Abstract
Management of fish populations is often focused on the exploitation of adult fish. Maintaining the habitat requirements of all life stages may also be an important consideration. We investigated the value of structured habitat to juvenile fishes within a northeastern New Zealand harbour using artificial seagrass units (ASUs). Specifically, we deployed ASUs across treatments with high vs. low habitat manipulations. We hypothesised that if the abundance of recruiting juvenile fishes was greater on the high habitat availability treatments this would suggest that the availability of habitat was limiting juvenile fish abundance. Our analyses were focused on the four most abundant fishes that settled on our ASUs: snapper (Pagrus auratus); spotty (Notolabrus celidotus); trevally (Pseudocaranx dentex); and an assemblage of pipefish species. For snapper, spotty and pipefish, abundance was greater on the high habitat availability treatments. This result would be unlikely to occur if the availability of juvenile fishes was limiting, suggesting that juvenile fish abundance is more likely to be limited by habitat. In terms of spatial gradients, spotty abundance and size increased with distance into the harbour. The spatial gradient present for spotty indicates the importance of placing the habitat dependency of juvenile fish into a landscape context. Overall, these results demonstrate that maintaining structured habitats may be an important consideration for some valuable inshore fish populations in northeastern New Zealand.
Introduction
Many inshore fish species utilise structured habitats during critical early life phases (Beck et al. Citation2001; Thrush et al. Citation2001; Heck et al. Citation2003; Mumby et al. Citation2004; Dahlgren et al. Citation2006; Parsons et al. Citation2013) before moving to separate adult habitat. As such, juvenile habitats that provide a disproportionate supply of recruits to the adult population have been termed ‘nurseries’ (Beck et al. Citation2001).
The nursery habitats juvenile fish use are often located in shallow, nearshore waters (Dahlgren et al. Citation2006) and these habitats are often comprised of living structural elements such as seagrass, sponges or bivalves (Beck et al. Citation2001). This makes these habitats particularly vulnerable to land-based impacts, for example sedimentation and eutrophication, as well as direct habitat disturbance. Fisheries, although focused on adult life stages, may directly benefit from management effort to ameliorate land-based effects on important juvenile habitat that may be separate from where adult fisheries occur.
Identifying which habitats make the largest contributions to adult populations (Gillanders & Kingsford Citation1996; Hamer et al. Citation2011) and whether certain habitats convey greater benefits (usually in terms of growth and survival) than others (Orth et al. Citation1984; Phelan et al. Citation2000; Heck et al. Citation2003) is a prudent approach in the research of important juvenile habitat. For species that are nearly always found associated with structured habitat as juveniles, however, describing whether less structured habitat will result in fewer juveniles may be more relevant than considering which structured habitats afford fish the greatest benefits. This may be especially true in light of the likely decline of structured habitats that juvenile fish utilise (Duarte Citation2002). In addition, the spatial context of where that structured habitat lies (both between and within estuaries) may also be relevant (Bell et al. Citation1988; Lipcius et al. Citation1997; Jelbart et al. Citation2007).
New Zealand estuaries and harbours provide important juvenile habitat for a number of inshore fish species (Francis et al. Citation2005; Francis et al. Citation2011). Seagrass beds within these harbours are one form of structured habitat that is utilised by a number of juvenile fishes (Francis et al. Citation2005). Globally, seagrass is under stress (Duarte Citation2002; Orth et al. Citation2006), and this trend is reflected by Zostera muelleri beds in New Zealand (Matheson et al. Citation2011). While the juvenile stages of multiple fish species utilise seagrass in New Zealand, seagrass decline is of particular concern for snapper (Sparidae, Pagrus auratus), an abundant fish in northern New Zealand that is the focus of important commercial and recreational fisheries. Juvenile snapper > 60 mm fork length (FL) are commonly observed across a variety of coastal habitats: low topography mud and sand (Francis Citation1995; Fowler & Jennings Citation2003; Hamer & Jenkins Citation2004); rocky reefs (Kingett & Choat Citation1981; Ross et al. Citation2007); and structured elements within soft sediment habitats (Thrush et al. Citation2002; Usmar Citation2009), but post settlement juveniles (c. 10–60 mm FL) have only been observed in high abundance over structured habitats within estuaries (especially seagrass) (Morrison Citation2008; Usmar Citation2009; Sim-Smith et al. Citation2012; Parsons et al. Citationin press) and rarely observed elsewhere (D. Parsons pers. obs.).
In the present study, we utilised artificial seagrass units (ASUs) to experimentally assess whether juvenile fish abundance was limited by habitat availability or the availability of settling juvenile fishes. Specifically, we hypothesised that the overall abundance of juvenile fishes would be greater on treatments with high habitat availability compared to treatments with low habitat availability. In addition, our experiment was deployed across a spatial gradient (in terms of distance from the harbour mouth) which allowed us to explore the relative importance of the spatial location of habitats for juvenile fishes within the estuary.
Materials and methods
Experiments were conducted within Whangapoua Harbour, Coromandel Peninsula, northeastern New Zealand (), because previous studies indicated consistent and strong fish recruitment within this harbour (M. Morrison pers. obs.). Whangapoua Harbour is a small (c. 13 km2) sand-barrier-lagoon type estuary within which all but the subtidal channels (c. 2 m depth) drain at low tide. Although extensive intertidal seagrass beds exist within the harbour, subtidal seagrass has not been present within the harbour since 2004–06 (M. Morrison pers. obs.). The area chosen for our experiment () was largely devoid of alternate structure that may attract juvenile fish, had no road access, experienced minimal boat traffic, and offered expanses of shallow subtidal flats of consistent depth within which to deploy ASUs.
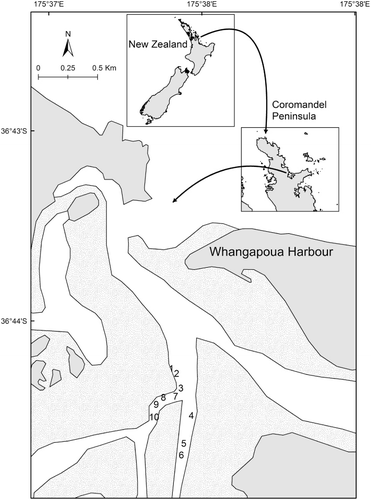
The ASUs that we deployed had dimensions of 1.8 × 1.8 m and were constructed on a metal grid. Seagrass was mimicked with individual plastic plants, each consisting of a rigid wire stem with multiple individual blades of c. 30 cm length. Each plant was attached at its base to the grid with cable ties. All ASUs had the same density of plastic plants (216 plants; equivalent to 1820 blades m−2). This density was the same as the ‘high’ density treatment used by Parsons et al. (Citation2013), and corresponds to the upper range of subtidal seagrass densities in northeastern New Zealand (M. Morrison, NIWA, unpubl. data).
A total of 60 ASUs were deployed across 10 sites within Whangapoua Harbour in early December 2011; about the time of peak snapper spawning (Scott et al. Citation1993), and therefore prior to the time when the majority of larval snapper should settle onto juvenile habitats. At each site ASUs were deployed within two treatments that had ‘high’ and ‘low’ habitat availability. Previous ASU deployments suggested that fish movement between ASUs was limited at 9 m separation (Parsons et al. Citation2013), so high and low treatments were conservatively separated by 25 m. Each high habitat availability treatment contained five ASUs (four on the vertices of a square with 5 m sides, with the fifth ASU in the centre) while each low habitat availability treatment consisted of a single ASU (physical layout of treatments is summarised in ). ASUs were secured in place with metal pegs driven into the substratum through eyelets on the edge of the ASU's base. The minimum water depth over ASUs on spring tides was c. 40 cm. This ensured that fish could remain on ASUs regardless of the tidal state, while also providing a tidal window within which we could effectively sample the ASUs.
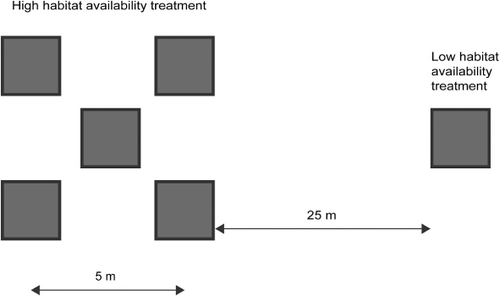
Sampling of juvenile fishes that had recruited to the ASUs was conducted once, over a two day period during mid-February. At this time, one ASU was missing (from site 8), so the entire site was abandoned. All ASUs at the remaining nine sites were sampled for juvenile fishes as described below.
Juvenile fishes were sampled using a netting method developed by Parsons et al. (Citation2013). This sampling method is conceptually similar to a ‘brush and dust pan’, in that juvenile fish are ‘swept’ off the ASU with the brush (a fine meshed net weighted at the bottom with chain) and herded into the dustpan (a triangular fine meshed net with a floor). Sampling was conducted in wading depth water around low tide and performed twice on each ASU before haphazardly selecting another ASU to sample. We were conscious that disturbance during the sampling process may influence capture efficiency, particularly at high habitat availability treatments where fish could potentially avoid capture by moving between ASUs. Therefore, we were careful not to disturb ASUs that had not yet been sampled. Furthermore, underwater observations suggested that only limited fish movement between ASUs was likely while fish sampling was being conducted (D. Parsons pers. obs.). If it did occur it should result in underestimates for high habitat availability treatments, making predictions about the ability of habitat to increase fish abundance conservative. All fish captured during this process were immediately bagged and labelled according to the particular site, treatment and ASU. Fish were later identified and measured in the laboratory.
The experimental design described above was based on Stier & Osenberg (Citation2010). While Stier & Osenberg (Citation2010) were able to specifically focus their empirical tests on fish as they settled (i.e. the field-of-dreams vs. redirection hypotheses), in the present experiment settlers were allowed to accumulate over a 2 month period before being sampled. As such, the present study does not make inferences regarding the redirection of fish larvae, but does allow us to ask if the abundance of juvenile fish is limited by the availability of habitat or the availability of settling larvae/juveniles? Because habitat was 5 times more abundant within our high treatments, a 5:1 ratio in observed fish abundance would suggest that habitat and fish abundance increase proportionally. Alternatively, if fish abundance was the same across high availability and low availability treatments (a 1:1 ratio), this would suggest that fish abundance was fully limited by the availability of juvenile fishes. A partial increase in juvenile fish abundance on high habitat availability treatments (i.e. between the two ratios stated above) would still indicate that additional habitat increases the abundance of juvenile fishes. We used t-tests for each species to compare fish abundance for high vs. low habitat availability treatments (e.g. high/low) to the 1:1 ratio expected if additional habitat did not increase fish abundance. We added 1 to all fish abundances because of the presence of zero abundances for some replicates.
To compare the length frequency distributions of fish captured from the high and low habitat availability treatments, separate kernel density estimates (KDEs) were constructed for each species. This method provides a data driven method for approximating length frequency data with probability density functions without assigning the data into arbitrarily chosen bin increments (Langlois et al. Citation2012). Statistical tests were based on a null model of no difference and a permutation test where the geometric mean between bandwidths for high vs. low habitat availability treatments was calculated for each species. The statistical test compared the area between the pair of KDEs from high vs. low habitat availability treatments to that resulting from permutations of the data into random pairs. This method is described in detail by Langlois et al. (Citation2012).
The influence of location within the harbour on abundance and mean length of fish recruiting to ASUs was assessed with linear regressions for each species (where distance from the ASU closest to the harbour mouth was the independent variable). This allowed us to address whether the abundance and size of juvenile fish was influenced by environmental gradients that may exist as distance to the mouth of the harbour increased. The dependent variables for these regressions (abundance and mean fish length) were calculated by pooling the fish captured at each site (i.e. combining data across the high and low habitat availability treatments at each site). The assumptions of normality and heterogeneity of variance were assessed for each regression and transformations (square root and log) were performed where required.
Results
A total of 1029 juvenile fishes from 11 species or species groups were captured from the single mid-February sampling event of ASUs deployed in Whangapoua Harbour (). Juvenile snapper accounted for more than half of all fish caught, but four other species or species groups were present on most ASUs. Of these species or groups we excluded yellow eyed mullet (Aldrichetta forsteri) as they are pelagic and not exclusively associated with ASUs and many of the individuals captured were not recently settled juveniles. This left four species or groups (hereafter the focal species) where abundance was reasonable, for which we concentrated our analyses: snapper (Pagrus auratus); spotty (Notolabrus celidotus); pipefish (an assemblage of species from the genera Stigmatopora, Leptonotus and Lissocampus); and trevally (Pseudocaranx dentex).
Table 1 Juvenile fish species (and their abundances) captured from ASUs deployed at sites within Whangapoua Harbour. All fish were captured from a single, 2 day sampling event during mid-February. Pipefish and triplefins were not identified to the species level but likely consist of multispecies assemblages from the genera Stigmatopora, Leptonotus and Lissocampus (pipefish), and Forsterygion and Grahamina (triplefin).
The relative abundance of the four focal species (across high and low habitat availability treatments) is presented in . Juvenile snapper, spotty and pipefish were caught roughly in proportion to the amount of habitat available and, despite high variability, these abundances were significantly greater than would be expected if fish abundance was fully limited by the availability of settling juvenile fishes (snapper: t = 2.45, df = 8, p < 0.02; spotty t = 2.02, df = 8, p < 0.04; pipefish t = 3.50, df = 8, p < 0.005). Trevally relative abundance was higher than the three other focal species. Trevally abundance was, however, highly variable and was not significantly greater than would be expected if fish abundance was fully limited by the availability of settling juvenile fishes (t = 1.67, df = 8, p > 0.06).
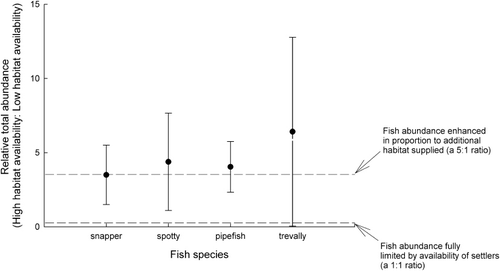
The length frequency distributions of the four focal species are presented in . There were no significant differences in the length distribution between high and low habitat availability treatments for three of the focal species (pipefish: p = 0.17; spotty p = 0.65; trevally: p = 0.6). For snapper, however, there was a significant difference in the length distributions for high and low habitat availability treatments (p < 0.01), but no significant difference once these distributions had been standardised (p > 0.08). This indicates that larger juvenile snapper were associated with the high habitat availability treatments, while the shape of the length frequency distributions were similar.
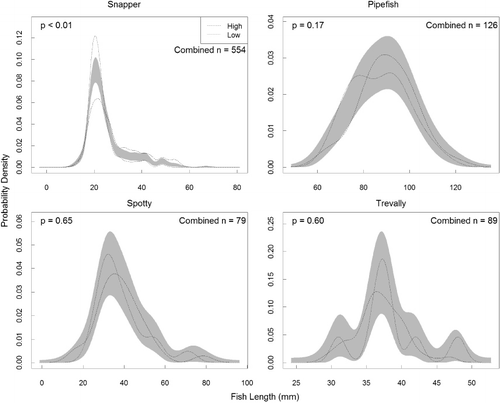
Gradients in abundance of the four focal fish species on ASUs within Whangapoua Harbour (pooled across high and low habitat availability treatments within each site) were assessed with linear regressions with the distance of each site from the site closest to the harbour mouth (). For snapper and trevally, abundance was highest at the site closest to the harbour mouth and displayed relatively little trend over the remaining sites. Pipefish similarly displayed little trend in abundance aside from a single site (at c. 500 m from the site closest to the harbour mouth) that had much higher abundance than the other sites. Spotty, however, demonstrated an increasing trend in abundance as distance from the harbour mouth increased (p = 0.01, r2 = 0.63).
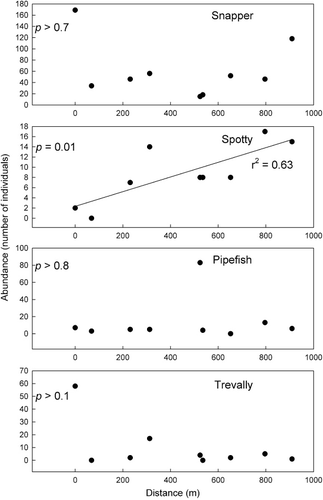
Gradients in the mean size of the four focal fish species were assessed with linear regressions as above for abundance (). Little trend in mean size was displayed for pipefish or trevally. Snapper mean size also had no significant trend apart from one site that had a much larger fish size situated c. 300 m from the site closest to the harbour mouth. Spotty mean size increased with distance from the harbour mouth (p = 0.01, r2 = 0.70).
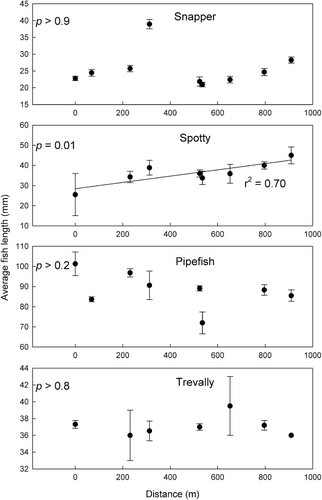
Discussion
We successfully attracted more than 1000 juvenile fishes from 11 different species to the ASUs deployed in Whangapoua Harbour. For three of the four focal species (snapper, spotty and pipefish) an increase in the availability of habitat resulted in an increase in fish abundance proportional to the amount of additional habitat. For juvenile snapper, where additional habitat was available, more large juvenile snapper were present. For the fourth focal species (trevally) there were indications that abundance did respond to additional habitat when it was present, but firm conclusions were prevented by high variability. Together, these results suggest that, at the level of habitat availability tested in the present study, declines in the extent of structured habitat within estuaries may also lead to reduced abundances of some juvenile fishes. Many previous studies have also demonstrated that additional structured habitat can elevate the abundance of settling fish (Peterson et al. Citation2003; Laurel et al. Citation2003a; McDermott & Shima Citation2006; White & Caselle Citation2008; Stier & Osenberg Citation2010), but the present study is the first to do so for an exploited New Zealand fish species.
In the present study, juvenile fishes were allowed to accumulate on ASUs over a 2 month period. As a result, the comparison of juvenile fish abundance between high and low habitat availability treatments incorporated density dependent and independent post-settlement processes which may have influenced our results. We have no indication as to whether these processes operated at different rates between low and high habitat availability treatments. It may be possible, however, that our results were influenced by the existence of density dependent processes where habitat availability was lower (i.e. on the low habitat availability treatment). If this were true, it in itself implies that the availability of juvenile fish was in excess of the availability of habitat. This is consistent with our interpretation that our results present a test of whether the availability of settling juvenile fishes is limited, or whether the amount of habitat is limited. Furthermore, in a realistic setting, juvenile fishes will accumulate on structured habitat as new settlers arrive, so the present study reflects how habitat would be utilised in nature.
Aside from just offering additional habitat, our experimental manipulations also presented an altered landscape of habitat to juvenile fishes. The majority of previous studies that have investigated the response of fishes to seagrass landscape attributes did not demonstrate a relationship, but positive and negative responses have been observed for some species (see review by Connolly & Hindell Citation2006). In the present study, the patchy nature of the high habitat availability treatments may have reduced the encounter probability for settling larvae (McNeill & Fairweather Citation1993) because these treatments effectively have a reduced perimeter to area ratio (all five ASUs were encompassed within a 5 × 5 m area, so there is an effective perimeter to area ratio of 1.54 vs. 2.22 for the low habitat availability treatments). Alternatively, the larger total area of habitat offered at the high habitat availability treatments may have been able to support higher densities of predators and predation on juvenile fishes (Laurel et al. Citation2003b). The higher proportion of large snapper on high habitat availability treatments may suggest that differences in predation rates did occur between the treatments, but alternative explanations cannot be excluded. Regardless of the specific influence of landscape attributes for the juvenile fishes in the present study, if the availability of settling juveniles was not sufficient then an increased abundance of juvenile fishes would not have been observed within the high habitat availability treatments.
An aspect of seagrass landscapes that has frequently been observed as important to juvenile fishes is the position of habitat within an estuary (Connolly & Hindell Citation2006). In the present study, three of the four focal species showed no response, while the fourth (spotty) demonstrated higher abundance and mean length towards the upper harbour end of the c. 1 km gradient that the experimental sites encompassed. For the species where no pattern was observed, it is important to note that experimental sites were only located in the central portion of the harbour, so those species may have exhibited spatial gradients further towards or away from the harbour mouth. For spotty, the patterns exhibited could have either been the result of the availability and behaviour of settling larvae (Bell et al. Citation1988; Milicich et al. Citation1992) or post-settlement processes that modify survival and growth (Levin et al. Citation1997; Almany & Webster Citation2006). In a previous experiment conducted in Whangapoua Harbour, we also assessed spatial patterns in the settlement of snapper and spotty over a c. 200 m portion of the harbour (Parsons et al. Citation2013). In that study, no pattern in spotty colonisation was observed, but juvenile snapper were more abundant at the harbour mouth end of the ASU array. As ASUs were deployed over a smaller spatial extent and at a higher density than in the present study (9 m spacing), it is possible that these previous results may reflect a depletion effect (where the availability of settlers is reduced by the ASUs closest to the harbour mouth; Jones Citation1997; Stier & Osenberg Citation2010) rather than a gradient in the delivery or preference of settling juvenile snapper and spotty.
Managing the fishery removal of adult life stages is an obvious and necessary part of ensuring the sustainability of a resource. Potentially as important, however, is ensuring that all fish life history stages have the necessary habitat requirements to allow progression to the adult stage (Rosenberg et al. Citation2000). Furthermore, if the extent of critical habitat is restored (or habitat decline halted), this may also elevate the overall productivity of the fishery. The present study suggests that, for three fish species (one of which forms an important commercial and recreational fishery), additional structured habitat has the potential to increase the abundance of juvenile fishes. There were also strong indications that additional habitat had enhanced abundance for the fourth fish species investigated. While only demonstrated at small scales, our results are of particular relevance because structured habitat elements, such as seagrass, are threatened by land-based stressors such as urbanisation, forestry, farming and direct physical disturbance (Orth et al. Citation2006; Morrison et al. Citation2009). As such, the identification and protection or restoration of significant fishery habitats will be an important part of a broader ecosystem approach to fishery management if it is adopted in New Zealand.
Acknowledgements
Work conducted for this manuscript was funded by NIWA's Fisheries Programme 2 (2011/12 SCI) and Coasts and Oceans Programme 3 (2012/13 SCI). We thank Alistair Dunn, Simon Thrush, Mark Morrison, Bruce Hartill, Jeremy McKenzie, Ian Tuck and Timothy Langlois for project advice, as well as Keren Spong, Dane Buckthought, Marie Jordan and Bruce Hartill for assistance in the laboratory and field.
References
- Almany GR, Webster MS 2006. The predation gauntlet: early post-settlement mortality in reef fishes. Coral Reefs 25: 19–22. 10.1007/s00338-005-0044-y
- Beck MW, Heck KL, Able KW, Childers DL, Eggleston DB, Gillanders BM et al. 2001. The identification, conservation, and management of estuarine and marine nurseries for fish and invertebrates. BioScience 51: 633–641. 10.1641/0006-3568(2001)051[0633:TICAMO]2.0.CO;2
- Bell JD, Steffe AS, Westoby M 1988. Location of seagrass beds in estuaries: effects on associated fish and decapods. Journal of Experimental Marine Biology and Ecology 122: 127–146. 10.1016/0022-0981(88)90180-3
- Connolly RM, Hindell JS 2006. Review of nekton patterns and ecological processes in seagrass landscapes. Estuarine, Coastal and Shelf Science 68: 433–444. 10.1016/j.ecss.2006.01.023
- Dahlgren CP, Kellison GT, Adams AJ, Gillanders BM, Kendall MS, Layman CA et al. 2006. Marine nurseries and effective juvenile habitats: concepts and applications. Marine Ecology Progress Series 312: 291–295. 10.3354/meps312291
- Duarte CM 2002. The future of seagrass meadows. Environmental Conservation 29: 192–206. 10.1017/S0376892902000127
- Fowler AJ, Jennings PR 2003. Dynamics in 0+ recruitment and early life history for snapper (Pagrus auratus, Sparidae) in South Australia. Marine and Freshwater Research 54: 941–956. 10.1071/MF02172
- Francis MP 1995. Spatial and seasonal variation in the abundance of juvenile snapper (Pagrus auratus) in the north-western Hauraki Gulf. New Zealand Journal of Marine and Freshwater Research 29: 565–579. 10.1080/00288330.1995.9516688
- Francis MP, Morrison MA, Leathwick J, Walsh C 2011. Predicting patterns of richness, occurrence and abundance of small fish in New Zealand estuaries. Marine and Freshwater Research 62: 1327–1341. 10.1071/MF11067
- Francis MP, Morrison MA, Leathwick J, Walsh C, Middleton C 2005. Predictive models of small fish presence and abundance in northern New Zealand harbours. Estuarine, Coastal and Shelf Science 64: 419–435. 10.1016/j.ecss.2005.03.007
- Gillanders BM, Kingsford MJ 1996. Elements in otoliths may elucidate the contribution of estuarine recruitment to sustaining coastal reef populations of a temperate reef fish. Marine Ecology Progress Series 141: 13–20. 10.3354/meps141013
- Hamer PA, Acevedo S, Jenkins GP, Newman A 2011. Connectivity of a large embayment and coastal fishery: spawning aggregations in one bay source local and broadscale fishery replenishment. Journal of Fish Biology 78: 1090–1109. 10.1111/j.1095-8649.2011.02921.x
- Hamer PA, Jenkins GP 2004. High levels of spatial and temporal recruitment variability in the temperate sparid Pagrus auratus. Marine and Freshwater Research 55: 663–673. 10.1071/MF04024
- Heck KL, Hays G, Orth RJ 2003. Critical evaluation of the nursery role hypothesis for seagrass meadows. Marine Ecology Progress Series 253: 123–136. 10.3354/meps253123
- Jelbart JE, Ross PM, Connolly RM 2007. Patterns of small fish distributions in seagrass beds in a temperate Australian estuary. Journal of the Marine Biological Association of the United Kingdom 87: 1297–1307. 10.1017/S0025315407053283
- Jones GP 1997. Relationships between recruitment and postrecruitment processes in lagoonal populations of two coral reef fishes. Journal of Experimental Marine Biology and Ecology 213: 231–246. 10.1016/S0022-0981(96)02763-3
- Kingett PD, Choat JH 1981. Analysis of density and distribution patterns in Chrysophrys auratus (Pisces: Sparidae) within a reef environment: an experimental approach. Marine Ecology Progress Series 5: 289–290. 10.3354/meps005283
- Langlois TJ, Fitzpatrick BR, Fairclough DV, Wakefield CB, Hesp SA, McLean DL et al. 2012. Similarities between line fishing and baited stereo-video estimations of length-frequency: novel application of kernel density estimates. PLoS ONE 7: e45973. 10.1371/journal.pone.0045973
- Laurel BJ, Gregory RS, Brown JA 2003a. Settlement and distribution of Age-0 juvenile cod, Gadus morhua and G. ogac, following a large-scale habitat manipulation. Marine Ecology Progress Series 262: 241–252. 10.3354/meps262241
- Laurel BJ, Gregory RS, Brown JA 2003b. Predator distribution and habitat patch area determine predation rares on Age-0 juvenile cod Gadus spp. Marine Ecology Progress Series 251: 245–254. 10.3354/meps251245
- Levin P, Petrik R, Malone J 1997. Interactive effects of habitat selection, food supply and predation on recruitment of an estuarine fish. Oecologia 112: 55–63. 10.1007/s004420050283
- Lipcius RN, Stockhausen WT, Eggleston DB, Marshall LSJ, Hickey B 1997. Hydrodynamic decoupling of recruitment, habitat quality and adult abundance in Caribbean spiny lobster: source-sink dynamics. Marine and Freshwater Research 48: 807–815. 10.1071/MF97194
- Matheson FE, Lundquist CJ, Gemmill CEC, Pilditch CA 2011. New Zealand seagrass: more threatened than IUCN review indicates. Biological Conservation 144: 2749–2750. 10.1016/j.biocon.2011.08.020
- McDermott CJ, Shima JS 2006. Ontogenetic shifts in microhabitat preference of the temperate reef fish Forsterygion lapillum: implications for population limitation. Marine Ecology Progress Series 320: 259–266. 10.3354/meps320259
- McNeill SE, Fairweather PG 1993. Single large or several small marine reserves? An experimental approach with seagrass fauna. Journal of Biogeography 20: 429–440. 10.2307/2845591
- Milicich MJ, Meekan MJ, Doherty PJ 1992. Larval supply: a good predictor of recruitment of three species of reef fishes (Pomacentridae). Marine Ecology Progress Series 86: 153–166. 10.3354/meps086153
- Morrison M 2008. Tracking snapper origins. Water and Atmosphere 16: 4.
- Morrison M, Lowe M, Parsons DM, Usmar NR, McLeod I 2009. A review of land-based effects on coastal fisheries and supporting biodiversity in New Zealand. New Zealand Aquatic Environment and Biodiversity Report No. 37. Ministry of Fisheries, Wellington. 100 p.
- Mumby PJ, Edwards AJ, Arias-Gonzalez JE, Lindeman KC, Blackwell PG, Gall A et al. 2004. Mangroves enhance the biomass of coral reef fish communities in the Caribbean. Nature 427: 533–536. 10.1038/nature02286
- Orth RJ, Heck Jr KL, van Montfrans J 1984. Faunal communities in seagrass beds: a review of the influence of plant structure and prey characteristics on predator-prey relationships. Estuaries 7: 339–350. 10.2307/1351618
- Orth RJ, Carruthers TJB, Dennison WC, Duarte CM, Fourqurean JW, Heck KL et al. 2006. A global crisis for seagrass ecosystems. Bioscience 56: 987–996. 10.1641/0006-3568(2006)56[987:AGCFSE]2.0.CO;2
- Parsons D, Morrison M, Thrush SF, Middleton C, Smith M, Keren S et al. 2013. The influence of habitat structure on juvenile fish in a New Zealand estuary. Marine Ecology 34: 492–500.
- Peterson CH, Grabowski JH, Powers SP 2003. Estimated enhancement of fish production resulting from restoring oyster reef habitat: quantitative valuation. Marine Ecology Progress Series 264: 249–264. 10.3354/meps264249
- Phelan BA, Goldberg R, Bejda AJ, Pereira J, Hagan S, Clark P et al. 2000. Estuarine and habitat-related differences in growth rates of young-of-the-year winter flounder (Pseudopleuronectes americanus) and tautog (Tautoga onitis) in three northeastern U.S. estuaries. Journal of Experimental Marine Biology and Ecology 247: 1–28. 10.1016/S0022-0981(99)00184-7
- Rosenberg A, Bigford TE, Leathery S, Hill RL, Bickers K 2000. Ecosystem approaches to fishery management through essential fish habitat. Bulletin of Marine Science 66: 535–542.
- Ross PM, Thrush SF, Montgomery JC, Walker JW, Parsons DM 2007. Habitat complexity and predation risk determine juvenile snapper (Pagrus auratus) and goatfish (Upeneichthys lineatus) behaviour and distribution. Marine and Freshwater Research 58: 1144–1151. 10.1071/MF07017
- Scott SG, Zeldis JR, Pankhurst NW 1993. Evidence of daily spawning in natural populations of the New Zealand snapper Pagrus auratus (Sparidae). Environmental Biology of Fishes 36: 149–156. 10.1007/BF00002794
- Sim-Smith CJ, Jeffs AG, Radford CA 2012. Variation in the growth of larval and early juvenile snapper, Chrysophrys auratus (Sparidae). Marine and Freshwater Research 63: 1231–1243. 10.1071/MF12176
- Stier AC, Osenberg CW 2010. Propagule redirection: habitat availability reduces colonization and increases recruitment in reef fishes. Ecology 91: 2826–2832. 10.1890/09-1993.1
- Thrush SF, Hewitt JE, Funnell GA, Cummings VJ, Ellis J, Schultz D et al. 2001. Fishing disturbance and marine biodiversity: role of habitat structure in simple soft-sediment systems. Marine Ecology Progress Series 221: 255–264. 10.3354/meps221255
- Thrush SF, Schultz D, Hewitt JE, Talley D 2002. Habitat structure in soft-sediment environments and abundance of juvenile snapper Pagrus auratus. Marine Ecology Progress Series 245: 273–280. 10.3354/meps245273
- Usmar NR 2009. Ontogeny and ecology of snapper (Pagrus auratus) in an estuary, the Mahurangi Harbour. Unpublished PhD thesis. Auckland, University of Auckland. 173 p.
- White JW, Caselle JE 2008. Scale-depdendent changes in the importance of larval supply and habitat to abundance of a reef fish. Ecology 89: 1323–1333. 10.1890/07-0840.1