1016/j.watres.2007.06.056
- Galil , BS . 2007 . Loss or gain? Invasive aliens and biodiversity in the Mediterranean Sea . Marine Pollution Bulletin , 55 : 314 – 322 . doi: 10.1016/j.marpolbul.2006.11.008
- Gescher , C , Metfies , K and Medlin , LK . 2008 . The ALEX CHIP: development of a DNA chip for identification and monitoring of Alexandrium . Harmful Algae , 7 : 485 – 494 . doi: 10.1016/j.hal.2007.11.001
- Girones , R , Ferrús , MA , Alonso , JL , Rodriguez-Manzano , J , Calgua , B , de Abreu Corrêa , A , Hundesa , A , Carratala , A and Bofill-Mas , S . 2010 . Molecular detection of pathogens in water: the pros and cons of molecular techniques . Water Research , 44 : 4325 – 4339 . doi: 10.1016/j.watres.2010.06.030
- Glenn , TC . 2011 . Field guide to next-generation DNA sequencers . Molecular Ecology Resources , 11 : 759 – 769 . doi: 10.1111/j.1755-0998.2011.03024.x
- Godhe , A , Cusack , C , Pedersen , J , Anderson , P , Anderson , DM , Breasnan , E , Cembella , A , Dahl , E , Diercks , S , Elbrachter , M , Edler , L , Galluzzi , L , Gescher , C , Gladstone , M , Karlson , B , Kulis , D , LeGresley , M , Lindahl , O , Marin , R , McDermott , G , Medlin , LK , Naustvoll , L-J , Penna , A and Tobe , K . 2007 . Intercalibration of classical and molecular techniques for identification of Alexandrium fundyense (Dinophyceae) and estimation of cell densities . Harmful Algae , 6 : 56 – 72 . doi: 10.1016/j.hal.2006.06.002
- Goldberg , CS , Pilliod , DS , Arkle , RS and Waits , LP . 2011 . Molecular detection of vertebrates in stream water: a demonstration using Rocky Mountain tailed frogs and Idaho giant salamanders . PLoS ONE , 6 : e22746 doi: 10.1371/journal.pone.0022746
- Gordon DP 2012 . The New Zealand inventory of biodiversity . Kingdom Animalia: Radiata, Lophotrochozoa, and Deuterostomia . 1 Christchurch , , New Zealand , Canterbury University Press . 566
- Greenfield , DI , Marin , R , Doucette , GJ , Mikulski , C , Jones , K , Jensen , S , Roman , B , Alvarado , N , Feldman , J and Scholin , C . 2008 . Field applications of the second-generation environmental sample processor (ESP) for remote detection of harmful algae: 2006–2007 . Limnology and Oceanography-Methods , 6 : 667 – 679 . doi: 10.4319/lom.2008.6.667
- Griffin , DW , Lipp , EK , McLaughlin , MR and Rose , JB . 2001 . Marine recreation and public health microbiology: quest for the ideal indicator . Bioscience , 51 : 817 – 826 . doi: 10.1641/0006-3568(2001)051[0817:MRAPHM]2.0.CO;2
- Hajibabaei , M , Shokralla , S , Zhou , X , Singer , GAC and Baird , DJ . 2011 . Environmental barcoding: a next-generation sequencing approach for biomonitoring applications using river benthos . PLoS ONE , 6 ( 4 ) : 17497 doi: 10.1371/journal.pone.0017497
- Harvey , JBJ , Hoy , MS and Rodriguez , RJ . 2009 . Molecular detection of native and invasive marine invertebrate larvae present in ballast and open water environmental samples collected in Puget Sound . Journal of Experimental Marine Biology and Ecology , 369 : 93 – 99 . doi: 10.1016/j.jembe.2008.10.030
- Harvey , JBJ , Ryan , JP , Marin , R , Preston , CM , Alvarado , N , Scholin , CA and Vrijenhoek , RC . 2012 . Robotic sampling, in situ monitoring and molecular detection of marine zooplankton . Journal of Experimental Marine Biology and Ecology , 413 : 60 – 70 .
- Havens , KE . 2008 . Cyanobacteria blooms: effects on aquatic ecosystems . Advances in Experimental Medicine and Biology , 619 : 733 – 747 .
- Hayes , KR , Cannon , R , Neil , K and Inglis , G . 2005 . Sensitivity and cost considerations for the detection and eradication of marine pests in ports . Marine Pollution Bulletin , 50 : 823 – 834 . doi: 10.1016/j.marpolbul.2005.02.032
- Heath , M , Wood , SA and Ryan , K . 2010 . Polyphasic assessment of freshwater benthic mat forming cyanobacteria isolated from New Zealand . FEMS Microbiology Ecology , 73 : 95 – 109 .
- Hebert , PDN , Cywinska , A , Ball , SL and deWaard , JR . 2003 . Biological identification through DNA barcodes . Proceedings of the Royal Society of London B , 270 : 313 – 321 . doi: 10.1098/rspb.2002.2218
- Heid , CA , Stevens , J , Livak , KJ and Williams , PM . 1996 . Real-time quantitative PCR . Genome Research , 6 : 986 – 994 . doi: 10.1101/gr.6.10.986
- Hewitt , CL , Willing , J , Bauckham , A , Cassidy , AM , Cox , CMS , Jones , L and Wotton , DM . 2004 . New Zealand marine biosecurity: delivering outcomes in a fluid environment . New Zealand Journal of Marine and Freshwater Research , 38 : 429 – 438 . doi: 10.1080/00288330.2004.9517250
- Hewitt , J , Bell , D , Simmons , GC , Rivera-Aban , M , Wolf , S and Greening , GE . 2007 . Gastroenteritis outbreak caused by waterborne norovirus at a New Zealand ski resort . Applied and Environmental Microbiology , 73 : 7853 – 7857 . doi: 10.1128/AEM.00718-07
- Hickey , CW . 1995 . Ecotoxicity in New Zealand . Australasian Journal of Ecotoxicology , 1 : 43 – 50 .
- Hogg , ID , Smith , BJ , Banks , JC , Dewaard , JR and Hebert , PDN . 2009 . Testing use of mitochondrial COI sequences for the identification and phylogenetic analysis of New Zealand caddis flies (Trichoptera) . New Zealand Journal of Freshwater and Marine Research , 43 : 1137 – 1146 . doi: 10.1080/00288330.2009.9626536
- Hopkins , GA and Forrest , BM . 2010 . Challenges associated with pre-border management of biofouling on oil rigs . Marine Pollution Bulletin , 60 : 1924 – 1929 . doi: 10.1016/j.marpolbul.2010.07.015
- Humbert , JF , Quiblier , C and Gugger , M . 2010 . Molecular approaches for monitoring potential toxic marine and freshwater phytoplankton species . Analytical and Bioanalytical Chemistry , 397 : 1723 – 1732 . doi: 10.1007/s00216-010-3642-7
- Jasperse JA 1993 . Marine toxins and New Zealand shellfish. Proceedings of a workshop on research issues , 10–11 June 1993 . Royal Society of New Zealand Miscellaneous Series 24 . 68
- Jerde , CL , Mahon , AR , Chadderton , WL and Lodge , DM . 2011 . Sight-unseen’ detection of rare aquatic species using environmental DNA . Conservation Letters , 4 : 150 – 157 . doi: 10.1111/j.1755-263X.2010.00158.x
- Jofre , J , Blasi , M , Bosch , A and Lucena , F . 1989 . Occurrence of bacteriophages infecting Bacteroides fragilis and other viruses in polluted marine sediments . Water Science and Technology , 21 : 15 – 19 .
- John , U , Medlin , LK and Groben , R . 2005 . Development of specific rRNA probes to distinguish between geographic clades of the Alexandrium tamarense species complex . Journal of Plankton Research , 27 : 199 – 204 . doi: 10.1093/plankt/fbh160
- Jones , WJ , Preston , CM , Marin , R , Scholin , CA and Vrijenhoek , RC . 2008 . A robotic molecular method for in situ detection of marine invertebrate larvae . Molecular Ecology Resources , 8 : 540 – 550 . doi: 10.1111/j.1471-8286.2007.02021.x
- Kellmann , R , Mihali , TK , Jeon , YJ , Pickford , R , Pomati , F and Neilan , BA . 2008 . Biosynthetic intermediate analysis and functional homology reveal a saxitoxin gene cluster in cyanobacteria . Applied and Environmental Microbiology , 7 : 4044 – 4053 . doi: 10.1128/AEM.00353-08
- Ki , JS and Han , MS . 2006 . A low-density oligonucleotide array study for parallel detection of harmful algal species using hybridization of consensus PCR products of LSU rDNA D2 domain . Biosensors and Bioelectronics , 21 : 1812 – 1821 . doi: 10.1016/j.bios.2005.09.006
- Kilroy C 2004 . A new alien diatom, Didymosphenia geminata (Lyngbye) Schmidt: its biology, distribution, effects and potential risks for New Zealand fresh waters . Client Report CHC2004-128 . Christchurch , , New Zealand , National Institute of Water and Atmospheric Research . 34
- Kilroy , C , Larned , ST and Biggs , BJF . 2009 . The non-indigenous diatom Didymosphenia geminata alters benthic communities in New Zealand rivers . Freshwater Biology , 54 : 1990 – 2002 . doi: 10.1111/j.1365-2427.2009.02247.x
- Kim , KC and Byrne , LB . 2006 . Biodiversity loss and the taxonomic bottleneck: emerging biodiversity science . Ecological Research , 21 : 794 – 810 . doi: 10.1007/s11284-006-0035-7
- Kirs M , Cornelisen C 2011 . MST technology for shellfish . Final report. Cawthron Report 2002 Nelson New Zealand Cawthron Institute 36
- Kirs M , Harwood VJ , Gillespie PA , Fidler AE , Cornelisen CD , Fyfe WR 2008 . Application of microbial source tracking (MST) technologies for identifying the source of microbial contamination in the lower Maitai River and Little Sydney Stream . Prepared for the Nelson City Council. Cawthron Report 1447 Nelson New Zealand Cawthron Institute 42
- Kirs , M , Harwood , VJ , Fidler , AE , Gillespie , PA , Fyfe , WR , Blackwood , AD and Cornelisen , CD . 2011 . Source tracking faecal contamination in an urbanised and a rural waterway in the Nelson-Tasman region, New Zealand . New Zealand Journal of Marine and Freshwater Research , 45 : 43 – 58 . doi: 10.1080/00288330.2010.535494
- Knox MA , Hicks BJ , Banks JC , Hogg ID 2009 . Fish biosurveillance by genetic methods: a feasibility study . CBER Contract Report 90. . 26 Hamilton New Zealand Waikato University
- Kuhajek J , Smith KF , Adamson JE , Wood SA 2011 . PCR assay for the Mediterranean fan worm (Sabella spallanzanii) . Prepared for MAF. Cawthron Report 1940 Nelson New Zealand Cawthron Institute . 42
- Laurie , AD . 2004 . Quantitation of metallothionein mRNA from the New Zealand common bully (Gobiomorphus cotidianus) and its implications for biomonitoring . New Zealand Journal of Marine and Freshwater Research , 38 : 869 – 877 . doi: 10.1080/00288330.2004.9517286
- Lear , G , Boothroyd , IKG , Turner , SJ , Roberts , K and Lewis , GD . 2009 . A comparison of bacterial and benthic invertebrate community assemblages as indicators of ecological health within freshwater streams . Freshwater Biology , 54 : 1532 – 1543 . doi: 10.1111/j.1365-2427.2009.02190.x
- Lear , G and Lewis , GD . 2009 . Impact of catchment land use on bacterial communities within stream biofilms . Ecological Indicators , 9 : 848 – 855 . doi: 10.1016/j.ecolind.2008.10.001
- Lecroq , B , Lejzerowicz , F , Christen , R , Esling , P , Baerlocher , L , Osteras , M , Farinelli , L and Pawlowski , J . 2011 . Ultra-deep sequencing of foraminiferal microbarcodes unveils hidden richness of early monothalamous lineages in deep-sea sediments . Proceedings of the National Academy of Sciences USA , 108 : 13177 – 13182 . doi: 10.1073/pnas.1018426108
- Leusch , FDL , van den Heuvel , MR , Laurie , AD , Chapman , HF , Gooneratne , SR and Tremblay , LA . 2005 . Quantification of vitellogenin mRNA induction in mosquitofish (Gambusia affinis) by reverse transcription real-time polymerase chain reaction (RT-PCR) . Biomarkers , 10 : 429 – 438 . doi: 10.1080/13547500500343381
- Lewis GD , Washington V , Lear G , Roberts K , Curran J , Fan V , Neale M 2011 . A bacterial community index (BCI) for New Zealand streams . Auckland Regional Council Technical Report TR 2010/068 Auckland New Zealand Auckland City Council . 200
- Lipp , EK , Farrah , SA and Rose , JB . 2001 . Assessment and impact of microbial fecal pollution and human enteric pathogens in a coastal community . Marine Pollution Bulletin , 42 : 286 – 293 . doi: 10.1016/S0025-326X(00)00152-1
- Liu , W , Marsh , T , Cheng , H and Forney , L . 1997 . Characterization of microbial diversity by determining terminal restriction fragment length polymorphisms of genes encoding 16S rRNA . Applied and Environmental Microbiology , 63 : 4516 – 4522 .
- Lougheed , VL , Crosbie , B and Chow-Fraser , P . 1998 . Predictions on the effect of common carp (Cyprinus carpio) exclusion on water quality, zooplankton, and submergent macrophytes in a Great Lakes wetland . Canadian Journal of Fisheries and Aquatic Sciences , 55 : 1189 – 1197 . doi: 10.1139/f97-315
- MacKenzie , L , de Salas , M , Adamson , J and Beuzenberg , V . 2004 . The dinoflagellate genus Alexandrium (Halim) in New Zealand coastal waters: comparative morphology, toxicity and molecular genetics . Harmful Algae , 3 : 71 – 92 .
- Mackenzie , L . 1991 . Toxic and noxious phytoplankton in Big Glory Bay, Stewart Island, New Zealand . Journal of Applied Phycology , 3 : 19 – 34 . doi: 10.1007/BF00003916
- MacKenzie , LA , Smith , KF , Rhodes , LL , Brown , A , Langi , V , Edgar , M , Lovell , G and Preece , M . 2011a . Mortalities of sea-cage salmon (Oncorhynchus tshawytscha) due to a bloom of Pseudochattonella verruculosa (Dictyochophyceae) in Queen Charlotte Sound, New Zealand . Harmful Algae , 11 : 45 – 53 . doi: 10.1016/j.hal.2011.07.003
- MacKenzie L , Harwood T , Boundy M , Smith K , Knight B , Jiang W , McNabb P , Selwood A , van Ginkel R , Langi V , Edgar M , Moisan C 2011b . An Alexandrium catenella bloom and associated saxitoxin contamination of shellfish, Queen Charlotte Sound . March–April 2011 Prepared for MAF Food Safety, Cawthron Report 1945 . 31
- McCulloch GA 2011 . Evolutionary genetics of southern stoneflies . PhD thesis . Department of Zoology. University of Otago , Dunedin , , New Zealand .
- McDowall RM 1990 . New Zealand freshwater fishes: a natural history and guide . Auckland , , New Zealand , Heinemann Reed . 553
- McDowall RM 2000 . The Reed guide to New Zealand freshwater fishes . Auckland , , New Zealand , Reed Books . 224
- McDowall , RM . 2004 . Shoot first, and then ask questions: a look at aquarium fish imports and invasiveness in New Zealand . New Zealand Journal of Marine and Freshwater Research , 38 : 503 – 510 . doi: 10.1080/00288330.2004.9517256
- Metzker , ML . 2010 . Sequencing technologies: the next generation . Nature Reviews Genetics , 11 : 31 – 46 . doi: 10.1038/nrg2626
- Meyerson , LA and Reaser , JK . 2002 . Biosecurity: moving toward a comprehensive approach . Bioscience , 52 : 593 – 600 . doi: 10.1641/0006-3568(2002)052[0593:BMTACA]2.0.CO;2
- Mihali , TK , Kellmann , R , Muenchoff , J , Barrow , KD and Neilan , BA . 2008 . Characterization of the gene cluster responsible for cylindrospermopsin biosynthesis . Applied and Environmental Microbiology , 74 : 716 – 722 . doi: 10.1128/AEM.01988-07
- Minamoto T , Yamanaka H , Takahara T , Honjo M , Kawabata Z 2011 . Surveillance of fish species composition using environmental DNA . Limnology . doi: 10.1007/s10201-011-0362-4: 1–5
- Ministry for the Environment 2007 . Environment New Zealand 2007 . Wellington , , New Zealand , Ministry for the Environment . 455
- Ministry for the Environment and Ministry of Health 2009 . New Zealand guidelines for managing cyanobacteria in recreational fresh waters: interim guidelines . Prepared for the Ministry for the Environment and the Ministry of Health by SA Wood, DP Hamilton, WJ Paul, KA Safi, WM Williamson . Wellington , , New Zealand , Ministry for the Environment . 89
- Monis , PT , Giglio , S and Saint , CP . 2005 . Comparison of SYTO9 and SYBR Green I for real-time polymerase chain reaction and investigation of the effect of dye concentration on amplification and DNA melting curve analysis . Analytical and Bioanalytical Chemistry , 340 : 24 – 34 .
- Moriarty E , Gilpin B 2009 . Faecal source tracking in the Avon River, Christchurch March–May 2009 . Report R09/67 prepared for Environment Canterbury . Christchurch New Zealand Institute of Environmental Science and Research Ltd . 36
- Mountfort , D , Rhodes , L , Broom , J , Gladstone , M and Tyrell , J . 2007 . Fluorescent in situ hybridization assay as a species-specific identifier of the Northern Pacific seastar, Asterias amurensis . New Zealand Journal of Marine and Freshwater Research , 41 : 283 – 290 . doi: 10.1080/00288330709509915
- Mountfort , D , Smith , KF , Kirs , M , Kuhajek , J , Adamson , JE and Wood , SA . 2012 . Development of single and multispecies detection methods for the surveillance and monitoring of marine pests in New Zealand . Aquatic Invasions , 7 : 125 – 128 . doi: 10.3391/ai.2012.7.1.013
- Murray , SA , Wiese , M , Stuken , A , Brett , S , Kellmann , R , Hallegraeff , G and Neilan , BA . 2011 . sxtA-based quantitative molecular assay to identify saxitoxin-producing harmful algal blooms in marine waters . Applied and Environmental Microbiology , 77 : 7050 – 7057 . doi: 10.1128/AEM.05308-11
- Myers , JH , Simberloff , D , Kuris , AM and Carey , JR . 2000 . Eradication revisited: dealing with exotic species . Trends in Ecology & Evolution , 15 : 316 – 320 . doi: 10.1016/S0169-5347(00)01914-5
- Novis , P , Halle , C , Wilson , B and Tremblay , LA . 2009 . Identification and characterisation of freshwater algae isolated from a pollution gradient using rbcL sequences and toxicity testing . Archives of Environmental Contamination and Toxicology , 57 : 504 – 514 . doi: 10.1007/s00244-009-9312-0
- Olsen D , Wood SA , Fidler A , Hitchings T , Waters J 2007 . Phylogeny of New Zealand leptophlebiid mayflies: molecular evidence . In : Joint meeting of the New Zealand Freshwater Sciences Society and the Australian Society for Limnology , 3–7 December . Queenstown New Zealand .
- Penna , A , Bertozzini , E , Battocchi , C , Galluzzi , L , Giacobbe , MG , Vila , M , Garces , E , Luglie , A and Magnani , M . 2007 . Monitoring of HAB species in the Mediterranean Sea through molecular methods . Journal of Plankton Research , 29 : 19 – 38 . doi: 10.1093/plankt/fbl053
- Petrosino , JF , Highlander , S , Luna , RA , Gibbs , RA and Versalovic , J . 2009 . Metagenomic pyrosequencing and microbial identification . Clinical Chemistry , 55 : 856 – 866 . doi: 10.1373/clinchem.2008.107565
- Pina , B , Casado , M and Quiro , L . 2007 . Analysis of gene expression as a new tool in ecotoxicology and environmental monitoring . Trends in Analytical Chemistry , 26 : 1145 – 1154 . doi: 10.1016/j.trac.2007.09.009
- Preston , CM , Harris , A , Ryan , JP , Roman , B , Marin , R III , Jensen , S , Everlove , C , Birch , J , Dzenitis , JM , Pargett , D , Adachi , M , Turk , K , Zehr , JP and Scholin , CA . 2011 . Underwater application of quantitative PCR on an ocean mooring . PLoS ONE , 6 : e22522 doi: 10.1371/journal.pone.0022522
- Ranjard , L , Poly , F , Lata , JC , Mougel , C , Thioulouse , J and Nazaret , S . 2001 . Characterization of bacterial and fungal soil communities by automated ribosomal intergenic spacer analysis fingerprints: biological and methodological variability . Applied and Environmental Microbiology , 67 : 4479 – 4487 . doi: 10.1128/AEM.67.10.4479-4487.2001
- Ratnasingham NS , Hebert PDN 2007 . The barcode of life data system ( www.barcodinglife.co.org ). Molecular Ecology Notes 7 : 355 – 364 . doi: 10.1111/j.1471-8286.2007.01678.x
- Read , GB , Inglis , G , Stratford , P and Ahyong , ST . 2011 . Arrival of the alien fanworm Sabella spallanzanii (Gmelin, 1791) (Polychaeta: Sabellidae) in two New Zealand harbours . Aquatic Invasions , 6 : 273 – 279 . doi: 10.3391/ai.2011.6.3.04
- Rhodes , L , Scholin , C and Garthwaite , I . 1998 . Pseudo-nitzschia in New Zealand and the role of DNA probes and immunoassays in refining marine biotoxin monitoring programmes . Natural Toxins , 6 : 105 – 111 . doi: 10.1002/(SICI)1522-7189(199805/08)6:3/4%3C105::AID-NT13%3E3.0.CO;2-9
- Rhodes , LL , Mackenzie , AL , Kaspar , HF and Todd , KE . 2001a . Harmful algae and mariculture in New Zealand . ICES Journal of Marine Science , 58 : 398 – 403 . doi: 10.1006/jmsc.2000.1023
- Rhodes L , Scholin CA , Tyrrell JV , Adamson J , Todd K 2001b . The integration of DNA probes into New Zealand's routine phytoplankton monitoring programs . In : Hallegraeff GM , Blackburn SI , Bolch CJ , Lewis RJ Harmful algal blooms 2000 . Proceedings of the 9th International Conference on Harmful Algal Blooms . Paris , Intergovernmental Oceanographic Commission of UNESCO . 429 – 432 .
- Rhodes , LL , Adamson , JE , Rublee , PA and Schaefer , E . 2006 . Geographic distribution of Pfiesteria spp. (Pfiesteriaceae) in Tasman Bay and Canterbury, New Zealand (2002–03) . New Zealand Journal of Marine and Freshwater Research , 40 : 211 – 220 .
- Rhodes , L , Smith , K and de Salas , M . 2007 . DNA probes, targeting large sub-unit rRNA, for the rapid identification of the paralytic shellfish poison producing dinoflagellate, Gymnodinium catenatum . New Zealand Journal of Marine and Freshwater Research , 41 : 385 – 390 . doi: 10.1080/00288330709509928
- Rhodes LL , Smith KF , Moisan C 2012 . Shifts and stasis in marine HAB monitoring in New Zealand . Environmental Science and Pollution Research . In press .
- Richardson , P . 2010 . Special issue: next generation DNA sequencing . Genes , 1 : 385 – 387 . doi: 10.3390/genes1030385
- Robarts , RD , Waiser , MJ , Arts , MT and Evans , MS . 2005 . Seasonal and diel changes of dissolved oxygen in a hypertrophic prairie lake . Lakes & Reservoirs: Research and Management , 10 : 167 – 177 . doi: 10.1111/j.1440-1770.2005.00273.x
- Rothberg , JM , Hinz , W , Rearick , TM , Schultz , J , Mileski , W Davey , M . 2011 . An integrated semiconductor device enabling non-optical genome sequencing . Nature , 475 : 348 – 352 . doi: 10.1038/nature10242
- Rueckert , A and Cary , SC . 2009 . Use of an armored RNA standard to measure microcystin synthetase E gene expression in toxic Microcystis sp. by reverse-transcription QPCR . Limnology and Oceanography: Methods , 7 : 509 – 520 . doi: 10.4319/lom.2009.7.509
- Rueckert , A , Wood , SA and Cary , SC . 2007 . Development and field assessment of a quantitative PCR for the detection and enumeration of the noxious bloom-former Anabaena planktonica . Limnology and Oceanography: Methods , 5 : 474 – 483 . doi: 10.4319/lom.2007.5.474
- Ryan , J , Greenfield , D , Marin , R , Preston , C , Roman , B , Jensen , S , Pargett , D , Birch , J , Mikulski , C , Doucette , G and Scholin , C . 2011 . Harmful phytoplankton ecology studies using an autonomous molecular analytical and ocean observing network . Limnology and Oceanography , 56 : 1255 – 1272 . doi: 10.4319/lo.2011.56.4.1255
- Sanger , F and Coulson , AR . 1975 . A rapid method for determining sequences in DNA by primed synthesis with DNA polymerase . Journal of Molecular Biology , 94 : 441 – 448 . doi: 10.1016/0022-2836(75)90213-2
- Scholin , CA , Buck , KR , Britschgi , T , Cangelosi , G and Chavez , FP . 1996 . Identification of Pseudo-nitzschia australis (Bacillariophyceae) using rRNA-targeted probes in whole cell and sandwich hybridization formats . Phycologia , 35 : 190 – 197 . doi: 10.2216/i0031-8884-35-3-190.1
- Scholin , CA , Miller , PE , Buck , K , Chavez , F , Harris , P , Haydock , P , Howard , J and Cangelosi , G . 1997 . Detection and quantification of Pseudo-nitzschia australis in cultured and natural populations using LSU rRNA-targeted probes . Limnology and Oceanography , 42 : 1265 – 1272 . doi: 10.4319/lo.1997.42.5_part_2.1265
- Scholin , CA , Vreiling , E , Peperzak , L , Rhodes , L and Rublee , P . 2003 . “ Detection of HAB species using lectin, antibody and DNA probes ” . In Manual on harmful marine micro-algae , Edited by: Hallegraeff , GM , Anderson , DM and Cembella , AD . 131 – 164 . Paris : UNESCO Publishing .
- Scholin , C , Doucette , G , Jensen , S , Roman , B , Pargett , D , Marin , R , Preston , C , Jones , W , Feldman , J , Everlove , C , Harris , A , Alvarado , N , Massion , E , Birch , J , Greenfield , D , Vrijenhoek , R , Mikulski , C and Jones , K . 2009 . Remote detection of marine microbes, small invertebrates, harmful algae, and biotoxins using the environmental sample processor (ESP) . Oceanography , 22 : 158 – 167 . doi: 10.5670/oceanog.2009.46
- Scott , TM , Rose , JB , Jenkins , TM , Farrah , SR and Lukasik , J . 2002 . Microbial source tracking: current methodology and future directions . Applied and Environmental Microbiology , 68 : 5796 – 5803 . doi: 10.1128/AEM.68.12.5796-5803.2002
- Seafood Industry Council 2012 . Economic review of the seafood industry, , Edition 1 , June 2012 Wellington , New Zealand Seafood Industry Council Ltd . 6
- Shokralla , S , Spall , JL , Gibson , JF and Hajibabaei , M . 2012 . Next-generation sequencing technologies for environmental DNA research . Molecular Ecology , 21 : 1794 – 1805 . doi: 10.1111/j.1365-294X.2012.05538.x
- Simberloff , D . 2001 . Eradication of island invasives: practical actions and results achieved . Trends in Ecology and Evolution , 16 : 273 – 274 . doi: 10.1016/S0169-5347(01)02154-1
- Simmons , G , Garbutt , C , Hewitt , J and Greening , G . 2007 . A New Zealand outbreak of norovirus gastroenteritis linked to the consumption of imported raw Korean oysters . The New Zealand Medical Journal , 120 ( 1264 ) : U2773
- Smith , F , Wood , SA , Ginkel , Rv , Broady , P and Gaw , S . 2011 . First report of saxitoxin production by a species of the freshwater benthic cyanobacterium, Scytonema Agardh . Toxicon , 57 : 566 – 573 . doi: 10.1016/j.toxicon.2010.12.020
- Smith , KF , Rhodes , LL , Selwood , AI , Marfell , MJ , Zeewoldt , CM , de Salas , MF , Haywood , AJ and Scholin , CA . 2007 . Massive Karenia mikimotoi bloom in Northland, New Zealand: use of traditional and molecular techniques for rapid identification of HAB species . Harmful Algae News , 34 : 1 – 3 .
- Smith , KF , Cahill , PL and Fidler , AE . 2010 . First record of the solitary ascidian Ciona savignyi Herdman, 1882 in the southern hemisphere . Aquatic Invasions , 5 : 363 – 368 . doi: 10.3391/ai.2010.5.4.05
- Smith , KF , Rhodes , LL , Adamson , JE , Tyrrell , JV , Mountfort , DO and Jones , WJ . 2011 . Application of a sandwich hybridisation assay for rapid detection of the Northern Pacific seastar, Asterias amurensis . New Zealand Journal of Marine and Freshwater Research , 45 : 145 – 152 . doi: 10.1080/00288330.2010.526124
- Smith , KF , Thia , J , Gemmill , CEC , Cary , SC and Fidler , AE . 2012a . Barcoding of the cytochrome oxidase I (COI) indicates a recent introduction of Ciona savignyi into New Zealand and a rapid method for Ciona species discrimination . Aquatic Invasions , 7 : 305 – 313 . doi: 10.3391/ai.2012.7.3.002
- Smith , KF , Wood , SA , Mountfort , DO and Cary , SC . 2012b . Development of a real-time PCR assay for the detection of the invasive clam, Corbula amurensis, in environmental samples . Journal of Experimental Marine Biology and Ecology , 412 : 52 – 57 . doi: 10.1016/j.jembe.2011.10.021
- Smith , PJ , Webber , WR , McVeagh , SM , Inglis , GJ and Gust , N . 2003 . DNA and morphological identification of an invasive swimming crab, Charybdis japonica, in New Zealand waters . New Zealand Journal of Marine and Freshwater Research , 37 : 753 – 762 . doi: 10.1080/00288330.2003.9517205
- Smith , PJ , Page , M , Handley , SJ , McVeagh , SM and Ekins , M . 2007 . First record of the Australian ascidian Eudistoma elongatum in northern New Zealand . New Zealand Journal of Marine and Freshwater Research , 41 : 347 – 355 . doi: 10.1080/00288330709509924
- Sogin , ML , Morrison , HG Huber , JA . 2006 . Microbial diversity in the deep sea and the underexplored ‘rare biosphere’ . Proceedings of the National Academy of Sciences USA , 103 : 12115 – 12120 . doi: 10.1073/pnas.0605127103
- Sousa , R , Gutiérrez , J and Aldridge , D . 2009 . Non-indigenous invasive bivalves as ecosystem engineers . Biological Invasions , 11 : 2367 – 2385 . doi: 10.1007/s10530-009-9422-7
- Souza , V , Rocha , M , Valera , A and Eguiarte , LE . 1999 . Genetic structure of natural populations of Escherichia coli in wild hosts on different continents . Applied and Environmental Microbiology , 65 : 3373 – 3385 .
- Stark JD 1985 . A macroinvertebrate community index of water quality for stony streams . Water and Soil Miscellaneous Publication 87 . Wellington , , New Zealand , National Water and Soil Conservation Authority . 53
- Stoeck , T , Bass , D , Nebel , M , Christen , R , Jones , MDM , Breiner , HW and Richards , TA . 2010 . Multiple marker parallel tag environmental DNA sequencing reveals a highly complex eukaryotic community in marine anoxic water . Molecular Ecology , 19 : 21 – 31 . doi: 10.1111/j.1365-294X.2009.04480.x
- Taberlet , P , Coissac , E , Hajibabaei , M and Rieseberg , LH . 2012 . Environmental DNA: a special issue on DNA metabarcoding . Molecular Ecology , 21 : 1789 – 1793 . doi: 10.1111/j.1365-294X.2012.05542.x
- Tillett , D , Dittmann , E , Erhard , M , Dohren , H , Borner , T. and Neilan , BA . 2000 . Structural organization of microcystin biosynthesis in Microcystis aeruginosa PCC7806: an integrated peptide-polyketide synthetase system . Chemistry and Biology , 7 : 753 – 764 . doi: 10.1016/S1074-5521(00)00021-1
- Thomsen PF , Kielgast J , Iversen LL , Møller PR , Rasmussen M , Willerslev E 2012 . Detection of a diverse marine fish fauna using environmental DNA from seawater samples . PLoS ONE 7 8 : e41732 . doi: 10.1371/journal.pone.0041732
- Townsend , CR and Winterbourn , MJ . 1992 . Assessment of the environmental risk posed by an exotic fish: the proposed introduction of channel catfish (Ictalurus punctatus) to New Zealand . Conservation Biology , 6 : 273 – 282 . doi: 10.1046/j.1523-1739.1992.620273.x
- Townsend , CR . 2003 . Individual, population, community, and ecosystem consequences of a fish invader in New Zealand streams . Conservation Biology , 17 : 38 – 47 . doi: 10.1046/j.1523-1739.2003.02017.x
- Tyrrell , J , Bergquist , PR , Saul , D , Mackenzie , L and Bergquist , PL . 1997 . Oligonucleotide probe technology as applied to the study of harmful algal blooms . New Zealand Journal of Marine and Freshwater Research , 31 : 551 – 560 . doi: 10.1080/00288330.1997.9516788
- Tyrrell , JV , Connell , LB and Scholin , CA . 2002 . Monitoring for Heterosigma akashiwo using a sandwich hybridization assay . Harmful Algae , 1 : 205 – 214 . doi: 10.1016/S1568-9883(02)00012-4
- Utermöhl , H . 1958 . Zur vervollkommung der quantitativen phytoplankton methodik (Towards a perfection of quantitative phytoplankton methodology) . Mitteilungen der Internationale Vereinigung für Theoretische und Angewandte Limnologie , 9 : 1 – 38 .
- Vaitomaa , J , Rantala , A , Halinen , K , Rouhiainen , L , Tallberg , P , Mokelke , L and Sivonen , K . 2003 . Quantitative real-time PCR for determination of microcystin synthetase E copy numbers for Microcystis and Anabaena in lakes . Applied and Environmental Microbiology , 69 : 7289 – 7297 . doi: 10.1128/AEM.69.12.7289-7297.2003
- van den Heuvel , MR , Michel , C , Stevens , MI , Clarke , AC , Stölting , KN , Hicks , BJ and Tremblay , LA . 2007 . Monitoring the effects of pulp and paper effluent is restricted in genetically distinct populations of common bully (Gobiomorphus cotidianus) . Environmental Science and Technology , 41 : 2602 – 2608 . doi: 10.1021/es061922q
- Vitousek , PM , D'Antonio , CM , Loope , LL , Rejmanek , M and Westbrooks , R . 1997 . Introduced species: a significant component of human-caused global change . New Zealand Journal of Ecology , 21 : 1 – 16 .
- Wallentinus , I and Nyberg , CD . 2007 . Introduced marine organisms as habitat modifiers . Marine Pollution Bulletin , 55 : 323 – 332 . doi: 10.1016/j.marpolbul.2006.11.010
- Wang , W , Chen , K and Xu , C . 2006 . DNA quantification using EvaGreen and a real-time PCR instrument . Analytical Biochemistry , 356 : 303 – 305 . doi: 10.1016/j.ab.2006.05.027
- Wang , Z , Gerstein , M and Snyder , M . 2009 . RNA–Seq: a revolutionary tool for transcriptomics . Nature Reviews Genetics , 10 : 57 – 63 . doi: 10.1038/nrg2484
- Wear , RG and Gardner , JPA . 2001 . Biological effects of the toxic algal bloom of February and March 1998 on the benthos of Wellington Harbour, New Zealand . Marine Ecology Progress Series , 218 : 63 – 76 . doi: 10.3354/meps218063
- Wittenberg R , Cock MJW . 2001 . Invasive alien species: a toolkit of best prevention and management practices . Wallingford , , UK , CAB International . 228
- Wittwer , CT , Reed , GH , Gundry , CN , Vandersteen , JG and Pryor , RJ . 2003 . High-resolution genotyping by amplicon melting analysis using LCGreen . Clinical Chemistry , 49 : 853 – 860 . doi: 10.1373/49.6.853
- Wold , B and Myers , RM . 2008 . Sequence census methods for functional genomics . Nature Methods , 5 : 19 – 21 . doi: 10.1038/nmeth1157
- Wood , SA , Stirling , DJ , Briggs , LR , Sprosen , J , Holland , PT , Ruck , JG and Wear , RG . 2006 . Survey of cyanotoxins in New Zealand waterbodies between 2001 and 2004 . New Zealand Journal of Marine and Freshwater Research , 40 : 585 – 595 . doi: 10.1080/00288330.2006.9517447
- Wood , SA , Selwood , AI , Rueckert , A , Holland , PT , Milne , JR , Smith , KF , Smits , B , Watts , LF and Cary , CS . 2007 . First report of homoanatoxin-a and associated dog neurotoxicosis in New Zealand . Toxicon , 50 : 292 – 301 . doi: 10.1016/j.toxicon.2007.03.025
- Wood , SA , Jentzsch , K , Rueckert , A , Hamilton , D and Cary , SC . 2008a . Hindcasting cyanobacterial communities in Lake Okaro with germination experiments and genetic analyses . FEMS Microbiology Ecology , 67 : 252 – 260 . doi: 10.1111/j.1574-6941.2008.00630.x
- Wood , SA , Rueckert , A , Cowan , DA and Cary , SC . 2008b . Sources of edaphic cyanobacterial diversity in the Dry Valleys of Eastern Antarctica . ISME Journal , 2 : 308 – 320 . doi: 10.1038/ismej.2007.104
- Wood , SA , Heath , M , McGregor , G , Holland , PT , Munday , R and Ryan , K . 2010a . Identification of a benthic microcystin producing filamentous cyanobacterium (Oscillatoriales) associated with a dog poisoning in New Zealand . Toxicon , 55 : 987 – 903 . doi: 10.1016/j.toxicon.2009.12.019
- Wood , SA , Heath , M , Kuhajek , J and Ryan , K . 2010b . Fine scale spatial variability of anatoxin-a and homoanatoxin-a production in benthic cyanobacteria; implication for monitoring and management . Journal of Applied Microbial Ecology , 109 : 2011 – 2018 .
- Wood , SA , Rueckert , A , Cary , SC , Hamilton , DP and Dietrich , DR . 2010c . Switching toxin production on and off: intermittent microcystin synthesis in a Microcystis bloom . Environmental Microbiology Reports , 3 : 118 – 124 . doi: 10.1111/j.1758-2229.2010.00196.x
- Wood , SA , Dietrich , DR , Cary , SC and Hamilton , DP . 2012 . Increasing Microcystis cell abundance enhances microcystin synthesis: a mesocosm study . Inland Waters , 2 : 17 – 22 .
- Zamor , RM , Glenn , KL and Hambright , KD . 2012 . Incorporating molecular tools into routine HAB monitoring programs: using qPCR to track invasive Prymnesium . Harmful Algae , 15 : 1 – 7 . doi: 10.1016/j.hal.2011.10.028
- Zhang , J , Chiodini , R , Badr , A and Zhang , G . 2011 . The impact of next-generation sequencing on genomics . Journal of Genetic Genomics , 38 : 95 – 109 . doi: 10.1016/j.jgg.2011.02.003