Abstract
The effects of two probiotic feeds containing two and three bacterial isolates were evaluated on the growth performance of New Zealand abalone (Haliotis iris). Probiotic bacteria were isolated from the guts of healthy adult abalone. The isolates were screened qualitatively and quantitatively according to their ability to hydrolyse nutrients (i.e. proteins, starch and alginate), produce acid and resist bile salts. Based on the screening results, we developed a multi-strain conglomerate of 2- and 3- probiotic bacterial strains that were supplemented into a commercial abalone feed to use in our experiments. The 2-probiotic conglomerate consisted of Exiguobacterium JHEb1 and Vibrio JH1, and the 3-probiotic conglomerate consisted of Exiguobacterium JHEb1, Vibrio JH1 and Enterococcus JHLDc. The probiotic feeding trial involved abalone juveniles (20–30 mm in maximum shell length). Both probiotic feeds significantly improved abalone growth compared to that of the unsupplemented feed. The 3-probiotic supplemented feed produced a significant shell length increase of 20.9%, a wet weight gain of 19.8% and a five-fold reduction in mortality compared to the controls. The 2-probiotic supplemented feed resulted in significant increases in shell length (15.4%) and reduced mortality (five-fold), but not in weight gain, compared to controls.
Introduction
The black-footed abalone (Haliotis iris) is endemic to New Zealand, and is the only abalone species cultivated for the export market. In addition to the ‘cocktail size’ seafood product, the iridescent shells are polished and sold as decorative accents and jewellery to tourists. The New Zealand abalone industry is entirely land-based, with one major operator, OceaNZ Blue Ltd, as the dominant producer for this growing export industry (Heath & Moss Citation2009). Within the controlled environmental conditions of these abalone farms formulated feeds are used to enhance growth, which currently takes four to five years to attain a market size of 80–100 mm in shell length. Thus, any reduction in the cultivation period would lead to a more profitable commercial operation. Previous studies to optimise this production have focused on better designs of the culture system (Preece & Mladenov Citation1999), application of feed stimulants (Allen et al. Citation2006) and use of different protein sources (Tung & Alfaro Citation2011a) to increase growth and survival rates. Tung & Alfaro (Citation2011a) observed that the shell length and wet weight of juvenile H. iris increased with increasing protein levels in the diet, and that growth was further enhanced with increasing water temperatures. In addition, the source and amount of protein in the diet have been shown to affect the nutritional composition of juvenile H. iris soft tissues (Tung & Alfaro Citation2011b) and shell morphology (i.e. length, width, height, thickness, weight) (Tung & Alfaro Citation2011c).
Another approach to increase growth rates of cultivated species has been the use of probiotics (Macey & Coyne Citation2005, Citation2006; ten Doeschate & Coyne Citation2008; Silva-Aciares et al. Citation2011, Citation2013). The advantage of using these beneficial microorganisms is that they may be targeted to enhance both health and nutrition. Indeed, a variety of benefits have been reported for aquaculture, including competitive exclusion of pathogens, increased utilisation of nutrients, enhanced immune responses, detoxification of potentially harmful compounds, production of vitamins and improvement of water quality (Gatesoupe Citation1999; Rengpipat et al. Citation2000; Verschuere et al. Citation2000; Irianto & Austin Citation2002; Balcázar et al. Citation2006; Vine et al. Citation2006; Kesarcodi-Watson et al. Citation2008; Sahu et al. Citation2008; Mohapatra et al. Citation2012). However, the careful selection of appropriate probiotic organisms is crucial for their successful application. Several criteria need to be met for an organism to be selected as a probiotic agent. For probiotic bacteria, it is ideal if they are isolated from the host animal, not be pathogenic, be able to produce extracellular digestive enzymes, be able to colonise the host, not possess antibiotic-resistance genes, resist low pH values, and remain viable during processing and storage prior to delivery to the host (Irianto & Austin Citation2002; Kesarcodi-Watson et al. Citation2008; Perez-Sanchez et al. Citation2013). While it is relatively easy to test for some of these properties in vitro, the only true test for the effectiveness of any probiotic is by using in vivo feeding trials over sustained time periods (Verschuere et al. Citation2000).
The application of probiotics in abalone aquaculture has been reported for a range of species throughout the world (Cruz et al. Citation2012). Macey & Coyne (Citation2005) isolated one bacterial strain (SY9) and two yeast strains (SS1 and AY1) from the gastrointestinal tract of H. midae, and incorporated the probionts in the feed for cultivated abalone in South Africa. The results showed increased survival and growth rates of the animals when compared with those fed control feeds. Increased protease activity was detected in the digestive tract of the animals fed the probiotic-supplemented feed, and there was an increased resistance to a challenge from a pathogenic bacterium. The probiotic organisms were subsequently identified as Vibrio midae, Cryptococcus sp. and Debaromyces hansenii, and were re-isolated from the gut of the experimental H. midae, suggesting that some colonisation had occurred (Macey & Coyne Citation2006). Subsequently, ten Doeschate & Coyne (Citation2008) demonstrated that H. midae exhibited an increased growth rate when fed a kelp diet supplemented with Pseudoalteromonas sp., and they recorded a significantly higher alginate lyase activity (presumably assisting the digestion of the kelp) in the digestive tract of those animals fed the supplemented diet. In addition, Iehata et al. (Citation2009) isolated lactic acid bacteria from a range of sources and, based on in vitro tests for inhibition of pathogens and resistance to bile acids and gastric juice, selected a Lactobacillus sp. and an Enterococcus sp. to supplement the diet of the Japanese abalone (H. gigantea). The results indicated that the bacteria colonised the guts of the abalone receiving the supplemented diet and were associated with elevated digestive enzyme activity and production of some short chain fatty acids. Similar results were later reported using Pediococcus sp. Ab1 as the probiotic organism for H. gigantea (Iehata et al. Citation2010). Recently, Silva-Aciares et al. (Citation2011) reported that, when H. rufescens was fed an algal diet supplemented with a mixture of three bacteria (two Vibrio spp. and Agarivorans albus), there was a significant increase in growth and survival of abalone fed probiotic diets compared to control animals fed unsupplemented diets.
Based on the reported successful application of probiotics in various abalone species throughout the world, it was envisaged that this approach would be beneficial for the cultivation of the New Zealand black-footed abalone (H. iris). Thus, the aim of this research was to isolate bacteria from healthy and fast growing H. iris, and to test bacterial candidates for probiotic activity in vivo to develop a probiotic-supplemented feed that would increase growth and decrease mortality for juveniles of this abalone species in an aquaculture setting.
Materials and methods
The strategy taken was to isolate bacteria from fast-growing adult abalone, and to select potential probiotic candidates based on their ability to hydrolyse protein, starch and alginate, which are major components of a commercial seaweed-based feed. Initial screening also included production of acid, and resistance to bile acids and low pH values. Finally, selected probiotic candidates were tested in juvenile abalone feeding trials.
Isolation of potential probiotic bacteria
Microorganisms were isolated from the gastrointestinal tract of adult abalone obtained from OceaNZ Blue Ltd, Ruakaka, New Zealand. Samples were taken directly from the stomach, intestine, digestive gland, hepatopancreas, rectum and anus, using sterile cotton swabs. All samples were diluted in Marine Broth and plated on Marine Agar, MacConkey Agar, MRS Agar, TCBS Agar, and Plate Count Agar. All media were obtained from Difco and were supplemented with NaCl (20 g L−1), as needed. Plates were incubated both aerobically and anaerobically at 16 °C and 22 °C. Selected isolates were stored at −80 °C in Marine Broth supplemented with 15% glycerol (Vishnivetskaya & Kathariou Citation2009).
Screening the isolates for hydrolysis of nutrients
Isolates were cultured on plates containing: 2 g L−1 sodium caseinate; 0.2 g L−1 MgSO4; 1.0 g L−1 glucose; 0.01 g L−1 FeSO4; 0.2 g L−1 K2HPO; and 15.0 g L−1 agar. Cultures were incubated at 22 °C until colonies were observed. Protein hydrolysis was indicated by the presence of a clear halo surrounding the colonies, the diameters of which were taken as a measure of proteolytic activity.
For starch hydrolysis, the isolates were cultured on Nutrient Agar (Difco) plates supplemented with starch (10 g L−1) and NaCl (20 g L−1) at 22 °C. After colonies were visible, the plates were flooded with Gram iodine solution. Starch hydrolysis was indicated by disappearance of the blue colour surrounding the colonies.
For alginate hydrolysis, the isolates were cultured on plates containing: 20 g L −1 sodium alginate (Sigma); 5 g L−1 yeast extract (Difco); 10 g L−1 agar (Difco); 0.2 g L−1 MgSO4; 1 g L−1 NH4H2PO4; 1 g L−1 K2HPO4; 20 g L−1 NaCl; 0.03 g L−1 bromothymolblue blue (modified from Nakamura Citation1987) at 22 °C. After incubation (2 to 5 days), alginate hydrolysis was detected by a yellow zone surrounding the colonies. Confirmation of hydrolysis was performed by the addition of ethanol (70%) for 1 h. Unhydrolysed alginate was observed as a precipitate.
Potential probiotic bacteria also were screened for alginate hydrolysis in a broth containing: 1 g L−1 sodium alginate; 5 g L−1 peptone; 1 g L−1 yeast extract; 20 g L−1 NaCl (Kitamikado et al. Citation1990). Alginate hydrolysis was determined every 24 h of incubation using a precipitation test with acidic albumin (Kitamikado et al. Citation1990). In the presence of alginate the albumin was precipitated, and this was quantified by measuring the transmission of the resulting suspension at 600 nm in a spectrophotometer.
Screening of isolates for acid production was determined by growing the isolates in Marine Broth containing glucose (50 g L−1). After incubation, cells were removed by centrifugation and the acid concentration in the supernatant was estimated by titration with 0.1 M NaOH using phenolphthalein as an indicator, and expressed as lactic acid (g L−1) (ADMI Citation1971).
For acid tolerance, isolates were assessed in Marine Broth for survival at pH values as low as pH 1.5, although the pH value in the normal gut rarely drops below pH 5.5 (experimental observation during this study). The broths were inoculated from a colony and incubated at 22 °C for 3 h. Survival was determined by comparing the viable plate count after 3 h with that at 0 h (incubated at pH 6.5) (Iehata et al. Citation2009).
Identification of isolates
Isolates were characterised using standard phenotypical and biochemical methodology to allow preliminary identification to the genus level (Bergey et al. Citation1994). Subsequently, selected isolates were subjected to 16S rRNA analysis at LandCare Research Ltd (Auckland, New Zealand). The primers used to amplify the 16S rRNA gene were 27F (forward) and 1525R (reverse) (Lane Citation1991).
Enzyme assays
Isolates were grown in Marine Broth supplemented with 10 g L−1 of starch, casein or alginate for subsequent assays of amylolytic, proteolytic or alginolytic activity, respectively. Cultures were incubated at 22 °C with gentle shaking for 48 h (for casein and starch) or 120 h (for alginate). After incubation, cells were removed by centrifugation and the supernatants were filtered through a 0.22 µm filter prior to being assayed for enzyme activity.
Proteolytic activity was assayed using a substrate of azocasein (Bisswanger Citation2004). One unit of activity is defined as the amount of enzyme that gave an increase in optical density at 340 nm of 0.1 in 30 min at 25 °C.
Amylolytic activity was assayed using a substrate of starch (Wang et al. Citation2008). The liberation of reducing sugar was measured using dinitrosalicylic acid with a standard of glucose. One unit of activity is defined as the amount of enzyme that released 1 µmol of reducing sugar per minute.
Alginolytic activity was assayed using a substrate of sodium alginate (Hu et al. Citation2006). The liberation of reducing sugar was measured using dinitrosalicylic acid with a standard of glucose. One unit of activity is defined as the amount of enzyme that released 1µmol of reducing sugar per minute.
Preparation of feed
The commercial feed used in the feeding trials was AbMax 16, which is manufactured by E.N. Hutchinson Ltd, Auckland, New Zealand. The ingredients in this feed included wheat flour, fish meal, seaweed powder, defatted soy flour, and a range of vitamins and minerals. The unaltered commercial feed was used as the control. The two treatments included the addition of different probiotic complexes added to the feed. One of the treatments contained a 2-probiotic consortium (2-P), including Exiguobacterium sp. strain JHEb1 and Vibrio sp. strain JH1, while the second contained a 3-probiotic consortium (3-P) of the two strains in the previous treatment and Enterococcus sp. strain JHLDc. These bacteria were isolated from adult abalone, as described above. Prior to the addition to the feed, the bacteria were cultured in Marine Broth, concentrated by centrifugation, and resuspended as a mixture in Marine Broth. The Exiguobacterium strain was added at half the concentration of the other bacteria as it was a faster growing bacterium. For inoculation, feed pellets were spread on plastic trays and sprayed with the probiotic mixture, after which the pellets were air dried. The average total viable bacterial counts in the pellets ranged from 2 × 108 CFU/g for the 2-P conglomerate to 3 × 109 CFU/g for the 3-P conglomerate. No counts were performed on the pellets after they were placed in the tanks.
Feeding trials
Animals
Juvenile abalone (20–30 mm shell length) were obtained from OceaNZ Blue Ltd, Ruakaka, New Zealand. Each animal was tagged with a unique number for later identification. All tagged animals were placed in a tank in the laboratory, and acclimatised for one week prior to the experiment. During this time, the animals were fed the unsupplemented commercial feed. Based on pilot data, a ration equivalent to 1.1% of biomass per day was used and considered sufficient for ad libitum feeding.
Growth trials
The probiotic feeding experiment was conducted over a 60-day period from October to December 2011 at the Aquaculture Laboratory, Auckland University of Technology. Three 42 L black plastic tanks were used for each of the two treatments and control for a total of nine tanks. Each tank was covered with mesh to prevent the escape of abalone. In addition, a plastic plate was placed on top to inhibit algal growth and to provide darkness in the tank for maximum feeding by abalone. Twenty animals were placed inside each tank. Once in the tanks, the animals were starved for 3 days prior to the start of the trial to empty their guts. Feeding was performed once per day at a rate of about 1.1% body weight. Prior to daily feeding, the tanks were cleaned to remove uneaten feed and faeces. The tanks were aerated using two air stones to achieve dissolved oxygen levels close to 100%, and the water was checked regularly for salinity (32–34 ppt), pH (7.9–8.3), NH3 (0.5 mg L−1) and nitrite (5 mg L−1). The temperature was maintained at 16.0–16.9 °C for the duration of the experiment. Counts of viable bacteria for each water tank were performed weekly using Plate Count Agar to monitor total (probiotic plus non-probiotic) bacterial concentrations. At the beginning and end of the trials, the animals were measured for their wet weights and shell lengths. For the weight, individual animals were blotted dry using tissue and then weighed to the nearest 0.01 g. Shell length measurements were taken on the longest axis using digital vernier calipers to the nearest 0.01 mm.
The seawater used for this experiment was obtained from Okahu Bay, Auckland, and was filtered through a 0.2 µm filter prior to use within one week. For the entire experimental period, a 50% seawater exchange was undertaken daily for two days, followed by a 100% exchange on the third day. When the entire water volume was exchanged (every 3 days), the tank walls and the bottom were cleaned using tissue paper to remove biofilms. Any dead animals were removed from the tanks during the trial and replaced with new ones. These new animals were not included in any analyses, but were kept in the tanks simply to maintain a constant density. During the last 10 days of the trial, faecal samples were collected for analysis of protein, lipid, carbohydrate and ash. At the end of the trial, the individually tagged animals were once again weighed and measured for their maximum lengths. Mortality counts were recorded throughout the experiment for the three different feeding regimes.
Biochemical analysis of commercial feed and faeces
To determine feed digestibility, dried samples (0.5–1 g) of faeces from each tank and samples of commercial feed were analysed biochemically. Crude protein was estimated using the Kjeldahl method for total nitrogen (AOAC 2005). Crude lipid contents were determined using the Bligh & Dyer (Citation1959) method and ash was determined based on the AOAC (Citation1970) methods. The samples were dried in the oven at 100 °C for 3 h to determine the moisture content (De Knegt & van den Brink Citation1998), and heated in a furnace at 520 °C for approximately 2 h for ash content. The carbohydrate content was determined by difference (Porter & Earl Citation1990).
Statistical analyses
The collected data for biochemical analyses, growth (animal weight and shell length) and mortality were analysed using an SPSS statistics program (IBM SPSS version 20). One-way analysis of variance (ANOVA) and Tukey post hoc tests were used to determine significant differences between the sample means at P < 0.05.
Results
Identification and selection of bacterial isolates
Colonies were first isolated from original samples after plating out on the standard media listed in ‘Materials and methods’. After isolation of representative dominant colonies, cultures were tested for hydrolytic activity against protein, starch and alginate on solid media. Cultures showing high levels of hydrolytic activity were subsequently tested for their enzyme activity, as described in ‘Materials and methods’, followed by testing for acid production and tolerance to low pH values. Based on these biochemical tests, three potential probiotic isolates were identified.
Isolate JHEb1 showed high proteolytic (128 IU ml−1) and amylolytic (358 IU ml−1) activities in the culture supernatant. Isolate JH1 showed high proteolytic (62 IU ml−1), amylolytic (187 IU ml−1) and alginolytic (241 IU ml−1) activities in the culture supernatant. Isolate JHLDc displayed high acid production (6.7 g L−1 expressed as lactic acid) in the culture supernatant. All three isolates were reasonably acid tolerant, showing no reduction in numbers during 3 h incubation at pH 5 and less than 1-log reduction at pH 2. The combination of phenotypic tests and 16S rRNA analysis resulted in the identification of JHEb1as Exiguobacterium sp., JH1 as Vibrio sp. and JHLDc as Enterococcus sp.
Feeding trials
Maximum shell length
Abalone that were fed the 2- and 3-P consortia had significantly (P < 0.05) greater shell length increases (mm per 60 days) of 4.03 ± 0.13 and 3.84 ± 0.08, respectively, compared to those that were fed the unsupplemented feed (3.33 ± 0.14). Hence, 3-P and 2-P resulted in shell length gains of 20.9% and 15.4%, respectively. However, there was no significant difference between shell lengths of abalone that were fed the 2-P and 3-P conglomerates (P > 0.05) ().
Abalone wet weight
Haliotis iris fed with Abfeed supplemented with 3-P exhibited a significant (P < 0.05) increase in wet weight after 60 days of feeding trial compared to those grown on unsupplemented feed (). The 3-P feeding resulted in a wet weight gain (g per 60 days) of 0.43 ± 0.04, representing a statistically significant (P < 0.05) increase of 19.8% compared with the control (0.37 ± 0.02). The 2-P treatment also produced a wet weight gain higher than that of the unsupplemented feed, but this increase of 9.5% was not statistically significant (P > 0.05).
Mortality
During the course of the feeding experiment, a low mortality was observed in abalone fed with the 2-P and 3-P consortia (3.33% ± 0.33 and 3.33% ± 0.66, respectively) (). These mortality results were significantly lower (P < 0.05) than those observed with the unsupplemented feed (16.67% ± 3.33).
Faecal analysis
Faecal samples taken during the last 10 days of the feeding trial indicate that there was a significant (P < 0.05) decrease in crude protein, crude lipid and crude carbohydrate from the two probiotic groups (2-P and 3-P) compared to those from the control group (). However, there was no statistical difference between the faecal components in the 2-P and 3-P conglomerates.
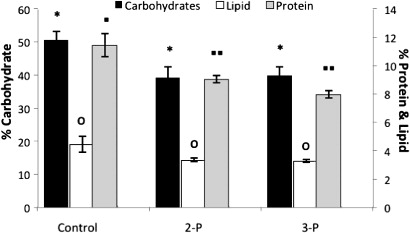
Viable bacteria counts
The average bacterial counts obtained from the rearing water during the feeding trials were 3 × 104 CFU ml−1 in the tanks fed with 2-P supplemented feed and 4 × 105 CFU ml−1 in the tanks fed the 3-P supplemented feed. The control tanks contained 2 × 102 CFU ml−1.
Discussion
The 60-day feeding trial resulted in a significant increase in growth of abalone when using the supplementation of feed with selected live probiotic bacteria. The highest growth improvements in terms of weight and shell length (19.8% and 20.9%, respectively) were obtained when feeding with a 3-P feed. The 3-P conglomerate consisted of three putative probiotic bacteria Exiguobacterium JHEb1, Vibrio JH1 and Enterococcus JHLDc. A lower, but significant, increase in shell length was observed in juvenile abalone fed with a 2-P supplemented feed, which contained Exiguobacterium JHEb1 and Vibrio JH1. There was no significant weight gain obtained with the 2-P supplemented feed, suggesting that the more metabolically diverse the probiotic bacteria used, the better the growth performance of the abalone. Although this study was performed over a relatively short time period (60 days, compared to the 4–5 years for the animals to grow 8 cm), due to resource constraints, the results clearly demonstrate proof of concept. A similar feeding trial using a different abalone species, H. midae, was carried out for a longer period of 163 days (ten Doeschate & Coyne Citation2008). In that study, a single probiont was used, a Pseudoalteromonas strain C4 that was alginolytic and appropriate for the kelp cakes used. After 163 days, the presence of strain C4 resulted in an increased shell length of 38% and weight of 39%. Using 60 days as a basis of comparison, the C4 strain would have produced only a 14% increase in shell length. The results in the present study for the same sized abalone appear to be superior to those obtained with the C4 strain. However, this must be qualified by the fact that the nutritional content of the feed and the growth rates of the two abalone species were probably different.
The growth improvements obtained in the present study are higher than those reported by Macey & Coyne (Citation2005) using H. midae fed with probiotic-supplemented feed containing three different microorganisms. Haliotis midae fed with a combination of a proteolytic bacteria and two amylolytic yeasts exhibited a lower improvement in shell length and weight (7% and 8%, respectively). However, the experimental conditions used in that study were different from those in the present work.
Iehata et al. (Citation2009) isolated lactic acid bacteria from a range of species and, based on in vitro tests for inhibition of pathogens and resistance to bile acid and gastric juice, selected a Lactobacillus sp. and an Enterococcus sp. to supplement the diet of the Japanese abalone (H. gigantea). Increased survival and growth rates were observed when compared with animals fed control diets. Similar results were reported using Pediococcus sp. Ab1 as the probiotic organism for H. gigantea (Iehata et al. Citation2010), and when using a mixture of three bacteria (two Vibrio sp. and Agarivorans albus) for H. rufescens (Silva-Aciares et al. Citation2011).
The 2-P conglomerate of Vibrio sp. and Exiguobacterium sp. was used in the present work to ensure delivery of high levels of three hydrolytic enzyme activities. Enterococcus sp. was included in the 3-P conglomerate to determine if the ability to produce high levels of acid showed any additive effects on the abalone growth. Interestingly, a significant increase in animal weight was achieved only when Enterococcus sp. was present. Enterococcus sp. was selected on the basis of its ability to produce acid, as it has been suggested that production of volatile short chain fatty acids, including lactic acid, may be beneficial to abalone growth (El-Shanshoury et al. Citation1994; Joborn et al. Citation1997; Iehata et al. Citation2010). The results of the present study support the idea that acid production is a key criterion in the selection of useful probiotic bacteria.
One mechanism by which these positive results were achieved is presumably related to the hydrolytic activities of the probiotics (Erasmus et al. Citation1997). In the present work, the Vibrio and the Exiguobacterium species were selected for use on the basis of their high hydrolytic activities. The bacteria presumably make available more assimilable nutrients within the gastrointestinal tract, and also hydrolyse the polymers externally to the host followed by ingestion of sugars and amino acids rather than starch, protein and alginate, as proposed by Olmos et al. (Citation2011).
The results of the feeding trials show that the addition of the 2-P and 3-P conglomerates to the commercial feed resulted in a significantly reduced mortality and a significant increase in shell length when compared to the unsupplemented feed treatment. The improvement in survival (lower mortality) observed with the probiotic treatments may be explained by the effect of the bacteria in enhancing the immune system of the abalone. Low mortality can be related to immunomodulation as a possible mechanism of probiotic activity, as probiotics have been shown to be capable of improving immunity in abalone (Macey & Coyne Citation2005; ten Doeschate & Coyne Citation2008). However, no assessment of immunostimulation was performed during the current study,
It should also be noted that the higher mortality observed in the control tanks in the current study may be related to the presence of an additional variable (i.e. as dead animals were removed from the tanks new ones were introduced). Hence some other unknown factor may have been introduced into the tanks. At this stage, this possibility cannot be eliminated but the magnitude of the observed differences in mortality is considered unlikely to have been caused by this potential variable. Vibrio species are known to be important bacterial pathogens for animals reared in aquaculture, including abalone (Cai et al. Citation2006), but they are also known to be members of the bacterial flora of healthy abalone (Iehata et al. Citation2010). Further, there are examples of probiotic Vibrio species being effective in reducing diseases caused by pathogenic Vibrio spp. (Austin et al. Citation1995). Macey & Coyne (Citation2006) isolated V. midae from the gastrointestinal tract of the abalone H. midae, and used this species as a probiotic agent in combination with two yeasts. Recently, Silva-Aciares et al. (Citation2011) used two Vibrio species in a 3-probiotic conglomerate to supplement an algal diet for the abalone H. rufescens, and showed significant improvement in growth and survival. However, as some Vibrio species are known pathogens, caution should be exercised as the possibility of pathogenic gene transfer to non-pathogens is a potential risk.
There are few reports of Exiguobacterium sp. being used in aquaculture. A recent study reported this bacterium to have pathogenic effects in abalone (H. rufescens) postlarvae (Anguiano-Beltrán et al. Citation2012). This result contrasts markedly to the results in the present work in which the presence of Exiguobacterium sp. resulted in probiotic benefits, at least in combination with other bacteria.
In the present study, neither the Vibrio sp. nor the Exiguobacterium sp. were used alone to supplement the feed of the abalone, so it is impossible to assess the individual contribution of each to the observed results. This will be the subject of future work.
Statistical analyses of the faecal protein composition showed that both of the probiotic feeds were significantly more digestible than the unsupplemented feed. These results support the concept that the use of probiotic-supplemented feed can assist abalone to derive more benefits from the feed. The positive and congruent results obtained herein, regarding improved digestibility of nutrients and reduced levels of protein in the faecal material, are encouraging and reflect an improvement from those of Macey & Coyne (Citation2005) who observed enhanced digestion and absorption of protein, but no noticeable difference in faecal protein content after using their probiotics.
The no significant difference in lipid content observed in animals fed the 2-P and 3-P diets may be explained by the lack of lipolytic isolates in the probiotic feeds. Only Exiguobacterium JHEb1 had lipid-degrading ability according to its phenotypic properties. However, it should be noted that lipid degradation was not included in the main criteria of probiotic selection.
Both probiotic diets included two highly amylolytic bacteria (Exiguobacterium JHEb1 and Vibrio JH1). However, the amounts of carbohydrates remaining in the faeces were not significantly different between the treatment and control groups. The explanation for this is not certain, but it could be due to enzyme regulation, such as catabolite repression or substrate inhibition that occurs in each isolate.
The viable bacterial counts in the water were, as expected, higher when the feed was supplemented with the probiotics. However, these counts include all bacteria in addition to the added probiotics. Ideally, probiotic bacteria should colonise the host and avoid the need for regular supply in the feed. However, as shown by Macey & Coyne (Citation2006), even if some colonisation takes place, the maximum benefit is achieved only when there is a regular supply of probiotics in the feed. Indeed, Macey & Coyne (Citation2006) observed a significant decrease in viable counts of probiotics once the animals were switched to an unsupplemented feed.
For future work, in vitro antimicrobial effects of the selected probiotics against potential pathogens will be determined. However, it is clear from the present results that the bacteria themselves are not pathogens, at least under the conditions used in the present study. Several studies have demonstrated the inhibitory role of beneficial microorganisms against pathogens in aquaculture (Verschuere et al. Citation2000; Vine et al. Citation2006), and this topic will need further investigation for H. iris.
Based on the about 20% increase in growth of abalone fed the probiotic complex developed herein, it appears that the formulation approach and the probiotic consortia identified may be a significant improvement on the commercial cultivation of H. iris in New Zealand. In addition to the increased growth and reduced mortality associated with the identified probiotic complexes, it is likely that this approach also may reduce the use of costly chemicals and/or antibiotics to control pathogens within aquaculture settings.
Acknowledgements
The authors wish to thank Professor John Brooks, Dr. Colleen Higgins, and others in the Aquaculture Biotechnology Group at Auckland University of Technology (AUT) for their involvement in many discussions on this work. Special thanks to Mark Duxbury for his help with enzyme activity, and to the laboratory technicians in the School of Applied Sciences, AUT for their valuable assistance. This work would not have been possible without the animals kindly provided by OceaNZ Blue Ltd, Ruakaka, New Zealand.
References
- ADMI 1971. Standards for grades of dry milks including method of analysis. Bulletin 916 (Revised). Chicago, IL, American Dry Milk Institute, Inc.
- Allen VJ, Marsden ID, Ragg NLC, Gieseg S 2006. The effects of tactile stimulants on feeding, growth, behaviour, and meat quality of cultured blackfoot abalone, Haliotis iris. Aquaculture 257: 294–308. 10.1016/j.aquaculture.2006.02.070
- Anguiano-Beltrán C, Searcy-Bernal R, García-Ortega AM, García-Esquivel Z, Valenzuela-Espinoza, E 2012. Effect of three bacterial isolates from a commercial hatchery on early red abalone (Haliotis rufescens) postlarvae. Aquaculture International 20: 993–1001. 10.1007/s10499-012-9523-7
- AOAC 1970. Official methods of analysis of the Association of Official Analytical Chemists, Washington, DC, AOAC International.
- Austin B, Alsina M, Austin DA 1995. Identification and typing of Vibrio anguillarum: a comparison of different methods. Systematic Applied Microbiology 18: 285–302. 10.1016/S0723-2020(11)80400-5
- Balcázar JL, Blas ID, Ruiz-Zarzuela I, Cunningham D, Vendrell D, Múzquiz JL 2006. The role of probiotics in aquaculture. Veterinary Microbiology 114: 173–186. 10.1016/j.vetmic.2006.01.009
- Bergey DH, Holt JG, Krieg NR, Sneath PHA 1994. Bergey's manual of determinative bacteriology. 9th edition. Baltimore, MD, Lippincott Williams & Wilkins.
- Bisswanger H 2004. Practical enzymology. Tubingen, Germany, Wiley-VCH Verlag GmbH & Co. KGaA, Weinheim.
- Bligh EG, Dyer WJ 1959. A rapid method of total lipid extraction and purification. Canadian Journal of Biochemistry and Physiology 37: 911–917. 10.1111/j.1365-2109.2006.01552.x
- Cai HH, Song Z, Zhou CLJ 2006. Isolation and characterization of pathogenic Vibrio alginolyticus from diseased postlarval abalone, Haliotis diversicolor supertexta (Lischke). Aquaculture Research 37: 1222–1226. 10.1111/j.1365-2109.2006.01552.x
- Cruz PM, Ibanez AL, Monroy Hermosillo OA, Ramirez Saad, HC 2012. Use of probiotics in aquaculture. International Scholarly Research Network ISRN Microbiology 2012: 916845.
- De Knegt RJ, van den Brink H 1998. Improvement of the drying oven method for the determination of the moisture content of milk powder. International Dairy Journal 8: 733–738. 10.1016/S0958-6946(97)00110-6
- El-Shanshoury AR, Mona MH, Shoukr FA, E1-Bossery AM 1994. The enumeration and characterization of bacteria and fungi associated with marine wood-boring isopods, and the ability of these microorganisms to digest cellulose and wood. Marine Biology 119: 321–326. 10.1007/BF00347528
- Erasmus JH, Cook PA, Coyne VE 1997. The role of bacteria in the digestion of seaweed by the abalone Haliotis midae. Aquaculture 155: 377–386. 10.1016/S0044-8486(97)00112-9
- Gatesoupe FJ 1999. The use of probiotics in aquaculture. Aquaculture 180: 147–165. 10.1016/S0044-8486(99)00187-8
- Heath PL, Moss GA 2009. Is size grading important for farming the abalone Haliotis iris? Aquaculture 290: 80–86. 10.1016/j.aquaculture.2009.01.038
- Hu X, Jiang X, Hwang HM 2006. Purification and characterization of an alginate lyase from marine bacterium Vibrio sp. mutant strain 510-64. Current Microbiology 53: 135–140. 10.1007/s00284-005-0347-9
- Iehata S, Inagaki T, Okunishi S, Nakano M, Tanaka R, Maeda H 2009. Colonization and probiotic effects of lactic acid bacteria in the gut of the abalone Haliotis gigantea. Fisheries Science 75: 1285–1293. 10.1007/s12562-009-0138-5
- Iehata S, Inagaki T, Okunishi S, Nakano M, Tanaka R, Maeda H 2010. Improved gut environment of abalone Haliotis gigantea through Pediococcus sp. Ab1 treatment. Aquaculture 305: 59–65. 10.1016/j.aquaculture.2010.04.012
- Irianto A, Austin B 2002. Probiotics in aquaculture. Journal of Fish Diseases 25: 633–642. 10.1046/j.1365-2761.2002.00422.x
- Joborn A, Olsson C, Westerdahl A, Conway P, Kjelleberg S 1997. Colonisation in the fish intestinal tract and production of inhibitory substances in intestinal mucus and faecal extracts by Curnohuctrrium sp. strain K 1. Journal of Fish Diseases 20: 383–392. 10.1046/j.1365-2761.1997.00316.x
- Kesarcodi-Watson A, Kaspar H, Lategan M, Gibson L 2008. Probiotics in aquaculture: the need, principles and mechanisms of action and screening processes. Aquaculture 274: 1–14. 10.1016/j.aquaculture.2007.11.019
- Kitamikado M, Yamaguchi K, Tseng C-H, Okabe BI 1990. Method designed to detect alginate-degrading bacteria. Applied Environmenatl Microbiology 56: 2939–2940.
- Lane DJ 1991. 16S/23S rRNA sequencing. In: Stackebrandt E, Goodfellow M eds. Nucleic acid techniques in bacterial systematics. Chichester, UK, Wiley. Pp. 115–175.
- Macey BM, Coyne VE 2005. Improved growth rate and disease resistance in farmed Haliotis midae through probiotic treatment. Aquaculture 245: 249–261. 10.1016/j.aquaculture.2004.11.031
- Macey BM, Coyne VE 2006. Colonization of the gastrointestinal tract of the farmed South African abalone Haliotis midae by the probionts Vibrio midae SY9, Cryptococcus sp. SS1, and Debaryomyces hansenii AY1. Marine Biotechnology 8: 246–259. 10.1007/s10126-005-0113-9
- Mohapatra S, Chakraborty T, Prusty AK, Das P, Paniprasad K, Mohanta KN 2012. Use of different microbial probiotics in the diet of rohu, Labeo rohita fingerlings: effects on growth, nutrient digestibility and retention, digestive enzyme activities and intestinal microflora. Aquaculture Nutrition 18: 1–11. 10.1111/j.1365-2095.2011.00866.x
- Nakamura LK 1987. Bacillus alginolyticus sp. nov. and Bacillus chondroitinus sp. nov., two alginate-degrading species. International Journal of Systematic Bacteriology 37: 284–286. 10.1099/00207713-37-3-284
- Olmos J, Ochoa L, Paniagua-Michel J, Contreras R. 2011. Functional feed assessment on Litopenaeus vannamei using 100% fish meal replacement by soybean meal, high levels of complex carbohydrates and Bacillus probiotic strains Marine Drugs 9: 1119–1132. 10.3390/md9061119
- Perez-Sanchez T, Ruiz-Zarzuela I, de Blas I, Balcaraz JL 2013. Probiotics in aquaculture: a current assessment. Reviews in Aquaculture 5: 1–14. 10.1111/j.1753-5131.2012.01073.x
- Porter D, Earl O 1990. Nutrition labeling issues and directions for the 1990s. Washington, DC, National Academy Press.
- Preece MA, Mladenov PV 1999. Growth and mortality of the New Zealand abalone Haliotis iris Martyn 1784 cultured in offshore structures and fed artificial diets. Aquaculture Research 30: 865–877. 10.1046/j.1365-2109.1999.00415.x
- Rengpipat S, Rukpratanporn S, Piyatiratitivorakul S, Menasaveta P 2000. Immunity enhancement in black tiger shrimp (Penaeus monodon) by a probiont bacterium (Bacillus S11). Aquaculture 191: 271–288. 10.1016/S0044-8486(00)00440-3
- Sahu MK, Swarnakumar NS, Sivakumar K, Thangaradjou T, Kannan L 2008. Probiotics in aquaculture: importance and future perspectives. Indian Journal of Microbiology 48: 299–308. 10.1007/s12088-008-0024-3
- Silva-Aciares FR, Carvajal PO, Mejías CA, Riquelme CE 2011. Use of macroalgae supplemented with probiotics in the Haliotis rufescens (Swainson, 1822) culture in northern Chile. Aquaculture Research 42: 953–961. 10.1111/j.1365-2109.2010.02678.x
- Silva-Aciares FR, Moraga D, Auffret M, Tanguy A, Riquelme C. 2013. Transcriptomic and cellular response to bacterial challenge (pathogenic Vibrio parahaemolyticus) in farmed juvenile Haliotis rufescens fed with or without probiotic diet. Journal of Invertebrate Pathology 113: 163–176. 10.1016/j.jip.2013.03.004
- ten Doeschate KI, Coyne VE 2008. Improved growth rate in farmed Haliotis midae through probiotic treatment. Aquaculture 284: 174–179. 10.1016/j.aquaculture.2008.07.018
- Tung C-H, Alfaro AC 2011a. Effect of dietary protein and temperature on the growth and health of juvenile New Zealand black-footed abalone (Haliotis iris). Aquaculture Research 42: 366–385. 10.1111/j.1365-2109.2010.02631.x
- Tung C-H, Alfaro AC 2011b. Alternative protein sources in artificial diets for New Zealand's black-footed abalone, Haliotis iris, Martyn 1784, juveniles. Journal of the World Aquaculture Society 43: 1–29. 10.1111/j.1749-7345.2011.00545.x
- Tung C-H, Alfaro AC 2011c. Effect of dietary protein source and amount on shell morphology of juvenile abalone Haliotis iris. Journal of FIsheries and Aquatic Science 6: 107–118. 10.3923/jfas.2011.107.118
- Verschuere L, Rombaut G, Sorgeloos P, Verstraete W 2000. Probiotic bacteria as biological control agents in aquaculture. Microbiology and Molecular Biology Reviews 64: 655. 10.1128/MMBR.64.4.655-671.2000
- Vine NG, Leukes WD, Kaiser H 2006. Probiotics inmarine larviculture. FEM Microbiology Reviews 30: 404–427. 10.1111/j.1574-6976.2006.00017.x
- Vishnivetskaya TA, Kathariou S 2009. The Exiguobacterium genus: biodiversity and biogeography. Extremophiles 13: 541–555. 10.1007/s00792-009-0243-5
- Wang Y-B, Li J-R, Lin J 2008. Probiotics in aquaculture: challenges and outlook. Aquaculture 281: 1–4. 10.1016/j.aquaculture.2008.06.002