Abstract
Disturbance events can regularly impact stream ecosystems; however, large-scale catastrophic disturbances are rare. From September 2010 to September 2011 Christchurch City experienced over 8500 earthquakes including a magnitude 7.1. One consequence was catastrophic additions of silt and sand into waterways throughout the city. Of 161 km of permanent waterways, 102 km (63%) were affected by earthquake siltation. Benthic invertebrates and fish communities were compared across 16 streams with differing siltation. Invertebrate taxonomic richness decreased significantly (mean 17 taxa reduced to 10 taxa) and EPT taxa (Ephemeroptera, Plecoptera and Trichoptera) were removed entirely from streams receiving heavy siltation. Fish richness and density decreased significantly, with fish absent from some heavily silted streams. Many of these urban streams are sourced from springs and their stable flows and low gradient limit their ability to flush sediment. We predict that without human intervention there will be a long-term sediment legacy and it may take many years for these streams to recover from this catastrophic disturbance.
Introduction
Disturbance is an important concept in stream ecology and disturbance events are well recognised as critical drivers of stream communities (Resh et al. Citation1988; Lake Citation2000). The effects of several stream disturbance types have been well documented, including: floods and droughts (Grimm & Fisher Citation1989; Scrimgeour & Winterbourn Citation1989); fires (Mihuc & Minshall Citation1995); land use changes related to agriculture, forestry and urbanisation (Davies & Nelson Citation1994; Harding et al. Citation1998; Harding Citation2003; Winterbourn et al. Citation2007); and dam construction (Young et al. Citation2004). The effects of large-scale tectonic disturbances such as volcanic eruptions on streams have also been documented; however, the effects of catastrophic disturbance events such as earthquakes have seldom been recorded (Collier Citation2002; Chen et al. Citation2004; Xu et al. Citation2011; Wells et al. Citation2013).
Major earthquakes (local magnitude, ML, 7+) are a global phenomenon and occur relatively frequently around the Pacific Rim. Despite their frequency, and the significant destruction they bring, the effect of earthquakes on the ecology of streams and rivers has not been well studied. Not surprisingly, the focus after these events is on the safety and recovery of people, and the impacts on the ecology of the affected waterways have been a lower priority. On 4 September 2010, the Canterbury region experienced an earthquake 7.1 ML; six months later on 22 February 2011, Christchurch City was struck by a further 6.3 ML quake. On 13 June 2011 the city experienced double quakes of 5.6 and 6.0 ML. Overall between 4 September 2010 and 23 April 2011 the wider Christchurch region experienced 6553 earthquakes, and by 12 September 2011 this had reached 8583 earthquakes. These earthquakes have caused loss of life, injuries and significant damage to parts of the city. In addition to the human cost of this disaster the earthquakes triggered marked changes in the morphology and hydrology of stream networks throughout the city.
The impacts of earthquakes on stream systems are liable to be highly variable depending on the magnitude of the earthquake(s), regional geological conditions, season, and the type and intensity of any human activities occurring in close proximity to affected waterways. The Canterbury earthquakes impacted streams throughout the city primarily through three pathways: the hydrology of the rivers was fundamentally modified; physical in-stream and bank habitat conditions were altered; and water quality was directly affected. Hydrological changes occurred due to movement in the bed height, in-filling by sediment and changes in the water table. Water quality was affected as untreated sewage from burst sewers overflowed directly into the mid to low reaches of the rivers. For example, on 22 June 2011 an estimated 47,000 m3 of sewage was pumped from damaged sewers into one of the city's main rivers, the Avon River (Christchurch City Council, pers. comm. 2011). A combination of significant ground subsidence and ground uplift (up to 1 m in either direction) occurred across the city and, in combination with alterations to aquifer flows, resulted in changes to the water table by several metres in some areas. Bank collapse and slumping caused channel narrowing and bed in-filling due to sand and silt associated with the earthquakes. These hydrological changes resulted in flooding and bank overflow in many lower reaches.
One major unusual consequence of these earthquakes was widespread liquefaction throughout the northern and eastern suburbs of the city. Liquefaction occurs when the vibration of subsoils causes sand and silt to liquefy and breach the surface. The February and June earthquakes resulted in excess of 580,000 tonnes of sand and silt erupting and ‘flooding’ streets and properties. This liquefied sediment also flooded into streams from the bed, banks and via the stormwater system. As a result, numerous small tributary streams received catastrophic waves of sediment up to 1 m deep, which completely filled in some streams and severely clogged others.
The aims of this study were to determine the extent and magnitude of earthquake-derived sand and silt in the main urban streams of Christchurch, and also determine the impact on benthic invertebrate and fish communities; changes in hydrology and sewage impacts are not addressed here. Unfortunately, there is limited data on the benthic or fish communities at these sites prior to the earthquakes and so we have compared streams with minimal earthquake impacts with those more severely affected and assumed that major differences in communities are the result of earthquake impacts.
Methods
Study sites
Christchurch City lies on the eastern coastal edge of the Canterbury Plains, in the South Island of New Zealand. Christchurch prior to the earthquake of 4 September 2010 had a population of 376,700 (Christchurch City Council Citation2010). The city includes four major river systems. The Styx River to the north of the city is lightly urbanised with newer residential subdivisions and horticulture. In contrast, two other systems, the Avon and Heathcote rivers are almost entirely within the city's urban area, while the fourth, the Halswell River, lies on the southeastern boundary of the city and is lightly urbanised. This study focused on the two main central city rivers, the Avon and Heathcote, both of which flow west to east and are fed by numerous small first order springs and channelised water courses. The Avon is a third order river, with approximately 99 km of permanent waterways and 48 named tributaries (11 of which have ephemeral surface flow). The Heathcote is a fifth order river, with 62 km of waterways and 45 named tributaries, 15 of which are ephemeral tributaries. Both river systems flow into the Avon–Heathcote Estuary which forms the eastern boundary of the city. There are a number of other small first order channelised streams which are not connected to either the Avon or Heathcote rivers but which flow into the estuary and have not been included in this analysis.
Due to the damage to roads and bridges, issues with health and safety, and a lack of access to equipment, this study was not conducted as quickly as preferred. Although a number of sites were sampled as early as March 2011, most parameters presented here were sampled intensively in September 2011, three months after the June sequence of silt-generating earthquakes.
Spatial extent of waterways impacted by earthquake-derived silt
A visual survey was conducted of 104 sites on tributary streams and the main stem of the Avon and Heathcote rivers. Christchurch's urban streams had significant silt deposits prior to these earthquakes; however, the sand and silt generated by liquefaction was visually distinctive and had little organic matter. Laboratory analysis has shown that this earthquake-derived sand and silt is < 500 µm, and 80% of its composition ranges from 45–80 µm (authors' unpublished data). During the survey, sediment depth and cover were estimated at each stream reach. The reach was then placed into one of four categories: no earthquake-derived silt; light siltation (< 1 cm depth); medium siltation (1–10 cm, generally < 5 cm); or heavy siltation (> 10 cm depth). The length of stream upstream and downstream to the next tributary were treated as belonging to the sediment category of that site. This data was then merged with a GIS river network (Christchurch City Council, pers. comm. 2009) using ArcGIS and used to generate estimates of the spatial extent of silt in the Avon and Heathcote rivers.
How much silt was deposited in waterways?
In order to determine the magnitude of earthquake-derived silt in these waterways we sampled 16 streams; four with no obvious earthquake-derived silt; four with light siltation (< 1 cm depth); four with medium levels of siltation (1–10 cm, generally < 5 cm); and four with heavy siltation (> 10 cm depth). At each stream a 50 m reach was identified and a visual estimate of the percentage of fine sediment was made at five random transects across the stream; visual estimates have been shown to provide remarkably accurate indications of sediment cover in New Zealand (Clapcott et al. Citation2011). In addition, sediment depth was measured at five points along each transect. These data were used to determine the four silt stream types (no silt, light, medium and heavily silted) and estimate sediment bed load at each reach and for each stream.
Water chemistry and physical conditions
A range of water chemistry, in-stream physical and riparian habitat conditions were measured at each site. Spot measurements of pH, specific conductivity and temperature were collected using a YSI 63 meter and dissolved oxygen was measured using a YSI 660 meter. Each site was characterised physically by measuring mean wetted width (across three randomly selected locations), mean water depth and mean velocity (at five points across one transect). Mean velocity was measured with a Marsh-McBirney Flo-mate 2000 at 60% of the depth of the water column. Mean bed substrate size was estimated at each site by measuring the particle size of approximately 50 randomly collected particles and determining a substrate index (SI) score modified from Jowett & Richardson (Citation1990). This score allocates particles to Wentworth particle size classes, and scores these on a scale ranging from 1–8 (1 being entirely vegetation and 8 entirely bedrock). The particle size score is then weighted based on the percent occurrence. Overall stream reach physical stability was assessed using the procedure of Pfankuch (Citation1975) and a range of riparian conditions were also assessed at each site using the Stream Habitat Assessment Protocol 2 of Harding et al. (Citation2009).
Stream biota
In each reach four benthic invertebrate samples were collected. These included three Surber samples (0.1 m2, 0.5 mm mesh) in riffles/runs and a single composite kick-net sample (mesh 0.5 mm) taken across a range of microhabitats (e.g. riffles, runs, pools, leaf packs) after Protocol 2 in Stark et al. (Citation2001). The samples were preserved in the field in 70% ethanol and returned to the laboratory for processing. Benthic invertebrates were counted and identified to the lowest taxonomic level possible using keys by Winterbourn et al. (Citation2000).
Fish communities were assessed by night spotlighting 50 m reaches at each site. Night spotlighting enables night active species (most indigenous New Zealand fish species) to be recorded; however, it is less effective in fast, white water and highly turbid conditions. Due to ongoing earthquakes electric fishing equipment was not available at the time of sampling.
Data analysis and statistics
A range of benthic invertebrate metrics were calculated in order to determine stream health. EPT richness (Ephemeroptera, Plecoptera and Trichoptera), the number of mayfly, stonefly and caddisfly taxa present at a site, is often used as an indicator of impacts as these taxa are generally considered sensitive to anthropogenic pollution. Another metric used was the Macroinvertebrate Community Index (MCI; Stark Citation1985). This is an organic pollution index that allocates tolerance scores to all taxa present in a sample. Combined taxa scores for a reach are summed and a score ranging from 0 to 200 is calculated. Scores above 120 indicate excellent health while scores < 60 indicate very poor health.
Water chemistry, sediment and biotic indices were tested for normality and compared across sediment classes using one-way Analysis of Variance (ANOVA) in R (The R Foundation for Statistical Computing). Significant differences, at P < 0.05, between treatment means were evaluated using Tukey's post hoc comparisons.
Results
Spatial extent of siltation in waterways
GIS analysis indicated that of the 161 km of waterways in the combined Avon and Heathcote rivers approximately 102 km (63%) were affected by earthquake-derived silt and sand. Both rivers had similar silt coverage, with 62% of the Avon and 64% of the Heathcote having light to heavy silt coverage (). Heavily silted sites were predominantly in the eastern suburbs of Christchurch and occurred in smaller first and second order tributaries, as well as the main stems of the lower Avon and Heathcote rivers. Liquefaction seemed to affect the full range of stream sizes occurring in the eastern catchments of the city.
Degree of siltation in waterways
Substrate characteristics such as mean substrate size, fine sediment cover and deposited sediment were significantly different across the four categories (). At non- and lightly silted sites, substrate size was significantly larger (one-way ANOVA, F3,12 = 11.764, P < 0.001) and fine sediment cover and deposited sediment were significantly reduced compared to medium and heavily silted sites (ANOVA F3,12 = 14.859, P < 0.001 and F3,12 = 3.820, P < 0.05, respectively). Several medium and all heavily silted sites had 100% silt cover and, in the worst cases, this silt was up to 1 m deep (). Digging into this silt revealed that many of the pre-earthquake beds were dominated by cobbles and gravels.
Sites were allocated to one of four categories of siltation (n = 4), statistically significant differences are shown with statistical values in text. **Indicates statistically significant differences at P < 0.01. Statistical values are shown in text. The absence of error bars indicates no variability.
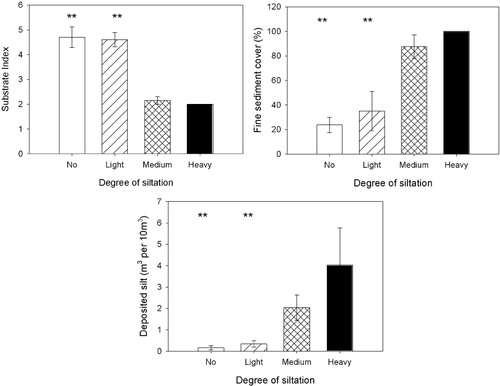
Effects on physical habitat and water chemistry
Water chemistry characteristics showed minor variation between the four categories of sites. All sites had circum-neutral pH and although specific conductivity increased with higher sediment loads, no statistically significant differences were detected (). Dissolved oxygen levels were lower in sites with light sedimentation; however, these values were still above those likely to have any effect on benthic communities. Measures of channel stability and riparian condition were also not significantly different between sediment categories ().
Table 1 Physical and chemical characteristics of 16 sites on the Avon and Heathcote rivers within four sediment categories (no silt, lightly silted, medium siltation and heavily silted) in September 2011.
Impacts on stream biota
At total of 48 benthic invertebrate taxa were recorded across all 16 sites. These were dominated by caddisflies, diptera, snails and crustacea. All benthic invertebrate metrics indicated that high-silt communities were markedly impacted. Taxonomic richness was highest in a site with light siltation (25 taxa) and lowest at a site with heavy siltation (six taxa), and mean taxonomic richness was significantly reduced at heavily silted sites compared to the other sites (; one-way ANOVA, F3,12 = 9.121, P < 0.01). The numbers of EPT taxa were generally low at all sites, and mayflies and stoneflies were not recorded at any sites. However, EPT were greatly reduced in medium siltation and no EPT taxa were found in any heavily silted sites (; one-way ANOVA, F3,12 = 4.907, P < 0.05). The MCI was also low at all sites, ranging from 86 in a non-silted site to 50 in a heavily silted site. MCI values declined as the degree of siltation increased; however, significantly different values were only recorded between no silt and heavy silt sites (; one-way ANOVA, F3,12 = 6.170, P < 0.01). The community composition of benthic communities also changed markedly with increased siltation (). Caddisflies (including the Hydroptilid Oxyethira, and the Leptocerid Hudsonema) and amphipods (e.g. Paracalliope sp.) declined as levels of silt increased, and in heavily silted sites diptera (primarily Chironomidae) and oligochaetes became more dominant. The common New Zealand Hydrobiid snail Potamopyrgus also declined markedly between medium and heavily silted sites.
Sites were allocated to one of four categories of siltation (n = 4). **Indicates statistically significant differences at P < 0.01; * indicates statistically significant differences at P < 0.05. Statistical values are shown in text.
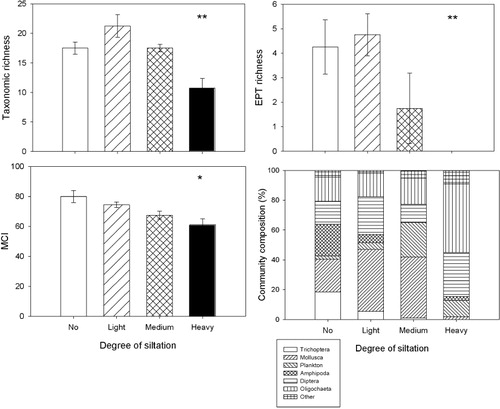
Fish diversity was generally low across the city with only six taxa able to be confidently identified. This included juvenile galaxiids which might comprise of up to five species, but were probably either inanga (Galaxias maculatus) or kōaro (G. brevipinnis), as these two species account for almost all of the migratory population in September. Fish communities were severely impacted by siltation, with fish richness significantly lower in heavily silted sites compared to non-silted sites, and fish density significantly higher in no and light silt compared to medium and heavy silt sites (; one-way ANOVA, F3,12 = 4.200, P < 0.05 and F3,12 = 7.929, P < 0.01, respectively). In no and light silt sites common bullies (Gobiomorphus cotidianus) dominated the community; however, in the four heavily silted sites only a single eel (Anguilla sp.) and a single whitebait (Galaxias sp.) were observed.
Sites were allocated to one of four categories of siltation (n = 4). **Indicates statistically significant differences at P < 0.01. Statistical values are shown in text. The absence of an error bar indicates no variability.
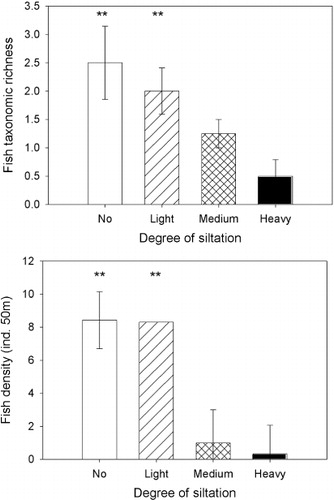
Discussion
Despite the frequency of earthquakes globally very little research seems to have been done on their impacts on the ecology of streams and rivers (Chen et al. Citation2004; Xu et al. Citation2011). Many recent major earthquakes have occurred in regions where freshwater researchers are rare (e.g. Haiti) and, not surprisingly, the emphasis post-earthquake is on the rescue of citizens and preserving life. The Canterbury earthquakes in 2010–2011 are unusual in this context, in that although there were fatalities and considerable damage to areas of the city there were also major portions of the city which were relatively unaffected and there was the capacity to undertake some post-earthquake assessment of the impacts on streams and rivers.
The impacts of these earthquakes on streams were multifaceted. Ruptured sewer systems resulted in the discharge of untreated sewage into the lower reaches of the main rivers; riverbank subsidence and alterations in the water table resulted in flooding and marked changes in localised channel morphology and hydrology. Despite these potentially confounding factors we were able to access a range of sites which were impacted primarily by the catastrophic addition of large quantities of ‘liquefied’ silt and sand. The process of liquefaction was known prior to these earthquakes, although the risk of liquefaction was generally considered low as it had not actually occurred at any scale in New Zealand. Thus the extent and volume of silt generation by liquefaction which occurred in September 2010, and February and June 2011, were unprecedented. We were able to determine that, in at least some small stream systems, a wave of silt and sand (akin to a small tsunami) moved down these waterways. As a result tens of thousands of tonnes of silt and sand entered the Avon and Heathcote rivers. The exact mechanism by which this sediment entered the streams is unclear. Anecdotal observations suggest that at least some silt erupted in the streambeds and small silt ‘volcanoes’ were present post-earthquakes in several streams. Large quantities of silt and sand almost certainly entered the streams via the stormwater system and, in some areas, overland run-off may have occurred (although there was little visual evidence for this). Regardless of the source and pathways for its addition to the waterways, the result was an overwhelming pulse and subsequent press disturbance at a scale well beyond anything that had been recorded in these systems.
The liquefied silt and sand was almost entirely inorganic (99%) and essentially devoid of pollutants (e.g. metals). As a result the main effect of this disturbance has probably been through smothering, habitat removal and clogging of interstitial spaces. Much of the algae and macrophytes in these waterways were completely smothered, as probably were many of the benthic invertebrates.
All stream reaches in this study were in a heavily urbanised area and so benthic communities were degraded prior to the earthquakes (Winterbourn et al. Citation2007). Unfortunately, we have virtually no pre-earthquake biological data on the medium and heavily silted reaches, so we cannot confidently determine the degree of impact at individual sites. Our data does, however, show that compared to other sites which had little or no liquefied silt, benthic invertebrate diversity, metrics of invertebrate stream health and community composition were all significantly different. These results are consistent with other studies on the effects of sediment on benthic communities. Angradi (Citation1999) experimentally added sediment from 5%–30% cover and found EPT richness and benthic invertebrate density were negatively affected by sediment, while Burdon et al. (Citation2013) showed that fine sediment cover of > 20% markedly reduced EPT richness.
Reduced fish diversity is consistent with the findings of Chen et al. (Citation2004) who measured the response of fish abundance to sediment input as a result of landslides caused by the Chi Chi earthquakes in Taiwan in 1999 and a subsequent typhoon. Collier (Citation2002) investigated the effect of managed sediment flushing from a dam after a volcanic eruption deposited fine sediment into a river and found that benthic taxonomic richness and biomass decreased significantly after post-eruption flushing. However, this study concluded that hydraulic effects rather than sediment might have been responsible for these decreases. Over the time of our study no large storm events or extreme changes in discharge (apart from the liquefaction) occurred in our study sites. All sites were also upstream of sites receiving emergency sewage discharges. Thus we conclude that reductions in invertebrate and fish richness and density were almost certainly due to siltation causing smothering, habitat loss and depletion of food resources rather than other impacts.
Physical disturbance is a natural process which all ecosystems experience over geological time. Therefore, communities within most ecosystems might be expected to have adapted to some level of disturbance. In New Zealand, the dominance of mountainous terrain and associated precipitation results in stream systems which can be subjected to frequent flood disturbance (Scrimgeour & Winterbourn Citation1989). Furthermore, changing climatic conditions in conjunction with changes associated with human land and water use has led to more streams experiencing droughts or ephemeral flows. Winterbourn et al. (Citation1981) suggested that New Zealand's stream invertebrate communities are comprised of ecologically flexible species with poorly synchronised life histories because of the temporally unpredictable nature of our streams. It might therefore be expected that some New Zealand taxa might recover relatively quickly from disturbance events. However, the magnitude of disturbance documented in this study, and the fact that these urban streams already had depauperate and degraded communities prior to these events, may mean that their ability to recover is severely reduced.
The ability of smaller tributaries to flush heavy sediments is limited. These types of streams are often spring-fed and low gradient with relatively stable flows and, despite high flows from stormwater discharges, our observations indicate that without active human intervention this earthquake-derived silt may take many years to be flushed through these systems. We would contend that although the earthquake was a natural event, the extent of siltation in these streams has been greatly exacerbated via the flow of silt and sand from the stormwater network. As a consequence, the silt loads in many parts of the river network are artificially deep and without intervention the recovery of benthic invertebrate and fish communities is likely to be significantly delayed.
Acknowledgements
Thanks to Steve Pohe for assistance in the field and Hayley Stoddart who processed the benthic invertebrate samples. Milen Marinov conducted the GIS analysis. Funding to PGJ by NIWA under Freshwater and Estuaries Programme 2, Sustainable Water Allocation (2013/14 SCI), assisted with manuscript preparation.
References
- Angradi TR 1999. Fine sediment and macroinvertebrate assemblages in Appalachian streams: a field experiment with biomonitoring applications. Journal of the North American Benthological Society 18: 49–66. 10.2307/1468008
- Burdon F, Harding JS, McIntosh AR 2013. Habitat loss drives threshold response of benthic invertebrate communities to deposited sediment in agricultural streams. Ecological Applications 23: 1036–1047. 10.1890/12-1190.1
- Chen L-H, Chu KC-M, Chiu Y-W 2004. Impacts of natural disturbance on fish communities in the Tachia River, Taiwan. Hydrobiologia 522: 149–164. 10.1023/B:HYDR.0000029992.15038.31
- Christchurch City Council 2010. Facts, stats & figures reporting sheet. Canterbury, Christchurch City Council.
- Clapcott JE, Young RG, Harding JS, Matthaei CD, Quinn JM, Death RG 2011. Sediment assessment methods: protocols and guidelines for assessing the effects of deposited sediment on in-stream values. Nelson, Cawthron Institute.
- Collier KJ 2002. Effects of flow regulation and sediment flushing on instream habitat and benthic invertebrate in a New Zealand river influenced by a volcanic eruption. River Research and Applications 18: 213–226. 10.1002/rra.666
- Davies PE, Nelson M 1994. Relationships between riparian buffer widths and the effects of logging on stream habitat, invertebrate community composition and fish abundance. Australian Journal of Marine & Freshwater Research 45: 1289–1305. 10.1071/MF9941289
- Grimm NB, Fisher SG 1989. Stability of Periphyton and Macroinvertebrates to disturbance by flash floods in a desert stream. Journal of the North American Benthological Society 8: 293–307. 10.2307/1467493
- Harding JS 2003. Historic deforestation and the fate of endemic species in streams. New Zealand Journal of Marine and Freshwater Research 37: 333–345. 10.1080/00288330.2003.9517171
- Harding JS, Benfield EF, Bolstad PV, Helfman GS, Jones EBD 1998. Stream biodiversity: the ghost of land-use past. Proceedings of the National Academy of Sciences 95: 14843–14847. 10.1073/pnas.95.25.14843
- Harding JS, Clapcott JE, Quinn JM, Hayes JW, Joy MK, Storey RG et al. 2009. Stream habitat assessment protocols for wadeable rivers and streams of New Zealand. Christchurch, University of Canterbury. 133 p.
- Jowett IG, Richardson J 1990. Microhabitat preferences of benthic invertebrates in a New Zealand river and the development of in-stream flow-habitat models for Deleatidium spp. New Zealand Journal of Marine & Freshwater Research 24: 19–30. 10.1080/00288330.1990.9516399
- Lake PS 2000. Disturbance, patchiness and diversity in streams. Journal of the North American Benthological Society 19: 573–592. 10.2307/1468118
- Mihuc TB, Minshall GW 1995. Trophic generalists vs. trophic specialists: implications for food web dynamics in post-fire streams. Ecology 76: 2361–2372. 10.2307/2265813
- Pfankuch DJ 1975. Stream reach inventory and channel stability evaluation. Missoula, MT, US Department of Agriculture & Forest Service, Northern Region.
- Resh VH, Brown AV, Covich AP, Gurtz ME, Li HW, Minshall GW et al. 1988. The role of disturbance in stream ecology. Journal of the North American Benthological Society 7: 433–455. 10.2307/1467300
- Scrimgeour GJ, Winterbourn MJ 1989. Effects of floods on epilithon and benthic invertebrate populations in an unstable New Zealand river. Hydrobiologia 171: 33–44. 10.1007/BF00005722
- Stark JD 1985. A Macroinvertebrate Community Index of water quality for stony streams. Water and Soil Miscellaneous Publication 87. Wellington, National Water and Soil Conservation Authority. 53 p.
- Stark JD, Boothroyd IK, Harding JS, Maxted JR, Scarsbrook MR 2001. Protocols for sampling macroinvertebrates in wadeable streams. New Zealand Macroinvertebrate Working Group Report No. 1. Wellington, Ministry for the Environment. 57 p.
- Wells NS, Clough TJ, Condron LM, Baisden WT, Harding JS, Dong Y et al. 2013. Biogeochemistry and community ecology in a spring-fed urban river following a major earthquake. Environmental Pollution 182: 190–200. 10.1016/j.envpol.2013.07.017
- Winterbourn MJ, Gregson KLD, Dolphin CH 2000. Guide to aquatic insects of New Zealand. 3rd edition. Auckland, Bulletin of the Entomological Society of New Zealand.
- Winterbourn MJ, Harding JS, McIntosh AR 2007. Response of the benthic fauna of an urban stream during six years of restoration. New Zealand Natural Sciences 32: 1–12.
- Winterbourn MJ, Rounick JS, Cowie B 1981. Are New Zealand stream ecosystems really different? New Zealand Journal of Marine & Freshwater Research 15: 321–328. 10.1080/00288330.1981.9515927
- Xu M, Wang Z, Qi L, Liu L, Zhang K 2011. Disaster chains initiated by the Wenchuan earthquake. Environmental Earth Science 65: 975–985. 10.1007/s12665-011-0905-3
- Young R, Smart G, Harding JS 2004. Impacts of hydro-dams, irrigation schemes and river control works. In: Harding JS, Mosley P, Pearson C, Sorrell B eds. Freshwaters of New Zealand. Christchurch, New Zealand Limnological and Hydrological Societies. Pp. 37.1–37.15.