Abstract
A ridge-like structure on the Campbell Plateau southeast of New Zealand, previously identified by Squires as a coral coppice, has been relocated (50° 41.46′S, 167° 41.45′E) and its extent and morphology mapped using multibeam and backscatter data obtained during an opportunistic survey. There are at least three other similar features at this location on a seafloor bank, although only two were fully mapped. ‘Squires’ Coral Coppice' is extensive, measuring 6700 m long, 900 m wide and up to 50 m high relative to the surrounding seafloor, in water depths of 273–325 m. Bathymetric profiles, backscatter data and historical samples of deep-sea coral (mainly Goniocorella dumosa) were used to infer that the ridge-like structures are coral reefs. The presence of deep-water coral reefs in the area could be due to the unique combination of geological (hard substrata), biological (high productivity) and oceanographic (relatively strong eastward flowing currents, seasonal vertical mixing) conditions east of Auckland Island. Squires' Coral Coppice covers an area of 9.2 km2 which makes it similar in size to some of the deep-water reefs and mounds in the North Atlantic, and it could represent the only known large coral reef (> 1 km maximum continuous extent) in the Southern Hemisphere. Further surveys are required to visually confirm that the relocated features are coral reefs, and to determine if similar features/coral reefs occur elsewhere on the Campbell Plateau. Deep-water coral reefs can support high levels of biodiversity and are vulnerable marine ecosystems that should be considered for protection in the region.
Introduction
Deep-water scleractinian corals that form complex three-dimensional structures are found in many areas around the world (Hovland Citation2008; Roberts et al. Citation2009a). Many of these structure-forming corals are located on the North-East Atlantic Margin, predominantly at shelf breaks and on the upper continental slope (Roberts et al. Citation2006). Some structures can be very large, forming ‘reefs’ that extend over kilometres in length (Fosså et al. Citation2005), while ‘coral carbonate mounds’ and other patch-like structures such as ‘thickets’ typically occupy areas of 1–10 km2 and < 1 km2, respectively (Wheeler et al. Citation2007). The presence of deep-water coral-dominated structural features is related to conditions particularly favourable for corals, for example, high nutrient and food supply for growth, currents or mixing to deliver the food and nutrients, and low sedimentation rates to allow efficient feeding and to avoid physical burial (see relevant references in Roberts et al. Citation2009a). Deep-water corals can be fragile, slow growing and long-lived (Adkins et al. Citation2004; Reed et al. Citation2007; Roark et al. Citation2009), and the biodiversity associated with these coral structures can be high (Jensen & Frederiksen Citation1992; Henry & Roberts Citation2007). These habitats are considered to be ‘vulnerable marine ecosystems’ that require protection (FAO Citation2009) from the impacts of deep-water fishing and drilling/mining (Fosså et al. Citation2002; Hall-Spencer et al. Citation2002; Van Dover Citation2011; White et al. Citation2012). Nearly all known deep-water coral reefs and mounds have been reported from the Northern Hemisphere. In the Southern Hemisphere, smaller patch reefs have been directly observed on many seamounts around New Zealand, Australia and Argentina (Althaus et al. Citation2009; Clark & Rowden Citation2009; Muñoz et al. Citation2012). However, the only indications that deep-water coral-dominated habitat in the Southern Hemisphere may occupy similar-sized areas to reefs or mounds found in the Northern Atlantic Ocean are the coral mounds recently found on the Uruguayan shelf and slope (Carranza et al. Citation2012) and the enigmatic and early record provided by Squires (Citation1965).
A coral ‘coppice’ (a term used to describe an intermediate phase in development between thicket and ‘bank’, now largely replaced in the ecological literature by either the term ‘mound’ or ‘reef’ depending on the genesis of the feature) was discovered during the course of a survey undertaken by the New Zealand Oceanographic Institute from the HMNZS Endeavour in January 1964. A ‘local bathymetric high’ was located using the ship's echo-sounder which showed 40 m relief from the surrounding seafloor of 334 m (Squires Citation1965). The lateral extent of this feature was not determined by the survey although the width was tentatively estimated to be up to 700 m. A large quantity of living and dead deep-water corals (primarily the scleractinian framework-building species Goniocorella dumosa) along with an abundance of ophiuroids, asteroids, anemones and molluscs were collected at a nearby trawl station. Together, these observations led Squires (Citation1965) to propose the existence of the coral coppice at 50° 38′S, 167° 38′E on the Campbell Plateau. Subsequent attempts to accurately relocate this coral-dominated feature failed, and therefore its full areal extent, and likely importance as a coral ecosystem in the region, has remained unknown.
Here, we: provide new multibeam and sub-bottom profile data that maps the dimensions and shape of Squires' Coral Coppice and other ridge-like structures; provide evidence that suggests these structures are coral reefs; discuss the local geological, biological and oceanographic conditions that could be responsible for the existence of a large deep-water coral reef ecosystem in the Southern Hemisphere; and consider the prospects for its further study and protection.
Methods
Study area
The Campbell Plateau is a large submarine plateau to the southeast of New Zealand. It originated in the Gondwanan breakup and is part of a largely submerged continent (Mortimer Citation2004). Most of the plateau sits at 600–1000 m, but comprises a number of broad rises shoaling to 250 m and depressions down to 1400 m. At the edge of the plateau the seafloor drops from 1000 to 4000 m. There are a number of island groups on the plateau including the volcanic Auckland and Campbell Islands (Gamble et al. Citation1986). The first recorded location for Squires' Coral Coppice is approximately 85 km east of the Auckland Islands ().
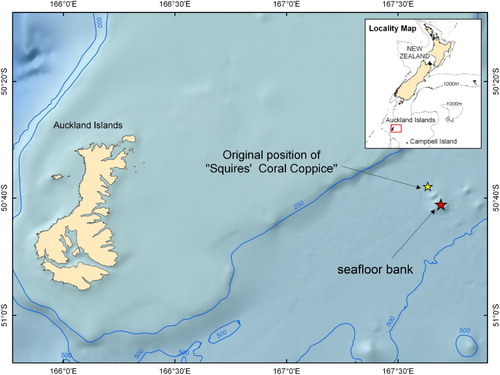
Pre-survey bathymetric analysis
In order to improve the chances of locating Squires' Coral Coppice by multibeam survey, a bathymetric model of the Campbell Plateau was first constructed. This digital elevation model was made from c. 500,000 depth soundings acquired by single-beam echo-sounder data from previous surveys that used GPS navigation in the area around Auckland and Campbell Islands, supplemented with transit multibeam echo-sounder data and soundings from published hydrographic charts. The model, made using the TOPOGRID tool in ArcGIS based on the ANUDEM program (Hutchinson Citation1989), showed a flat seafloor at the location published by Squires (Citation1965) but a seafloor bank approximately 12 km to the southeast of this location which was identified as the likely location for the coppice ().
The survey
Detailed seafloor mapping around the likely location of the coppice was undertaken opportunistically during the ‘Solander Trough’ voyage (TAN1106) of the RV Tangaroa in April 2011 (Bostock Citation2011). A Kongsberg EM302 multibeam system was used which comprises 288 beams/432 soundings per swath, with real-time beam steering compensating for ship motion. Motion sensing and positional navigation have accuracies of ≤ 0.028 and ± 5 m, respectively (Kleiner et al. Citation2001). Multibeam backscatter and bathymetry data were edited and processed using CARIS HIPS v7.0, then gridded at 25 m cell-size, with the latter having a vertical resolution of c. 1–2 m within 2000 m water depth.
The Tangaroa surveyed with the multibeam system, starting along a line drawn over the centre of the bank located on the bathymetric model. A ridge-like seafloor structure was seen upon approaching the bank, and the ship's track was altered to follow the axis of the ridge-like structure until the structure terminated. A further multibeam survey line was then run along this line over the ridge-like structure axis, crossing over the shallowest point. The ship's 3.5 kHz Knudsen chirp sub-bottom profiler was operated during this transit. The Tangaroa then proceeded to the location published by Squires (Citation1965) to re-confirm that there were in fact no structures located at that position.
No CTD station was occupied during the opportunistic survey of the target site. Water column profiles and samples for nutrients were collected with a 24 rosette Sea-bird CTD (SBE 911plus) at 51°S, 166.89°E (CTD station U6932) 105 km southwest of the target site as part of the main objectives of the Solander Trough voyage. Water samples were analysed for nutrients using standard methods (Woodward & Rees Citation2001; Law et al. Citation2011). There are no nearer CTD stations to the target site at which fluorescence data were obtained.
Data analysis
To assess the nature and extent of the bathymetric features, and determine whether the ridge-like structures detected by the TAN1106 survey include the same feature described by Squires (Citation1965), a number of tests were conducted. Initially, a comparison of bathymetry profiles between the 1964 EDO echo-sounder track and 2011 3.5 kHz Knudsen chirp sub-bottom profiler track was undertaken.
Bathymetric analysis was then undertaken in Fledermaus v7 and ArcGIS v10 software to determine the slopes of the ridge-like structures and the gradient of the surrounding seafloor. This analysis used both the processed multibeam data from TAN1106 as well as the historic single-beam echo-sounder dataset from the adjacent area with survey lines 5–12 km apart, which ran either side of the Squires' Coral Coppice location.
In addition, backscatter data were visually examined to determine any variation in reflectivity strength indicative of changes in substrate type, and whether any such changes were consistent with a change in the slope gradient from the bathymetric analyses. The 3.5 kHz Knudsen chirp sub-bottom profiler data was also used to identify any sub-surface structure around and through the ridge-like features.
Historical ground-truth data
It was not possible to take seabed samples or images during the 2011 survey, so data from six dredge samples and two camera deployments taken at stations in the vicinity of this feature during a previous attempt to relocate Squires' Coral Coppice in 1978 (New Zealand Oceanographic Institution [NZOI] cruise # 1080) were examined to provide ‘historical ground-truthing’ (). During this survey the position of stations was fixed using TRANSIT satellite navigation, which had an achievable accuracy of 0.25 to 0.5 nautical miles for a single-frequency receiver (Wood et al. Citation2003). The TRANSIT satellites were not in evenly spaced orbits, and the time between fixes was often more than 6 h during cruise # 1080, with navigation between fixes estimated using dead-reckoning. Comments made in the archived station logbook and voyage report, as well as the ship's navigation files, were examined to provide additional information. None of the aforementioned data have been published previously as a means to confirm the existence of Squires' Coral Coppice.
Results
Location
Bathymetric profiles obtained from both the 3.5 kHz Knudsen chirp sub-bottom profiler and the EM302 multibeam data were almost identical to the one obtained by the single beam survey conducted by Squires (Citation1965) (), suggesting that this is the same seafloor structure found by the HMNZS Endeavour in 1964. The position of this particular feature is 50° 41.46′S, 167° 41.45′E, 12.2 km southeast of the position recorded by Squires (Citation1965). Bathymetric data acquired by multibeam at the location published by Squires (Citation1965) shows an absence of elevated features and a flat, smooth seafloor at 420 m water depth.
General bathymetric morphology
Together, the digital elevation model and multibeam bathymetry show a seafloor bank 26 km from the Auckland Island shelf break. The basal depths surrounding the bank vary from 440 m on the southern margin to 415 m on the northern margin and rises up to an approximate depth of 340 m in the centre. Upon this bank are four ridge-like structures, of which only two are mapped in their entirety, aligned approximately west–east, and rising up a maximum of 50 m from the top of the bank ().
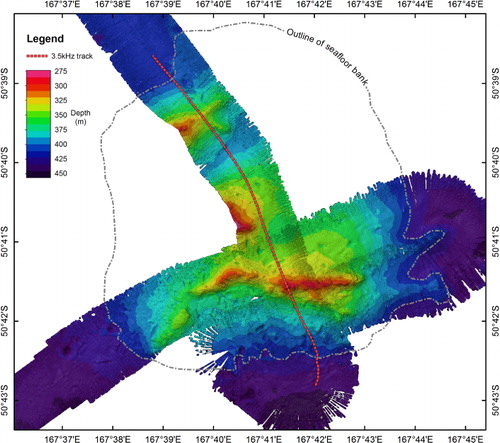
Coral reef interpretation
The ridge-like structures are delineated by a sharp increase in slope relative to the surrounding seafloor (8–19° compared to 1–2°) (). Sub-bottom profiles over the main ridge-like structure showed some penetration of the seafloor with weak reflectors along the lower slope of the bank and between the ridge structures, indicating sediment layers (). There was no sub-bottom penetration on the ridge-like structures themselves, consistent with a reflective substratum. Backscatter from the multibeam showed low reflectivity for the seafloor surrounding the ridge-like structures (), consistent with sandy foraminiferal ooze that is typical of the Campbell Plateau (Summerhayes Citation1969; Carter et al. Citation2000; Neil et al. Citation2004). The ridge-like structures themselves exhibited slightly higher reflectivity, with values consistent with a biogenic substratum, such as a coral reef (Roberts et al. Citation2009a), rather than volcanic or sedimentary bedrock. The area of higher reflectivity extended beyond the slope declination, but has a mottled texture and this area is interpreted as being coral rubble (i.e. the remains of coral that form at the periphery of living coral reefs) (Roberts et al. Citation2009b) ().
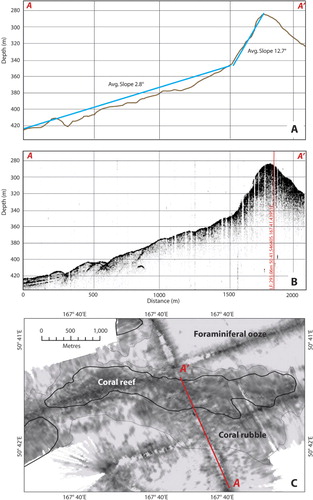
The seafloor at 400–500 m water depth to the south and west of the bank was marked by numerous grooves up to 20 m deep and up to 900 m long. These grooves are interpreted as iceberg scour marks (Brodie & Dawson Citation1971; Carter et al. Citation2002).
Coral reef extent
The spatial extents of what are interpreted as coral reefs were determined within ArcGIS v10 using the maximum change in the slope to define the base of the reef structures. The extent of the structures, termed R1–R4 (), were determined as follows: the main Squires' Coral Coppice (R1) is approximately 6700 m long (in an east–west direction) and 900 m wide and up to 50 m high relative to the surrounding seafloor of the bank, rising up to a shallowest depth of 273 m (R1: 273–325 m); a smaller structure (R2) extending off the western end of the main structure R1 and down the southern slope of the bank is 1800 m long, 700 m wide and 25 m high, rising to a depth of 345 m (S2: 345–370 m); the two structures (R3 and R4) that were partially surveyed appear to have the same east–west orientation as the other features and, although their summits were not mapped, are both a minimum of 45 m high relative to the surrounding seafloor of the bank (R3: 275–325 m; R4: 296–342 m) ().
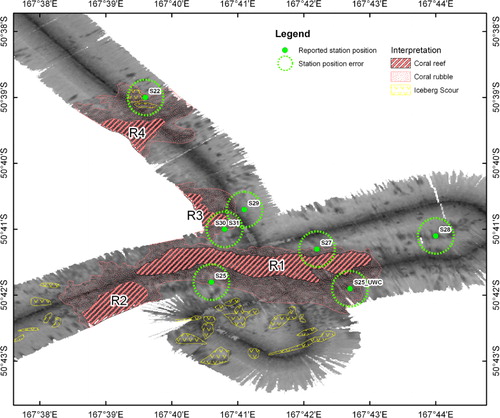
Coral reef ground-truthing
Dredge station S28 was located east of the ridge-like structures in relatively deep water on what is interpreted from the backscatter as foraminerferal ooze (). The dredge from this station was the only historical sample that included taxa typical of soft sediments (i.e. holothurians, sipunculids) and thus supports the backscatter interpretation at this location. Dredge stations S22, S25, S27 appear to have been taken from locations that are interpreted from the backscatter as coral rubble (). The recorded depths of these stations (400 m, 339 m and 335 m) are consistent with the dredges having been taken from the periphery of the ridge-like features (and are a reasonably close match with the depths recorded by new multibeam bathymetry–400 m, 373 m and 343 m). Faunal samples from these dredges were diverse (decapods, hydrozoans, anemones, asteroids, crinoids, ophiuroids, sponges, bivalves, gastropods, stony corals and sponges), and included some specimens of the deep-water coral Goniocorella dumosa. The abundance of which is described in the station log for S22 and S25 as ‘… but little Goniocorella’ and ‘some Goniocorella’. A diverse fauna but with only small amounts of living coral is consistent with these dredges being taken from what is interpreted as coral rubble habitat. The camera deployed at S25 was, according to the station log, positioned east of the dredge deployment and in deeper water (417 m in the logbook) and so, despite its apparent position in the coral rubble zone (see S25_UWC in ), it is more likely that the photographs were taken to the east (the ship's navigation data indicates that the ship was moving in this direction) on the substratum surrounding the presumed rubble. The seabed images recovered by the camera here confirm that, away from the supposed coral rubble, the surrounding seabed is foraminiferal ooze with a sparse fauna (). Dredges taken at stations S29 and S30 also appear to be located in or at the edge of the coral rubble zone (). However, these stations were recorded as being taken at depths of 300 m and 265 m, which is more consistent with the dredges being from a location that is interpreted from the backscatter as coral reef. The samples from these dredges included a high diversity of fauna (decapods, brachiopods, hydrozoans, sponges, polychaetes, anemones, crinoids, asteroids, ophiuroids, bivalves, gastropods, bryozoans) including numerous specimens of the deep-water coral Desmophylum dianthus and the matrix-forming scleractinian Goniocorella dumosa. The habitat-forming stony coral Solensimilia variablis was also recovered from station S30, with the station log recording a ‘large haul of angular rock with coral’ and describing the station as ‘the nearest thing to the original “coral bank”’. Thus, the contents of the dredges at these stations could be considered consistent with samples from a coral reef. However, it is also possible that they are from what is interpreted as coral rubble; the depth at these station positions is revealed by the new bathymetry to be 350 m and 340 m, which is more consistent with the dredges being from the periphery of the ridge-like structure rather than its peak. The camera station S31 was assigned the same position as the S30 in the logbook (‘navigationally identical’), but the depth was recorded as 275 m, which suggests that photographs of the seabed were taken somewhat downslope of the previous station and more likely at a position considered as coral rubble from the backscatter. The images from this station appear to confirm this interpretation, showing a very granular substratum with the larger remains of gastropods and brachiopods, on which there is an abundance of living ophiuroids ().
Water mass characteristics
The hydrographic and nutrient data obtained from the CTD cast are plotted in . There was a clear mixed layer down to a depth of 157 m, which has a temperature of 9.6 °C and a salinity of 34.5, high oxygen levels (265 µmol/L) and nutrients; phosphate 0.8 µmol/L, silicate 1.65 µmol/L and nitrate 14 µmol/L. High fluorometry measurements were also found within the mixed layer (0.6–1.5 µg/L (not calibrated with chlorophyll measurements). Below the mixed layer there was a peak in salinity at 175 m. There is significant mixing in the thermocline, with another water mass present above the seabed from 376–480 m. This lower water mass has temperature 8–8.1 °C, salinity 34.49, oxygen 240 µmol/L, high nutrients; phosphate 1.2 µmol/L, silicate 7 µmol/L, nitrate 22 µmol/L.
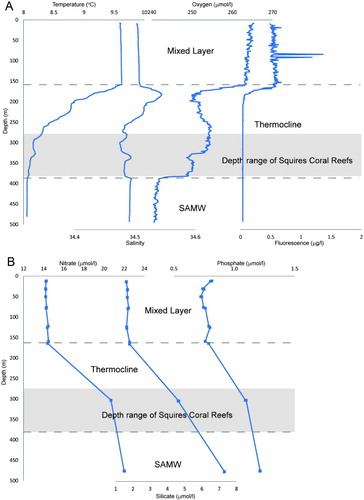
Discussion
The survey in 2011 provides convincing evidence to revise the location of a ridge-like structure originally discovered by Squires in 1964 on the Campbell Plateau, off New Zealand (Squires Citation1965). Modern geographical position fixing places this structure 12.2 km from the original recorded location. The survey revealed that there is more than one ridge-like structure in the general vicinity, which confirms the impression recorded in the voyage report from a previous relocation survey undertaken in 1978, but which failed to accurately locate the position of the coppice. In the context of all the bathymetric data recovered by surveys subsequent to the original finding (including the absence of any features at the original recorded position), and the similarity between the bathymetric profiles of the structure obtained by the 1964 and 2011 surveys, it is almost certain that the feature found by Squires has been rediscovered. Analysis of multibeam data, sub-bottom profile data and ‘historical ground-truth’ samples supports Squires' contention that the ridge-like structures could be coral reefs. These presumed coral reefs were determined to be large in extent, with Squires' Coral Coppice (R1) itself approximately 6700 m long and 900 m wide and up to 50 m high (covering an area of approximately 5.5 km2). In addition to the original feature, a further three structures were surveyed or partially surveyed. Together, the four surveyed structures cover a minimum area of 9.2 km2. The interpreted coral reefs occupied water depths of 273–370 m. This water depth and location is consistent with the recorded and predicted distribution of the habitat-forming coral Goniocorella dumosa (Tracey et al. Citation2011), the coral species that dominated the single sample recorded by Squires (Citation1965), and samples taken in the closest vicinity of the surveyed structures by the 1978 survey. Together, these observations provide evidence that the ridge-like structures east of the Auckland Islands are likely to be deep-water coral reefs. Further evidence for the probable existence of coral reefs is provided by the location of these features relative to the geological, biological and oceanographic characteristics of the region.
Structure- or habitat-forming deep-sea corals have been commonly found elsewhere in the New Zealand region, especially along the slope edge of the Chatham Rise and Campbell Plateau (approximately at the 1000 m depth contour) and on seamounts (Tracey et al. Citation2011), or the edges of shallower continental shelves and rises (approximately at the 250 m depth contour). This distribution of deep-sea corals is clearly evident around the Campbell Plateau (). Corals are known to favour shelf and slope break areas because this is where water currents are strong enough to deliver, or concentrate, sufficient food for these suspension-feeding organisms (Frederiksen et al. Citation1992; Mortensen & Buhl-Mortensen Citation2004; Bryan & Mataxas Citation2006; Thiem et al. Citation2006; Mortensen et al. Citation2001, Citation2008). In the study area, the Subantarctic Front and the associated fast flowing eastward moving Antarctic Circumpolar Current is deflected south around the edge of the plateau (Burling Citation1961; Heath Citation1981; Orsi et al. Citation1995; Carter & Wilkins Citation1999), while to the north of the plateau the Subtropical Front passes north of Auckland Island and forms the Southland Current which mixes warm, subtropical and coastal waters with cool, nutrient-rich subantarctic waters along the shelf edge (Heath Citation1972, Citation1981; Bradford & Taylor Citation1980; Sutton Citation2003; Smith et al. Citation2013). These currents transport nutrient-rich waters, which can deliver food to the corals along the slope and shelf edge. However, it is unknown whether corals form reef structures at these locations.
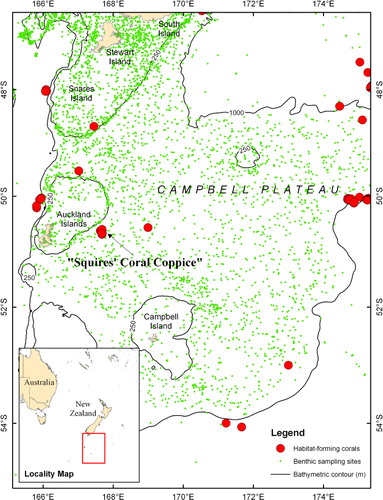
The suggested coral reefs on the top of the plateau are distant from these currents and fronts, but may be located there because of other unique oceanographic conditions that occur to the east of Auckland Island. The typically low chlorophyll levels on the Campbell Plateau (0.2 mg m−3) are interrupted by local phytoplankton accumulations around the subantarctic islands (Heath & Bradford Citation1980; Murphy et al. Citation2001). These year round plankton hotspots are especially evident in the SeaWiFS images northeast of the Auckland Islands between November to March where chlorophyll concentrations can reach > 1 mg m−3 (Murphy et al. Citation2001). These elevated chlorophyll concentrations around the islands may be due to increased flows and mixing generated by the predominantly eastward moving currents interacting with Auckland Island resulting in eddies or upwelling effects (Morris et al. Citation2001; Murphy et al. Citation2001).
The CTD data from April 2011 suggest that the water column in the general region of the presumed coral reefs is stratified, with an upper mixed layer of c. 160 m, which is due to seasonal heating (Morris et al. Citation2001; Neil et al. Citation2004). This stratification promotes phytoplankton blooms responsible for high chlorophyll a concentrations (Tyrrell & Merico Citation2004), as evident from the fluorescence data for this upper layer. The observed fluorescence is likely to be lower than the expected peak in summer, primarily because the voyage and these measurements were made at the end of the austral summer season when most of the nutrients had been used up (Boyd et al. Citation1999). The upper mixed waters overlay a mixed thermocline and the subantarctic mode waters, which exhibit low temperature stratification between 300–600 m water depth (Morris et al. Citation2001). While the water column is strongly stratified during summer, there is deep mixing over the plateau during winter, due to the strong westerly winds, and thus the water column on the plateau is only weakly stratified during the winter months (Morris et al. Citation2001; Neil et al. Citation2004). This winter mixing down to the base of the thermocline would help to transfer the phytoplankton and zooplankton (which feed on the phytoplankton) down to the depths where the Squires' Coral Coppice reefs are found. Downwelling has been demonstrated as an important food supply mechanism for corals at a reef complex in the North Atlantic (Davies et al. Citation2009).
Most stony corals require low sedimentation rates for successful settlement and to achieve extensive individual colony growth (Babcock & Mundy Citation1996). While the sub-bottom reflectors around the coral reefs indicate the accumulation of sedimentary layers, evidence from grab sampling shows that the Campbell Plateau is predominantly covered in sand-sized biogenic foraminiferal ooze (with echinoid spines, sponge spicules and glauconitic moulds of foraminifers), accumulating at a rate of 1–2 cm kyr−1 (Carter et al. Citation2000; Neil et al. Citation2004). There is very low input of terrigenous sediment (< 10%; Summerhayes Citation1969; Carter et al. Citation2000). The low sedimentation rates and limited terrigenous input prevent any chance of the reef-building corals being smothered by deposited sediment or suspended sediment interfering with the feeding efficiency of coral colonies attached to hard substrata on the plateau.
Although most deep-sea corals require some form of hard substrata on which to settle and grow, coral reefs and coral carbonate mounds have a different genesis. Reefs are ‘single-generation’, long-lived structures whose growth is balanced by (bio)erosion, while carbonate mounds are structures that accumulate through successive periods of reef development, sedimentation and (bio)erosion (Roberts et al. Citation2009a). The general morphology of reefs and carbonate mounds can be similar, but carbonate mounds can be distinguished from reefs because mounds usually occur in clusters arranged in ‘provinces’ along shelf and slope edges (Wheeler et al. Citation2007), and typically contain stratified sequences of reef deposits (De Mol et al. Citation2002). Although the 2011 multibeam survey identified a group of four structures, the regional digital elevation model, which also incorporates historic echo-sounder data, suggests that these features are isolated on a single local seafloor bank. Further support that the ridge-like structures are more likely to be reefs than mounds is provided by the sub-bottom profile data, which does not illustrate the conspicuous layering usually visible beneath coral carbonate mounds. A degree of sedimentation is required for significant mound development because baffling of sediment by the coral matrix serves to ‘grow’ the structure (Roberts et al. Citation2009a).
It is unclear from the survey what the interpreted coral reefs may have formed on. Iceberg scours were detected by the survey in the vicinity of the features, and although iceberg scour levees and flutes have been implicated in the initial formation of deep-water coral reefs elsewhere (Freiwald et al. Citation2002), they are unlikely to be important structuring agents on the Campbell Plateau because of the lack of coincidence between the geometry of the scours and the supposed reefs. Instead, the domed shape of the bank on which the ridge-like structures occur suggests that this bathymetric feature may be a granitic bedrock dome. There are several bathymetric banks, probably of similar foundation, identified elsewhere on the Campbell Plateau from other multibeam surveys and echo-soundings. Apart from one site 15 km northeast of Squires' Coral Coppice, these do not appear to have steep ridges indicative of coral reefs. There is a single record of Goniocorella dumosa collected 110 km east of Squires' Coral Coppice () that may be influenced by similar conditions as Squires' reefs, outlined here. Further surveys are required to better characterise the Squires' Coral Coppice features (and to see if other ridge-like features occur elsewhere on the bank) and determine whether other locations on the plateau could also support large coral reefs. Such surveys should include underwater cameras to confirm the structures are indeed constructed by habitat-forming corals, while deep-towed side-scan sonar and swath mapping will help refine the structure of any reefs, and seismic profiling and coring would confirm the genesis of any reefs and the substratum that they sit on.
The possible existence of large coral reefs at the Squires' Coral Coppice site, and potentially elsewhere in the area, will have important consequences for the protection of vulnerable marine ecosystems in the region. Where significant coral reef or coral carbonate mound structures occur in the Northern Hemisphere they have already been afforded protection from human activities at a number of locations. For example, Norway began to protect deep-water coral reefs in 1999 by closing a 978 km2 area of the seafloor (containing the Sula Ridge reef complex) to bottom trawling (Armstrong & van den Hove Citation2008); while in 2004 the European Commission closed a 1380 km2 area of the seafloor in order to protect the Darwin Mounds from the further impacts of bottom fishing (De Santo & Jones Citation2007). In the Southern Hemisphere, protection to smaller patch reefs has been granted in Australian and New Zealand waters. Off Australia, deep-water coral patch reefs on seamounts are conserved by a large marine reserve (12,780 km2) formed in 2007 (which includes a smaller area containing seamounts initially protected in 1999; www.environment.gov.au/coasts/mpa/southeast/huon/). In the New Zealand region, protection from bottom trawling is afforded only to small patch reefs on two of the Graveyard Seamounts on the Chatham Rise (Anon. Citation2001), although elsewhere unknown coral reefs may be similarly protected by nonspecific Benthic Protection Areas (Helson et al. Citation2010). Squires' Coral Coppice occurs in an area not covered by any form of protection and where bottom fishing effort is relatively high (Baird et al. Citation2011); as such it is vulnerable to disturbance. Considering the significance of coral reefs for biodiversity (e.g. Henry & Roberts Citation2007), including the provision of habitat for fishes (e.g. Söffker et al. Citation2011), it will be important to establish as soon as possible, and with absolute certainty, the existence of large deep-water coral reefs in the Southern Hemisphere and consider them as candidates for protection.
Conclusion
The location of Squires' Coral Coppice has been found along with additional data to support the possible existence of large coral reef structures atop a seafloor bank. The presence of coral reefs could be due to a unique combination of geological (hard substrata), biological (high productivity) and oceanographic (eastward flowing currents, seasonal vertical mixing) conditions east of Auckland Island on the Campbell Plateau. A further survey is required to confirm the presence and extent of the interpreted coral reefs and to examine in more detail the reasons for their possible occurrence. Surveys should also be conducted at locations where similar bathymetric highs occur on the Campbell Plateau, in case coral reefs occur elsewhere. If further survey confirms that Squires' Coral Coppice is a coral reef then it would be the only known Southern Hemisphere deep-water coral reef of similar size to the large deep-water reefs found in the North Atlantic. It is therefore likely to be an important deep-water marine ecosystem vulnerable to disturbance from bottom fishing, and as such should be considered for formal protection.
Acknowledgements
The authors would like to thank the captain, crew and scientists on the RV Tangaroa voyage TAN1106. We would also like to acknowledge the efforts of others who have tried in the past to find Squires' Coral Coppice, particularly Eliot Dawson the scientific leader of NZOI Southern Coral cruise #1080 who obtained the ‘historical ground-truth’ samples in 1978. This research was supported by NIWA's Consequences of Earth Ocean Change and Vulnerable Deep-Sea Communities projects (both funded by New Zealand's Ministry for Science and Innovation).
References
- Adkins JF, Henderson GM, Wang SL, O'Shea S, Mokadem F 2004. Growth rates of the deep-sea scleractinia Desmophyllum cristagalli and Enallopsammia rostrata. Earth and Planetary Science Letters 227: 481–490. 10.1016/j.epsl.2004.08.022
- Althaus F, Williams A, Schlacher TA, Kloser RK, Green MA, Barker BA et al. 2009. Impacts of bottom trawling on deep-coral ecosystems of seamounts are long-lasting. Marine Ecology Progress Series 397: 279–294. 10.3354/meps08248
- Anon. 2001. Seamount closures. Seafood New Zealand 9: 21.
- Armstrong C, van den Hove S 2008. The formation of policy for protection of coldwater coral off the coast of Norway. Marine Policy 32: 66–73. 10.1016/j.marpol.2007.04.007
- Babcock R, Mundy C 1996. Coral recruitment: consequences of settlement choice for early growth and survivorship in two scleractinians. Journal of Experimental Marine Biology and Ecology 206: 179–201. 10.1016/S0022-0981(96)02622-6
- Baird SJ, Wood BA, Bagley NW 2011. Nature and extent of commercial fishing effort on or near the seafloor within the New Zealand 200 n. mile Exclusive Economic Zone, 1989–90 to 2004–05. NZ Aquatic Environment and Biodiversity Report No. 73. New Zealand Ministry of Fisheries, Wellington.
- Bostock HC 2011. RV Tangaroa Voyage Report. TAN1106 - Solander Trough. 10 April–1 May 2011. Unpublished voyage report. New Zealand, National Institute of Water and Atmospheric Research.
- Boyd P, LaRoche J, Gall M, Frew R, McKay RML 1999. The role of iron, light and silicate in controlling algal biomass in sub-Antarctic water SE of New Zealand. Journal of Geophysical Research 104: 13395–13408. 10.1029/1999JC900009
- Bradford JM, Taylor FJ 1980. New Zealand region reactive phosphorous (October–April) surface. New Zealand Oceanographic Institute Chart Miscellaneous Series 46. Wellington, New Zealand Government Printer.
- Brodie JW, Dawson EW 1971. Antarctic icebergs near New Zealand. New Zealand Journal of Marine and Freshwater Research 5: 80–85. 10.1080/00288330.1971.9515370
- Bryan TL, Metaxas A 2006. Distribution of deep-water corals along the North American continental margins: relationships with environmental factors. Deep-Sea Research I 53: 1865–1879. 10.1016/j.dsr.2006.09.006
- Burling RW 1961. Hydrology of circumpolar waters south of New Zealand. New Zealand Oceanographic Institute Memoir 10, Wellington, NIWA. 66 p.
- Carranza A, Muñoz Recio A, Kitahara M, Scarabino F, Ortega L, López G et al. 2012. Deep sea coral reefs from the Uruguayan outer shelf and slope. Marine Biodiversity 42: 411–414. 10.1007/s12526-012-0115-6
- Carter L, Neil HL, McCave IN 2000. Glacial to interglacial changes in non-carbonate and carbonate accumulation in the SW Pacific Ocean, New Zealand. Palaeogeography, Palaeoclimatology, Palaeoecology 162: 333–365. 10.1016/S0031-0182(00)00137-1
- Carter L, Neil HL, Northcote L 2002. Late Quaternary incursions of icebergs into the southwest Pacific Ocean off eastern New Zealand. Marine Geology 191: 19–35. 10.1016/S0025-3227(02)00509-1
- Carter, L, Wilkin, J 1999. Abyssal circulation around New Zealand: a comparison between observations and a global circulation model. Marine Geology 159: 221–239. 10.1016/S0025-3227(98)00205-9
- Clark MR, Rowden AA 2009. Effect of deepwater trawling on the macro-invertebrate assemblages of seamounts on the Chatham Rise, New Zealand. Deep-Sea Research I 56: 1540–1544. 10.1016/j.dsr.2009.04.015
- Davies AJ, Duineveld GCA, Lavaleye MSS, Bergman MJN, van Haren H, Roberts JM 2009. Downwelling and deepwater bottom currents as food supply mechanisms to the cold-water coral Lophelia pertusa (Scleractinia) at the Mingulay Reef complex. Limnology and Oceanography 54: 620–629. 10.4319/lo.2009.54.2.0620
- De Mol B, Van Rensbergen P, Pillen S, Van Herreweghe K, Van Rooij D, McDonnell A et al. 2002. Large deep-water coral banks in the Porcupine Basin, southwest of Ireland. Marine Geology 188: 193–231. 10.1016/S0025-3227(02)00281-5
- De Santo EM, Jones PJS 2007. The Darwin Mounds: from undiscovered coral to the development of an offshore marine protected area regime. In: George, RY, Cairns SD ed. Conservation and adaptive management of seamount and deep-sea coral ecosystems. Miami, FL, Rosenstiel School of Marine and Atmospheric Science, University of Miami. Pp. 147–156.
- Food and Agriculture Organisation (FAO) 2009. International guidelines for the management of deep-sea fisheries in the high-seas. Rome, Food and Agriculture Organisation of the United Nations. 73 p.
- Fosså JH, Lindberg B, Christensen O, Lundälv T, Ingvald S, Mortensen PB et al. 2005. Mapping of Lophelia reefs in Norway: experiences and survey methods. In: Freiwald A, Roberts JM eds. Cold-water corals and ecosystems. Cambridge, UK, Cambridge University Press.
- Fosså JH, Mortensen PB, Furevik DM 2002. The deep-water coral Lophelia pertusa in Norwegian waters: distribution and fishery impacts. Hydrobiologica, 471: 1–12. 10.1023/A:1016504430684
- Frederiksen R, Jensen A, Westerberg H 1992. The distribution of the scleractinian coral Lophelia pertusa around the Faroe Islands and the relation to internal tidal mixing. Sarsia 77: 157–171.
- Freiwald A, Hühnerbach V, Lindberg B, Wilson JB, Campbell J 2002. The Sula Reef Complex, Norwegian Shelf. Facies 47: 179–200. 10.1007/BF02667712
- Gamble JA, Morris PA, Adams CJ 1986. The geology, petrology and geochemistry of Cenozoic volcanic rocks from the Campbell Plateau and Chatham Rise. In: Smith J ed. Late Cenozoic volcanism in New Zealand. Wellington, The Royal Society of New Zealand. Pp. 344–365.
- Hall-Spencer JV, Allain V, Fosså JH 2002. Trawling damage to Northeast Atlantic ancient coral reefs. Proceedings of the Royal Society B: Biological Sciences 269: 507–511. 10.1098/rspb.2001.1910
- Heath RA 1972. The Southland Current. New Zealand Journal of Marine and Freshwater Research 6: 497–533. 10.1080/00288330.1972.9515444
- Heath RA 1981. Oceanic fronts around southern New Zealand. Deep-Sea Research 28A: 547–560. 10.1016/0198-0149(81)90116-3
- Heath RA, Bradford JM 1980. Factors affecting the phytoplankton production over the Campbell Plateau, New Zealand. Journal of Plankton Research 2: 169–181. 10.1093/plankt/2.2.169
- Helson J, Leslie S, Clement G, Wells R, Wood R 2010. Private rights, public benefits: Industry-driven seabed protection. Marine Policy 34: 557–566. 10.1016/j.marpol.2009.11.002
- Henry LA, Roberts JM 2007. Biodiversity and ecological composition of macrobenthos on cold-water coral mounds and adjacent off mound habitat in the bathyal Porcupine Seabight, NE Atlantic. Deep-Sea Research I 54: 654–672. 10.1016/j.dsr.2007.01.005
- Hovland M 2008. Deep-water coral reefs: unique biodiversity hotspots. Chichester, UK, Praxis Publishing (Springer).
- Hutchinson MF 1989. A new procedure for gridding elevation and stream line data with automatic removal of spurious pits. Journal of Hydrology 106: 211–232. 10.1016/0022-1694(89)90073-5
- Jensen A, Frederiksen R 1992. The fauna associated with the bankforming deepwater coral Lophelia pertusa (Scleractinaria) on the Faroe shelf. Sarsia 77: 53–69.
- Kleiner A, Chance J, Ching N, Mitchell J, Smits F, Spittal J et al. 2001. A collaborative effort to meet New Zealand's mapping requirements: multibeam echosounder integration, acceptance testing, and Antarctic mapping aboard the research vessel Tangaroa. OCEANS 2001 MTS/IEEE Conference Proceedings, Vol. 2. Washington, DC, Marine Technology Society. Pp. 1248–1256. doi:10.1109/OCEANS.2001.968289
- Law C, Woodward EMS, Ellwood MJ, Marriner A, Bury SJ, Safi KA 2011. Response of surface nutrient inventories and nitrogen fixation to a tropical cyclone in the southwest Pacific. Limnology and Oceanography 56: 1372–1385. 10.4319/lo.2011.56.4.1372
- Morris M, Stanton B, Neil HL 2001. Subantarctic oceanography around New Zealand: Preliminary results from an ongoing survey. New Zealand Journal of Marine and Freshwater Research 35: 499–519. 10.1080/00288330.2001.9517018
- Mortensen PB, Buhl-Mortensen L 2004. Distribution of deepwater gorgonian corals in relation to benthic habitat features in the Northeast Channel (Atlantic Canada). Marine Biology 144: 1223–1238. 10.1007/s00227-003-1280-8
- Mortensen PB, Hovland MT, Fosså JH, Furevik DM 2001. Distribution, abundance and size of Lophelia pertusa coral reefs in mid-Norway in relation to seabed characteristics. Journal of the Marine Biological Association of the UK 81: 581–597. 10.1017/S002531540100426X
- Mortensen PB, Buhl-Mortensen L, Gebruk AV, Krylovab EM 2008. Occurrence of deep-water corals on the Mid-Atlantic Ridge based on MAR-ECO data. Deep-Sea Research II 55: 142–152. 10.1016/j.dsr2.2007.09.018
- Mortimer N 2004. New Zealand's geological foundations. Gondwana Research 7: 261–272. 10.1016/S1342-937X(05)70324-5
- Muñoz A, Cristobo J, Rios P, Druet M, Polonio V, Uchupi E et al. 2012. Sediment drifts and cold-water coral reefs in the Patagonian upper and middle continental slope. Marine and Petroleum Geology.
- Murphy RJ, Pinkerton MH, Richardson KM, Bradford-Grieve JM, Boyd PW 2001. Phytoplankton distributions around New Zealand derived from SeaWiFS remotely sensed ocean colour data. New Zealand Journal of Marine and Freshwater Research 35: 343–362. 10.1080/00288330.2001.9517005
- Neil HL, Carter L, Morris MY 2004. Thermal isolation of Campbell Plateau, New Zealand, by the Antarctic Circumpolar Current over the past 130 kyr. Paleoceanography 19: PA4008.
- Orsi AH, Witworth III T, Nowlin Jr. WD 1995. On the meridional extent and fronts of the Antarctic Circumpolar Current. Deep-Sea Research I 42: 641–673. 10.1016/0967-0637(95)00021-W
- Reed JK, Koenig CC, Shepard AN 2007. Impacts of bottom trawling on a deep-water Oculina coral ecosystem off Florida. Bulletin of Marine Science 81: 481–496.
- Roark EB, Guilderson TP, Dunbar RB, Fallon SJ, Mucciarone DA 2009. Extreme longevity in proteinaceous deep-sea corals. Proceedings of the National Academy of Sciences 106: 5204–5208. 10.1073/pnas.0810875106
- Roberts JM, Wheeler AJ, Freiwald A 2006. Reefs of the deep: the biology and geology of cold-water coral ecosystems. Science 312: 543–547. 10.1126/science.1119861
- Roberts JM, Wheeler AJ, Freiwald A, Cairns S 2009a. Coldwater corals: the biology and geology of deep-sea coral habitats. Cambridge, Cambridge University Press.
- Roberts JM, Davies AJ, Henr LA, Dodds LA, Duineveld GCA, Lavaleye MSS et al. 2009b. Mingulay reef complex: an interdisciplinary study of cold-water coral habitat, hydrography and biodiversity. Marine Ecology Progress Series 397: 139–151. 10.3354/meps08112
- Smith RO, Vennell R, Bostock HC, Williams MJM 2013. Interaction of the subtropical front with topography around southern New Zealand. Deep Sea Research Part I. Oceanographic Research Papers 76: 13–26. 10.1017/S002531540100426X
- Söffker M, Sloman KA, Hall-Spencer JM 2011. In situ observations of fish associated with coral reefs off Ireland. Deep-Sea Research 58: 818–825. 10.1016/j.dsr.2011.06.002
- Squires DF 1965. Deep-water coral structure on the Campbell Plateau, New Zealand. Deep-Sea Research 12: 785–788.
- Summerhayes CP 1969. Marine geology of the New Zealand subantarctic sea floor. New Zealand Oceanographic Institute Memoir 50. 92 p.
- Sutton PJH 2003. The Southland Current: a subantarctic current. New Zealand Journal of Marine and Freshwater Research 37: 645–652. 10.1080/00288330.2003.9517195
- Thiem Ø, Ravagnan E, Fosså JH, Bersten J 2006. Food supply mechanisms for coldwater corals along a continental shelf edge. Journal of Marine Systems 60: 207–219. 10.1016/j.jmarsys.2005.12.004
- Tracey DM, Rowden AA, Mackay KA, Compton T 2011. Habitat-forming cold-water corals show affinity for seamounts in the New Zealand region. Marine Ecology Progress Series 430: 1–22. 10.3354/meps09164
- Tyrrell T, Merico A 2004. Emiliania huxleyi: bloom observations and the conditions that induce them. In: Thierstein HR, Young JR eds. Coccolithophores: from Molecular processes to global impact. Berlin, Springer-Verlag. Pp. 75–97.
- Van Dover CL 2011. Mining seafloor massive sulphides and biodiversity: what is at risk? ICES Journal of Marine Science 68: 341–348. 10.1093/icesjms/fsq086
- Wheeler AJ, Beyer A, Freiwald A, de Haas H, Huvenne VAI, Kozachenko M et al. 2007. Morphology and environment of cold-water coral carbonate mounds on the NW European margin. International Journal of Earth Sciences 96: 37–56. 10.1007/s00531-006-0130-6
- White HK, Hsing P-Y, Choc W, Shank TM, Cordes EE, Quattrini AM et al. 2012. Impact of the Deepwater Horizon oil spill on a deep-water coral community in the Gulf of Mexico. Proceedings of the National Academy of Sciences. www.pnas.org/cgi/doi/10.1073/pnas.1118029109 (accessed 3 September 2014).
- Wood R, Stagpoole V, Wright I, Davy B, Barnes P 2003. New Zealand's Continental Shelf and UNCLOS Article 76. Institute of Geological & Nuclear Sciences information series 56. NIWA Technical Report 123. Wellington, NIWA. 56 p.
- Woodward EMS, Rees AP 2001. Nutrient distribution in an anticyclonic eddy in the North East Atlantic Ocean, with reference to nanomolar ammonium concentrations. Deep-Sea Research II 48: 775–794. 10.1016/S0967-0645(00)00097-7