Abstract
The upstream migrations of juvenile galaxiids, or ‘whitebait’, form the basis of an important New Zealand fishery. This study used mark and recapture experiments with juvenile galaxiids in the Mokau River to examine the effect of flow on migration and capture rates. Recapture rates of marked fish ranged between 2.7% and 26.9%, with the proportion of fish recaptured positively correlated with increasing river flow. Increasing river flow negatively affected the upstream migration rates of fish. Migration rates through the tidal zone could be rapid, with fish travelling upstream at speeds up to 1.4 km h−1 within 24 h of release. However, not all marked fish exhibited rapid upstream movement. Fish captured on the second day following release had travelled at slower speeds up to 0.7 km h−1, suggesting that these fish did not initiate their migration on the tidal cycle subsequent to their liberation. A small proportion of fish were also found to travel up to 15 km downstream following release at the upper end of the tidal zone. Results indicated that some fish migrated on both diurnal and nocturnal tidal cycles. Nocturnal migrations by juvenile galaxiids could reduce capture rates and increase recruitment, especially in smaller rivers and streams where fish can pass the tidal fishing area on a single overnight tidal cycle. These results have management implications for river systems where juvenile galaxiids are exposed to multiple days fishing pressure, as reducing fishable zones could be one means of reducing pressure on unstable or declining galaxiid populations.
Introduction
New Zealand contains five diadromous galaxiid species (inanga, Galaxias maculatus, kōaro, G. brevipinnis, banded kōkopu, G. fasciatus, giant kōkopu, G. argenteus and shortjaw kōkopu, G. postvectis), whose juveniles are collectively termed ‘whitebait’, and form the basis of important commercial, recreational and customary fisheries. Inanga spawn in the tidal reaches of rivers, with larvae migrating to the sea upon hatching. Kōaro and kōkopu species spawn in freshwater streams, with larvae washed out to sea during spates. After several months, juveniles return to freshwater as ‘whitebait’, and migrate upriver to adult habitats. Nationwide, mixed species' shoals are harvested as they enter river mouths.
The harvesting of juvenile galaxiids is widespread across the Southern Hemisphere, with fisheries also found in Australia (Allen et al. Citation2002) and Chile (Hugo Citation1973). These whitebait fisheries are one of the few fisheries worldwide based on capturing post-larval juveniles (Hickford & Schiel Citation2010).
Within New Zealand, inanga are the primary species in the whitebait catch, comprising some 95% of catches (McDowall Citation1984; Rowe et al. Citation1992). Inanga are essentially an annual species, with adults reaching sexual maturity in their first year, and the majority of fish dying after spawning (McDowall Citation1990). As such, annual recruitment of inanga is a critical bottleneck for successfully maintaining adult breeding stocks and ensuring the whitebait fishery remains sustainable.
Over the last century, there has been an extensive decline in whitebait abundance in New Zealand,owing largely to deforestation of land, draining of swamps, diversion and pollution of rivers (McDowall Citation1990; Rowe et al. Citation2000), and from the introduction of exotic species (McDowall Citation2006; McIntosh et al. Citation2010). The relative impact of these factors has not been quantified, but McDowall (Citation1990) attributed the majority of the decline to habitat loss. In addition, Swales (Citation1991) suggested that overfishing of whitebait is a major factor in the decline of the fishery. While river restoration is a recent initiative in maintaining and enhancing the New Zealand whitebait fishery (Baker & Smith Citation2007; Jowett et al. Citation2009; Hickford & Schiel Citation2010; Franklin & Bartels Citation2012), ensuring juvenile recruitment to available habitats is undoubtedly a key factor in managing the whitebait fishery.
During their upstream migration, fishing pressure on juvenile galaxiids is largely confined to the estuarine and lower reaches of the river that are influenced by the tide. Therefore, the migration rate and retention time of juveniles within the tidal zone will affect the length of time fish are exposed to fishing pressure, and therefore the probability of capture. However, there is little information on the movement patterns of juvenile galaxiids upon river entry, and the time taken for fish to pass through the tidal zone. Such knowledge is critical in predicting escapement of whitebait, and hence levels of recruitment for these species.
Whilst in the tidal zone, environmental variables such as tidal height, river clarity and flow rate, along with fishing effort and the efficiency of the whitebait trap/net determines the proportion of the whitebait run that will be captured, and hence the proportion of juvenile galaxiids available to recruit into the adult populations (McDowall & Eldon Citation1980; Allibone et al. Citation1999). Previous studies correlating environmental factors, such as tide height, river flow and rainfall to the size of fishermen's catches suggest that larger catches occur on higher tides and immediately after floods, when the river flow is elevated and the water is more turbid (McDowall & Eldon Citation1980; Stancliff et al. Citation1988a). These correlations were supported by Allibone et al. (Citation1999) who found that higher flows forced whitebait to swim closer to the bank where they were subjected to increased fishing pressure. Presently, Allibone et al. (Citation1999) is the only mark and recapture investigation with whitebait that has been undertaken, and releases focused on the relatively small Awakino River (see ). Therefore, the size of the migration run relative to fishermen's catches, and the effect of environmental factors on the proportion of the whitebait migration that is captured, are still largely unknown. This study used mark and recapture techniques to investigate the effect of different flows on whitebait migration and capture rates, along with an assessment of trap efficiency relative to water velocities, to gain a better understanding of the effects of fishing pressure on juvenile galaxiid recruitment.
Materials and methods
Study stream
The Mokau River (174°37′E, 38°42′S) is representative of a large New Zealand whitebaiting river () with a large tidal zone reaching 22 km upriver. There are 244 registered fishing stands lining the river between the mouth and the end of the tidal zone, with most fishermen setting whitebait traps from these stands. Scoop netting is limited to the mouth of the river. The Mokau River has a mean flow of 36.4 m3 s−1, a median flow of 22.8 m3 s−1, and a mean annual seven day low flow of 5.7 m3 s−1 (NIWA's records from Totoro Bridge, 80 km upstream of the river mouth; ).
Staining procedure
Live whitebait were captured from whitebait stands on the Mokau River during migration runs. Whitebait runs in the Mokau River are dominated by inanga (G. maculatus), which comprise between 98%–99% of the catch (Hanchet & Hayes Citation1989). Banded kōkopu (G. fasciatus) and kōaro (G. brevipinnis) constitute the remainder of the catch, with small numbers of giant kōkopu (G. argenteus) appearing late in the season. No shortjaw kōkopu (G. postvectis) have been recorded in the Mokau catchment.
Fish were batch stained by immersion in solutions of Rhodamine B (0.2 g L−1) or Bismark brown (0.05 g L−1) immediately after capture. Rhodamine B leaves the whole fish stained pink, while Bismark brown stains the whole fish orange. To increase survival and stain retention, seawater was added to the solutions to produce a salinity of c. 17%. During staining, the solution was aerated and ice was used to maintain the solutions at ambient river temperature, when required. Fish were immersed for 3 h in either solution before being removed with dip nets and either batch weighed or counted before release. When the fish were weighed (accuracy 0.1 g), the mean fish weight (determined by weighing and counting 10 subsamples) was used to estimate the total number of fish released. Fish were either released immediately or held in 60 L live bins within the river for up to 12 h before release.
Laboratory trials had previously determined the mortality rate from the staining solution was negligible, and that the stains were visible for seven days. No behavioural changes were observed after exposure to either stain. During the study, control samples of stained fish were held in live bins for the duration of each trial (usually five days). Fish were not fed during the trial period, but were inspected daily for mortality and stain retention (). Although the staining procedure does not appear to influence natural mortality, it may increase predation levels of whitebait by increasing their visibility to predators, especially birds given inanga migrate in the top 1 m of the water column (Stancliff et al. Citation1988b).
Table 1 Stained whitebait recapture data for migration rate experiments carried out on the Mokau River, September 1998, September–October 2001 and September–October 2003.
Migration rate experiment
In total, nine mark and recapture trials with stained inanga were undertaken during the migration season (August–November) in 1998, 2001 and 2003 (). Seven trials were carried out to examine the effect of flow on the migration rates of whitebait; three trials at near mean river flows, two trials at low river flow (close to the mean annual seven day low flow) and two trials at high river flows (). To determine if whitebait migrate at night, fish were released both in the morning (after sunrise) and in the evening (c. sunset; ).
For all seven trials, fish were released on the true right river margin (right side of the river when facing downstream) approximately 1 km upstream of the mouth of the Mokau River (), on incoming (rising) tides of similar amplitude and timing (max. height 1.35 m to 1.46 m and high tide time 0927 h to 1111 h; ).




![Figure 2 The distribution of recaptured whitebait on the Mokau River. A, A morning release with an average river flow; B, an evening release with an average river flow. The percentage of total whitebait recaptures at sites along the rivers are indicated by different coloured circles, [Display full size] greater than 10% of recaptured fish, [Display full size] between 5% and 10% of recaptured fish, [Display full size] between 1% and 5% of recaptured fish, [Display full size] less than 1% of recaptured fish. The black cross represents the downriver release point of stained fish, and AB represents Awakau Bridge, the upriver release point. The stand used for trap efficiency trials is indicated by the star.](/cms/asset/6543b060-01d7-43f0-a8f6-42f6b0822f35/tnzm_a_941372_f0002_c.jpg)
In addition, two releases (a morning and an evening release) were made at the end of the tidal zone, 22 km upriver, at the Awakau Bridge (). At this point, the river is still similar in nature to the lower reaches but no whitebaiting occurs upstream of the bridge. As inanga will recruit and reside in tidal reaches of large rivers, these upriver releases were undertaken to determine the proportion of whitebait that remain in the tidal zone or move back downstream once they reach the upper limit of tidal influence.
Once a trial commenced, fishermen were surveyed for the next three to five days. The number of stained whitebait caught by each fisherman was recorded, along with the GPS coordinate, and the time and date of capture. Fishing could only be carried out during the regulation daylight hours of 0500 h to 2000 h (NZST). Fishermen were also supplied with a form that could be placed in drop boxes in case they ceased fishing before the information could be collected.
Trap efficiency experiment
The effect of water velocity around the whitebait trap on the capture rate of whitebait was examined at one fishing stand 5.3 km upriver of the mouth (). A standard whitebait trap was used, measuring 1.7 m in length, with an opening of 1.3 × 0.8 m, set with an adjoining screen which allowed the trap to protrude 6 m from the water's edge. Five trials were undertaken over two consecutive days in November 2003 at mean daily flows of 27.2 and 23.1 m3 s−1. Each release occurred at a different point of the tidal cycle (height of high tides 1.25–1.46 m), encompassing both an incoming and outgoing tide.
For each trial, 100 stained fish (using Rhodamine B [0.2 g L–1] as previously described) were released c. 3 m downstream (relative to river flow) of the whitebait trap. Fish were released immediately after being removed from the solution and were monitored for 2 h, recording the number of fish captured. Velocity measurements (Marsh McBirney Flo-mate 2000 electromagnetic velocity meter) were taken along the face of the screen and trap, and at 0.1 and 1 m from the outside edge of the trap before each release.
Statistical analysis
The migration rate of whitebait was calculated from the distance travelled (i.e. from release to capture point), divided by the time taken from release to capture. Due to the complexity of a river estuary, where rising and falling tides cause water velocities to change continually, individual fish may experience different local water velocities throughout the tidal cycle. Therefore, the migration rate as calculated does not reflect the swimming speed of the fish, but rather the relative speed of travel taking into account the influence of the river current and the tide.
The calculated migration rates should only ever be considered estimates, as they are based on a small proportion of marked fish recaptured by fishermen (less than 30% of all fish released). Furthermore, fishermen generally only lift their net two to four times per hour, so the precise time fish were captured is not known.
Significant differences in the migration rates of whitebait between day 1 and day 2 were identified using t-tests. Only recaptures for the first two days have been analysed, as this accounted for the majority of fish recaptured. As multiple comparisons were performed, the Sidak inequality (Hsu Citation1996) was applied to each comparison, reducing the significance level for any individual comparison to α = 0.02 to set the experiment-wise error rate at α = 0.05.
Results
The percentage of whitebait recaptured ranged between 2.7% and 26.9% () with a mean recapture rate of 11.1%. The number of fish recaptured was highest in the first two days after release. Both river flow and water velocity around the whitebait trap affected the recapture rate of fish. Recapture rates of stained fish increased with river flow () and with increasing velocity around the whitebait trap (). Based on trap efficiency trials (), the highest recapture rates occurred on an outgoing tide when water velocity around the trap was highest and the lowest recapture rates were recorded on an incoming tide, when water velocities were moving in an upstream direction.
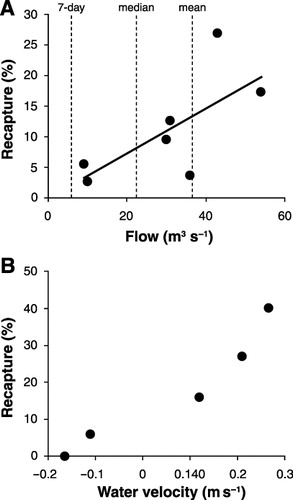
Fish travelled upriver faster on the first day after release than on the second day (), with increasing river flow reducing migration rates (cf. –). For evening releases, when the river flow was low () fish moved upstream between 0.2 and 1.1 km h−1, with an average migration rate of 0.61 km h−1 for the first day of capture. Fish released at near average river flow () travelled upstream between 0.1 to 0.7 km h−1, with an average migration rate of 0.39 km h−1, significantly slower than when the river flow was low (P < 0.0001). Fish released when the river flow was higher than average () travelled upstream between 0.1 and 0.5 km h−1, with an average migration rate of 0.32 km h−1. This is significantly slower (P < 0.0001) than at average and low river flows.
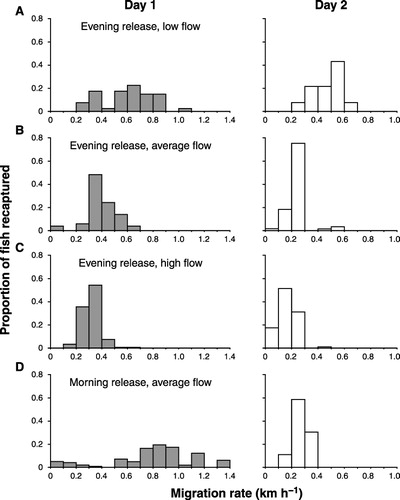
Similar effects of flow on migration rates were observed on the second day after release. Fish released on a low river flow () were moving upstream significantly faster (P < 0.0001) than fish released on an average river flow (), which in turn were travelling upstream significantly faster than those fish released on a higher than average river flow (; P < 0.0001).
At an average river flow, fish released in the morning exhibited greater variation in their migration rate than those released in the evening. Most fish released in the morning and captured on the first day of fishing travelled between 0.5 and 1.2 km h−1 (). This was significantly faster than fish released in the evening at a similar river flow (P < 0.0001).
Time of release affected the distance fish travelled upriver on the first day after release. When fish were released in the evening at an average river flow (), fish recaptured the following day (recaptures were recorded from 13 to 21 h after release) had travelled between 4 and 12 km upriver, with an average distance of 7.7 km. In comparison, fish released in the morning with an average river flow () (recaptures were recorded from 2 to 9 h after release) had reached a maximum of 4 km upriver, with an average distance of 2.3 km travelled. This is significantly less distance (P < 0.0001) than fish released in the evening with comparable river flow. also highlights the difference in recapture locations for whitebait released on a morning and an evening tide. The tide height and timing of high tide during all the trials were similar () and therefore are expected to have had a similar influence on migration rate. If fish were only moving diurnally, then subtracting the hours of darkness (11 h, 20 min), fish released in the evening would have travelled between 4 and 12 km in c. 1.5 to 9.5 h, which is three times the distance travelled by fish released in the morning. This indicates that fish either make extensive use of the dawn and dusk period when there is no fishing undertaken, and/or fish are able to progress upriver at night.
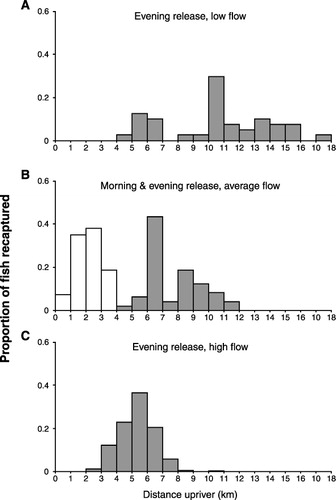
River flow also influenced migration rates of fish. When fish were released in the evening at a low river flow, they were recaptured between 4 and 18 km upstream within 21 h, giving a mean distance travelled of 10.6 km (). Fish released in the evening at an average river flow () travelled only 12 km upriver, with a mean distance travelled of 7.7 km, which is significantly less than at low flow (P < 0.0001),. Fish released in the evening with a higher than average flow () travelled significantly less distance upriver than at mean and low flows (P < 0.0001), reaching a mean distance of 5.3 km upstream.
When fish were released 22 km upriver, near the end of the tidal zone, between 1% and 8.5% of stained fish moved back downstream (). These recapture rates were markedly lower than for fish released near the mouth of the river on the same day (). The majority of recaptured fish (c. 63%) were caught within 500 m of the release point (). The remaining fish were recaptured up to 15 km downstream over the five days following release.
Discussion and conclusions
Increasing river flow was positively correlated to the recapture rates of upstream migrant juvenile galaxiids. This is in agreement with McDowall & Eldon (Citation1980) who suggested that larger whitebait catches would be expected after floods when river flow is high and the river is more turbid. Juvenile galaxiids prefer low velocity waters for migration (< 0.1 m s−1; McDowall & Eldon Citation1980), and fish often move upstream in the low velocity surface waters (≤1.0 m deep) along riverbank margins (Stancliff et al. Citation1988b). As tidal waters recede, river flows produce stronger water velocities around whitebait traps for fish to negotiate. The results of the present study suggest that once water velocities around fishermen's traps surpass 0.1 m s−1, marked increases in whitebait catches are expected. Higher flows are generally associated with higher turbidity (Hicks et al. Citation2004) and this could also reduce the ability of fish to visually detect the trap, leading to increased catches.
The proportion of stained fish recaptured during the study ranged between 2.7% and 26.9%. These figures are expected to be the minimum proportion of the whitebait run captured by fishermen. This is because some fishermen may not have reported their true catch of marked fish, and some captures may have occurred outside of the monitoring period, after the stain was no longer easily recognisable. Some fish may also have moved back out to sea after release. In addition, the staining procedure may have rendered whitebait more vulnerable to predation and therefore recapture rates may represent a higher proportion of the marked population. That given, the proportion of whitebait escaping fishing pressure and accessing upriver habitats is likely to be around 70% of entrants in the Mokau River.
Increasing river flow negatively impacted on the migration rates of marked fish. However, across all trials, juvenile galaxiids were travelling upstream at between 0.1 and 1.4 km h−1 within 24 h of release. These rates of movement are similar to those obtained from mark and recapture studies of juvenile galaxiids in the tidal zone of other New Zealand rivers, the Awakino River (up to 1.43 km h−1; Allibone et al. Citation1999) and the Oparau River (0.2 and 1.1 km h−1; C. Baker unpubl. data). However, these migration rates are substantially higher than those reported by Stancliff et al. (1Citation988b), who estimated that inanga migrated up the Waikato River at a rate of only 0.31 km day−1(c. 0.03 km h−1) and banded kōkopu moved upriver at 1.96 km day−1 (c. 0.16 km h−1). The present study is only looking at movement rates within the tidal zone, where fish can exploit upriver tidal transport, and migration rates are indeed rapid in comparison to upstream movement solely against a river flow.
However, not all whitebait exhibited rapid upstream migration following release. A lower migration rate was calculated for fish captured on the second day after release than on the first. This may be because not all fish began migrating upstream on the first tidal cycle. In general, fishermen have reported schools of whitebait moving downstream at the beginning of the season (McDowall & Eldon Citation1980), and in the present study fish liberated at the upper end of the tidal zone showed that a small proportion of fish can move a substantial distance downstream. Therefore, some degree of downstream movement, or staging within the tidal zone by juvenile galaxiids, may occur during their upriver migration.
Variable retention times within estuaries and tidal zones are commonly seen in fish species whose juveniles migrate from marine to freshwater systems. Upon entering estuaries, Sicyopterus stimpsoni remain in the estuary for 48 h while undergoing a metamorphosis of the cranium, which enables the goby to use its lips to negotiate waterfalls (Schoenfuss et al. Citation1997). Other gobies such as Lentipes concolor, remain in estuaries no longer than one day before entering riverine habitats (Keith Citation2003), whilst Awaous guamensis may spend four or more weeks in river estuaries (Tate Citation1997). After arriving at river mouths, a proportion of glass eels become resident in marine and estuarine habitats, whilst other individuals take advantage of upstream tidal transport and quickly colonise freshwater habitats (Edeline et al. Citation2006; Jellyman et al. Citation2009; Sullivan et al. Citation2009).
An array of ecological drivers is likely to control the migration rates of juvenile galaxiids through tidal zones. Fish condition, water temperature, olfactory cues and biological rhythms have all been implicated in influencing the migratory behaviour of glass eels upon entering a river system (Sola Citation1995; Edeline et al. Citation2004, Citation2006; Sullivan et al. Citation2009). Although speculative, retention in estuarine or tidal zones by juvenile galaxiids could represent a strategy utilised by smaller fish to enable further development before tackling strong river currents. Stancliff et al. (1Citation988b) found an increase in the size and condition of juvenile inanga with increasing distance upriver, which suggests inanga are feeding and growing during their upriver migration. Regardless of the factors controlling migratory behaviour of juvenile galaxiids, any delay to migration or downstream movements by fish whilst within the tidal zone of river systems would render fish more susceptible to repetitive fishing pressure, reducing their chances of successful recruitment to upstream habitats.
Results of the present study indicated that juvenile galaxiids in the Mokau River migrated upriver during the overnight tidal cycle. These results are in contrast to those of McDowall & Eldon (Citation1980) who, based on repeated fishing between dusk and dawn producing negligible returns, concluded that whitebait perform diurnal migrations and fish generally do not migrate at night. However, their trials were carried out on dark overcast nights, whereas the night releases in the Mokau River were undertaken within one day of the full moon, with clear skies most evenings. The light cast by the moon may therefore stimulate some fish to actively migrate at night. Diel migration patterns have also been observed in goby species. Although several juvenile gobies (L. concolor, Lentipes kaaea and Sicyopterus stimpsoni) have been observed to enter rivers primarily during daylight hours (Nishimoto & Kuamo'o Citation1997; Keith Citation2003; Benbow et al. Citation2004), other species (Awaous guamensis, Stenogobius hawaiiensis and Eleotris sandwicensis) have been recorded entering rivers both diurnally and nocturnally (Nishimoto & Kuamo'o Citation1997; Tate Citation1997). In addition, Eleotris fusca mainly utilise the evening tide during upriver migration (Keith Citation2003). Thus, fish species that take advantage of tidal transport during their upriver migration may not restrict activity exclusively to diurnal or nocturnal hours.
If whitebait only migrate upriver diurnally, fishing authorised during daylight hours would not aid the recruitment of whitebait to upstream habitats. Should whitebait progress upstream at night, higher levels of escapement leading to increased recruitment would be expected, especially in smaller rivers and streams where fish could pass the tidal fishing area on a single overnight tidal cycle. The long tidal reach in the Mokau River, however, subjects migrating whitebait to fishing pressure for some 22 km upriver. Results from the present study show that the mean distance travelled by whitebait was between 5 and 10 km per day, depending upon river flow. Therefore, fish will need two to four days to pass the fishing area, and this considerably reduces the chances of fish escaping capture. These findings have management implications for river systems where whitebait are exposed to multiple days fishing pressure. Reducing fishable zones could be one means of reducing pressure on unstable or declining whitebait populations. This could include restricting the length of river where fishing stands are permitted or, dependent upon river size, the number of fish stands permissible. Further options could include reducing the length of the fishing season or incorporating whitebait into the quota management system where each recreational fisher has a maximum allowable catch per season.
Acknowledgements
We wish to thank Ray and Bev Christiansen and Harry Page for supplying the whitebait used in the trials, Ray and Bev also assisted during the trap efficiency trials and made their whitebait stand available for this part of the study. We also thank the NIWA staff who participated in the study and all fishermen for their cooperation. This study was funded by the New Zealand Foundation for Research, Science and Technology (Contract No. C01X210).
References
- Allibone R, Boubèe J, West D 1999. The ones that got away: determining whitebait movements and rates of escape. Water & Atmosphere 7: 11–13.
- Allen GR, Midgley SH, Allen M 2002. Field guide to the freshwater fishes of Australia. Collingwood, Western Australian Museum. 394 p.
- Baker CF, Smith JP 2007. Habitat use by banded kokopu (Galaxias fasciatus) and giant kokopu (G. argenteus) co-occurring in streams draining the Hakarimata Range, New Zealand. New Zealand Journal of Marine and Freshwater Research 41: 25–33. 10.1080/00288330709509893
- Benbow ME, Burky AJ, Way CM 2004. The use of two modified Breder traps to quantitatively study amphidromous upstream migration. Hydrobiologia 527: 139–151. 10.1023/B:HYDR.0000043191.43333.d5
- Edeline E, Dufour S, Briand C, Fatin D, Elie P 2004. Thyroid status is related to migratory behavior in Anguilla anguilla glass eels. Marine Ecology Progress Series 282: 261–270. 10.3354/meps282261
- Edeline E, Lambert P, Rigaud C, Elie P 2006. Effects of body condition and water temperature on Anguilla anguilla glass eel migratory behavior. Journal of Experimental Marine Biology and Ecology 331: 217–225. 10.1016/j.jembe.2005.10.011
- Franklin PA, Bartels B 2012. Restoring connectivity for migratory native fish in a New Zealand stream: effectiveness of retrofitting a pipe culvert. Aquatic Conservation: Marine and Freshwater Ecosystems 22: 489–497. 10.1002/aqc.2232
- Hanchet SM, Hayes JW 1989. Fish and fisheries values of the Mokau River and tributaries draining the Mokau coalfield. New Zealand Freshwater Fisheries Report 110. 79 p.
- Hickford MJH, Schiel DR 2010. Population sinks resulting from degraded habitats of an obligate life-history pathway. Oecologia 166: 131–140. 10.1007/s00442-010-1834-7
- Hicks M, Quinn J, Trustrum N 2004. Sediment load and organic matter. In: Hardin, JS, Mosley MP, Pearson CP, Sorrell BK eds. Freshwaters of New Zealand. Wellington, New Zealand, New Zealand Hydrological Society and New Zealand Limnological Society. Pp. 12.1–12.16.
- Hsu JC 1996. Multiple comparisons: theory and methods. Florida, USA: Chapman and Hall. 277 p.
- Hugo CC 1973. Migration of Galaxias maculatus (Jenyns) (Galaxiidae, Pisces) in Valdivia Estuary, Chile. Hydrobiologia 43: 301–312. 10.1007/BF00015353
- Jellyman DJ, Booker DJ, Watene E 2009. Recruitment of Anguilla spp. Glass eels in the Waikato River, New Zealand. Evidence of declining migrations? Journal of Fish Biology 74: 2014–2033. 10.1111/j.1095-8649.2009.02241.x
- Jowett IG, Richardson J, Boubée JAT 2009. Effects of riparian manipulation on stream communities in small streams: two case studies. New Zealand Journal of Marine and Freshwater Research 43: 763–774. 10.1080/00288330909510040
- Keith P 2003. Biology and ecology of amphidromous Gobiidae of the Indo-Pacific and the Caribbean regions. Journal of Fish Biology 63: 831–847. 10.1046/j.1095-8649.2003.00197.x
- McDowall RM 1984. The New Zealand whitebait book. Wellington, New Zealand: A. H. & A. W. Reed Ltd. 210 p.
- McDowall RM 1990. New Zealand freshwater fishes. A natural history and guide. Auckland, New Zealand: Heinemann Reed. 553 p.
- McDowall RM 2006. Crying wolf, crying foul, or crying shame: alien salmonids and a biodiversity crisis in the southern cool-temperate galaxioid fishes? Reviews of Fish Biology and Fisheries 16: 233–422.
- McDowall RM, Eldon GA 1980. The ecology of whitebait migrations (Galaxiidae: Galaxias spp.). New Zealand Ministry of Agriculture and Fisheries, Fisheries Research Bulletin 20: 171 p.
- McIntosh AR, McHugh PA, Dunn NR, Goodman J, Howard SW, Jellyman PG, et al. 2010. The impact of trout on galaxiid fishes in New Zealand. New Zealand Journal of Ecology 34: 195–206.
- Nishimoto RT, Kuamo'o DGK 1997. Recruitment of goby postlarvae into Hakalau stream, Hawaii island. Micronesica 30: 41–49.
- Rowe DK, Saxton BA, Stancliff AG 1992. Species composition of whitebait (Galaxiidae) fisheries in 12 Bay of Plenty rivers, New Zealand: evidence for river mouth selection by juvenile Galaxias brevipinnis (Günther)). New Zealand Journal of Marine and Freshwater Research 26: 219–228. 10.1080/00288330.1992.9516517
- Rowe D, Hicks M, Richardson J 2000. Reduced abundance of banded kokopu (Galaxias fasciatus) and other native fish in turbid rivers of the North Island of New Zealand. New Zealand Journal of Marine and Freshwater Research 34: 547–558. 10.1080/00288330.2000.9516956
- Schoenfuss HL, Blanchard TA, Kuamo'o DG 1997. Metamorphosis in the cranium of postlarval Sicyopterus stimpsoni, an endemic Hawaiian stream goby. Micronesica 30: 93–104.
- Sola C 1995. Chemoattraction of upstream migrating glass eels Anguilla anguilla to earthy and green odorants. Environmental Biology of Fishes 43: 179–185. 10.1007/BF00002489
- Stancliff AG. Boubée JAT, Mitchell CP 1988a. The whitebait fishery of the Waikato River. New Zealand Freshwater Fisheries Report 95. 68 p.
- Stancliff AG. Boubée JAT, Palmer D, Mitchell CP 1988b. The upstream migration of whitebait species in the lower Waikato River. New Zealand Freshwater Fisheries Report 96. 44 p.
- Sullivan MC, Wuenschel MJ, Able KW 2009. Inter- and intra-estuary variability in ingress, condition and settlement of the American eel Anguilla rostrata: implications for estimating and understanding recruitment. Journal of Fish Biology 74: 1949–1969. 10.1111/j.1095-8649.2009.02252.x
- Swales S 1991. Threats and conservation of native fish. Freshwater Catch 45: 19–21.
- Tate DC 1997. The role of behavioral interactions of immature Hawaiian stream fishes (Pisces: Gobiodei) in population dispersal and distribution. Micronesica 30: 51–70.